ABSTRACT
Purpose
Autophagy plays a crucial role in intracellular quality control of crystalline lens and AMPK has regulatory effect on autophagy. However, whether AMPK regulated autophagy is involved in diabetic cataract (DC) progression remains unknown. This study aims to investigate the AMPK-FOXO3 and AMPK-TFEB induced autophagy activity in DC patients.
Materials and Methods
First, anterior capsule specimens from DC and age-related cataract (ARC) patients were obtained to compare the expression difference of autophagy-related genes. The phosphorylation levels of AMPK, AKT, and mTOR and the expression of FOXO3 and TFEB were measured. Then, human lens epithelial cells (LECs, SRA 01/04) were cultured with 30 mM or 5.5 mM glucose, and AMPK activator (AICAR) and inhibitor (Compound C) were applied to further investigate the regulatory role of AMPK on autophagy.
Results
Compared with ARC patients, the expression of autophagy-related genes ATG5, FYCO1, ATG8, ATG12, Beclin1, and ULK1 in anterior capsules LECs of DC patients were significantly down-regulated. Meanwhile, AMPK and AMPK-dependent transcription factors, FOXO3 and TFEB were also inhibited. Similar results were found in high glucose (HG) treated SRA 01/04 model. Notably, this down-regulation of autophagy activity was rescued by AICAR in vitro, which was manifested by inhibition of AKT and mTOR phosphorylation and up-regulation of FOXO3, TFEB, Beclin1 and LC3B-II expression.
Conclusions
Down-regulation of AMPK-FOXO3 and AMPK-TFEB induced autophagy activity was found in both LECs of anterior capsule from DC patients and SRA 01/04 cells under HG condition, which may be the underlying mechanism of DC formation. Thus, targeting AMPK-induced autophagy may be a potential therapeutic approach for diabetic cataract.
Introduction
According to statistics from the International Diabetes Federation, worldwide diabetic patients have increased to 463 million in 2019, and were predicted to reach 700 million by 2045.Citation1 Diabetic cataract (DC), a common complication of diabetes mellitus (DM), is the leading cause of visual impairment and blindness in diabetic patients.Citation2,Citation3 It has been reported that the diabetic patients have five-fold higher risk of developing cataract than nondiabetic patients, and it usually occurs much earlier in diabetic patients.Citation4,Citation5 Most of the DC patients are middle-aged and become disabled due to the blindness, resulting in significant economic productivity loss and reduced quality of life. As far, the underlying mechanism of DC is still unclear and needs further investigation, which may help to fundamentally reduce the incidence of DC.
Autophagy is a cellular process which involves the degradation of misfolded or aggregated proteins, removal of damaged organelles and elimination of intracellular pathogens through lysosome machinery.Citation6 Autophagy also plays a significant role in intracellular quality control of crystalline lens and suppression of cataract.Citation7 The single layer of lens epithelial cells (LECs) experiences removal of the cell nuclei and organelles to form organelle-free zone (OFZ) in the centre of the lens, which is indispensable for lens transparency. Autophagy and mitophagy are considered to participate in this organelle degradation in crystalline lens.Citation8 More than 40 autophagy-related genes (ATGs) have been detected in human lens epithelium and fibers.Citation9 Previous studies have demonstrated that mutation of ATGs, FYCO1Citation10 and Atg5,Citation11 could lead to congenital cataract and age-related cataract (ARC) respectively, which indicates that autophagy plays a critical role in maintaining lens transparency and homeostasis. However, whether autophagy is involved in DC progression is still undetermined.
