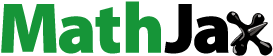
ABSTRACT
Purpose: To identify biochemical cues that could promote a keratocyte-like phenotype in human corneal stromal cells that had become fibroblastic when expanded in serum-supplemented media while also examining the effect on cell proliferation and migration.
Methods: Proliferation was assessed by PrestoBlue™, morphology was monitored by phase contrast microscopy, phenotype was analyzed by real-time polymerase chain reaction (qPCR), immunochemistry and flow cytometry, and migration was studied with a scratch assay.
Results: Ascorbic Acid (AA), Retinoic Acid (RA), Insulin-Transferrin-Selenium (ITS), Insulin-like Growth Factor 1 (IGF-1) and 3-isobutyl-1-methylxanthine (IBMX) promoted a dendritic morphology, increased the expression of keratocyte markers, such as keratocan, aldehyde dehydrogenase 3 family member A1 (ALDH3A1) and CD34, and prevented myofibroblast differentiation, while in some cases increasing proliferation. Transforming Growth Factor beta 1 (TGF-β1) and 3 (TGF-β3) promoted the differentiation toward myofibroblasts, with increased expression of α-SMA. Fibroblast Growth Factor 2 (FGF-2) supported a fibroblastic phenotype while Platelet-Derived Growth Factor Homodimer B (PDGF-BB) induced a pro-migratory fibroblastic phenotype. A combination of all the pro-keratocyte factors was also compared to the serum-free only, which significantly increased CD34 and keratocan expression.
Conclusions: Partially recovery towards a quiescent keratocyte-like phenotype was achieved by the removal of serum and the addition of AA, IGF-1, RA, ITS and IBMX to a basal medium. These findings can be used to develop cell-based corneal therapies and to study corneal diseases in vitro.
Introduction
Corneal diseases and injuries are among the most prevalent causes of blindness and affect more than 45 million people worldwide, only being surpassed by cataracts.Citation1,Citation2 While minor injuries to the cornea can heal quickly, deeper or more severe injuries can lead to scar formation and corneal haze. The main treatment offered to patients with corneal blindness is keratoplasty. However, due to the high demand for this procedure, the need of donor corneal tissue has increased and has resulted in donor shortage in several countries.Citation3–Citation5 For this reason alternatives to donor allografts are being developed such as cell-based therapies and tissue engineering strategies.Citation6,Citation7
To successfully develop new cell-based therapies, a suitable cell source is required. For injuries or diseases affecting the corneal stroma, keratocytes, the main cell type in the stroma that has characteristics associated with mesenchymal stromal cells, are the logical choice of cells for corneal regeneration. To obtain a large enough number of stromal cells required for cell-based therapies or tissue engineering, the cells must be expanded in vitro normally using serum-supplemented media. When keratocytes are exposed to serum, they become fibroblastic recapitulating the in vivo process of wound healing. Upon serum activation there is a change in morphology from a dendritic shape to an elongated spindle morphology and markers associated with the native keratocyte phenotype such as crystalline ALDH3A1 and keratin sulphate proteoglycans such as keratocan are lost.Citation8 When treated with Transforming Growth Factor β1 (TGF-β1) corneal fibroblasts transition to a myofibroblast phenotype, becoming larger and expressing alpha smooth muscle actin (α-SMA). These two distinct phenotypes are fairly easy to obtain in vitro from isolated corneal stromal cells via the supplementation of medium with fetal bovine serum (FBS) for fibroblasts and addition of TGF-β1 for myofibroblasts. Although some studies have reported a partial reversal to a keratocyte-like phenotype using serum-free media,Citation9–Citation12 there is still a lack of information on how individual media supplements such as vitamins or growth factors affect this process.
The aim of this study was to examine the effect of several biochemical reagents, ranging from small molecules to proteins, on the phenotype and activity of serum-expanded human corneal stromal cells when switched to a serum-free media. By doing this, the role of individual biochemical cues on the ability to restore a keratocyte phenotype in vitro can be examined.
Material and methods
Cell isolation
Cells were isolated from the healthy donor’s corneal-scleral ring remaining after a corneal transplant in accordance with the Declaration of Helsinki. The corneal-scleral rings were rinsed with sterile phosphate buffered saline (PBS) and the sclera, epithelium and endothelium were carefully removed using a scalpel blade. After a brief wash with sterile PBS, the corneal stroma was diced into small pieces, transferred into 25 cm2 culture flasks and let attach to the plastic. Media consisting of low-glucose Dulbecco’s Modified Eagle Medium (DMEM, Hyclone) supplemented with 10% Fetal Bovine Serum, (FBS, Gibco) and 2% Penicillin/Streptomycin (Pen/Strep, Invitrogen) was added and the stromal pieces were cultured at 37°C and 5% CO2 in a humidified incubator. Media was changed regularly until cells had migrated from the tissue and reached 80–90% confluence, then they were passaged to allow further expansion. Cells in this study were used at passage 4 from cryopreserved expanded cells.
Cell culture conditions
Cells were harvested and seeded at 10,000 cells per cm2 using low-glucose DMEM + 10% FBS and let attach overnight. The following day media was switched to DMEM/F12 (glucose concentration: 3.15 mg/ml, Hyclone) for a 3 day serum-starvation period, after which one of the following biochemical factors was added with the following concentrations: 100 µg/ml ascorbic acid (AA, Sigma); 0.1 µM retinoic acid (RA, Sigma); 10 ng/ml fibroblast growth factor 2 (FGF-2, R&D); 50 ng/ml platelet derived growth factor BB (PDGF-BB, Preprotech); 50 ng/ml Insulin-like Growth Factor 1 (IGF-1, ProSpec); 10 ng/ml transforming growth factor β1 (TGF-β1, ProSpec); 0.1 ng/ml transforming growth factor β3 (TGF-β3, R&D); 1x insulin-transferrin-selenium (ITS, Gibco) and 10−5 M 3-isobutyl-1-methylxanthine (IBMX, Sigma). Control groups were cells cultured in serum-free DMEM/F12 only and cells cultured in DMEM/F12 supplemented with 10% FBS. Medium was changed every 2 days for culture periods of 1, 7 and 14 days. Images of the cells were recorded over the culture period using an Olympus IX83 microscope (Tokyo, Japan).
