ABSTRACT
Purpose/Aim
Dry eye (DE) disease is a multifactorial disease in which uncontrolled inflammation can lead to corneal epithelium lesions and symptoms of discomfort. The aim of the present study was to evaluate the efficacy of two cyclosporine emulsions in a mouse model of DE with corneal epithelium lesions.
Materials and Methods
Six- to 9-week-old female C57BL/6 N mice were housed in a controlled-environment room to induce DE. Following DE development, mice were instilled with: QD 0.1%CsA cationic emulsion (CaEm), BID 0.05%CsA anionic emulsion (AEm), or left untreated. Aqueous tear production and corneal epithelium lesions were assessed throughout the experiment. At the end of the treatment period, left eyes were sampled, fixed, and stained for histology, while the cornea, conjunctiva, and lacrimal gland of right eyes were sampled for transcriptomic analysis.
Results
Corneal lesion scores were reduced by 10.4%, 18.4%, and 10.9% at day 6, 10, and 14, respectively, with CaEm (QD), and by 2.6%, 3.0%, and 5.5% at day 6, 10, and 14, respectively, with AEm (BID). Histology demonstrated that 7 out of 10 DE mice presented moderate to severe ocular lesions, while only 2 and 5 out of 10 mice presented slight to moderate ocular lesions when treated with the CaEm (QD) and AEm (BID), respectively. The transcriptomic profile analysis suggests that a different set of inflammatory genes are modulated in the cornea, conjunctiva, and lacrimal gland upon DE development. In addition, the two emulsions distinctively modulate the gene expression profile.
Conclusions
This study demonstrates that both emulsions were effective at reducing corneal lesions, with the CaEm (QD) being slightly better than the AEm (BID). Interestingly, this study suggests that ocular tissues may not respond similarly to a dry environment and that a different set of genes is modulated by the two formulations in the ocular tissues.
Introduction
Dry eye disease (DED) is a complex and multifactorial disease that affects close to 30% of the general population from different countries all over the five continents.Citation1–4 Patients afflicted by DED present signs of ocular surface lesions and symptoms of variable severity and intensity, which do not necessarily correlate. The present treatment strategies for DED signs and symptoms use various type of artificial tears (ATs)Citation5,Citation6 or eye drops containing anti-inflammatory compounds such as glucocorticoids (methyl-prednisolone, fluorometholone),Citation7 immunosuppressant for their anti-inflammatory properties (cyclosporine A (CsA) and tacrolimus)Citation8–11 and anti-inflammatory compounds (such as lifitegrast).Citation12 Polymer-based (such as carboxymethyl cellulose, hydroxypropyl guar, hyaluronic acid) aqueous and lipid-based (such as cationic and anionic emulsions) ATs are the first line therapies used for the management of mild to moderate symptoms of DED and allow only for a temporary relief of the symptoms.Citation6 Prescription medicine containing compounds with potent anti-inflammatory activity is generally restricted to the more severe DED patients presenting severe signs of ocular surface alterations that did not improve under ATs treatment.Citation13 Cyclosporine A is the most frequently used anti-inflammatory compound in eye drops for the management of signs and symptoms of DED patients. It is formulated as a cationic emulsion (0.1% CsA, CaEm, Santen),Citation11,Citation14,Citation15 or as an anionic emulsion (0.05% CsA, AEm, Allergan),Citation10,Citation16 for the treatment of severe keratitis in DED patients that do not improve upon treatment with ATs or for the restoration of tear secretion in DED patients, respectively.
Animal studies in a dry eye mouse model have demonstrated that CsA formulations were effective at reducing the severity of the corneal epithelium lesionsCitation17–19 or at restoring tear secretion.Citation20 However, in these studies, CsA eye drops are generally instilled at dosing regimens that are higher than the recommended human posology, and at volumes substantially in excess, when considering the small size of the eye in the mouse. In the proposed study, the two CsA formulations (the cationic and anionic emulsions of CsA) were instilled in a mouse model of dry eye at their recommended human posology; i.e., one drop a day (QD) for the CaEm of 0.1% CsA, and twice a day (BID) for the AEm of 0.05% CsA.Citation20 This study aimed to evaluate the final formulations of the CsA with its vehicle excipients on the management of the ocular surface lesions in an accepted translational murine model of dry eye. Indeed, excipients appear to have some positive/beneficialCitation21,Citation22 or negative/side effectsCitation23 depending on their concentration in the formulations, and on their dose on the eye upon instillation of the eye drops.
The aim of the present study was to characterize the efficacy profile of the cationic and anionic emulsions of CsA on the corneal epithelial lesions and explore the effects of the final formulations on the expression of immunology-related genes in the lacrimal gland, conjunctiva, and cornea of the treated animals.