5ʹ-AMP-activated protein kinase (AMPK), a serine/threonine kinase, is an energy sensor. Under starvation conditions, the decrease of ATP levels and increase of AMP levels result in a reduction of the ATP/AMP ratio, which leads to AMPK activation and subsequently induces autophagy by directly phosphorylating ULK1/2 or inhibiting mTOR.Citation12,Citation13 AMPK suppression was found in myocardium, glomerulus, and renal tubules of diabetes animal models, suggesting that malfunctioning of AMPK signaling is associated with DM.Citation14–16 Additionally, AMPK regulated autophagy has been found in yeast and mammalian cells.Citation17,Citation18 FOXO3Citation19 and TFEB,Citation20 the vital AMPK-dependent transcription factors, can be activated by AMPK and then promote the transcription and translation of autophagy-related proteins involved in the formation of autophagosomes and autophagolysosomes.Citation20,Citation21 Previous study demonstrated that transcription of foxo3 decreased in zebrafish with high-fat diet induced type 2 diabetesCitation22 and decreased TFEB expression was also found in diabetic nephropathy.Citation23 Therefore, we presumed that the down-regulation of AMPK-FOXO3 and AMPK-TFEB induced autophagy in high glucose (HG) condition may involve in the mechanism of DC, which may provide a potential target for DC prevention and treatment.
To our knowledge, there are no previous studies on whether autophagy regulated by AMPK-FOXO3 and AMPK-TFEB is involved in the pathogenesis of DC. In the present study, we aimed to investigate AMPK-induced autophagy activity in the progression of DC. We found that autophagy activity and the expression of AMPK-dependent transcription factors, FOXO3 and TFEB, were down-regulated in the anterior capsule specimens of DC patients compared with ARC patients. Similar results were also observed in SRA 01/04. Therefore, AMPK-FOXO3 and AMPK-TFEB regulated autophagy may be a new perspective to partly elucidate the mechanisms of DC and a potential therapeutic target to prevent DC progression.
Materials and methods
Human anterior capsule samples collection
Anterior capsule specimens with LECs from 60 DC patients (28 men; mean age: 64.15 ± 5.32) and 60 age and sex-matched ARC patients (23 men, mean age: 64.47 ± 4.21) were obtained during cataract surgery. The lens nucleus grades were from 2 to 4 in all patients based on the Emery-Little classification.Citation24 The mean age, the gender ratio and the lens nucleus grade of the DC patients were comparable to the ARC group (P = .718, P = .356, P = .971). Each capsule was about 5 mm in diameter and contains the central area. Written informed consent forms were obtained from the patients before surgery, and the tenets of the Declaration of Helsinki were followed throughout the study. The research protocol was approved by the Institutional Review Board/Ethics Committee of the Sun Yat-sen University.
Cell culture
Human lens epithelial cell line SRA 01/04 was cultured in Dulbecco’s modified Eagle’s medium (DMEM) containing 10% fetal bovine serum (FBS). Cells were grown in a humidified 37°C incubator with 5% CO2 and dissociated with 0.25% trypsin-0.02% ethylenediamine-tetraacetic acid (EDTA). Cells were seeded in six-well plates with the density of 1 × 105/well, supplemented with 5.5 mM low glucose (LG) as a control group or 30 mM high glucose (HG) as an experimental group and then incubated for 48 hours. This low and high glucose concentrations were applied in previous studies.Citation25,Citation26 For AMPK activator AICAR and inhibitor Compound C treatment, cells were treated with 1 mM AICAR (Selleckchem, Houston, TX, USA) and 10 μM Compound C (Selleckchem, Houston, TX, USA).
Western blot
For total protein extraction, cells were lysed in RIPA buffer with protease inhibitor cocktail. After mixing with 5× SDS sample buffer, protein samples were separated by 10% sodium dodecyl sulfate-polyacrylamide gel electrophoresis (SDS-PAGE) and transferred to polyvinyl difluoride (PVDF) membranes (Bio-Rad). The membrane was blocked in 5% non-fat milk and incubated with primary antibody at 4°C overnight, and washed with PBST (0.1% Tween-20 in PBS). Then the membrane was incubated with horseradish peroxidase (HRP)-conjugated secondary antibodies for 1 hour at room temperature, and washed with PBST. Protein bands were detected with chemiluminescence detection reagents. β-actin and GAPDH were used as loading control. Quantitative analysis was done using Image J 1.41 (National Institutes of Health, Bethesda, MD, USA). The sources and dilutions of antibodies were: rabbit anti-AKT (1:500, Cell Signaling Technology), rabbit anti-p-AKT (1:500, Cell Signaling Technology), rabbit anti-mTOR (1:2000, ABclonal), rabbit anti-p-mTOR (1:2000, ABclonal), rabbit anti-FOXO3 (1:2000, ABclonal), rabbit anti-TFEB (1:1000, Abcam, ABclonal), rabbit anti-Beclin1 (1:500, Cell Signaling Technology), rabbit anti-P62 (1:1000, ABclonal), rabbit anti-AMPK (1:500, Cell Signaling Technology), rabbit anti-p-AMPK (1:500, Cell Signaling Technology), rabbit anti-LC3B (1:500, Cell Signaling Technology) and HRP-conjugated goat anti-rabbit IgG (1:10000, ABclonal).
