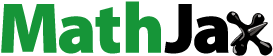
Abstract
Zebra Mussels (Dreissena polymorpha) were introduced into the US in the 1980s and have since spread throughout the country with concomitant impacts on aquatic ecosystems. We placed 20 m transects in a variety of sediment types and collected data on sediment size, mussel coverage (the percent of the bottom covered by mussels), and the presence of macrophytes within 0.25 m2 quadrats in White Bear Lake, MN. To assess the degree of Zebra Mussel aggregation, we calculated Morisita’s index of dispersion (Iδ) at four levels of spatial scale − 0.25 m2, 1 m2, 4 m2, and 40 m2. Understanding the pattern of aggregation in Zebra Mussels is crucial in detecting early invasions, monitoring populations, and attempting to eradicate them. There was greater Zebra Mussel coverage within quadrats with coarser substrates (gravel/cobble and mixed sediment – including rock and boulders) compared to those with finer substrates (silt and sand). Macrophyte coverage did not significantly influence Zebra Mussel coverage on the lake bed sediments. Spatial scale had a large impact on the measure of mussel aggregation. Zebra Mussels in sand and silt had the highest degree of aggregation and high levels of macrophytes resulted in greater degrees of aggregation on the lake bed sediments. Zebra mussel coverage measured at larger spatial scales generally resulted in lower aggregation values, across both sediment types and levels of macrophyte coverage. The appropriate selection of spatial scale for sampling or observation is critical when upscaling local density to lake populations.
KEY POLICY HIGHLIGHTS
Zebra Mussels have spread throughout the US and significantly alter aquatic ecosystems.
Zebra mussels are often aggregated on the bottom of aquatic systems and are found preferentially on lake beds with coarser material at the bed (gravel and rock).
Using the correct spatial scale to assess mussel coverage is crucial for accurate population estimates.
Zebra Mussel infestations might be managed by partial-lake treatment options; however, treatment areas need to be chosen based on Zebra Mussel density and distribution patterns. Knowledge of the relationship between spatial scale, sediment type, macrophyte availability and population coverage and aggregation is needed for these types of treatment programs.
Introduction
Dreissenids (Zebra Mussels (Dreissena polymorpha) and their congener Quagga Mussels (Dreissena bugensis)) were introduced into the Great Lakes in the US in the mid-to-late-1980s presumably through the transport of ballast water from eastern Europe and southeast Russia (Mackie and Schloesser Citation1996; McMahon Citation1996; Carlton Citation2008). They are spreading across North America (Strayer Citation2009) and have had a significant negative economic impact through clogging water intake pipes, affecting commercial fisheries,and shipping (Pimentel et al. Citation2005; Karatayev et al. Citation2007) and also have impacted ecosystems by shifting planktonic food webs to littoral food webs in lentic systems and influencing benthic communities in streams and rivers (Nalepa et al. Citation2003; Ward and Ricciardi Citation2007; Pejchar and Mooney Citation2009; Strayer Citation2009; Higgins and Vander Zanden Citation2010). Once established, Zebra Mussel colonies can reach densities of thousands of individuals per square meter within a few years (Cope et al. Citation2006, Kumar et al. Citation2016; Bossenbroek et al. Citation2007) and experience dramatic population swings (Strayer et al. Citation2011, Citation2019).
Many studies have examined the influence of sediment composition on the distribution of dreissenids (Bially and Macisaac Citation2000; Chakraborti et al. Citation2002). While early studies suggested that hard or rocky substrates are needed for the success of dreissenid colonization (Smit et al. Citation1993; Nalepa et al. Citation1995) more recent studies have found dreissenids colonizing soft sediments (Bially and Macisaac Citation2000; Chakraborti et al. Citation2002) and organic substrates including macrophytes (Londo et al. Citation2022). Ferry (Citation2013) found that Zebra Mussels selected for hard substrates (e.g. rocks) and avoided soft substrates (e.g. silt and sand). They also used macrophytes in proportion to their availability.
There are several factors that can influence the aggregation (i.e. the spatial distribution and clustering) of Zebra Mussels including habitat, behavior, and hydrodynamics that influence veliger settling (Wainman et al. Citation1996; Kobak et al. Citation2010, Tošenovský and Kobak Citation2015; Hasler et al. Citation2019). It has been suggested that Zebra Mussels may prefer forming colonies due to their active preference for conspecifics (Wainman et al. Citation1996; Kobak Citation2006). Dzierżyńska-Białończyk et al. (Citation2018) suggest that the aggregation of Zebra Mussels is a response to a lack of other suitable substrates rather than behavioral cues. Czarnołeski et al. (Citation2004) found that the density of settling recruits was higher on topographically complex rather than flat surfaces. Coakley et al. (Citation1997) hypothesized that clustering of Zebra Mussels into ‘stripes’ in soft substrates was likely due to the eddies and currents carrying them from hard-bottomed areas to soft substrates by storm-induced current- or ice-scour processes.
Understanding how populations are distributed and the scales at which population aggregation can be detected is important in developing appropriate sampling regimes to track changes in populations over time and space (Levin Citation1992; Hayes and Castillo Citation2017). Understanding the pattern of aggregation in Zebra Mussels is crucial in detecting early invasions and attempting to eradicate them. The measurement of the patterns of aggregation is dependent on the spatial scale at which populations are assessed (Levin Citation1992; Krebs Citation1999; Hayes and Castillo Citation2017). Spatial variability in homogenous environments tends to be a function of the scale at which the analysis takes place (Levin Citation1992). As the scale increases the spatial variability will tend to decrease (Wiegert Citation1962; Levin Citation1992; Golay et al. Citation2014). Measures of aggregation, such as Morisita’s index of dispersion (Morisita Citation1959; Krebs Citation1999) are often used to measure how individuals in populations are distributed (regular, random, or clumped). The density of populations that are regularly distributed have a variance/mean ratio <1, those randomly distributed have a variance/mean ratio = 1, and clumped populations have a variance/mean ratio >1 (Horne and Schneider Citation1995). Wimbush et al. (Citation2009) reported success in using the removal of a large fraction of the early invaded population of Zebra Mussels in a lake by SCUBA. The success of this removal was dependent on the early detection of the Zebra Mussels and knowledge of optimal habitats and clustering of the population.