Methods
Animals
Forty pigmented C57BL/6 N mice aged 6 to 9 weeks (Charles River Laboratories, Saint Germain Nuelles, France) were used in this study. The experimental protocol was in accordance with the European Committee directives (authorization number DAP64). All animals were treated according to the Directive 2010/63/UE European Convention for the Protection of Vertebrate Animals used for Experimental and Other Scientific Purposes and to the Association for Research in Vision and Ophthalmology (ARVO) statement for the Use of Animals in Ophthalmic and Vision Research.
Experimental procedure
Ten mice were assigned to the healthy control group, the other thirty mice were placed in a controlled environmental room (CER) for 14 days (temperature: 20–24°C; relative humidity: 27 ± 2%; airflow: 15 L/min) (), and treated with transdermal scopolamine patch administration (0.5 mg/72 h, Scopoderm® TTS, Novartis, Rueil-Malmaison, France) starting on day 0, and changed every other day, following a procedure as previously described.Citation24–27
Figure 1. Schematic representation of the experimental design of the study. Clinical evaluation, CFS, PRT were performed just before the mice were put in the dry environment. SP, scopolamine patch, CFS, corneal fluorescein staining, PRT, phenol red thread
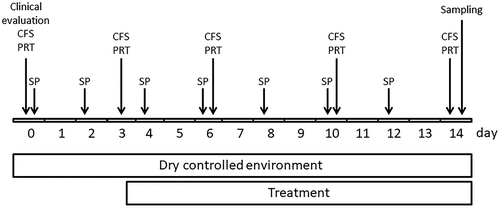
The thirty mice were then randomly assigned to the three DED groups (n = 10 per group) based on the corneal fluorescein staining (CFS) values obtained after 3 days of controlled environmental conditions (before any treatment): the DED baseline group (group 1; DED control group) received no treatment, and two treatment groups treated with cyclosporine A emulsions. The treatment with both CsA emulsions covered 10 days and started after a 3-day dry eye induction period (). Group 2 was treated with one 5 µL drop of 0.1% cyclosporine A CaEm (Santen, Evry, France) instilled once a day (QD, at 4 PM) in both eyes. Group 3 was treated with one 5 µL drop of 0.05% cyclosporine A AEm (Allergan, Irvine, CA, USA) instilled twice a day (BID, at 10 AM & 4 PM) in both eyes. The treatments were encoded, and the group allocation was blinded to the technician administering the treatment, and to the researcher assessing the outcome of the experiment. Group identification was uncovered at the end of the analysis.
Measurement of tear volume and cornea alterations
Tear volume was measured with phenol red thread (PRT) test (Zone-Quick, Lacrimedics, Eastsound, WA, USA), as described previously.Citation28 Corneal fluorescein staining (i.e., punctate keratitis, evaluated using the National Eye Institute scheme) was performed before dry eye induction (Day 0), and during the experiment at days 3, 6, 10, and 14 () according to a previously published protocol.Citation28 Briefly, 0.5 µL of a 0.5% fluorescein sodium solution (Fluoresceine Faure, 0.4-mL unit-dose vials, Novartis Pharma SAS, France) were instilled into the inferior conjunctival sac using a micropipette. The cornea was examined through a biomicroscope by light passing through a cobalt blue filter. The stained area was assessed and graded using the grading system from the NEI/Industry Workshop guideline.Citation29 The CFS scoring provided a stepwise categorization of the cornea; the cornea was divided into five sectors (central, superior, inferior, nasal, and temporal), within each sector the score based on the density of stained dots on a 0–3 scale (the more dots, the higher the score), for a total maximal score of 15.
Animal euthanasia and tissues sampling
The healthy untreated control mice and DED mice were euthanized at the end of the experiment by a systemic injection of overdosed pentobarbital, as recommended for euthanasia by the European authorities (French decree no. 2013–118).Citation30 Immediately after euthanasia, the left eyeballs were sampled (on day 14, ) and prepared for histology analysis, while the whole cornea, conjunctiva, and lacrimal gland from the right eyes were collected and immediately stored at −80°C for further total RNA extraction and subsequent Nanostring® analysis.Citation31
Histology
The left eyeballs were dehydrated, embedded in paraffin wax. The eyeballs (three sections: one nasal, one central, and one temporal per eye with back up slides) were cut and each section was stained (Hematoxylin-Eosin-Safran and Periodic Acid Schiff). The eyes were then microscopically examined by a histopathologist in a blind manner.