RNA extraction and quantitative PCR
Total RNA was extracted from fresh anterior capsule tissue or SRA 01/04 using Trizol reagent (Invitrogen, Carlsbad, CA, USA) according to the manufacturer’s protocol. RNA concentration was measured spectrophotometrically at 260 nm. Two-microgram RNA was used for reverse transcription to cDNA using a reverse transcription kit (Takara, Siga, Japan). SYBR PrimeScript RT-PCR kit (Takara) was used to amplify target genes by the ABI Prism 7000 sequence detection system (Applied Biosystems, Foster City, CA, USA). Glyceraldehyde 3-phosphate dehydrogenase (GAPDH) was used as an internal control. Primer sequences are listed in . All the primers were synthesized by Beijing Genomics Institute (Beijing, China).
Table 1. Primers used for real-time quantitative PCR
Statistical analysis
Experiments presented in the figures are representative of at least three repetitions. All data were represented as means ±SD. GraphPad Prism 8 software (GraphPad software) was used for statistical analysis. One-way analysis of variance (ANOVA) followed by Bonferroni’s post hoc test was used to compare means among three or more groups. Independent samples t-test was used to compare means between two groups. All statistical tests were two tailed. Values of P < .05 were considered statistically significant.
Results
Autophagy activity is downregulated in anterior capsule LECs from DC patients
Autophagy plays a critical role in maintaining intracellular stability, such as eliminating damaged organelles and misfolded proteins. Especially, it has also been found to participate in lens OFZ formationCitation8 and respond to oxidative stress,Citation27 which is vital for lens transparency. ATGs including ATG5, FYCO1, ATG8, ATG12, Beclin1, and ULK1 can be used as autophagy activity markers since they assist autophagosome formation.Citation28–30 We found that compared with ARC patients, the mRNA levels of these genes in anterior capsule LECs from DC patients were significantly decreased (). Besides, we demonstrated that the mRNA and protein expression of autophagy markers, Beclin1 and LC3B-II were significantly reduced in anterior capsule LECs from DC patients, while the autophagy substrate P62 expression was increased (). All the results mentioned above indicate that the autophagy level in anterior capsule LECs from DC patients is down-regulated in comparison with ARC.
Figure 1. The autophagy level is down-regulated in anterior capsule LECs from DC patients. (A) Total RNA was extracted from the anterior capsules of DC and ARC patients (control). The mRNA levels of ATG8, Beclin1, ULK1, FYCO1, ATG5 and ATG12 were determined using real-time PCR and normalized to GAPDH. *P < .05, **P < .01, ***P < .001, n = 5. Fold change relative to the level of the control groups is displayed. (B) Proteins were extracted from the anterior capsules of DC and ARC patients (control) and then probed for Beclin1, LC3B and P62. GAPDH was used as a loading control. (C) Quantification of the Beclin1, LC3B-II and P62 expression levels in B. Fold change relative to the level of control groups is displayed. *P < .05, **P < .01, n = 3
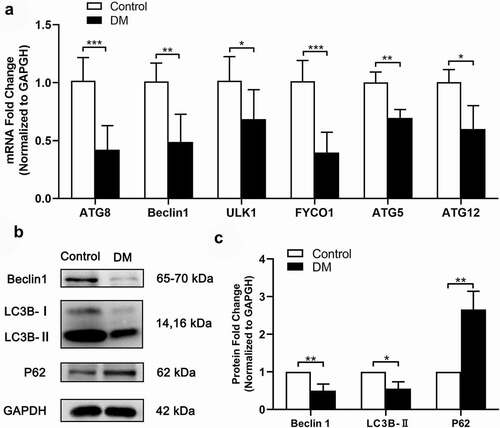
Down-regulation of autophagy is caused by AMPK inhibition in LECs under HG condition
Previous studies have reported that suppressed AMPK activity can inhibit autophagy under HG condition.Citation17,Citation31–33 However, the change of AMPK activity and its impact on autophagy in anterior capsule LECs from DC patients have not been reported. Our study first demonstrates that p-AMPK level is decreased in anterior capsule LECs from DC patients compared with ARC patients ().