Dreissenids were first detected in Minnesota in Lake Superior in 1989, and from the south through the Mississippi River in 1993 (Minnesota Aquatic Invasive Species Research Center, https://maisrc.umn.edu/zebra-mussels, accessed July 1, 2022). Quagga Mussels are currently restricted to Lake Superior and the St. Louis River, a Lake Superior tributary (https://nas.er.usgs.gov/queries/greatlakes/FactSheet.aspx?Species_ID=95&Potential=N&Type=0; assessed July 4, 2022). Zebra Mussels moved to inland lakes in Minnesota with the Minnesota Department of Natural Resources (MN DNR) listing 232 bodies of water as infested in the state by 2021 (https://www.dnr.state.mn.us/invasives/ais/infested.html#:∼:text=Less%20than%204%25%20of%20Minnesota,zebra%20mussels%20have%20been%20found.; accessed July 1, 2022).
White Bear Lake is a 978 ha lake in a suburb of the Twin Cities (St. Paul and Minneapolis), Minnesota. An assessment of the invasibility of the lake to Zebra Mussels was carried out in 2009 (McComas and Stuckert Citation2009). The report suggested that Zebra Mussels would be able to sustain long-term moderate growth but that optimal conditions for growth would be limited due to a lack of ideal substrate. They also concluded that 32% of the lake bottom consisted of substrate that was not suitable for Zebra Mussels (sand and silt), while 60% was suboptimal (sand) and 8% was considered optimal (gravel and rock). In addition, vertical substrates (plants, boat lifts, etc.) could provide additional habitat for colonization estimated as 46% of the bottom habitat. Zebra Mussels were first found in White Bear Lake in 2014 (Invasive Species Program Citation2015). The Minnesota Department of Natural Resources (MN DNR) has been assessing Zebra Mussel density at four transects in White Bear Lake in a variety of substrates since 2015 (personal communication April Londo MN DNR). The density was below 100 Zebra Mussels/m2 in 2015 and 2016, peaked at 4200 Zebra Mussels/m2 in 2017, and leveled off to approximately 1000–2000 Zebra Mussels/m2 in 2018–2020.
The purpose of this study was to examine the relationship between habitat types (substrate type and macrophyte presence) and the relative coverage and aggregation of live Zebra Mussels in White Bear Lake.
Methods
White Bear Lake is a mesotrophic lake located in a suburb of the Twin Cities, MN. It has a mean depth of 6 m and a maximum depth of 25 m () (https://www.dnr.state.mn.us/lakefind/lake.html?id=82016700, accessed July 9, 2022). We deployed 21 transect lines, each 20 m in length. Sampling occurred from June 15 to June 24, 2021. We specifically chose sites that would include a range of different substrate types and Zebra Mussel coverage to create a training dataset to relate acoustic data to substrate and mussel coverage. SCUBA divers placed 1-m2 quadrats subdivided into 0.25-m2 sections on each side of the transect line. The divers surveyed each 0.25-m2 section noting the percentage of the bottom covered by live and dead Zebra Mussels, submerged macrophyte percentage, and substrate type (silt, silt/sand mix, sand, gravel, cobble, and rock/boulder). In addition, divers noted the presence of live Zebra Mussels at the base of any macrophytes in the sampling area. Zebra Mussel coverage was not surveyed on vertical macrophyte surfaces. After recording the data the 1-m2 quadrats were moved one meter along the transect and the data collection process was repeated. This resulted in 40 1-m2 quadrats and 160 0.25-m2 sections sampled along each transect.
Figure 1. Map of the sampling area with transect locations. The percent coverage of Zebra Mussels is the mean for the transect.
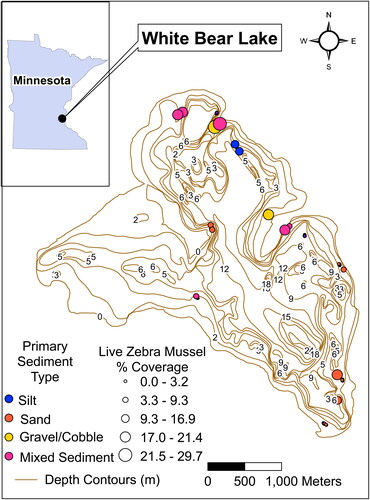
We classified the percent coverage of the six sediment types into four categories based on the visual examination of the distribution of sediment types. Sediment categories included silt (≥ 60% silt or ≥80% sand/silt mix), sand (≥80% sand), gravel/cobble (≥40% gravel or ≥40% cobble), and mixed sediment (rocks and boulders along with sand or a mix of sand, gravel, and cobble). Discriminant analysis on the four sediment categories was used to assess how well they were predicted based on the % composition data. We also combined the percent macrophyte coverage into 3 categories: low ≤ 10%, medium 10-30% and high > 30%.