RNA isolation and integrity and quality measurements
Total RNAs were extracted from whole corneas, conjunctivas, and lacrimal glands by adding TRIzol (Tri Reagent®, Zymo, Irvine, CA, USA) to the frozen tissues and following the manufacturer’s protocol. Each tissue was prepared individually and eyes were not pooled during the preparation. A High-Pure-RNA-tissue kit (Roche Diagnostics GmbH, Mannheim, Germany) was used for total RNA purification according to the manufacturer’s instruction. RNA yield and purity were assessed using NanoDrop ND-100 Spectrophotometer (NanoDrop technologies, Rockland, DE, USA). Total RNA integrity was evaluated with the Agilent 2100 bioanalyzer (Agilent Technologies, Wilmington, DE, USA) according to the manufacturer’s specifications. An RNA integrity number (RIN) greater than 7 was considered as an acceptable quality criterion for the analysis.
NanoString® nCounter assay
The transcriptomic profile was measured in the whole cornea, conjunctiva, and lacrimal gland through a multiplexed hybridization assay and specific fluorescent barcode probes with no amplification step. Eyes were not pooled, and each tissue was analyzed individually. Gene expression was measured with nCounter® Mouse Immunology panel (NanoString Technologies, Seattle, WA, USA) on the NanoString nCounter Analysis System (NanoString Technologies, Seattle, WA, USA).Citation31
The code set is constituted of biotinylated capture probes and reporter probes attached to color barcode tags for the 547 inflammation and immunology-related mouse genes and 14 internal reference genes. Briefly, purified RNA was diluted in nuclease-free water to 20 ng/μL, making a final assay dose of 100 ng. Samples were incubated 16–22 h at 65°C as per the manufacturer’s standard protocol to ensure hybridization with reporter and capture probes. After hybridization, the samples were processed in the PrepStation and counted in the digital analyzer.
NanoString® data analysis
The number of counts from RCC files of each gene in the code set was analyzed using nSolver 4.0 (NanoString, Technologies, Seattle, WA, USA) and Microsoft Excel softwares. The number of transcript copies was then normalized using the geometric mean of six reference genes and was log2-transformed for further analysis.
Statistical analysis
The statistical analysis was performed using the software GraphPad Prism 6.0b. CFS and PRT data were analyzed with Tuckey’s multiple comparison test. Statistical significance was set at a p value of 0.05. Results are presented as mean ± SD.
Gene expression data were log-transformed and an unpaired t-test with unequal variance was then used to assess the significance (p < .05) in the fold changes between healthy, DED-untreated, and DED-treated mice.
Results
Effects of CsA emulsions on corneal lesions (CFS scores) and lacrimation in the mouse DED model
Healthy control animals housed under normal temperature and air conditions have their CFS scores ranging from 2 to 5 over the 14 days of the experiment. These score values are similar to the CFS scores at baseline (i.e., before dry eye induction) for the three experimental groups (). After 3 days in the control environment room (CER) and scopolamine treatment, and before treatment initiation the CFS score increased to 12.4 ± 2.3, 12.5 ± 2.0, and 12.8 ± 1.9 in the untreated baseline DED, DED + CaEm, and DED + AEm groups, respectively. From day 3 to day 14, the CFS score remains elevated and slightly increased for the untreated DED animals. Upon QD treatment with the CaEm of CsA, the CFS score was significantly reduced to 10.0 ± 2.0 (p < .001), and 10.8 ± 1.4 (p < .05) at day 10, and 14, respectively (). By contrast, the CFS scores were only marginally decreased, without reaching any statistical significance by BID instillations of the AEm of CsA, down to a CFS score value of 11.7 ± 1.7 at day 14. The maximum CFS score reduction upon CsA treatments was reached at day 10 (−18.4 ± 19.9%, p < .05 vs untreated mice) following QD instillations of the CaEm of CsA, and at day 14 for the AEm of CsA (BID) (−5.5 ± 25.5%). Untreated DED animals have their CFS scores worsened by approximately 7% to 8% () from day 3 to 14.