Figure 2. AMPK activity is decreased in anterior capsule LECs from DC patients and suppression of autophagy and AMPK activity is found in SRA 01/04 under HG condition. (A) Proteins were extracted from the anterior capsules of DC and ARC patients (control) and then probed for total AMPK and p-AMPK. GAPDH was used as a loading control. (B) Quantification of p-AMPK/AMPK expression levels in A. Fold change relative to the level of control groups is displayed. ****P < .0001, n = 3. (C) SRA 01/04 were supplemented with 30 mM and 5.5 mM glucose and incubated for 48 hours. For experimental group, cells were treated with 1 mM AICAR and 10 mM Compound C for 48 hours respectively at the same time. Proteins were extracted and then probed for AMPK, p-AMPK, Beclin1 and LC3B. Without AICAR or Compound C treated LG (5.5 mM) cultured LECs were used as control groups. β-actin was used as a loading control. (D) Quantification of p-AMPK/AMPK, Beclin1 and LC3B-II expression levels in C. Fold change relative to the level of control groups is displayed. *P < .05, **P < .01, ***P < .001, ****P < .0001, n = 3. (E) The mRNA levels of Beclin1 and ATG8 were determined using real-time PCR and normalized to GAPDH. Fold change relative to the level of the control groups is displayed. *P < .05, **P < .01, ***P < .001, NS: not significant, n = 3
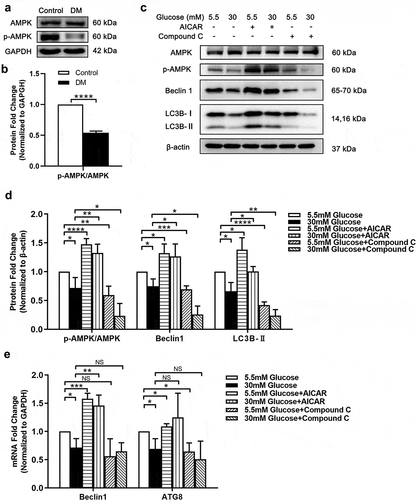
To further investigate the relationship between autophagy and AMPK activity in vitro, we established LG (5.5 mM) and HG (30 mM) model in SRA 01/04. The activity of AMPK in SRA 01/04 under HG condition was similar to the results in vivo (.C). Then, AMPK activator AICAR and inhibitor Compound C were implemented in HG and LG models. After treated with AICAR, AMPK activity was strongly induced, as well as the up-regulation of Beclin1, ATG8, and LC3B-II (). On the contrary, Compound C attenuated the mRNA level of ATG8 and the protein levels of Beclin1 and LC3B-II. The blockage of AMPK also caused restraint of autophagy in LG groups (). Thus, we can conclude that AICAR rescued the reduction of AMPK-induced autophagy in HG groups, suggesting that suppression of AMPK causes autophagy inhibition in LECs under HG condition.
Decreased AMPK activity inhibits autophagy through up-regulating the phosphorylation of AKT and mTOR in LECs under HG condition
The mammalian target of rapamycin (mTOR) functioned as an autophagy inhibitorCitation34 as well as a significant cell-growth regulator. mTOR can be inhibited by AMPK and activated by AKT,Citation34 while AMPK and AKT can phosphorylate and inhibit each other.Citation35 Thus, we measured the phosphorylation levels of AKT and mTOR in anterior capsule LECs from DC patients and SRA 01/04 to investigate the activity of AMPK pathway under HG condition. Consistent with our presumption, the results showed that the phosphorylation levels of AKT and mTOR were enhanced both in anterior capsule LECs from DC patients () and SRA 01/04 HG models (). Moreover, we observed that p-AKT and p-mTOR levels were significantly inhibited by AICAR which could subsequently rescue autophagy activity in SRA 01/04 under HG condition (). These results demonstrated that p-AMPK promotes autophagy through inhibiting the phosphorylation of AKT and mTOR in LECs.