We calculated Morisita’s index of dispersion (Iδ) at different spatial scales (Morisita Citation1959; Krebs Citation1999):
where,
(e.g. 0.25, 1, 4 or 40 m2 unit),
Morisita’s index provides a measure of the aggregation of the Zebra Mussels. An Iδ = 1 indicates a random distribution, <1 a uniform distribution, and >1 a clumped distribution. χ2 values were calculated to test for the difference in the significance of the index from 1 (Krebs Citation1999). We calculated Iδ based on the 0.25 m2 scale and then at the 1 m2, 4 m2 (2 contiguous 1-m2 quadrats on each side of the transect line), and at the transect scale (40 m2 – the 40 1 m2 quadrats along a transect). We plotted spatial scale versus Iδ to assess how sampling size influences our understanding of the distribution of Zebra Mussels.
To examine what factors might influence the degree of aggregation of Zebra Mussels we calculated the mean coverage of Zebra Mussels at the 4 spatial scales − 0.25 m2, 1 m2, 4 m2, and 40 m2. We then calculated Iδ at each of these spatial scales for the 4 sediment types we found and for the 3 levels of macrophyte coverage.
All statistical analyses were conducted with JMP Pro v 16.0.0 (SAS Institute, Cary, NC). Since the data were not normally distributed we used nonparametic Kruskal-Wallis tests to examine the impacts of sediment size and macrophyte coverage on the percent live Zebra Mussel coverage.
Results
Zebra Mussel coverage
Of the 3288 0.25 m2 quadrats that we sampled, 59.6% contained live Zebra Mussels and 46.6% contained dead Zebra Mussels. A contingency analysis indicated that the presence of live and dead Zebra Mussels were related (Likelihood Ratio = 837.2, p < 0.0001). A Fisher’s Exact test indicated that the probability of finding dead Zebra Mussels was greater if live Zebra Mussels were present (85% of the time live Zebra Mussels were present dead Zebra Mussels were found). There was a small, but statistically significant, positive correlation between the coverage of live and dead Zebra Mussels in the 0.25 m2 quadrats (r2 = 0.23, F1,3287 = 989.3, p < 0.0001). The mean coverage of live Zebra Mussels was 11.4% (std. dev. = 12.9%; range 0-80%) while it was 8.9% (std. dev. = 13.6%; range 0-90%) for dead Zebra Mussels in the 0.25 m2 quadrats. Further analysis focused on the live Zebra Mussel coverage since dead Zebra Mussels could easily be transported by water currents to a variety of locations.
The 6 sediment types for which we recorded percent composition (silt, silt/sand mix, sand, gravel, cobble, and rock/boulder) were classified into 4 categories based on an examination of the distribution of sediment types: silt, sand, gravel/cobble, and mixed sediment. Discriminant analysis indicated that our 4 categories were correctly predicted 95.5% of the time based on the original 6 types for which we collected percent composition data. A nonparametic Kruskal-Wallis test indicated there was a significant effect of substrate size on percent live Zebra Mussel coverage - = 985.9, p < 0.0001). Over 50% of the quadrats with sand or silt sediment had no live Zebra Mussels (). On the other hand, greater than 90% of quadrats with a substrate of either gravel/cobble or mixed sediments (which included rocks and boulders) had live Zebra Mussels. Consequently, Zebra Mussel coverage was much greater in gravel/cobble and mixed substrate than in sand or silt ().
Only about 30% of quadrats with low or medium coverage of macrophytes had live Zebra Mussels on the bottom substrate, while 48% of quadrats with high coverage of macrophytes had live Zebra Mussels (). Of the quadrats with silt, only 6% had medium or high levels of macrophytes. Sand substrates had the greatest coverage of macrophytes (49% with medium or low amounts), with gravel/cobble and mixed sediments having approximately 30-32% medium or high macrophyte coverage. There was no significant difference ( = 3.6, p = 0.17) in the percent Zebra Mussel coverage between the three levels of macrophyte coverage (). While we did not quantify the level of colonization of Zebra Mussels on macrophytes, we found that only 28% of the quadrats that had macrophytes harbored live Zebra Mussels on the lake bed.
Zebra Mussel aggregation and scale
Zebra Mussels were aggregated at all scales calculated (Iδ was statistically significantly > 1 at all scales). As the spatial scale increased, Iδ decreased ().
We examined whether Zebra Mussel aggregation varied among sediment types and spatial scales. Iδ was greatest for silt and sand and lowest for gravel/cobble and mixed sediment (). For silt, sand, and gravel/cobble, Iδ decreased with increasing spatial scale, similar to what was found when all data were analyzed together (). For mixed sediments, Iδ was low and was constant across spatial scales ().
Quadrats with low or medium macrophyte coverage had similar Iδ values, while those with high macrophyte coverage had higher levels of Iδ (). For both low and medium macrophyte coverage, Iδ declined with increasing spatial scale, while the trend was not as clear for those quadrats with high macrophyte coverage.
Discussion
While in many locations Zebra Mussels are found in large congregations called druses (Dzierżyńska-Białończyk et al. Citation2018), in White Bear Lake the vast majority of Zebra Mussels were found as individuals or as small clumps spread across the bottom. Sediment type influenced both the level of live Zebra Mussel coverage and the degree of aggregation. Coarser substrates had a greater Zebra Mussel coverage, but they had a lower degree of aggregation. Sand had the highest degree of aggregation at the 0.25 m2 scale while silt had the highest degree at the 1 m2 scale. Macrophyte coverage did not significantly influence Zebra Mussel coverage on the bottom but areas with high macrophyte coverage tended to have a greater degree of Zebra Mussel aggregation. While Live Zebra Mussels could potentially use dead Zebra Mussel shells as an attachment site (Depew et al. Citation2021), there was only a small positive relationship between live and dead Zebra Mussel coverage.