Metabolic activity
Metabolic activity was assessed by the PrestoBlue assay (Invitrogen) following the manufacturer’s instructions. 96-well plates were seeded separately for each time-point (day 1, 3, 7 and 14). Media was removed and 100 µl PrestoBlue working solution (1x from a 10x stock) was added and incubated at 37°C for 1 h. The plates were analyzed on a plate reader (BioTek™ Synergy HTX, Vermont, USA) by measuring the absorbance at 570 nm and 600 nm. Metabolic activity was calculated relative to the serum-free medium condition.
Gene expression
RNA from cultures was isolated using Trizol, according to the manufacturer’s instructions (Invitrogen). Briefly, 1 ml of Trizol was added to the monolayer and a cell scrapper was used to detach the cells. 200 µl of chloroform was added, vortexed and centrifuged at 12 000 g at 4°C. The supernatant was transferred to a new RNAse-free tube to which the same volume of isopropanol and 4 µl of Glycoblue (Applied Biosystems) were added. After an overnight incubation at −20°C, tubes were centrifuged at 12 000 g for 15 min at 4°C. The supernatant was discarded and 1 ml 70% ethanol was added and vortexed to wash the pellet. After a second centrifugation step of 12 000 g for 15 min at 4°C, the ethanol was removed, the pellet was air dried and dissolved in 11 µl of RNAse-free water. RNA yield and purity was quantified using a NanoDrop-1000 (ThermoFisher).
A high capacity cDNA reverse transcription kit (Invitrogen) was used to reverse transcribe 500 ng of RNA using a thermocycler. Real-time PCR of the resultant cDNA was performed using TaqMan gene expression assay primers and TaqMan Universal Master Mix II (all Applied Biosystems). The primers examined were glyceraldehyde-3-phosphate dehydrogenase (GAPDH, Hs02758991_g1), aldehyde dehydrogenase 3A1 (ALDH3A1, Hs00964880_m1), alpha smooth muscle actin (ACTA2, Hs00426835_g1), keratocan (KERA; Hs00559942_m1) and collagen 1a1 (COL1; Hs00164004_m1). Each gene of interest was normalized against GAPDH using the ΔΔCt method. Calculated values were expressed as a power of 2−ΔΔCt. For this study, all values were normalized to the serum-free controls at each time-point.
Immunochemistry
Cells were fixed with 4% Paraformaldehyde (PFA) for 15 min, followed by three washes with PBS and stored at 4°C until further staining. Blocking and permeabilization were carried out in a single step using 2% FBS and 0.5% Triton X-100 diluted in PBS for 30 min at room temperature, followed by three washing steps with PBS. Primary antibodies were incubated overnight at 4°C. Concentrations were used as follows: ALDH3A1 (Abcam) 1:250, keratocan (Santa Cruz) 1:100, α-SMA (Abcam) 1:250. After three washes with PBS, samples were incubated with the secondary antibody and phalloidin-rhodamine for 1 h at room temperature. Samples were washed three times with PBS and incubated for 10 min with DAPI. Images were obtained using an Olympus IX83 microscope.
Flow cytometry
Cell phenotype was further assessed by flow cytometry. Data was acquired using an LSRFortessa (BD Biosciences) flow cytometer and then analyzed using FlowJo software (Tree Star Inc., Oregon, USA). Cells were trypsinized and resuspended in 150 µl of 2% FBS in PBS and 50 µl of cell suspension was used for the staining. 1 µl of each of the following fluorophore-coupled antibodies was added to the cell suspension: CD34-allophycocyanin (APC), CD44-eFluor450, CD45-fluorescein isothiocyanate (FITC), CD73-PerCP-eFluor710, CD90-phycoerythrin (PE) and CD105-PE-Cy7 (all eBiosciences), and the final volume was adjusted to 100 µl (so that antibodies were used at 1:100 dilution). Samples were vortexed and incubated at 4°C for a minimum of 30 min. Unstained controls were treated the same way. Compensation beads (eBiosciences) were stained with each fluorophore-coupled antibody. Two washing steps were performed to remove unbound antibodies. The remaining pellet was resuspended in 300 µl of buffer and 5 µl of propidium iodide was added to recognize and exclude dead cells.
Wound healing assay
To assess the influence of biochemical cues in migration of human corneal keratocyte-like cells a scratch assay was performed. Cells were seeded in 6-well plates at a density of 10 000 cells per cm2 in expansion media, which was changed regularly until confluent. Cells were then serum-starved using DMEM/F12 for 3 days. A “scratch” was applied to the monolayer with a 200 µl pipette tip, which resulted in a cell-free area. Cells were then cultured in media containing each of the molecules to be tested, having two controls: one serum-free only and one containing 10% FBS. Cells were imaged on the same area every day for 7 days using phase contrast imaging in an inverted microscope (Olympus IX83) coupled to a digital camera. The cell-free area at each time-point was calculated using ImageJ (NIH) by finding the edges, adjusting the threshold, binarizing the images and selecting the cell-free areas with the tracing tool. The wound closure was calculated using the following formula:
Statistics analysis
All experiments were performed three times using a minimum of three replicates, unless otherwise stated. Statistical analyses were performed using GraphPad Prism Software 5.0 (GraphPad Software, Inc. La Jolla, CA, USA). All data are presented as the mean ± SD. To determine statistical significance one-way ANOVA with Dunnet post-hoc analyses were performed. Differences were considered to be statistically significant at p ≤ 0.05.
Results
Effect of biochemical cues on cell proliferation
Metabolic activity under the induction of each biochemical cue was analyzed using the PrestoBlue® assay and used as an estimate for cell proliferation (). The addition of FBS to the medium had a remarkable effect on proliferation, increasing 591% ± 131% (average ± SD) at day 7 compared to the serum-free control. TGF-β1 increased proliferation significantly from day 3 onwards, achieving values of 199% ± 29% by day 14. A similar trend was observed when cells were treated with TGF-β3, which increased proliferation at day 7 (164% ± 31%) and day 14 (203% ± 74%). IGF-1 increased proliferation significantly at day 3 (136% ± 19%) and day 7 (144% ± 25%) but then the effect appeared to stagnate. ITS promoted proliferation after one (156% ± 20%) and 2 weeks in culture (181% ± 94%). The rest of treatments did not have a positive nor negative effect on cell metabolic activity when compared to the serum-free control.