Figure 3. mTOR and AKT were up-regulated in both anterior capsule LECs from DC patients and SRA 01/04 under HG condition. (A) Proteins were extracted from the anterior capsules of DC and ARC patients (control) and then probed for total AKT and p-AKT, total mTOR and p-mTOR. GAPDH was used as a loading control. (B) Quantification of p-AKT/AKT and p-mTOR/mTOR expression levels in A. Fold change relative to the level of control groups is displayed. **P < .01, ***P < .001, n = 3. (C) SRA 01/04 were supplemented with 30 mM and 5.5 mM glucose and incubated for 48 hours. For experimental group, cells were treated with 1 mM AICAR and 10 mM Compound C for 48 hours respectively at the same time. Proteins were extracted and then probed for p-AKT/AKT and p-mTOR/mTOR. Without AICAR or Compound C treated LG (5.5 mM) cultured SRA 01/04 were used as control groups. β-actin was used as a loading control. (D) Quantification of p-AKT/AKT and p-mTOR/mTOR expression levels in C. Fold change relative to the level of control groups is displayed. *P < .05, **P < .01, ***P < .001, ****P < .0001, n = 3
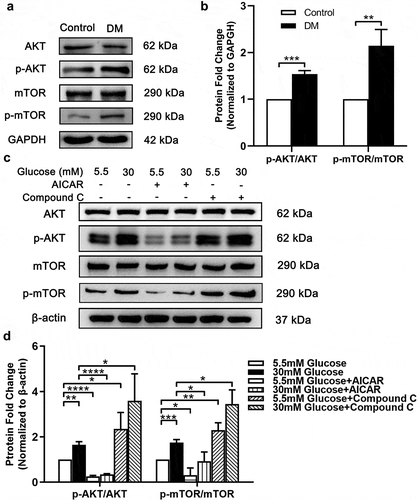
Down-regulated AMPK inhibits autophagy activity through inhibiting expression of FOXO3 and TFEB in LECs under HG condition
It has been reported that activation of the AMPK-dependent transcription factors FOXO3 and TFEB promotes autophagy through enhancing the transcription of ATGs.Citation19,Citation20 To further explore how down-regulated AMPK inhibits autophagy in DC, we examined the mRNA and protein levels of FOXO3 and TFEB in anterior capsule LECs from DC patients and SRA 01/04 HG models treated with AICAR and Compound C. The protein expression of two transcription factors exhibited suppression both in vivo and in vitro (), and the mRNA level of FOXO3 was also reduced (). Furthermore, this inhibition was promoted by AICAR and inhibited by Compound C in vitro (). To conclude, down-regulation of AMPK phosphorylation in DC may inhibit autophagy via reducing the expression of FOXO3 and TFEB.