Figure 2. (a) Corneal fluorescein staining scores over time in DED mice treated with CsA emulsions. (b) CFS score change over time. Data are mean ± SD. Tukeys’s multiple comparison test was used for the statistical analysis. (c) Representative CFS images of healthy and DED mice. *; p < .05, **; p < .01, ***; p < .001, ****; p < .0001. CaEM, cationic emulsion, AEm, anionic emulsion
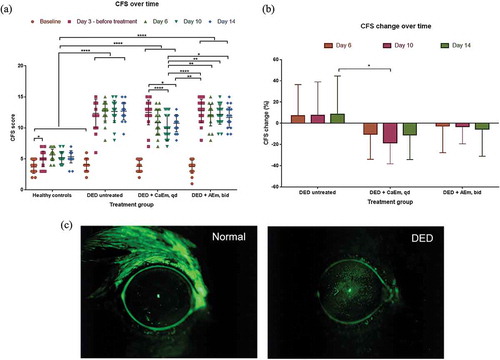
In healthy animals, tear secretion values were quite variable and ranged from 2 to 7 mm, with mean values ranging between 2.6 ± 1.2 and 3.8 ± 0.8 mm. Upon dry eye induction and scopolamine treatment, the tear secretion decreased with mean values below 1 mm at days 3, 6, and 10 for all DED animal groups. Interestingly, the lacrimation values are more centered on the mean except at day 14 following CsA treatments ().
Ocular surface histology following DED induction and subsequent treatment with CsA emulsions
At the end of the experimental period, the left eyes were sampled and prepared for histology analysis. The data presented in confirmed that DED induction was followed by histological changes in the conjunctival and corneal epithelia. The more frequently observed alterations following DED development were the thinning of the cornea and conjunctiva epithelium as well as a hyperplastic appearance of the corneal epithelium (, and ). The total number of clinical observations in the DED-untreated animal amounts to 28. Upon treatment with CsA emulsions, this number drastically decreases, reaching as few as 2 observations in mice treated with the CaEm of CsA and 5 observations in mice treated with the AEm of CsA. Indeed, eight out of 10 mice from the CaEm of the CsA group did not present any pathologic sign. In the AEm of CsA group, six mice had no pathologic signs at day 14.
Table 1. Summary of histology results at day 14
Exploration of the signaling pathways modulated by the DED environment
For this evaluation the transcriptomic profile from untreated DED animals (DED baseline) was compared to the transcriptomic profile of healthy animals (healthy baseline). At the end of the treatment period, the right eyes were collected and the cornea, conjunctiva, and lacrimal gland were sampled and prepared for total mRNA analysis with the NanoString® technology and mouse immunology panel. Briefly, this technology allows for the direct counting of the copy number of the mRNAs of interest without the need for an amplification step beforehand.Citation31 The use of color-coded probes highly specific to a specified mRNA sequence can directly assess the number of target mRNA copies present in the total mRNA extract purified from the tissue just by counting the colored probes. Whole tissues, i.e., the different cell layers from cornea and conjunctiva epitheliums, and lacrimal gland, as well as the infiltrating immune cells, were used for total mRNA preparation. Hence, genes expressed by both the tissues and the infiltrating immune cells can be detected. The large dynamic range of this detection technique renders it possible to detect mRNA with very low to very high copy numbers.
The Venn diagram presented in indicates the number of genes significantly modulated (p < .05) in the cornea (n = 36), conjunctiva (n = 18), and lacrimal gland (n = 48). Interestingly, there was only a few set of genes that were concomitantly modulated in the cornea and conjunctiva (n = 2, SMAD5, STAT1) and in the cornea and lacrimal gland (n = 4, FADD, NT5E, CXCL1, CD164). No genes were modulated at the same time in the conjunctiva and lacrimal gland or in the three tissues (). Even though very few genes were concomitantly modulated in the different ocular tissues, the same signaling pathways appear to be modulated upon DED development (). presents the top five signaling pathways modulated in the three tissues. It is noteworthy that the host–pathogen interaction signaling pathway contributes with 26, 16, and 7 genes in the lacrimal gland, cornea, and conjunctiva of DED animals, respectively; suggesting an important role for this pathway in DED. Whether this observation is specific to the mouse needs to be confirmed in human patients, and might support in-depth exploration of the role of the ocular surface microbiome in DED and meibomian gland dysfunctions.Citation32–34 In the cornea, in addition to the pathways present in the lacrimal gland and conjunctiva, two additional pathways: transcriptional regulation and the NLR signaling pathways, reached the top five position (with seven genes modulated in each pathway). It is important to note that all the genes identified are present in more than one pathway (see supplementary tables S1 to S3).
Table 2. Top five pathways modulated by the DED environment in ocular surface tissues
Genes modulated in the ocular tissues of DED mice upon treatment with CsA emulsions
It appeared that the different ocular tissues did not respond in a similar manner to CsA treatments. More genes were significantly modulated (p < .05) by the two CsA treatments in the lacrimal gland, than in the conjunctiva or cornea () of DED mice. There was only a reduced number of genes that were significantly modulated by the two treatments at the same time; 39 genes in the lacrimal gland, two (KIR3DL2, IFNAR2) in the conjunctiva, and one (CLEC4E) in the cornea. Supplement tables S4 to S6 list the genes modulated by the CsA treatments in the lacrimal gland, conjunctiva, and cornea, respectively. Genes identified as part of the host–pathogen interaction signaling pathway that are modulated by the two treatments are in italic in tables S4 to S6.