Effect of biochemical cues on cell morphology
Removal of serum from the medium had an effect on cell morphology, with the cells becoming smaller and the appearance of dendrites (). This morphology resembles that of quiescent keratocytes in vivo. More cells adopt this morphology upon the addition of IGF-1, AA, RA, ITS or IBMX. The addition of PDGF-BB induced the cells to cluster and adopt a very elongated spindle shape. FGF-2 maintained a fibroblastic-like morphology, with very few sparse cells having processes. TGF-β1 and TGF-β3 induced cells to adopt a spread-out shape with some cells becoming polygonal and stress fibers visible. Cells cultured with FBS maintained a spindle shape.
Figure 2. Morphology of cultured cells under the induction of studied biochemical cues after 14 days in culture: (a) ascorbic acid; (b) FGF-2; (c) PDGF-BB; (d) IGF-1; (e) retinoic acid; (f) insulin-transferrin-selenium; (g) IBMX; (h) TGF-β1; (I) TGF-β3; (J) foetal bovine serum; (k) serum-free control. Low magnification scale bar = 200 µm, high magnification scale bar = 50 µm.
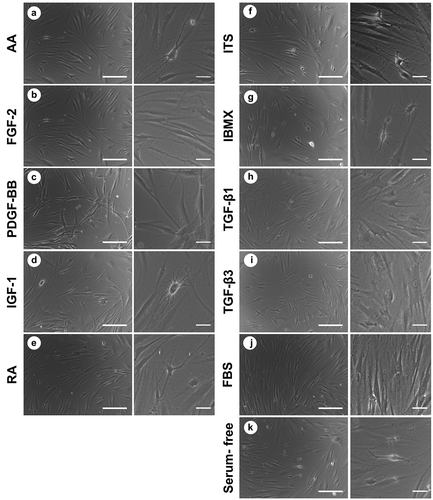
Effect of biochemical cues on cell phenotype
Removal of serum from the medium led to an increased expression of keratocyte markers ALDH3A1 and keratocan when compared to cells cultured in serum-supplemented media (). None of the biochemical reagents had a significantly positive effect on ALDH3A1 expression when compared to the serum-free control. TGF-β1, PDGF-BB and FBS down-regulated the expression of this gene. The expression of keratocan was significantly up-regulated upon treatment with AA and IBMX after 14 days of induction (3.82 ± 1.87 and 3.99 ± 4.58, respectively; average ± SD). RA, ITS and IGF-1 also up-regulated this marker, but to a limited extent (p > 0.05). FGF-2, PDGF-BB, TGF-β1 and TGF-β3 did not have a significant effect, while FBS down-regulated the expression of keratocan. Expression of collagen type I (COL1) was increased significantly at time-point day 1 with the addition of IGF-1, TGF-β1 and TGF-β3 (1.74 ± 0.28, 1.54 ± 0.34, 1.73 ± 0.11, respectively; average ± SD). At later time-points only AA and ITS increased significantly this gene’s expression (3.82 ± 0.20, 3.00 ± 0.44, respectively; average ± SD). TGF-β1 up-regulated significantly the expression of ACTA2 at all time-points tested (8.74 ± 2.29 at day 1, 7.55 ± 2.46 at day 7 and 4.33 ± 1.87 at day 14; average ± SD). TGF-β3 had the same effect as TGF-β1, with very similar fold changes compared with the serum-free control (8.42 ± 4.78 at day 1, 4.36 ± 3.43 at day 7 and 3.26 ± 2.14 at day 14; average ± SD). Levels of myofibroblastic marker ACTA2 did not change much over time, for all other treatments.
Figure 3. Effect of tested treatments on the expression of a) ALDH3A1, b) KERA, c) ACTA2 and d) COL1 as determined by qPCR relative to serum-free control at each time-point (n = 5–10). p* < 0.05, p** < 0.01, p*** < 0.001.
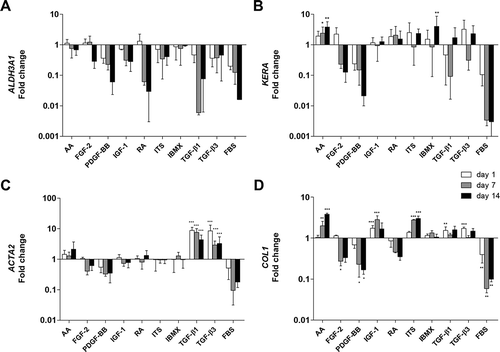
Expression of these markers was further analyzed with immunochemistry. While expression of ALDH3A1 was down-regulated at the mRNA level, staining showed positive cells in some conditions. Cells with a dendritic morphology showed more intense staining, especially when treated with ITS, IGF-1, IBMX, RA and in the serum-free control. Very low staining was seen in cultures treated with PDGF-BB, FBS, TGF-β1 and TGF-β3 (). Immunostaining of keratocan showed faint cytosolic staining in all conditions. When cells were treated with IGF-1, IBMX, ITS and RA, bright perinuclear staining was observed (). However, keratocan is a keratin sulphated proteoglycan that is deposited as ECM, hence was probably washed away during cell feedings. Expression of α-SMA correlated with expression of its gene ACTA2. Bright α-SMA stress fibers can be observed in cells treated with TGF-β1 and TGF-β3. Very sparse α-SMA+ cells were seen in treatments with AA and ITS ().
Figure 4. Effect of biochemical cues on the expression of markers determined by immunofluorescence after 14 days of treatment. a) ALDH3A1 (green), nuclei (blue) and F-actin (red). b) Keratocan (green), nuclei (blue). c)α-SMA (green), nuclei (blue) and F-actin (red). Scale bar = 200 µm.
