ABSTRACT
Aim: To test the feasibility of implanting human anterior lens capsules (HALCs) with porcine corneal endothelial cells (pCEC) in vivo in Göttingen minipigs and at the same time test the suitability of Göttingen minipig as model for endothelial keratoplasty.
Materials and Methods: Cell-carrier constructs of decellularized HALC with cultured (pCEC) were created for implementation in vivo. Eight Göttingen minipigs (6 months old) underwent surgery with descemetorhexis or removal of endothelium by scraping and implementation of HALC without (animal 1–4) and with (animal 5–8) pCEC. Follow-up examinations included optical coherence tomography (OCT) imaging (1,2 and 3 months) and slit-lamp examination (<1 week as well as 1,2 and 3 months).
Results: Intraoperative challenges included difficulties in maintaining an anterior chamber due to soft tissue and vitreous pressure, development of corneal edema and difficulties removing Descemet’s membrane because of strong adhesion to stroma. Therefore, descemetorhexis was replaced by mechanical scraping of the endothelium in animal 4–8. HALCs without pCEC were implanted in animal 1–4. Apposition to the back surface was not achieved in animal 1 and 3 because of corneal edema and poor visibility. Animal 5 was sacrificed because of a lens capsule tear. HALCs with pCEC were implanted in animal 6–8. Slit-lamp examination the first week revealed corneal edema in all animals, although mild in animals 4. One-month examination showed retrocorneal membranes with overlying corneal edema in all animals. Histology showed fibrosis in the AC and on the back surface of the cornea, compatible with the clinical diagnosis of retrocorneal membrane.
Conclusions: In conclusion, the minipig is not suitable for corneal transplantation studies in vivo because of intraoperative challenges and development of retrocorneal membrane postoperatively. For in vivo testing of the surgical handling and the therapeutic potential of tissue-engineered endothelial cell-carrier constructs other animal models are required.
Background
Endothelial keratoplasty is the most common treatment for corneal endothelial diseases, but worldwide shortage of donor corneas limits the number of transplantations that can be performed.Citation1 Tissue-engineered cornea analogs may provide an alternative to scarce human donor tissue,Citation2 but require in vivo testing in animals before introducing them into clinical practice. Both monkeys and rabbits have previously been used for this purpose,Citation3,Citation4 while pigs have been used for implantation of collagen inlays and testing of fish scale-derived biocorneas.5 Using pigs for preclinical studies is appealing because of the similarities between eyes in pigs and humans and a greater availability compared with monkeys.
Several corneal endothelial cell-carrier constructs were recently evaluated by Spinozzi et al. in terms of surgical in vitro handling and isolated human anterior lens capsules (HALC) proved to be the most suitable carrier in that study.Citation6 The purpose of this study was to test the feasibility of implanting tissue-engineered cell-carrier constructs (HALC with porcine corneal endothelial cells (pCEC)) in vivo in an animal model and test the suitability of Göttingen minipigs as a model for endothelial keratoplasty.
Materials and methods
Sample preparation
Isolation of pCECs was performed at Netherlands Institute for Innovative Ocular Surgery (NIIOS) as described previously.Citation6 After 2 passages, primary pCEC were seeded onto decellularized HALC. After cells reached confluence on the HALC carrier, samples were shipped to Aarhus in stabilization medium.Citation6,Citation7
Animal surgery
Ethical approval was obtained from the Danish Animal Experiments Inspectorate (2017-15-0201-01344). Surgery was performed in 8 six-month-old Göttingen Minipigs (Ellegaard, Denmark) under general anesthesia at Aarhus University’s animal facility Påskehøjgaard by an experienced cornea surgeon (JH). Preoperative baseline conditions were characterized by anterior segment optical coherence tomography (OCT) imaging (Telesto 2, Thorlabs). Surgical access to the anterior chamber (AC) was established via peripheral corneal incisions. Endothelium was removed either by descemetorhexis with a reversed Sinskey hook or mechanical scraping; both supported by either an AC maintainer (≈50cmH20) or an air-filled AC. HALCs were stained with trypan blue and injected with either an IOL-injector or a DMEK EndoGlide. After injection of the lens capsule into the AC, unfolding and positioning of the lens capsule against the posterior stroma were attempted. If unsuccessful, the lens capsule was left free-floating in the AC. Intracameral cefuroxime and subconjunctival triamcinolone was administered at the end of the procedure and the AC filled with a 20% SF6 gas-air mixture.
Follow up examinations
All animals underwent handheld (Kowa SL-17) slit-lamp examination (SLE) on day 3–7 as well as a SLE and OCT imaging in general anesthesia at 1 month, 2 months (animal 1–4) and 3 months (animal 1–3).