Table 3. Genes significantly modulated by CsA treatments in DED mice ocular tissues following induction of dry eye
Discussion
Dry eye disease is a complex and multifactorial disease that affects the ocular surface unit that contains the cornea, conjunctiva, and lacrimal gland.Citation35–38 These three tissues have the same embryological origin, as they are derived from the surface ectoderm. It is expected that these different tissues behave, to some extent, similarly upon normal or stressed conditions, and under treatment. Interestingly, it appears that these three tissues have their genes modulated in a slightly different manner upon DED development (see supplementary Tables S1 to S6), even though the same main signaling pathways appear to be modulated. There is growing evidence that the ocular surface microbiome plays a role in DED,Citation33,Citation39 hence the host–pathogen interaction signaling pathway appears to be critical for the management of DED. Anti-inflammatory treatment with CsA is already able to modulate a number of the genes from this pathway, however other targets from this pathway might represent alternative targets for a better management of chronic DED.
CsA treatment that modulates inflammatory pathways is effective at reducing corneal epithelium lesions (as evidenced by the reduction of CFS scores over time). Topical CsA eye drops have been widely used for the management of ocular surface diseases such as DED, Sjögren syndrome, or allergic conjunctivitis such as VKC.Citation40 As an anti-inflammatory compound, CsA blocks the production of pro-inflammatory cytokines and interleukins, such as IL2, and hence contributes to the management of signs and symptoms of DED.Citation41 However, it seems that the CaEm of CsA (0.1% CsA, QD) is slightly superior to the AEm of CsA (0.05% CsA, BID) at modulating the inflammatory response in vitro,Citation42 and at reducing the CFS scores. This observation was also supported by the NOD mouse model of dry eye data by Burade et al.,Citation20 and by clinical observations.Citation43 It is worth noting that in the NOD mouse model of dry eye QD instillations of the CaEm of CsA were able to restore tear secretion in a manner similar to BID instillations of a 0.09% CsA nanomicellar solution, with a statistical significance (p < .05) in favor of the BID nanomicellar solution instillations reached only after 60 days of treatment.Citation20 Patients with a mild to moderate DED condition (mean CFS score of 2.6–2.7 (Oxford scale) at baseline) have their corneal epithelium lesions improved by a CFS reduction of 0.84 with the AEm CsA,Citation10 while patients with severe keratitis (CFS score of 4 or higher at baseline) have their CFS improved by a mean CFS reduction of 1.76 upon treatment with the CaEm of CsA.Citation11,Citation44 Indeed, this is reflected by the difference in indication for both products: the CaEm of CsA is prescribed for the management of severe keratitis in DED patients that do not improve upon treatment with ATs, while the AEm of CsA indication is for the restoration of tear secretion in DED patients.Citation43
It is worth noting that only a very few set of genes is modulated at the same time by the two CsA formulations in the cornea, conjunctiva, and lacrimal gland. Both treatments deliver the same daily dose of CsA, as demonstrated by pharmacokinetic analysis in the rabbit,Citation45 however, it is evident that the two formulations do not have the same effects on the transcriptomic profile of the ocular tissues analyzed in this study. One of the hypotheses is that the excipients that compose the emulsion may also directly modulate the expression of some of the genes, or interact with these gene products,Citation22 and consequently may have an impact (either positive or negative) on the overall efficacy of the formulation (in addition/subtraction to the positive action of the active principle: CsA). The analysis of both emulsions’ empty vehicles would have allowed for a better dissection of the individual contribution of cyclosporine vs the excipients. Unfortunately, this was not technically feasible in the present study. The possibility that excipients may possess negative effect in a formulation is acknowledged by regulatory agencies that recommend in their guidelines for the safety evaluation of new drug products to test the final formulation (i.e., the association of the active principle with its excipient vehicle compounds) in at least 28-day local tolerance studies for eye drop prescription products. As a consequence, the possibility that these excipients may possess beneficial positive effects, that can contribute to the overall efficacy of a prescription medicine formulation, cannot be ruled out if it is agreed that negative effects exist and may lead to side effects with safety issues. Evidence supporting the positive effects of excipients for eye drop formulation efficacy were recently published and help explain the good clinical performance of the CaEm containing low doses of cetalkonium chloride and tyloxapol.Citation21,Citation46 On the other hand, the presence of potentially cytotoxic excipient, such as castor oil, may explain the ocular discomfort and reddened conjunctiva observed in both rabbit and dog studies with both the vehicle and the final formulation of the AEm of CsA, and the ocular burning sensations reported by patients in clinical trials, including patients receiving the vehicle. These tolerability issues might be explained by the presence of 1.25% castor oil in the AEm and its vehicle, which has been demonstrated to exert cytotoxic effects on conjunctival cells.Citation23 Discomfort ocular signs may also be related to the amount of surfactants delivered upon instillation (and to the frequency of instillations) of the eye drops. The surfactant/oil ratio in the AEm of CsA is 0.8 (1% polysorbate 80 for 1.25% castor oil). This is relatively high compared to the 0.2 surfactant/oil ratio found in the CaEm of CsA (0.3% tyloxapol, 0.1% poloxamer, and 0.005% cetalkonium chloride for 2% medium-chain triglyceride oil), even more considering the BID posology for the AEm of CsA vs. the QD posology for the CaEm of CsA.