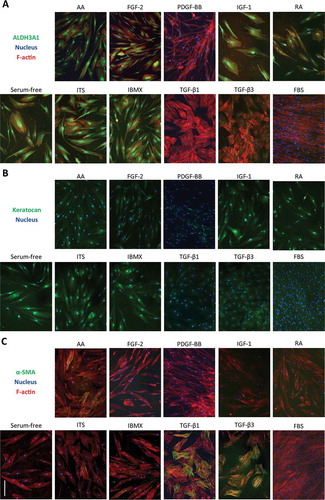
After 7 and 14 days in culture, cell surface markers were analyzed and quantified by flow cytometry (). Typical markers used for the identification of mesenchymal stromal cells were employed. CD34 was found to change the most for each treatment. After 7 days of treatment, the serum-free controls presented 9.13% of the population positive for this marker, while treatment with AA, IBMX, IGF-1, ITS and RA increased this percentage considerably (14.03, 10.81, 9.52, 13.55 and 31.75%, respectively). This trend was maintained and increased after 14 days. However, only treatment with RA increased the CD34+ population significantly. Other biochemical cues led to a reduction in the percentage of positive CD34 cells by day 7 (FBS: 0.08%, PDGF-BB: 0.47%, TGF-β1: 1.62% and TGF-β3: 2.09%) and day 14 (FBS: 1.47%, PDGF-BB: 1.49%, TGF-β1: 5.15% and TGF-β3: 5.03%). CD73 remained > 90% positive and was only reduced when treated with FBS and PDGF-BB (78.23% and 86.90%, respectively at day 7). After 14 days in culture, this marker remained positive (> 90%) in all conditions. CD90 was > 95% positive in all conditions, and most conditions induced an expression of > 99%. Expression of CD90 remained positive after 14 days (> 90%). Expression of CD105 also remained positive (> 88%) at culture time 7 days and 14 days. CD45 was < 2% in all conditions at day 7 and < 3.5% at day 14. CD44 was highly expressed (> 95% positive) in all conditions at day 7 without any noticeable difference among groups and remained positive after 14 days of treatment.
Table 1. Cell surface marker expression after 7 and 14 days of treatment (n = 4).
Effect of biochemical cues on wound healing ability
The influence of biochemical cues on the ability of wound healing was studied with a scratch assay (). Seven days after the scratch was performed, only cells fed with serum-supplemented medium (100% ± 0%; average ± SD) or medium with PDGF-BB were able to fully close the wound gap (93% ± 3%; average ± SD). Supplementation with IGF-1 and ITS increased the closing of the scratch significantly when compared to the serum-free control (73% ± 8% and 69% ± 8%, respectively; average ± SD). Addition of AA, FGF-2 and IBMX slightly increased cell migration but not significantly. RA, TGF-β1 and TGF-β3 reduced cell migration to some extent. Only PDGF-BB can be described to have a positive migration effect as the wound closure is only due to cell migration since this growth factor had little effect on cell proliferation.
Combinatorial effect of pro-keratocyte cues
A final experiment was conducted in which the cues that showed a promotion of the keratocyte phenotype were used in combination with the basal medium and compared to serum-free conditions. Cells cultured for 2 weeks in medium containing AA, ITS, RA and IBMX presented a higher number of dendritic cells, CD34+ population was highly increased and keratocan gene expression was particularly up-regulated. However, expression of ALDH3A1 was down-regulated and expression of COL1 did not increase. α-SMA remained negative at the gene and protein level. Cells were positive for CD44, CD90 and CD105 and negative for CD45 in both media conditions, while CD73 decreased slightly, yet significantly. These results are depicted in .
Figure 6. Effect of combined pro-keratocyte cues. a) Bright field images and immunofluorescence against ALDH3A1, Keratocan and α-SMA (all green, F-actin is shown in red and nuclei in blue). Scale bar = 100 µm. b) Flow cytometry profiling of cells cultured in serum-free medium or with the combination of pro-keratocyte cues (n = 3). c) Gene expression of cells cultured in serum-free medium or with the combination of pro-keratocyte cues (n = 6). p *** < 0.001.
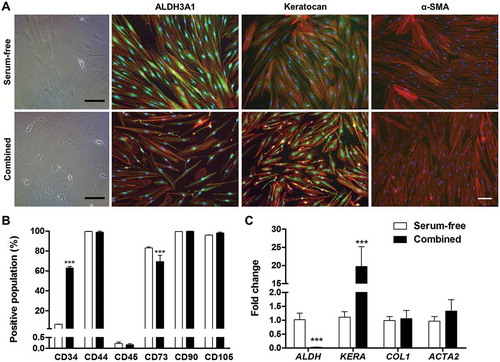
Discussion
Understanding the effect of different biochemical cues on cultured human corneal stromal cells is key to understanding their physiological and pathological behavior. A deep knowledge of cell culture conditions is essential when developing in vitro models of corneal diseases, as well as developing cell-based therapies and tissue engineering corneal substitutes. Here a thorough study of how human corneal stromal-derived cells, initially expanded in serum-containing medium, react to a range of biochemical cues under serum-free conditions is presented. A summary showing the overall effect of each cue on the cell behavior is shown in .
Table 2. Summary of results from this study.
Expansion of corneal stromal cells in FBS is known to result in a fibroblastic phenotype.Citation13 While alternatives to FBS, such as bovine pituitary extract, show a lot of promise as an approach for expanding keratocytes while maintaining the native phenotype,Citation14 most researchers still use FBS in the expansion phase. When compared to cells where FBS was removed, the cells in FBS proliferated rapidly, maintained a spindle morphology, and displayed low levels of keratocan and ALDH3A1 at both mRNA and the protein level as reported previously by other researchers.Citation15,Citation16 Despite the activated nature, the cells did not differentiate into myofibroblasts as very little α-SMA could be detected, either at the mRNA or the protein level. Similar results have been shown in our group and in others, especially when seeded at high densities.Citation10,Citation17–Citation19 MSC markers were expressed in the same pattern as the serum-free control, except no increase in CD34 was observed. Cells treated with FBS closed fully the wound in the scratch assay, although this could be due to proliferation.