Histology
The eyes were harvested after the animals were sacrificed and corneal-scleral rims were excised and fixed in 4% formaldehyde. The specimens were cut in parallel slices, paraffin-embedded, sectioned and stained with either hematoxylin-eosin (HE), Weigert’s elastic Van Gieson or periodic acid-Schiff (PAS) stain.
Results
Animal surgery
Maintaining an AC proved difficult in all minipigs due to vitreous pressure and a rather soft cornea resulting in loose incisions. Descemetorhexis was attempted and achieved to some extent in minipigs 1–3. Descemet membrane was highly adherent to the stroma which complicated and prolonged the procedure, often resulting in corneal edema and therefore the endothelium was scraped off in the remaining animals (4–8). Minipig 5 was sacrificed immediately due to damage to the lens during surgery. Implementation of HALCs without pCEC was successful in minipigs 2 and 4, but unsuccessful in minipigs 1 and 3 because of poor visibility due to corneal edema and the HALC was left free-floating in the AC. HALCs with pCEC were successfully positioned onto the posterior stroma in minipigs 6–8, however, in minipig 6 the pCEC-HALC construct was placed upside-down with cells facing the posterior stroma.
Follow up examinations
Animal welfare was good in all animals during the study period as monitored by the animal caretakers.
SLE of all animals at day 3–7 revealed corneal edema, although in minipig 4 it was very mild. At one month all animals had developed a central retrocorneal membrane with overlying corneal edema (). The only exception was minipig 4 which had a clear central cornea but formation of a peripheral retrocorneal membrane around the main incision. Anterior synechiae were present in minipigs 1–3.
Figure 1. Photography at one month showing retrocorneal membrane/fibrosis formation in all eyes (yellow asterisk). In minipig 4 there is only a small membrane located around the main incision
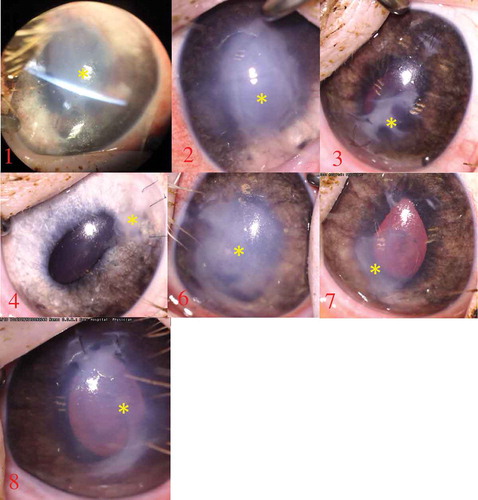
OCT imaging at one month confirmed the presence of retrocorneal membranes in all animals (). Although in minipig 4 it was less pronounced with only a small membrane located around an incision. In all eyes, the retrocorneal membranes were more prominent around the incisions. The central corneal thickness increased with >50% in all animals except minipig 4 ().
Figure 2. OCT imaging at one month showing retrocorneal membrane/fibrosis in all minipigs. Corneal edema was present in association with the membrane in all minipigs except for minipig 4, where retrocorneal membrane formation was confined to the area around the main incision. In minipigs 6 and 8 the membrane formed a web-like structure in the anterior chamber with adhesion to iris and lens
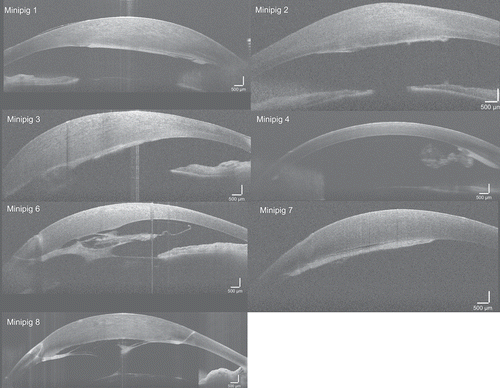
Figure 3. Examples of histological findings Top left: One month after endothelial removal in minipig 4 by scraping histology revealed endothelial cells present in the same area. Top right: Minipig 3 showed fibrosis with angiogenesis on the posterior cornea after descemetorhexis. Middle left: Fibrosis in the anterior chamber (minipig 6). Middle right: Fibrotic reaction around a partially detached HALC (minipig 7). Endothelial cells can be seen on the HALC. Bottom: Reactive changes and cellular infiltration between HALC and host cornea . No endothelial cells can be seen on the HALC (minipig 8). Arrow head = endothelial cells, arrow = human anterior lens capsule (HALC), asterisk = fibrosis DM = Descemet’s membrane
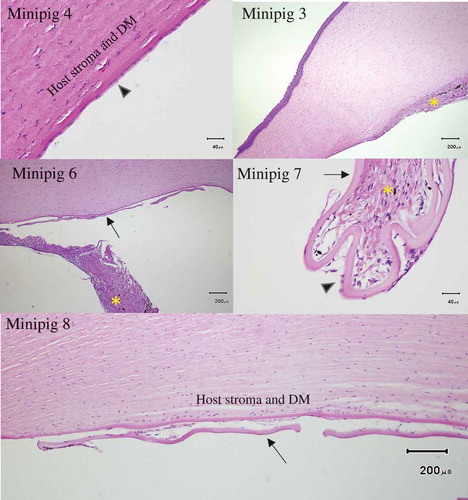
Apposition of HALC was difficult to confidently determine by OCT imaging as they were presumably covered by retrocorneal membranes. In minipig 4 the bare HALC had detached.