Figure 4. Down-regulated AMPK inhibits autophagy activity through inhibiting expression of FOXO3 and TFEB in LECs. (A) Proteins were extracted from the anterior capsules of DC and ARC patients (control) and then probed for FOXO3 and TFEB. GAPDH was used as a loading control. (B) Quantification of FOXO3 and TFEB expression levels in A. Fold change relative to the level of control groups is displayed. **P < .01, ***P < .001, n = 3. (C) Total RNA was extracted from the anterior capsules of DC and ARC patients (control). The mRNA levels of FOXO3 and TFEB were determined using real-time PCR and normalized to GAPDH. Fold change relative to the level of the control groups is displayed. ***P < .001, NS: not significant, n = 3. (D) SRA 01/04 were supplemented with 30 mM and 5.5 mM glucose and incubated for 48 hours. For experimental group, cells were treated with 1 mM AICAR and 10 mM Compound C for 48 hours respectively at the same time. Proteins were extracted and then probed for FOXO3 and TFEB. Without AICAR or Compound C treated LG (5.5 mM) cultured SRA 01/04 were used as control groups. β-actin was used as a loading control. (E) Quantification of FOXO3 and TFEB expression levels in D. Fold change relative to the level of control groups is displayed. *P < .05, **P < .01, ***P < .001, ****P < .0001, n = 3. (F) The mRNA levels of FOXO3 and TFEB were determined using real-time PCR and normalized to GAPDH. Fold change relative to the level of the control groups is displayed. *P < .05, **P < .01, ***P < .001, ****P < .0001, NS: not significant, n = 3
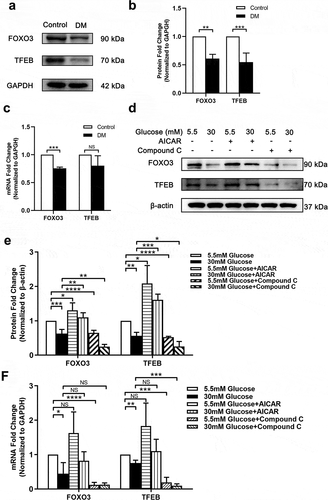
Discussion
In recent years, DC has drawn more attention due to the increasing number of diabetic patients and the unclear mechanism of this blind-causing complication. Previous studies have shown that autophagy may contribute to the development of cataract.Citation7 We presume that autophagy may also be involved in DC. Thus, targeting a key signal factor in autophagy may be an effective approach for DC prevention. In this study, we demonstrated that autophagy level in anterior capsule LECs from DC patients was down-regulated. In addition, the protein expression of p-AMPK and autophagy transcription factors, FOXO3 and TFEB were decreased in both anterior capsule LECs from DC patients and SRA 01/04 under HG conditions. Moreover, the suppression of autophagy can be restored by AICAR in vitro. These results indicate that the down-regulation of AMPK-FOXO3 and AMPK-TFEB induced autophagy may be an underlying mechanism of DC formation ().
Figure 5. A working mode for AMPK-FOXO3 and AMPK-TFEB in regulation of autophagy signaling pathway. AMPK can promote the transcription of autophagy related genes by activating FOXO3 and TFEB, the vital AMPK-dependent transcription factors, and then up-regulate autophagy level. The increase of the ATP/AMP ratio caused by high glucose condition can inhibit AMPK, thus inhibits autophagy through the blockade of AMPK-FOXO3 and AMPK-TFEB pathway
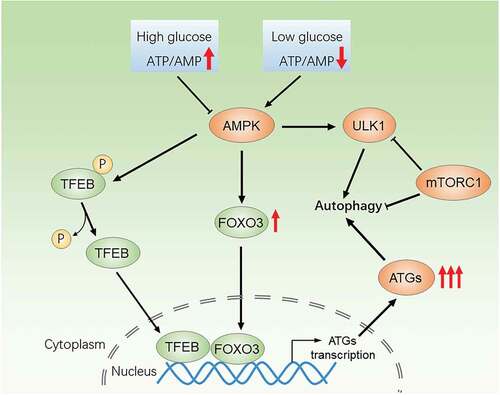
Previous studies have shown that the dysfunction of autophagy under HG conditions is involved in the pathogenesis of diabetic complications such as diabetic nephropathy, diabetic neuropathy, and diabetic retinopathy.Citation36–38 As far as we know, there are few published studies regarding the change of autophagy level and its role in the mechanism of DC progression. Here, we demonstrated that the mRNA levels of ATG5, FYCO1, ATG8, ATG12, Beclin1, and ULK1 were decreased in anterior capsule LECs from DC patients, preliminarily indicating the down-regulation of autophagy activity. During autophagy process, adaptor P62 as substrates degraded along with cytoplasmic cargoes.Citation39,Citation40 LC3 is cleaved to form cytosolic LC3-I, which is subsequently conjugated with phosphatidylethanolamine (PE) to form LC3-II.Citation39 LC3B is the most important subtype of LC3. Therefore, P62 and LC3B-II levels are generally used as markers for assessing autophagic activity. Our study showed that P62 was elevated in anterior capsule LECs from DC patients, while LC3B-II was decreased in both anterior capsule LECs from DC patients and SRA 01/04 treated with HG, indicating that autophagy is blocked under HG. However, limited studies of autophagy in DC have shown paradoxical results. Some researchers found increased autophagy level in LECs under HG environment, as evidenced by up-regulated LC3B-II proteins and the generation of autophagosome, which is opposite to our study.Citation25,Citation41 While, there are other studies consistent with our findings that autophagy activity was inhibited in HG-treated LECs.Citation42,Citation43 A possible explanation for this discrepancy may be the dynamic changes of autophagy in LEC, which augmented during early HG treatment, but blocked after continuous HG stimulation.Citation43 Since the progression of DC is a long-term process, extending the treatment time of HG in LEC may better simulate the HG environment of LEC in vivo.