Both AA and RA are antioxidant vitamins commonly used as culture media supplements to support cell growth. In this study both regents supported a dendritic morphology, had little effect on cell proliferation, inhibited α-SMA formation and promoted an increase in keratocan gene expression although only AA was statistically significant. The findings for AA agree with previous studies that show increased keratocan expressionCitation20 and no significant change in MSC markersCitation12 associated with this molecule. There are conflicting reports on the effect of AA on proliferation with both increases in proliferation or no alterations in cell number having been shown.Citation20,Citation21 It has long been known that AA stabilizes the triple helix of collagen as it is a co-substrate of peptidyl-prolyl hydroxylase which converts proline into hydroxyproline.Citation22 In this study AA increased expression of COL1 gene, similar as reported for other cell types such as skin fibroblasts and avian tendon cells.Citation23,Citation24 RA has also been associated with both increases and decreases in cell number although this is dependent on the concentration of RA used.Citation25,Citation26 RA has also been associated with increases in the expression of keratocyte markers in both ordinary cell cultureCitation25 and in collagen hydrogels.Citation27 RA had a significant effect in increasing the CD34+ population, while not modifying the expression of the other MSC markers, in a similar way as reported by Sidney and Hopkinson.Citation12 The observed reduction in migration of cells treated with RA also matches previous findings that used keratocytesCitation25 and adipose-derived stem cells in a keratocyte differentiation medium.Citation28 Gouveia and Connon showed that promoter regions of keratocyte marker genes did not have RARE sequences, and therefore RA should signal via alternative pathways. However, the gene encoding for CD34 has an enhancer region (GeneHancer identifier GH01I207846) with RARα binding domains which could explain the increase in CD34+ cells when treated with RA. These results indicate that both AA and RA may assist in inducing a partial restoration of the keratocyte phenotype.
FGF-2 did not have a significant effect on cell proliferation, contrary to some reports in the literatureCitation29,Citation30 although these studies used media formulations containing additional supplements. However, our FGF-2 proliferation data did agree with findings that used bovine keratocytes.Citation31 FGF-2 did not have an effect in changing cell morphology after serum-starvation as cells maintained their spindle shape, similar to some previously reported studies.Citation32 Expression of ALDH3A1, keratocan and α-SMA were not up-regulated. It has been postulated that the reduced expression of keratan sulfate proteoglycans such as Lumican or Keratocan by FGF-2 is mediated by the Rho and JNK pathways.Citation33,Citation34 Furthermore, FGF-2 supplementation led to a reduction in the number of CD34+ cells. The loss of CD34 in the cornea is associated with keratoconus where cells also become more fibroblastic.Citation35 Overall these findings suggest that FGF-2 induces a fibroblastic phenotype of cultured stromal cells.
PDGF-BB did not promote cell proliferation in this study, conflicting with some published research. However, in those studies the media used was supplemented with 0.5% FBSCitation36 or 2% FBS.Citation37 In the current study, cells treated with PDGF-BB show a fibroblastic-like shape but with a more elongated cell body than when treated with FBS, a similar finding to that previously described.Citation32 However, when cells treated with PDGF-BB were embedded in compressed collagen gels they presented branching processes indicating that the physical surroundings may play a role in how the cells react to the growth factor.Citation38 In our study, expression of keratocyte markers was down-regulated, while no upregulation of myofibroblastic markers was detected. As seen with FGF-2, treatment with PDGF-BB also reduced the number of CD34+ cells. This growth factor also displayed a strong chemotactic effect as has been previously demonstrated in 2D using Boyden chambersCitation39 and in 3D compressed collagen gels.Citation38 It is believed that PDGF influences cell migration via Erk, which phosphorylates FAK and paxillin regulating dynamics of focal adhesions.Citation40 Decrease in actin stress fibers can me mediated by N-WASP after downstream signaling via PI3K and Cdc42, a Rho GTPase.Citation41 Hence we can conclude that PDGF-BB promotes a motile fibroblastic phenotype.
In this study the roles of IGF-1 and ITS supplementation were examined. Insulin is a commonly used cell culture supplement that regulates the uptake of glucose and amino acids. Insulin binds to insulin receptor (INSR) through the alpha subunit, which produces a conformational change that will induce the phosphorylation of tyrosine residues in the beta subunit of the insulin receptor. This triggers a downstream signaling cascade through the MAPK pathway, inducing cell proliferation, and through the PI3K/Akt pathway leading to cell growth, and lipid, glycogen and protein synthesis.Citation42–Citation44 IGF-1 led to an early positive increase in cell proliferation, a dendritic morphology, increases in keratocan gene expression, ALDH3A1 staining and CD34+ cells. Etheredge and colleagues reported no effect on corneal fibroblast proliferation at a concentration of 10 ng/ml, while Yanai and colleagues showed increase in proliferation and inhibition of apoptosis at 305 nM, which corresponds to 2 µg/ml.Citation31,Citation45 Maintenance of a dendritic morphology in rabbit stromal cells when treated with IGF-1 has also previously been shown.Citation32 ITS had a positive effect in cell survival similar to that seen by other authors,Citation20 promoted a dendritic morphology with multiple processes and led to a significant increase in COL1 gene expression, ALDH3A1 immunofluorescence and more CD34+ cells. Similar to IGF-1, ITS promoted the closure of the wound in the scratch assay but the increase in proliferation renders the chemotactic effect elusive. No upregulation of ACTA2 or expression of α-SMA was detected for either IGF-1 or ITS. In fact, recent studies suggest that IGF-1 inhibits fibrosis initiated by TGF-β.Citation46 Taking these findings together, it can be said that both IGF-1 and ITS partially induce a quiescent keratocyte phenotype.
IBMX is a competitive non-selective inhibitor of phosphodiesterase that raises intracellular cAMP levels, which activate protein kinase A, phosphorylating in turn cAMP response element-binding proteins (CREB) in the nucleus which act as transcription factors.Citation47 Researchers have used IBMX to emulate the effects of low glucose conditions.Citation48 Upon treatment with IBMX, cells with multiple dendrite-like structures were observed similar to that reported previously.Citation48 A significant increase in keratocan gene expression was observed and bright perinuclear staining was observed for this marker. The increase of this marker could be due the presence of CRE sequences in the promoter of this gene.Citation48 ALH3A1 was highly expressed in cells with processes, similar to observations by Foster and colleagues for ALDH1.Citation48 These authors reported an increase of CD34 at the transcriptional level, while our study showed an increase in the CD34+ population via flow cytometry. IBMX did not induce changes in any other MSC markers and did not show a chemotactic effect. The addition of this chemical did not influence expression of COL1 or ACTA2. This data indicates that treatment with IBMX promoted the restoration of a keratocyte-like phenotype.