Histology
Histology revealed that descemetorhexis (minipigs 1–3) induced a fibrotic response with associated angiogenesis. Scraping of the endothelium without persistent apposition of the HALC against the posterior cornea (minipig 4) resulted in minor localized fibrosis. The scraped area was covered by endothelial cells () and showed no obvious difference in cell count and morphology compared to control (minipig 3, non-operated eye). Some fibrosis on the posterior surface and in the AC was present in animals (6–8) that had the endothelium removed by scraping and a HALC successfully positioned against the posterior cornea. Examination of the interface between HALC and posterior cornea revealed signs of a reactive response with cellular infiltration that included both fibroblasts but also other cells of unknown identity that might be transformed endothelial cells. There were endothelial cells remaining on the lens capsules but the numbers appeared sparse.
Figure 4. Central corneal thickness (µm) in all minipigs before and after surgery. The animals are color coded according to procedure and outcome. Black: descemetorhexis and implantation of lens capsule without endothelial cells. Blue: scraped endothelium with detached capsule without endothelial cells. Green: scraped endothelium and lens capsule with endothelial cells but positioned upside-down. Red: scraped endothelium and lens capsule placed correctly with endothelial cells facing the anterior chamber. Follow-up examinations were reduced in animals 4–8 due to minimal clinical changes after one month in the first cohort of animals undergoing surgery (animals 1–3)
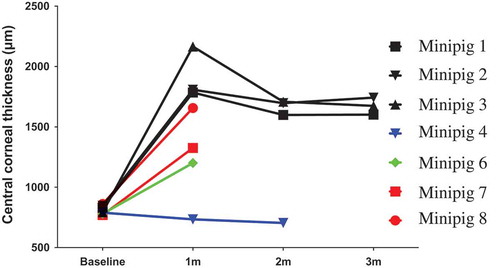
Discussion
The aim of this study was to test a tissue-engineered endothelial cell-carrier construct in vivo and to test the suitability of the minipig as a model for endothelial keratoplasty. Owing to the challenges encountered with using the minipig, the main outcome of the study regards the suitability of the minipig for transplant-related corneal research.
Our study present intraoperative and postoperative challenges with the minipig which makes it a less ideal model for endothelial keratoplasty. Descemetorhexis is routinely done in humans, although remnants can remain and may pose a risk for graft detachment.Citation8 In the minipig descemetorhexis was difficult to perform. Therefore, the central endothelium was removed by scraping in four animals. The two procedures, however, seem to result in different outcomes: minipig 1 (descemetorhexis) developed a central retrocorneal membrane and corneal edema whereas minipig 4 (scraping) had a clear cornea with only a minor retrocorneal membrane around the main incision and evenly distributed endothelial cells on histology. This suggests that, contrary to primates,Citation4, 5 pCEC can proliferate in vivo since it is unlikely that migration of remaining endothelial cells can compensate for such a large loss of cells.
Retrocorneal membranes developed in experiments with HALCs with and without pCEC, suggesting that it is not a pure rejection response to the transplanted endothelial cells. Retrocorneal membrane formation seemed to originate from the corneal incisions and may develop via either (1) epithelial downgrowth (2) fibroblastic or stromal (keratocytic) downgrowth, or (3) fibrous metaplasia of the corneal endothelium.Citation9 Any of these mechanisms may be involved here, though stromal keratocytes likely play the major role since the retrocorneal membrane formation seemed to originate from areas where the stroma had been traumatized, i.e. from the corneal incisions or over the descemetorhexis area. Recently, a studyCitation10 demonstrated that in pig-to-monkey penetrating keratoplasty all monkeys developed retrocorneal membranes due to activation of stromal keratocytes in the pig graft. These findings also suggest that the pig/minipig is prone to developing retrocorneal membranes and that this limits the use of pigs/minipigs for corneal surgery studies.