Conclusion
This study demonstrates that the DED environment differentially affects the transcriptomic profile in the cornea, conjunctiva, and lacrimal gland, which might potentially explain why some treatment might have little efficacy on DED signs (i.e., CFS and corneal epithelium lesions) while modulating tear biomarkers (secreted from the lacrimal gland). In addition, this study suggests that the excipients, while generally considered as neutral and without any positive or negative effect, can, when present at the optimal concentration contribute to the overall efficacy of eye drop drug products or when present in too large a concentration may alter the beneficial effect of the active principle. Further gene expression evaluation in conjunctival imprints from patients with signs and symptoms of DED will be necessary to further explore the present transcriptomic observations and their implication in disease (i.e., identify specific disease biomarkers vs diagnostic or efficacy biomarkers) and for chronic DED management.
Supplemental Material
Download Zip (829.6 KB)Disclosure statement
The authors wish to acknowledge the following financial interests:
P.D., J-S.G.: Employee – Santen SAS
T.N.: Employee – Santen Pharmaceutical Co., Ltd
E.G., L.F.: Employee – Iris Pharma
S.B.: none
Supplementary material
Supplemental data for this article can be accessed on the publisher’s website.
Additional information
Funding
References
- Ferrero A, Alassane S, Binquet C, Bretillon L, Acar N, Arnould L, Muselier-Mathieu A, Delcourt C, Bron AM, Creuzot-Garcher C. Dry eye disease in the elderly in a French population-based study (the montrachet study: maculopathy, optic nerve, nutrition, neurovascular and heart diseases): prevalence and associated factors. Ocul Surf. 2018;16:112–19.
- Song P, Xia W, Wang M, Chang X, Wang J, Jin S, Wang J, Wei W, Rudan I. Variations of dry eye disease prevalence by age, sex and geographic characteristics in china: a systematic review and meta-analysis. J Glob Health. 2018;8:020503.
- Stapleton F, Alves M, Bunya VY, Jalbert I, Lekhanont K, Malet F, Na KS, Schaumberg D, Uchino M, Vehof J, et al. Tfos dews ii epidemiology report. Ocul Surf. 2017;15(3):334–65.
- Uchino M. What we know about the epidemiology of dry eye disease in japan. Invest Ophthalmol Vis Sci. 2018;59:DES1–DES6.
- Alves M, Fonseca EC, Alves MF, Malki LT, Arruda GV, Reinach PS, Rocha EM. Dry eye disease treatment: a systematic review of published trials and a critical appraisal of therapeutic strategies. Ocul Surf. 2013;11:181–92.
- Moshirfar M, Pierson K, Hanamaikai K, Santiago-Caban L, Muthappan V, Passi SF. Artificial tears potpourri: a literature review. Clin Ophthalmol. 2014;8:1419–33.
- Cutolo CA, Barabino S, Bonzano C, Traverso CE. The use of topical corticosteroids for treatment of dry eye syndrome. Ocul Immunol Inflamm. 2019;27:266–75.
- Moscovici BK, Holzchuh R, Chiacchio BB, Santo RM, Shimazaki J, Hida RY. Clinical treatment of dry eye using 0.03% tacrolimus eye drops. Cornea. 2012;31:945–49.
- Moscovici BK, Holzchuh R, Sakassegawa-Naves FE, Hoshino-Ruiz DR, Albers MB, Santo RM, Hida RY. Treatment of sjogren’s syndrome dry eye using 0.03% tacrolimus eye drop: prospective double-blind randomized study. Contact Lens Anterior Eye. 2015;38:373–78.