AMPK plays a crucial role in physiological activities such as glucose, lipid and amino acid metabolism, and endocrine regulation.Citation44 The suppression of AMPK activity was found in diabetes animal models, for example, AMPK activity and autophagy in myocardium were reported to be down-regulated in transgenic diabetic mice.Citation15,Citation16 Whether dysregulation of AMPK signal pathway and autophagy activity are associated with the formation of lens opacity in DC patients is worthy of investigation. In our present study, we proved the restraint of p-AMPK in LECs in vivo and in vitro. The core negative regulator of autophagy, mTOR, is one of the downstream effectors of AMPK and AKT.Citation45 Since the activity of autophagy can be attenuated by AKT activated mTOR,Citation13 p-AKT/AKT, and p-mTOR/mTOR are also used as indicators of autophagy.Citation46–48 In summary, we demonstrated that down-regulation of AMPK phosphorylation in LECs under HG condition inhibited autophagy through up-regulating the phosphorylation of AKT and mTOR.
Previous research has proved that FOXO3 can induce autophagy by promoting the transcription of a variety of autophagy-related genes including LC3B-II, Beclin1, Gabarapl1, Ulk2, and Atg4b.Citation49 TFEB, the master gene of lysosome, is widely expressed and plays an important role in the synthesis of lysosomes and autophagosomes.Citation50 Both FOXO3 and TFEB expression levels have significantly decreased in our study of DC patients, which means decreased p-AMPK inhibit autophagy through reducing these two transcription factors. To further verified the down-regulation of autophagy activity is caused by the changes of these AMPK downstream factors, the AMPK agonist AICAR and inhibitor compound C were applied in SRA 01/04. When treated with AICAR, p-mTOR/mTOR and p-AKT/AKT level reduced, whereas FOXO3 and TFEB expression was restored compared with HG control group. Reversed results were shown in the compound C group. Consequently, we concluded that AMPK activation can up-regulate the expression of FOXO3 and TFEB, thereby inducing autophagy. It has been reported that AMPK activator AICAR, A769662, and C24 had potential therapeutic significance for diabetes and its complications.Citation51–55 Thus, targeting AMPK as a therapeutic approach for DC may have a vast perspective.
Autophagy maintains the function of pancreatic beta cellsCitation56 and is necessary for the removal of damaged proteins in RPECitation57 under HG-induced oxidative stress, suggesting that autophagy plays an important role in maintaining normal glucose levels and antagonizing diabetes-induced oxidative injury. In this way, there is a great possibility to prevent DC by promoting autophagy. Then, the application of AMPK agonist AICAR can be a potential pharmaceutical target of DC through restoring autophagy level. Notably, the injury indicators were not tested in our study. Accumulation of reactive oxygen species (ROS) is considered as the key factor of cataract formationCitation58,Citation59 and could induce autophagy. Autophagy dysfunction, in turn, leads to the accumulation of ROS. Thus, investigation of apoptosis and oxidative injury in LECs may be included in further studies. In addition, diabetic animal models need to be established to verify whether AMPK agonists can rescue or delay the development of DC, which will be included in our future research to further prove our hypothesis.