Regarding the cell-carrier constructs, information obtained from this study was limited by the surgical difficulties and the strong wound-healing reaction. However, it was possible to sufficiently stain the cell-carrier constructs for visualization, inject them into the anterior chamber and position them against the posterior stroma. Histology images suggest a low number of retained pCEC on the HALC carrier after one month, possibly because of the surgical trauma and/or retrocorneal membrane formation. In addition, it suggests that host CEC did not proliferate/migrate onto the HALCs.
Endothelial keratoplasty is target for substantial research interest and there is a need for a good animal model. Many of the intraoperative challenges that can occur in a new model can likely be handled by alterations of the techniques used, but generating results with translational potential is important. Therefore, we suggest three criteria for a good model; eye bulb size similar to humans, removable descemet’s membrane and non-proliferative CECs. Monkeys fulfil these criteria but are limited by accessibility. Rabbits are likely the best option. Although rabbits have proliferative CEC this may not be a problem as long as study duration is not too long (< 1 month) and graft CEC are labelled (e.g. DiI).
In conclusion, the minipig is not an ideal animal model for in vivo testing of tissue-engineered endothelial cell-carrier constructs and for endothelial keratoplasty studies in general.
Declaration of interest
No conflict of interest
Additional information
Funding
References
- Gain P, Jullienne R, He Z, Aldossary M, Acquart S, Cognasse F, Thuret G. Global survey of corneal transplantation and eye banking. JAMA Ophthalmol. 2016;134(2):167–7. doi:10.1001/jamaophthalmol.2015.4776
- Mimura T, Yamagami S, Amano S. Corneal endothelial regeneration and tissue engineering. Prog Retin Eye Res. 2013;35:1–17. doi:10.1016/j.preteyeres.2013.01.003
- Mimura T, Yamagami S, Yokoo S, Usui T, Tanaka K, Hattori S, Irie S, Miyata K, Araie M, Amano S. Cultured human corneal endothelial cell transplantation with a collagen sheet in a rabbit model. Invest Ophthalmol Vis Sci. 2004;45(9):2992–7. doi:10.1167/iovs.03-1174
- Koizumi N, Sakamoto Y, Okumura N, Okahara N, Tsuchiya H, Torii R, Cooper LJ, Ban Y, Tanioka H, Kinoshita S. Cultivated corneal endothelial cell sheet transplantation in a primate model. Invest Ophthalmol Vis Sci. 2007;48(10):4519–26. doi:10.1167/iovs.07-0567
- Chen SC, Telinius N, Lin HT, Huang MC, Lin CC, Chou CH, Hjortdal J. Use of fish scale-derived biocornea to seal full-thickness corneal perforations in pig models. PLoS One. 2015 Nov 24;10(11):e0143511.
- Spinozzi D, Miron A, Bruinsma M, Dapena I, Lavy I, Binder PS, Rafat M, Oellerich S, Melles GRJ. Evaluation of the suitability of biocompatible carriers as artificial transplants using cultured porcine corneal endothelial cells. Curr Eye Res. 2019;44(3):243–9. doi:10.1080/02713683.2018.1536215
- Spinozzi D, Miron A, Bruinsma M, Lie JT, Dapena I, Oellerich S, Melles GRJ. Improving the success rate of human corneal endothelial cell cultures from single donor corneas with stabilization medium. Cell Tissue Bank. 2018;19:9–17. doi: 10.1007/s10561-017-9665-y
- Brockmann T, Brockmann C, Maier AK, Schroeter J, Pleyer U, Bertelmann E, Joussen AM, Torun N et al. Clinicopathology of graft detachment after Descemet’s membrane endothelial keratoplasty. Acta Ophthalmol. 2014;92(7):e556–61 doi:10.1111/aos.12419
- Bayyoud T, Rohrbach JM, Bartz-Schmidt KU, Thaler S. Histopathology of a retrocorneal membrane after Descemet membrane endothelial keratoplasty: a case report. J Med Case Rep. 2019;13(1):31–4. doi:10.1186/s13256-018-1960-0
- Lee W, Mammen A, Dhaliwal DK, Long C, Miyagawa Y, Ayares D, Cooper DKC, Hara H. Development of retrocorneal membrane following pig-to-monkey penetrating keratoplasty. Xenotransplantation. 2016;24(1):e12276–18. doi:10.1111/xen.12276