Both TGF-β1 and TGF-β3 had a similar effect on all the parameters examined in this study, particularly the upregulation and immunochemical staining of α-SMA, a known myofibroblast marker. It has previously been well established that TGF-β1 induces a myofibroblastic phenotype.Citation30–Citation32,Citation49,Citation50 Transcription of α-SMA is induced by the translocation to the nucleus of phosphorylated Smad2/3/4 complex. These proteins are activated by ALK5, which is phosphorylated by TGFβ Receptor I and II upon ligand binding.Citation51 Fibroblasts expressing α-SMA display a retarded motility using a scratch assayCitation52 which coincides with the current findings where α-SMA positive cells were unable to bridge the gap during the scratch assay. In fact, the edge of the wound was always clear and straight, and the whole front would move together, suggesting that any apparent migration was due to cell proliferation. Despite an initial upregulation of COL1 by both TGFs, at later time-points the expression was similar to that of the serum-free control. The effect of TGF-β3 in this study was interesting since it contradicts some other studies that suggest TGF-β3 has an antifibrotic effect.Citation53,Citation54 One possible explanation is that the authors used a self-assembled culture, medium containing 10% FBS and AA and cultured their cells for 4 weeks, hence multiple factors may be contributing to the cell behavior. Sidney and Hopkinson showed that TGF-β3 led to lower levels of ALDH3A1 and vimentin and higher levels α-SMA gene expression when compared to cells cultured under serum and growth factor free conditions.Citation12 Another study recently showed positive α-SMA staining resulting from the addition of TGF-β3 although the amount of staining was significantly less than at the same concentration of TGF-β1Citation55 From our results it would appear that both TGF-β1 and TGF-β3 promote a myofibroblastic phenotype.
When AA, ITS, RA and IBMX were used in combination, keratocyte markers CD34 and keratocan were up-regulated, while ALDH3A1 was down-regulated and COL1 remained unchanged. Since RA individually decreased these two markers one could speculate that RA is the main reagent responsible and that the other cues could not synergistically overcome this.
As discussed throughout, multiple signaling pathways are involved after supplementation with the factors studied here. Although outside of this study’s scope, investigating the expression of some of the key down-stream proteins is key to understanding the mechanisms of keratocyte activation and return to quiescence. The use of advanced molecular techniques with high-throughput potential, such as microarrays, would be extremely beneficial for this. By understanding influence of these signaling pathways, better control of the cell phenotype could be achieved.
This study reveals once more the importance of biochemical cues to promote the reversal of activated keratocytes toward a quiescent keratocyte phenotype in vitro. However, biochemical supplementation of the medium is unlikely to lead to a full phenotype reversal. Other environmental cues such as substrate stiffness, material topography and 3D culture play an important role as multiple studies have demonstrated.Citation10,Citation11,Citation56 Furthermore, factors such as the donor’s age and health, the number of population doublings of the cells and the time in culture are among other variables that need to be considered. Since tissue remodeling and wound healing are long lasting processes in the corneal stromaCitation57, it is therefore logical to suspect that phenotype reversal in vitro can only be achieved after longer culture periods.
From this study we can conclude that human corneal stromal-derived cells originally expanded in serum-containing medium can partially recover a quiescent keratocyte-like phenotype by the removal of serum and the addition of AA, IGF-1, RA, ITS and IBMX to a basal medium. The keratocyte-like phenotype was demonstrated by a dendritic morphology and upregulation of keratocyte markers such as keratocan, ALDH3A1, COL1 and CD34. These findings are important for the optimization of culture conditions to enable the expansion of stromal derived cells for regenerative therapies and tissue engineering.
Acknowledgments
The authors would like to thank Dr Barry Moran for the support in the flow cytometry experiments.
Disclosure statement
No potential conflict of interest was reported by the authors.
Additional information
Funding
References
- Whitcher JP, Srinivasan M, Upadhyay MP. Corneal blindness: a global perspective. Bull World Health Organ. 2001;79(3):214–21. doi:10.1111/ceo.12330.
- Pascolini D, Mariotti SP. Global estimates of visual impairment: 2010. Br J Ophthalmol. 2012;96(5):614–18. doi:10.1136/bjophthalmol-2011-300539.
- Rasouli M, Caraiscos VB, Slomovic AR. Efficacy of routine notification and request on reducing corneal transplantation wait times in Canada. Can J Ophthalmol. 2009;44(1):31–35. doi:10.3129/i08-187.
- Reinhard T, Böhringer D, Bogen A, Sundmacher R. The transplantation law: a chance to overcome the shortage of corneal grafts in Germany? Transplant Proc. 2002;34(4):1322–24. doi:10.1016/s0041-1345(02)02783-5.
- de By TM. Shortage in the face of plenty: improving the allocation of corneas for transplantation. Dev Ophthalmol. 2003;36:56–61. doi:10.1159/000067656.
- Griffith M, Alarcon EI, Brunette I. Regenerative approaches for the cornea. J Intern Med. 2016;280(3):276–86. doi:10.1111/joim.12502.
- Ghezzi CE, Rnjak-Kovacina J, Kaplan DL. Corneal tissue engineering: recent advances and future perspectives. Tissue Eng Part B Rev. 2015;21(3):278–87. doi:10.1089/ten.teb.2014.0397.
- Jester JV, Huang J, Møller-Pedersen T, Sax CM, Kays WT, Cavanagh HD, Petroll WM, Piatigorsky J. The cellular basis of corneal transparency: evidence for “corneal crystallins.”. J Cell Sci. 1999;112:613–22.
- Berryhill BL, Kader R, Kane BP, Birk DE, Feng J, Hassell JR. Partial restoration of the keratocyte phenotype to bovine keratocytes made fibroblastic by serum. Invest Ophthalmol Vis Sci. 2002;43:3416–21.
- Lynch AP, O’Sullivan F, Ahearne M. The effect of growth factor supplementation on corneal stromal cell phenotype in vitro using a serum-free media. Exp Eye Res. 2016;151:26–37. doi:10.1016/j.exer.2016.07.015.
- Wilson SL, Wimpenny I, Ahearne M, Rauz S, El Haj AJ, Yang Y. Chemical and topographical effects on cell differentiation and matrix elasticity in a corneal stromal layer model. Adv Funct Mater. 2012;22(17):3641–49. doi:10.1002/adfm.201200655.