When Zebra Mussels began to colonize North America the general consensus was they only colonized hard substrates to which they could attach their byssal threads (Karatayev et al. Citation1998). There continues to be suggestions that when fine substrates are found in deeper portions of a lake or in areas of physical disturbance, Zebra Mussels are limited to harder and larger substrates (Depew et al. Citation2021). When McComas and Stuckert (Citation2009) examined White Bear Lake before Zebra Mussel invasion, they used these assumptions to conclude that 32% of the bottom of White Bear Lake consisted of substrate that was not suitable for Zebra Mussels (sand and silt), while 60% was suboptimal (sand) and 8% was optimal (gravel and rock). Other studies have found that Zebra Mussels can colonize soft sediments as well as hard sediments (Berkman et al. Citation1998; Bially and Macisaac Citation2000; Chakraborti et al. Citation2002). In our study, there was a greater Zebra Mussel coverage on the lake bottom in areas of coarser substrates including gravel, cobble and mixed sediments that contained rocks and boulders. We found similar levels of coverage of live Zebra Mussels in sand and silt, but coverage was less than in areas with coarser substrates. Londo (Citation2015), examining four west-central lakes in MN, with mainly gravel, sand, and silt sediments, found that a majority of sampling sites with Zebra Mussels had substrate sizes of 0-1 phi units, indicative of sand substrates. She found a strong, but not statistically significant (p = 0.054) relationship between larger sediment sizes (gravel and cobble) and Zebra Mussel density. Sites with very small sediment sizes (silt) had very few Zebra Mussels, generally less than 2000 Zebra Mussels per m2. Berkman et al. (Citation1998) found very low coverage of Zebra Mussels in silt (generally less than 15%) while sand had more coverage (∼20-30%). In our study both silt and sand sites had coverage at 10% or lower in the 0.25 m2 quadrats. The presence of gravel as a habitat in White Bear Lake may provide an opportunity for greater colonization in those areas. The differentiation between sand and gravel may be important in estimating Zebra Mussel density. While Haltuch et al. (Citation2000), using side scan sonar, identified sand/gravel as the habitat with the greatest density of Zebra Mussels in fine sediment habitats in Lake Erie; the greater Zebra Mussel coverage in gravel/cobble substrates and the greater degree of aggregation in sandy substrates in White Bear Lake suggests that it may be advantageous to use a smaller spatial scale for investigation of substrate type and Zebra Mussel aggregation.
Though organic materials can serve as a substrate for Zebra Mussels (Londo et al. Citation2022) we found that only 20-30% of quadrats had live Zebra Mussels on macrophytes at the lake bed. We did not measure Zebra Mussel coverage on macrophytes in the water column. Stańczykowska and Lewandowski (Citation1993) suggested that macrophytes support higher densities of Zebra Mussels than either sand or silt. However, the density of Zebra Mussels supported on macrophytes varies by species (Lewandowski and Ozimek Citation1997; Zhu et al. Citation2007). McComas and Stuckert (Citation2009) estimated that vertical substrates (plants, boat lifts, etc.) in White Bear Lake could provide a habitat for colonization equal to about 46% of the bottom habitat. Despite that prediction, we noted only 21% of the 0.25 m2 quadrats had Zebra Mussels at the base of macrophytes and likely did not provide a significant amount of habitat for them although Zebra Mussels might be colonizing portions of the macrophytes we did not examine. Additionally it was early in the reproductive season and we may not have been able to detect newly settled Zebra Mussels.
To better understand the differences in Zebra Mussel coverage between sediment types we examined the degree of aggregation. There could be several reasons why Zebra Mussels might be found in clumps. One could be a selection for specific substrates, another could be an active response to be near conspecifics. Dzierżyńska-Białończyk et al. (Citation2018) found that Zebra Mussels tended to avoid conspecifics at high densities if alternative substrate was available. However, if the substrate was less than optimal (e.g. sand) mussels tended to stay in their initial positions, not moving towards or away from conspecifics. They suggested that dense mussel aggregations are formed due to the lack of alternative attachment sites and not behavioral responses. Aggregated mussels may move less, and attach to the substratum more strongly, than single Zebra Mussels (Kobak et al. Citation2009). Burlakova et al. (Citation2006) found that Zebra Mussel distribution within the three lakes they studied was quite patchy at first but patchiness decreased over time after initial colonization. They attributed this to the saturation of suitable substrates. They also indicated that Zebra Mussels tend to reach maximum density 2-3 years after populations are large enough to be detected. Since Zebra Mussels invaded White Bear Lake in 2014, density peaked in 2017 at approximately 2000–5000 Zebra Mussels/m2 and has leveled off at approximately 1000–3000 Zebra Mussels/m2 through 2020 (April Londo, MN DNR, personal communication). Thus, the level of aggregation we observed may be indicative of a stable Zebra Mussel population state in White Bear Lake.
It is important to consider the spatial scale at which the determination of aggregation takes place (Levin Citation1992; Hayes and Castillo Citation2017). We found that Iδ decreased with increasing scale. Okuda et al. (Citation1997) found a similar pattern in tree distribution in a lowland tropical forest and Ueno et al. (Citation2002) found a similar trend when studying a broad-leaved evergreen but neither study indicated why this might be occurring. Golay et al. Citation2014, point out that when the quadrat size is plotted against Iδ, every value of Iδ would fluctuate around 1 if individuals are randomly distributed. If however individuals are clumped and a small quadrat was used a large number of empty quadrats would result in a large dispersion index. However, with larger quadrat sizes the number of empty quadrats would be lower and thus the dispersion index would decrease. Levin (Citation1992) states that in homogeneous environments, variability will be a function of the spatial scale with variability decreasing with increasing spatial scale. He suggests that on a log-log plot this would provide a line with a slope between 0 and −1. A slope of 0 indicates a perfect spatial correlation, i.e. where the level of coverage in one area is correlated with that in other areas and thus low levels of clumping, and a slope of −1 indicates no spatial correlation and thus a random distribution. In our study, the slope was −0.07 indicating some level of aggregation of Zebra Mussels. The level of aggregation was greatest in fine sediments.