- Sall K, Stevenson OD, Mundorf TK, Reis BL. Two multicenter, randomized studies of the efficacy and safety of cyclosporine ophthalmic emulsion in moderate to severe dry eye disease. Csa Phase 3 Study Group. Ophthalmology. 2000;107:631–39.
- Leonardi A, Van Setten G, Amrane M, Ismail D, Garrigue J-S, Figueiredo FC, Baudouin C. Efficacy and safety of 0.1% cyclosporine a cationic emulsion in the treatment of severe dry eye disease: a multicenter randomized trial. Eur J Ophthalmol. 2016;26:287–96.
- Nichols KK, Donnenfeld ED, Karpecki PM, Hovanesian JA, Raychaudhuri A, Shojaei A, Zhang S. Safety and tolerability of lifitegrast ophthalmic solution 5.0%: pooled analysis of five randomized controlled trials in dry eye disease. Eur J Ophthalmol. 2019;29:394–401.
- Holland EJ, Darvish M, Nichols KK, Jones L, Karpecki PM. Efficacy of topical ophthalmic drugs in the treatment of dry eye disease: a systematic literature review. Ocul Surf. 2019;17:412–23.
- Lallemand F, Daull P, Benita S, Buggage R, Garrigue JS. Successfully improving ocular drug delivery using the cationic nanoemulsion novasorb. J Drug Deliv. 2012;2012:604204.
- Daull P, Lallemand F, Garrigue JS. Benefits of cetalkonium chloride cationic oil-in-water nanoemulsions for topical ophthalmic drug delivery. JPP. 2014;66:531–41.
- Sall KN, Cohen SM, Christensen MT, Stein JM. An evaluation of the efficacy of a cyclosporine-based dry eye therapy when used with marketed artificial tears as supportive therapy in dry eye. Eye Contact Lens. 2006;32:21–26.
- Okanobo A, Chauhan SK, Dastjerdi MH, Kodati S, Dana R. Efficacy of topical blockade of interleukin-1 in experimental dry eye disease. Am J Ophthalmol. 2012;154:63–71.
- Daull P, Feraille L, Barabino S, Cimbolini N, Antonelli S, Mauro V, Garrigue JS. Efficacy of a new topical cationic emulsion of cyclosporine a on dry eye clinical signs in an experimental mouse model of dry eye. Exp Eye Res. 2016;153:159–64.
- Daull P, Barabino S, Feraille L, Kessal K, Docquier M, Parsadaniantz SM, Baudouin C, Garrigue JS. Modulation of inflammation-related genes in the cornea of a mouse model of dry eye upon treatment with cyclosporine eye drops. Curr Eye Res. 2019;44:476–85.
- Burade V, Zalawadia R, Patel A, Ogundele A. Preclinical efficacy comparison of cyclosporine ophthalmic solution 0.09% vs cyclosporine ophthalmic emulsion 0.05% vs ciclosporin ophthalmic emulsion 0.1% in a nod mouse model of dry eye disease. Clin Ophthalmology. 2020;14:2747–55.
- Daull P, Guenin S, Hamon de Almeida V, JS G. Anti-inflammatory activity of ckc-containing cationic emulsion eye drop vehicles. Mol Vis. 2018;24:459–70.
- Pottel J, Armstrong D, Zou L, Fekete A, Huang XP, Torosyan H, Bednarczyk D, Whitebread S, Bhhatarai B, Liang G, et al. The activities of drug inactive ingredients on biological targets. Science. 2020;369(6502):403–13.
- Said T, Dutot M, Christon R, Beaudeux JL, Martin C, Warnet JM, Rat P. Benefits and side effects of different vegetable oil vectors on apoptosis, oxidative stress, and p2x7 cell death receptor activation. Invest Ophthalmol Vis Sci. 2007;48:5000–06.
- Dursun D, Wang M, Monroy D, Li DQ, Lokeshwar BL, Stern ME, Pflugfelder SC. A mouse model of keratoconjunctivitis sicca. Invest Ophthalmol Vis Sci. 2002;43:632–38.
- Barabino S, Shen L, Chen L, Rashid S, Rolando M, Dana MR. The controlled-environment chamber: a new mouse model of dry eye. Invest Ophthalmol Vis Sci. 2005;46:2766–71.
- Žiniauskaitė A, Ragauskas S, Hakkarainen JJ, Rich CC, Baumgartner R, Kalesnykas G, Albers DS, Kaja S. Efficacy of trabodenoson in a mouse keratoconjunctivitis sicca (kcs) model for dry-eye syndrome. Invest Ophthalmol Vis Sci. 2018;59:3088–93.