- Sidney LE, Hopkinson A. Corneal keratocyte transition to mesenchymal stem cell phenotype and reversal using serum-free medium supplemented with FGF-2, TGF-ß3 and retinoic acid. J Tissue Eng Regen Med. 2016;12(1):e203–15. doi:10.1002/term.2316.
- Fini ME. Keratocyte and fibroblast phenotypes in the repairing cornea. Prog Retin Eye Res. 1999;18(4):529–51. doi:10.1016/S1350-9462(98)00033-0.
- Xu -Z-Z, Li Z-J, Du L-X, Li J, Wang L-Y. Using bovine pituitary extract to increase proliferation of keratocytes and maintain their phenotype in vitro. Int J Ophthalmol. 2013;6:758–65.
- Beales MP, Funderburgh JL, Jester JV, Hassell JR. Proteoglycan synthesis by bovine keratocytes and corneal fibroblasts: maintenance of the keratocyte phenotype in culture. Invest Ophthalmol Vis Sci. 1999;40:1658–63.
- Espana EM, He H, Kawakita T, Di Pascuale MA, Raju VK, Liu CY, Tseng SC. Human keratocytes cultured on amniotic membrane stroma preserve morphology and express keratocan. Invest Ophthalmol Vis Sci. 2003;44(12):5136–41. doi:10.1167/iovs.03-0484.
- Masur SK, Dewal HS, Dinh TT, Erenburg I, Petridou S. Myofibroblasts differentiate from fibroblasts when plated at low density. Proc Natl Acad Sci USA. 1996;93(9):4219–23. doi:10.1073/pnas.93.9.4219.
- Pot SA, Liliensiek SJ, Myrna KE, Bentley E, Jester JV, Nealey PF, Murphy CJ. Nanoscale topography-induced modulation of fundamental cell behaviors of rabbit corneal keratocytes, fibroblasts, and myofibroblasts. Invest Ophthalmol Vis Sci. 2010;51(3):1373–81. doi:10.1167/iovs.09-4074.
- Guerriero E, Chen J, Sado Y, Mohan RR, Wilson SE, Funderburgh JL, SundarRaj N. Loss of alpha3(IV) collagen expression associated with corneal keratocyte activation. Invest Ophthalmol Vis Sci. 2007;48(2):627–35. doi:10.1167/iovs.06-0635.
- Musselmann K, Kane BP, Alexandrou B, Hassell JR. Stimulation of collagen synthesis by insulin and proteoglycan accumulation by ascorbate in bovine keratocytes in vitro. Invest Ophthalmol Vis Sci. 2006;47(12):5260–66. doi:10.1167/iovs.06-0612.
- Saika S, Kanagawa R, Uenoyama K, Hiroi K, Hiraoka J. L-ascorbic acid 2-phosphate, a phosphate derivative of L-ascorbic acid, enhances the growth of cultured rabbit keratocytes. Graefe’s Arch Clin Exp Ophthalmol. 1991;229(1):79–83. doi:10.1007/bf00172267.
- Stone N, Meister A. Function of ascorbic acid in the conversion of proline to collagen hydroxyproline. Nature. 1962;194(4828):555–57. doi:10.1038/194555a0.
- Tajima S, Pinnell SR. Regulation of collagen synthesis by ascorbic acid increases type I procollagen mRNA. Biochem Biophys Res Commun. 1982;106(2):632–37. doi:10.1016/0006-291X(82)91157-3.
- Lyons BL, Schwarz RI. Ascorbate stimulation of PAT cells causes an increase in transcription rates and a decrease in degradation rates of procollagen mRNA. Nucleic Acids Res. 1984;12(5):2569–79. doi:10.1093/nar/12.5.2569.
- Gouveia RM, Connon CJ. The effects of retinoic acid on human corneal stromal keratocytes cultured in vitro under serum-free conditions. Invest Opthalmol Vis Sci. 2013;54(12):7483. doi:10.1167/iovs.13-13092.
- Kenney MC, Shih LM, Labermeir U, Satterfield D. Modulation of rabbit keratocyte production of collagen, sulfated glycosaminoglycans and fibronectin by retinol and retinoic acid. Biochim Biophys Acta. 1986;889:156–62. doi:10.1016/0167-4889(86)90099-6.
- Abidin FZ, Gouveia RM, Connon CJ. Application of retinoic acid improves form and function of tissue engineered corneal construct. Organogenesis. 2015;11(3):122–36. doi:10.1080/15476278.2015.1093267.
- Lynch AP, Ahearne M. Retinoic acid enhances the differentiation of adipose-derived stem cells to keratocytes in vitro. Trans Vis Sci Technol. 2017;6(1):6. doi:10.1167/tvst.6.1.6.
- Long CJ, Roth MR, Tasheva ES, Funderburgh ML, Smit R, Conrad GW, Funderburgh JL. Fibroblast growth factor-2 promotes keratan sulfate proteoglycan expression by keratocytes in vitro. J Biol Chem. 2000;275(18):13918–23. doi:10.1074/jbc.275.18.13918.
- Jester JV, Barry-Lane P, Cavanagh HD, Petroll WM. Induction of smooth muscle actin expression and myofibroblast transformation in cultured corneal keratocytes. Cornea. 1996;505–16. doi:10.1097/00003226-199609000-00011.
- Etheredge L, Kane BP, Hassell JR. The effect of growth factor signaling on keratocytes in vitro and its relationship to the phases of stromal wound repair. Invest Ophthalmol Vis Sci. 2009;50(7):3128–36. doi:10.1167/iovs.08-3077.
- Jester JV, Ho-Chang J. Modulation of cultured corneal keratocyte phenotype by growth factors/cytokines control in vitro contractility and extracellular matrix contraction. Exp Eye Res. 2003;77(5):581–92. doi:10.1016/s0014-4835(03)00188-x.
- Chen J, Guerriero E, Sado Y, Sundarraj N. Rho-mediated regulation of TGF-beta1-and FGF-2-induced activation of corneal stromal keratocytes. Invest Ophthalmol Vis Sci. 2009;50(8):3662–70. doi:10.1167/iovs.08-3276.