Zebra Mussel distribution was more aggregated (higher Iδ values) in sand and silt than in gravel/cobble and mixed sediments. Tošenovský and Kobak (Citation2015) in an experimental study, found that Zebra Mussels tended to form more clusters on sand than on solid substratum, but the difference was not statistically significant. Kobak and Ryńska (Citation2014) however did find Zebra Mussels aggregating more on sand than on hard substrates. This behavior could partially explain the higher level of aggregation at the 0.25 m2 level. The difference in aggregation levels between sediments is likely due to a greater number of quadrats with 0 Zebra Mussels in silt and sand compared to the other sediment types. For all of the substrates except for mixed sediment there was a decrease in Iδ with increasing scale. This indicates that the distributions are clumped. For the mixed sediment category, the relatively low Iδ and the fact that increasing the sampling area does not result in a decrease in the dispersion index could mean that these sediments are at their carrying capacity. In our study, mixed sediment did have a high live Zebra Mussel coverage, although not as high as that in gravel/cobble sediment. At the 0.25 m2 scale, mixed sediment had the lowest coefficient of variation (standard deviation/mean) suggesting that the quadrats were more uniform.
While the density of Zebra Mussels and the coverage of the bottom seems to confirm McComas and Stuckert (Citation2009) prediction that White Bear Lake provides suboptimal habitat for Zebra Mussels, these non-native animals appear to be having significant impacts on the ecology of the lake. Historically there were three species of native mussels (unionids) found in White Bear Lake: Lampsilis siliquoidea, Pyganadon lacustris, and P. grandis (Neira et al. Citation2022). Divers were able to collect 198 native mussels in 190 min in 2013 (Mark Hove, UMN and Bernard Sietman MN DNR, personal communication). During our dives, no living or dead unionids were observed. From the mid-1970s until the early 2000s, Secchi disc transparency increased in White Bear Lake from 1-3 m to 4-5 m. During this time there was a significant decrease in total phosphorus in the lake, from 20-40 μg/L to 12-20 μg/L. Since 2014 the water transparency has shown an increasing clarity reaching an average of approximately 6.8 m in 2021 (https://webapp.pca.state.mn.us/cmp/stations/82-0167-00-221/trends; accessed July 12, 2022). While we cannot say with certainty that the increase in water clarity is the result of a reduction in phytoplankton due to Zebra Mussels, the trend of increasing water clarity coincident with Zebra Mussel infestation is common in other systems, especially in shallow lakes (Makarewicz et al. Citation1999; Cha et al. Citation2013; Rowe et al. Citation2015). Competition for food between native mussels and Zebra Mussels, resulting from the reduction of food resources, likely played a key role in the loss of native mussels in White Bear Lake (Baker and Hornbach Citation1997; Baker and Levinton Citation2003; Higgins and Vander Zanden Citation2010; Beason and Schwalb Citation2022).
As of November 2021, there were over 230 lakes in MN that have been declared as infested with Zebra Mussels by the MN DNR, although they have confirmed sightings in 270 lakes (https://www.dnr.state.mn.us/invasives/ais/infested.html#:∼:text=Less%20than%204%25%20of%20Minnesota,zebra%20mussels%20have%20been%20found; accessed July 26, 20222). It appears that in lakes with only modest amounts of hard (coarse) substrates, Zebra Mussels can colonize sub-optimal substrates and maintain small but consequential self-sustaining populations. It has been suggested that with early detection and the development of a swift response plan to infestation, eradication of Zebra Mussel populations in lakes with sub-optimal habitats may be possible (Wimbush et al. Citation2009). Knowledge of the level of Zebra Mussel coverage requires sampling at the appropriate spatial scale (Ferguson et al. Citation2019) and thus knowledge of the degree of aggregation is crucial for accurate estimates of coverage. Lund et al. (Citation2018) suggested that for early response, partial-lake treatment options to manage Zebra Mussel infestations, treatment areas need to be chosen based on Zebra Mussel density and distribution patterns. Knowledge of the relationship between spatial scale, sediment type, macrophyte availability and population coverage and aggregation is needed for these types of treatment programs.
Acknowledgments
Thanks to Chris Rounds from the University of Minnesota for help with field collections. We also acknowledge the assistance and input of Justin Townsend, Environmental Resources Specialist, with Ramsey County.
Disclosure statement
No potential conflict of interest was reported by the authors.
Data availability statement
The data that support the findings of this study are openly available in the Data Repository for the University of Minnesota at https://conservancy.umn.edu/drum.
Additional information
Funding
Notes on contributors
Daniel J. Hornbach
Daniel Hornbach is an aquatic ecologist and the John S. Holl Professor of Environmental Studies at Macalester College. He received his B.S. and M.S. in Biology from the University of Dayton and his Ph.D in Zoology from Miami University, Oxford, OH. He has published numerous articles on the biology and ecology of freshwater mussels.
Jessica L. Kozarek
Jessica Kozarek is a research associate at St. Anthony Falls Laboratory of the University of Minnesota, She received her B.S. from Penn State and her M.S. and Ph.D. from Virginia Tech. She conducts experiments linking physical, chemical and biological processes in streams and rivers in the lab, the field, and in the Outdoor StreamLab, a field-scale stream and floodplain experimental facility. Her research interests are in the areas of stream restoration and management; ecohydraulics; interactions between flow, sediment, and in-stream biota; use of hydraulic models to develop restoration guidance; and water quality and nutrient dynamics.