- Žiniauskaitė A, Ragauskas S, Ghosh AK, Thapa R, Roessler AE, Koulen P, Kalesnykas G, Hakkarainen JJ, Manganese(iii KS. tetrakis(1-methyl-4-pyridyl) porphyrin, a superoxide dismutase mimetic, reduces disease severity in in vitro and in vivo models for dry-eye disease. Ocul Surf. 2019;17:257–64.
- Barabino S, Antonelli S, Cimbolini N, Mauro V, Bouzin M. The effect of preservatives and antiglaucoma treatments on the ocular surface of mice with dry eye. Invest Ophthalmol Vis Sci. 2014;55:6499–504.
- Lemp MA. Report of the national eye institute/industry workshop on clinical trials in dry eyes. Clao J. 1995;21:221–32.
- French decree n 2013-118, dated february 1, 2013, publishing the European directive 2010/63/ue. J. Offic. Rep. Fr. 2013; text 24 out of 130. 2013.
- Geiss GK, Bumgarner RE, Birditt B, Dahl T, Dowidar N, Dunaway DL, Fell HP, Ferree S, George RD, Grogan T, et al. Direct multiplexed measurement of gene expression with color-coded probe pairs. Nat Biotechnol. 2008;26(3):317–25.
- Li Z, Gong Y, Chen S, Li S, Zhang Y, Zhong H, Wang Z, Chen Y, Deng Q, Jiang Y, et al. Comparative portrayal of ocular surface microbe with and without dry eye. J Microbiol. 2019;57(11):1025–32.
- Andersson J, Vogt JK, Dalgaard MD, Pedersen O, Holmgaard K, Heegaard S. Ocular surface microbiota in patients with aqueous tear-deficient dry eye. Ocul Surf. 2020;S1542-0124(20):30144–0.
- Zilliox MJ, Gange WS, Kuffel G, Mores CR, Joyce C, de Bustros P, Bouchard CS. Assessing the ocular surface microbiome in severe ocular surface diseases. Ocul Surf. 2020;18:706–12.
- Baudouin C. The pathology of dry eye. Surv Ophthalmol. 2001;45:S211–220.
- Bron A. The definition and classification of dry eye disease. In: Dry eye: a practical approach. Berlin, Heidelberg: Springer-Verlag; 2015. p. 1–19.
- Bron AJ, de Paiva CS, Chauhan SK, Bonini S, Gabison EE, Jain S, Knop E, Markoulli M, Ogawa Y, Perez V, et al. Tfos dews ii pathophysiology report. Ocul Surf. 2017;15(3):438–510.
- Wei Y, Asbell PA. The core mechanism of dry eye disease is inflammation. Eye Contact Lens. 2014;40:248–56.
- Willis KA, Postnikoff CK, Freeman A, Rezonzew G, Nichols K, Gaggar A, Lal CV. The closed eye harbours a unique microbiome in dry eye disease. Sci Rep. 2020;10:12035.
- de Paiva CS, Pflugfelder SC, Ng SM, Akpek EK. Topical cyclosporine a therapy for dry eye syndrome. Cochrane Database Syst Rev. 2019;9:CD010051.
- Donnenfeld E, Pflugfelder SC. Topical ophthalmic cyclosporine: pharmacology and clinical uses. Surv Ophthalmol. 2009;54:321–38.
- Hwang SB, Park JH, Kang SS, Kang DH, Lee JH, Oh SJ, Lee JY, Kim JY, Tchah H. Protective effects of cyclosporine a emulsion versus cyclosporine a cationic emulsion against desiccation stress in human corneal epithelial cells. Cornea. 2020;39:508–13.
- Olafsson J, ØA U, Chen X, Olafsson S, Raeder S, Dartt DA, Tashbayev B, Utheim TP. Changes in signs and symptoms of dry eye disease after using 0.1% or 0.05% emulsions of cyclosporine a eye drops. Invest Ophthalmol Vis Sci. 2018;59:912–912.
- Baudouin C, de la Maza MS, Amrane M, Garrigue JS, Ismail D, Figueiredo FC, Leonardi A. One-year efficacy and safety of 0.1% cyclosporine a cationic emulsion in the treatment of severe dry eye disease. Eur J Ophthalmol. 2017;27:678–85.
- Daull P, Lallemand F, Philips B, Lambert G, Buggage R, Garrigue JS. Distribution of cyclosporine a in ocular tissues after topical administration of cyclosporine a cationic emulsions to pigmented rabbits. Cornea. 2013;32:345–54.
- Daull P, Feraille L, Elena PP, Garrigue JS. Comparison of the anti-inflammatory effects of artificial tears in a rat model of corneal scraping. J Ocul Pharmacol Ther. 2016;32:109–18.