- Chen J, Wong-Chong J, SundarRaj N. FGF-2- and TGF-β1-induced downregulation of lumican and keratocan in activated corneal keratocytes by JNK signaling pathway. Invest Ophthalmol Vis Sci. 2011;52(12):8957–64. doi:10.1167/iovs.11-8078.
- Toti P, Tosi GM, Traversi C, Schürfeld K, Cardone C, Caporossi A. CD-34 stromal expression pattern in normal and altered human corneas. Ophthalmology. 2002;109(6):1167–71. doi:10.1016/s0161-6420(02)01042-4.
- Kim W-J, Mohan RR, Mohan RR, Wilson SE. Effect of PDGF, IL-la, and BMP2/4 on corneal fibroblast chemotaxis: expression of the platelet derived growth factor system in the cornea. Invest Ophthalmol Vis Sci. 1999;40:1364–72.
- Hoppenreijs VPT, Pels E, Vrensen GFJM, Felten PC, Treffers WF. Platelet-derived growth factor: receptor expression in corneas and effects on corneal cells. Invest Ophthalmol Vis Sci. 1993;34:637–49.
- Kim A, Lakshman N, Karamichos D, Petroll WM. Growth factor regulation of corneal keratocyte differentiation and migration in compressed collagen matrices. Invest Ophthalmol Vis Sci. 2010;51(2):864. doi:10.1167/iovs.09-4200.
- Kamiyama K, Iguchi I, Wang X, Imanishi J. Effects of PDGF on the migration of rabbit corneal fibroblasts and epithelial cells. Cornea. 1998;17(3):315–25. doi:10.1097/00003226-199805000-00013.
- Huang C, Jacobson K, Schaller MD. MAP kinases and cell migration. J Cell Sci. 2004;117:4619–28. doi:10.1242/jcs.01481.
- Jiménez C, Portela RA, Mellado M, Rodríguez-Frade JM, Collard J, Serrano A, Martínez AC, Avila J, Carrera AC. Role of the PI3K regulatory subunit in the control of actin organization and cell migration. J Cell Biol. 2000;151(2):249–62. doi:10.1083/jcb.151.2.249.
- Saltiel AR, Kahn CR. Insulin signalling and the regulation of glucose and lipid metabolism. Nature. 2001;414(6865):799–806. doi:10.1038/414799a.
- LeRoith D, Roberts CT. The insulin-like growth factor system and cancer. Cancer Lett. 2003;195(2):127–37. doi:10.1016/s0304-3835(03)00159-9.
- Haeusler RA, McGraw TE, Accili D. Biochemical and cellular properties of insulin receptor signalling. Nat Rev Mol Cell Biol. 2017;19(1):31–44. doi:10.1038/nrm.2017.89.
- Yanai R, Yamada N, Kugimiya N, Inui M, Nishida T. Mitogenic and antiapoptotic effects of various growth factors on human corneal fibroblasts. Invest Ophthalmol Vis Sci. 2002;43:2122–26.
- Sarenac T, Trapecar M, Gradisnik L, Rupnik MS, Pahor D. Single-cell analysis reveals IGF-1 potentiation of inhibition of the TGF-β/Smad pathway of fibrosis in human keratocytes in vitro. Sci Rep. 2016;6(1):34373. doi:10.1038/srep34373.
- Parsons WJ, Ramkumar V, Stiles GL. Isobutylmethylxanthine stimulates adenylate cyclase by blocking the inhibitory regulatory protein, Gi. Mol Pharmacol. 1988;34:37–41.
- Foster JW, Gouveia RM, Connon CJ. Low-glucose enhances keratocyte-characteristic phenotype from corneal stromal cells in serum-free conditions. Sci Rep. 2015;5:10839. doi:10.1038/srep10839.
- Andresen JL, Ledet T, Ehlers N. Keratocyte migration and peptide growth factors: the effect of PDGF, bFGF, EGF, IGF-I, aFGF and TGF-beta on human keratocyte migration in a collagen gel. Curr Eye Res. 1997;16(6):605–13. doi:10.1076/ceyr.16.6.605.5081.
- West-Mays JA, Cook JR, Sadow PM, Mullady DK, Bargagna-Mohan P, Strissel KJ, Fini ME. Differential inhibition of collagenase and interleukin-l a gene expression in cultured corneal fibroblasts by TGF-b, dexamethasone, and retinoic acid. Invest Ophthalmol Vis Sci. 1999;40:887–96.
- Massagué J, Wotton D. Transcriptional control by the TGF-beta/Smad signaling system. The EMBO Journal. 2000;19(8):1745–1754. doi:10.1093/emboj/19.8.1745.
- Rønnov-Jessen L, Petersen OW. A function for filamentous α-smooth muscle actin: retardation of motility in fibroblasts. J Cell Biol. 1996;134(1):67–80. doi:10.1083/jcb.134.1.67.
- Karamichos D, Hutcheon AEK, Zieske JD. Reversal of fibrosis by TGF-β3 in a 3D in vitro model. Exp Eye Res. 2014;124:31–36. doi:10.1016/j.exer.2014.04.020.
- Karamichos D, Hutcheon AEK, Zieske JD. Transforming growth factor-β3 regulates assembly of a non-fibrotic matrix in a 3D corneal model. J Tissue Eng Regen Med. 2011;5:228–38. doi:10.1016/j.exer.2014.04.020.
- Sriram S, Tran JA, Guo X, Hutcheon AEK, Lei H, Zieske JD. PDGFR a is a key regulator of T1 and T3’s differential effect on SMA expression in human corneal fibroblasts. Invest Ophthalmol Vis Sci. 2017;58:1179–86. doi:10.1167/iovs.16-20016.
- Lakshman N, Petroll WM. Growth factor regulation of corneal keratocyte mechanical phenotypes in 3-D collagen matrices. Invest Ophthalmol Vis Sci. 2012;53(3):1077–86. doi:10.1167/iovs.11-8609.
- Wilson SE, Mohan RR, Mohan RR, Ambrósio R, Hong J, Lee J. The corneal wound healing response: cytokine-mediated interaction of the epithelium, stroma, and inflammatory cells. Prog Retin Eye Res. 2001;20(5):625–37. doi:10.1016/S1350-9462(01)00008-8.