Naomi Blinick
Naomi Blinick is a researcher in the department of Fisheries, Wildlife, and Conservation Biology at the University of Minnesota. She has an MS in Fisheries and Aquatic Biology from the University of Minnesota and a BA in Marine Studies from Prescott College in Arizona. Her research focuses on aquatic invasive species impacts on native organisms and exploring effective management strategies.
Mark C. Hove
Mark Hove is a research biologist at the University of Minnesota and Macalester College. He has a B.S. from the University of Minnesota and a M.S. from Virginia Polytechnic Institute and State University. He has conducted research on the interactions between freshwater mussels and their host fishes. He has also worked closely with a number of state and federal agencies to assist in the conservation of freshwater organisms.
Paul Grams
Paul Grams is a geomorphologist at the Southwest Biological Science Center’s, Grand Canyon Monitoring and Research Center. He has a BA in Geology from Middlebury College an M.S. in Geology from Utah State University and a Ph.D. in Geography and Environmental Engineering from Johns Hopkins University. He manages a set of projects collectively called the River Geomorphology and Geomorphic Change project. These include a range of studies that describe, quantify, and predict geomorphic change, mostly on large rivers in the western United States.
Matt Kaplinski
Matt Kaplinski is a geomorphologist at the Southwest Biological Science Center’s, Grand Canyon Monitoring and Research Center specializing in the application of multibeam sonar to inland rivers and lakes. He has spent over 30 years working in Grand Canyon as a research scientist and river guide. He has a B.S. from Western Michigan University and an M.S. from Northern Arizona University. His current research is focused on studying the effects of flows from Glen Canyon Dam on Colorado River sediment resources.
Kelly R. MacGregor
Kelly MacGregor is a Professor of Geology at Macalester College. Her academic area of expertise is glacial geomorphology of alpine systems, with a focus on how temperate glaciers erode and shape mountainous landscapes. This research extends to sediment transport and deposition in proglacial and post-glacial rivers and lakes. She works with an interdisciplinary team of ecologists and engineers examining feedbacks between sediment dynamics and native & invasive mussel populations in Minnesota rivers and lakes. She has a B.A. in Geology from Williams College and a Ph.D. in Earth Sciences from the University of California, Santa Cruz.
Chris Milliren
Chris Milliren is an associate engineer at the St. Anthony Falls Laboratory of the University of Minnesota. He has experience designing and building remote monitoring systems, human machine interface (HMI) systems, and motion control. At SAFL, Chris has designed and built remote sensor systems for wind turbine foundation structural health monitoring, designed and built a portable anechoic chamber for researchers to use while assessing the hearing capabilities of bald eagles, and engineered and fabricated other custom equipment for laboratory research. He has a B.C.E. degree in Civil Engineering from the University of Minnesota.
Andrew T. Riesgraf
Andrew T. Riesgraf is a researcher at the St. Anthony Falls Laboratory of the University of Minnesota. He holds a B.S. in Fisheries, Wildlife, and Conservation Biology and a M.S. in Fisheries and Aquatic Biology from the University of Minnesota. He has years of experience conducting research focused on freshwater lake and river ecosystems, including various aquatic invasive species.
References
- Baker SM, Hornbach DJ. 1997. Acute physiological effects of zebra mussel (Dreissena polymorpha) infestation on two unionid mussels, Actionaias ligamentina and Amblema plicata. Can J Fish Aquat Sci. 54(3):1–16.
- Baker SM, Levinton JS. 2003. Selective feeding by three North American freshwater mussels implies food competition with zebra mussels. Hydrobiologia. 505(1–3):97–105.
- Beason E, Schwalb AN. 2022. Impact of zebra mussels on physiological conditions of unionid mussels in Texas. Aquat Sci. 84:21pp. https://doi.org/10.1007/s00027-022-00853-8
- Berkman PA, Haltuch MA, Tichich E, Garton DW, Kennedy GW, Gannon JE, Mackey SD, Fuller JA, Liebenthal DL. 1998. Zebra mussels invade Lake Erie muds. Nature. 393(6680):27–28.
- Bially A, Macisaac HJ. 2000. Fouling mussels (Dreissena spp.) colonize soft sediments in Lake Erie and facilitate benthic invertebrates. Freshwater Biol. 43(1):85–97.
- Bossenbroek JM, Johnson LE, Peters B, Lodge DM. 2007. Forecasting the expansion of zebra mussels in the United States. Conserv Biol. 21(3):800–810.
- Burlakova LE, Karatayev AY, Padilla DK. 2006. Changes in the distribution and abundance of Dreissena polymorpha within lakes through time. Hydrobiologia. 571(1):133–146.
- Carlton JT. 2008. The zebra mussel Dreissena polymorpha found in North America in 1986 and 1987. J Great Lakes Res. 34(4):770–773.
- Cha Y, Stow CA, Bernhardt ES. 2013. Impacts of dreissenid mussel invasions on chlorophyll and total phosphorus in 25 lakes in the USA. Freshwater Biol. 58(1):192–206.
- Chakraborti RK, Kaur J, DePinto JV. 2002. Analysis of factors affecting zebra mussel (Dreissena polymorpha) growth in Saginaw Bay: a GIS-based modeling approach. J Great Lakes Res. 28(3):396–410.
- Coakley JP, Brown GR, Ioannou SE, Charlton MN. 1997. Colonization patterns and densities of zebra mussel Dreissena in muddy offshore sediments of western Lake Erie, Canada. Water Air Soil Pollut. 99(1–4):623–632.
- Cope WG, Bartsch MR, Hightower JE. 2006. Population dynamics of zebra mussels Dreissena polymorpha (Pallas, 1771) during the initial invasion of the upper Mississippi River, USA. J Molluscan Stud. 72(2):179–188.
- Czarnołeski M, Michalczyk Ł, Pajdak-Stós A. 2004. Substrate preference in settling zebra mussels Dreissena polymorpha. Arch_Hydrobiol. 159(2):263–270.
- Depew DC, Krutzelmann E, Watchorn KK, Caskenette A, Enders EC. 2021. The distribution, density, and biomass of the zebra mussel (Dreissena polymorpha) on natural substrates in Lake Winnipeg 2017–2019. J Great Lakes Res. 47(3):556–566.
- Dzierżyńska-Białończyk A, Skrzypczak A, Kobak J. 2018. Happy together? Avoidance of conspecifics by gregarious mussels. Curr Zool. 64(1):53–61.
- Ferguson JM, McCartney MA, Blinick NS, Schroeder L, Fieberg J. 2019. Using distance sampling to estimate densities of Zebra Mussels (Dreissena polymorpha) in early-stage invasions. Freshwater Sci. 38(4):856–868.
- Ferry MM. 2013. Zebra mussel habitat selection, growth and mortality in lakes of northeastern Wisconsin and the upper peninsula of Michigan [masters thesis]. Stevens Point: University of Wisconsin, Stevens Point. p. 93. [accessed 2022 July 22]. https://invasivemusselcollaborative.net/wp-content/uploads/2018/11/Ferry-2013.pdf
- Golay J, Kanevski M, Vega Orozco CD, Leuenberger M. 2014. The multipoint Morisita index for the analysis of spatial patterns. Phys A: Stat Mech Appl. 406:191–202.
- Haltuch MA, Berkman PA, Garton DW. 2000. Geographic information system (GIS) analysis of ecosystem invasion: exotic mussels in Lake Erie. Limnol Oceanogr. 45(8):1778–1787.
- Hasler CT, Leathers J, Ducharme A, Casson NJ. 2019. Biological effects of water velocity and other hydrodynamic characteristics of flow on dreissenid mussels. Hydrobiologia. 837(1):1–14.
- Hayes JJ, Castillo O. 2017. A new approach for interpreting the Morisita index of aggregation through quadrat size. ISPRS Int J Geo-Inf. 6(10):296.
- Higgins SN, Vander Zanden MJ. 2010. What a difference a species makes: a meta-analysis of dreissenid impacts on freshwater ecosystems. Ecol Monogr. 80(2):179–196.
- Horne JK, Schneider DC. 1995. Spatial variance in ecology. Oikos. 74(1):18–26.
- Invasive Species Program. 2015. Invasive species of aquatic plants and wild animals in Minnesota; annual report for 2015. St. Paul (MN): Minnesota Department of Natural Resources. [accessed 2022 Jul 1]. Available from: https://www.leg.mn.gov/docs/2016/mandated/160477.pdf.
- Karatayev AY, Burlakova LE, Padilla DK. 1998. Physical factors that limit the distribution and abundance of Dreissena polymorpha (Pall). J Shellfish Res. 17:1219–1235.
- Karatayev AY, Padilla DK, Minchin D, Boltovskoy D, Burlakova LE. 2007. Changes in global economies and trade: the potential spread of exotic freshwater bivalves. Biol Invasions. 9(2):161–180.
- Kobak J. 2006. Factors influencing the attachment strength of Dreissena polymorpha (Bivalvia). Biofouling. 22(3):141–150.
- Kobak J, Kakareko T, Poznańska M. 2010. Changes in attachment strength and aggregation of zebra mussel, Dreissena polymorpha in the presence of potential fish predators of various species and size. Hydrobiologia. 644(1):195–206.
- Kobak J, Poznanska M, Kakareko T. 2009. Effect of attachment status and aggregation on the behaviour of zebra mussel Dreissena polymorpha. J Molluscan Stud. 75(2):119–126.
- Kobak J, Ryńska A. 2014. Environmental factors affecting behavioural responses of an invasive bivalve to conspecific alarm cues. Anim Behav. 96:177–186.
- Krebs CJ. 1999. Ecological methodology. 2nd ed. Menlo Park (CA): Benjamin Cummings, 620 pp.
- Kumar R, Varkey D, Pitcher TJ. 2016. Simulation of zebra mussels (Dreissena polymorpha) invasion and evaluation of impacts on Mille Lacs Lake, Minnesota: an ecosystem model. Ecol Modell. 331:68–76.
- Levin SA. 1992. The problem of pattern and scale in ecology. Ecology. 73(6):1943–1967.
- Lewandowski K, Ozimek T. 1997. Relationship of Dreissena polymorpha (Pall.) to various species of submerged macrophytes. Pol Arch Hydrobiol. 44:457–466.
- Londo AR, Fisher SJ, Krenz JD, Collison RM. 2022. Assessment of organic substrates as sites for zebra mussel (Dreissena polymorpha) attachment in four West-Central Minnesota lakes. J Freshwater Ecol. 37(1):71–83.
- Londo AR. 2015. Zebra Mussel (Dreissena polymorpha) habitat associations in four west-central Minnesota lakes [master’s thesis]. Cornerstone: A Collection of Scholarly and Creative Works for Minnesota State University, Mankato. [accessed 2022 Jul 8]. https://cornerstone.lib.mnsu.edu/etds/521/
- Lund K, Cattoor KB, Fieldseth E, Sweet J, McCartney MA. 2018. Zebra mussel (Dreissena polymorpha) eradication efforts in Christmas Lake, Minnesota. Lake Reservoir Manage. 34(1):7–20.
- Mackie GL, Schloesser DW. 1996. Comparative biology of zebra mussels in Europe and North America: an overview. Am Zool. 36(3):244–258.
- Makarewicz JC, Lewis TW, Bertram P. 1999. Phytoplankton composition and biomass in the offshore waters of Lake Erie: pre- and post-Dreissena introduction (1983–1993). J Great Lakes Res. 25(1):135–148.
- McComas S, Stuckert J. 2009. White Bear Lake habitat suitability assessment for invasive zebra mussels. Report prepared for White Bear Lake Conservation District, White Bear Lake, MN. St. Paul (MN): Prepared by Blue Water Science. [accessed 2022 Jul 1]. Available from: https://wblcd.org/pdf/misc/WBLreport_Zebra_mussels2010.pdf.
- McMahon RF. 1996. The physiological ecology of the zebra mussel, Dreissena polymorpha, in North America and Europe. Am Zool. 36(3):339–363.
- Morisita M. 1959. Measuring the dispersion of individuals and analysis of the distributional patterns. Memoirs of the Faculty of Science. Kyushu Univ Ser E (Biology).2:215–235.
- Nalepa TF, Fanslow DL, Lansing MB, Lang GA. 2003. Trends in the benthic macroinvertebrate community of Saginaw Bay, Lake Huron, 1987 to 1996: responses to phosphorus abatement and the zebra mussel Dreissena polymorpha. J Great Lakes Res. 29(1):14–33.
- Nalepa TF, Wojcik JA, Fanslow DL, Lang GA. 1995. Initial colonization of the zebra mussel (Dreissena polymorpha) in Saginaw Bay, Lake Huron: population recruitment, density and size structure. J Great Lakes Res. 21(4):417–434.
- Neira E, Hove M, Wilke C, McQuown M, Vande Glind J, Nelson E, Hornbach D. 2022. Unionids of Rice Creek, an urban central Minnesota stream. Ellipsaria. 24(2):12–16.
- Okuda T, Kachi N, Yap SK, Manokaran N. 1997. Tree distribution pattern and fate of juveniles in a lowland tropical rain forest – implications for regeneration and maintenance of species diversity. Plant Ecol. 131(2):155–171.
- Pejchar L, Mooney HA. 2009. Invasive species, ecosystem services and human well-being. Trends Ecol Evol. 24(9):497–504.
- Pimentel D, Zuniga R, Morrison D. 2005. Update on the environmental and economic costs associated with alien-invasive species in the United States. Ecol Econ. 52(3):273–288.
- Rowe MD, Obenour DR, Nalepa TF, Vanderploeg HA, Yousef F, Kerfoot WC. 2015. Mapping the spatial distribution of the biomass and filter-feeding effect of invasive dreissenid mussels on the winter phytoplankton bloom in Lake Michigan. Freshw Biol. 60(11):2270–2285.
- Smit H, Bij De Vaate A, Reeders HH, Van Nes EH, Noordhuis R. 1993. Colonization, ecology, and positive aspects of zebra mussels (Dreissena polymorpha) in the Netherlands. In: Nalepa T, Schloesser D, editors. Zebra mussels: biology, impacts and control. Boca Raton (FL): Lewis; p. 55–77.
- Stańczykowska A, Lewandowski K. 1993. Thirty years of studies of Dreissena polymorpha ecology in Mazurian Lakes or Northeastern Poland. In: Schloesser DW, Nalepa TF, editors. Zebra mussels; biology, impact and control. Boca Raton: Lewis Publishers; p. 3–37.
- Strayer DL. 2009. Twenty years of zebra mussels: lessons from the mollusk that made headlines. Front Ecol Environ. 7(3):135–141.
- Strayer DB, Adamovich R, Adrian DC, Aldridge C, Balogh LE, Burlakova HB, Fried-Petersen LG, Tóth AL, Hetherington TS, Jones AY, et al. 2019. Long-term population dynamics of dreissenid mussels (Dreissena polymorpha and D. rostriformis): a cross-system analysis. Ecosphere. 10(4):e02701.
- Strayer DL, Cid N, Malcom HM. 2011. Long-term changes in a population of an invasive bivalve and its effects. Oecolgia. 165(4):1063–1072.
- Tošenovský E, Kobak J. 2015. Impact of abiotic factors on aggregation behavior of the zebra mussel Dreissena polymorpha. J Mollus Stud. 82:eyv033.
- Ueno S, Tomaru N, Yoshimaru H, Manabe T, Yamamoto S. 2002. Size-class differences in genetic structure and individual distribution of Camellia japonica L. in a Japanese old-growth forest. Heredity (Edinb). 89(2):120–126.
- Wainman BC, Hincks SS, Kaushik NK, Mackie GL. 1996. Biofilm and substrate preference in the dreissenid larvae of Lake Erie. Can J Fish Aquat Sci. 53(1):134–140.
- Ward JM, Ricciardi A. 2007. Impacts of Dreissena invasions on benthic macroinvertebrate communities: a meta-analysis. Divers Distrib. 13(2):155–165.
- Wiegert RG. 1962. The selection of an optimum quadrat size for sampling the standing crop of grasses and forbs. Ecology. 43(1):125–129.
- Wimbush J, Frischer ME, Zarzynski JW, Nierzwicki-Bauer SA. 2009. Eradication of colonizing populations of zebra mussels (Dreissena polymorpha) by early detection and SCUBA removal: Lake George, NY. Aquat Conserv: Mar Freshwater Ecosyst. 19(6):703–713.
- Zhu B, Mayer CM, Heckathorn SA, Rudstam LG. 2007. Can dreissenid attachment and biodeposition affect submerged macrophyte growth? J Aquat Plant Manage. 45:71–76.