Expression of ATGs, activity of AMPK and its downstream regulators TFEB and FOXO3 were found suppressed in anterior capsule samples from DC patients. Notably, this down-regulation of autophagy activity was rescued by AICAR in HG treated SRA 01/04 model. Taken together, we presumed that HG condition can inhibit AMPK, thus down-regulates the expression of ATGs through AMPK-FOXO3 and AMPK-TFEB pathway. Our work reveals a potential molecular mechanism of DC by investigating the role of AMPK in human LECs, and provides clinical evidence for the prevention of DC development by using AMPK as a potential therapeutic target.
Disclosure statement
No potential conflict of interest was reported by the author(s).
Data availability statement
The authors confirm that the data supporting the findings of this study are available within the article or its supplementary materials.
Correction Statement
This article has been republished with minor changes. These changes do not impact the academic content of the article.
Additional information
Funding
References
- IDF. Diabetes atlas. 7th ed. Vancouver: International diabetes federation; Accessed 19 November 2016. http://www.diabetesatlas.org/.
- Drinkwater JJ, Davis WA, Davis TME. A systematic review of risk factors for cataract in type 2 diabetes. Diabetes Metab Res Rev. 2019;35(1):e3073. doi:https://doi.org/10.1002/dmrr.3073.
- Xu X, He J, Xu X, Du X, Bai X, Zhang B, He X, Lu L, Zhu J, Zou H. Cataract was the principle cause of visual impairment and blindness in shanghai residents with type 2 diabetes. Acta Ophthalmol. 2016;94(3):e246–e247. doi:https://doi.org/10.1111/aos.12930.
- Obrosova IG, Chung SS, Kador PF. Diabetic cataracts: mechanisms and management. Diabetes Metab Res Rev. 2010;26(3):172–80. doi:https://doi.org/10.1002/dmrr.1075.
- Klein BE, Klein R, Moss SE. Prevalence of cataracts in a population-based study of persons with diabetes mellitus. Ophthalmology. 1985;92(9):1191–96. doi:https://doi.org/10.1016/S0161-6420(85)33877-0.
- Glick D, Barth S, Macleod KF. Autophagy: cellular and molecular mechanisms. J Pathol. 2010;221(1):3–12. doi:https://doi.org/10.1002/path.2697.
- Morishita H, Mizushima N. Autophagy in the lens. Exp Eye Res. 2016;144:22–28. doi:https://doi.org/10.1016/j.exer.2015.08.019.
- Costello MJ, Brennan LA, Basu S, Chauss D, Mohamed A, Gilliland KO, Johnsen S, Menko S, Kantorow M. Autophagy and mitophagy participate in ocular lens organelle degradation. Exp Eye Res. 2013;116:141–50. doi:https://doi.org/10.1016/j.exer.2013.08.017.
- Brennan LA, Kantorow WL, Chauss D, McGreal R, He S, Mattucci L, Wei J, Riazuddin SA, Cvekl A, Hejtmancik JF, et al. Spatial expression patterns of autophagy genes in the eye lens and induction of autophagy in lens cells. Mol Vis. 2012;18:1773–86.
- Chen J, Ma Z, Jiao X, Fariss R, Kantorow WL, Kantorow M, Pras E, Frydman M, Pras E, Riazuddin S, et al. Mutations in fyco1 cause autosomal-recessive congenital cataracts. Am J Hum Genet. 2011;88(6):827–38. doi:https://doi.org/10.1016/j.ajhg.2011.05.008.
- Morishita H, Eguchi S, Kimura H, Sasaki J, Sakamaki Y, Robinson ML, Sasaki T, Mizushima N. Deletion of autophagy-related 5 (atg5) and pik3c3 genes in the lens causes cataract independent of programmed organelle degradation. J Biol Chem. 2013;288(16):11436–47. doi:https://doi.org/10.1074/jbc.M112.437103.
- Kim J, Kundu M, Viollet B, Guan KL. Ampk and mtor regulate autophagy through direct phosphorylation of ulk1. Nat Cell Biol. 2011;13:132–41.
- Egan DF, Shackelford DB, Mihaylova MM, Gelino S, Kohnz RA, Mair W, Vasquez DS, Joshi A, Gwinn DM, Taylor R, et al. Phosphorylation of ulk1 (hatg1) by amp-activated protein kinase connects energy sensing to mitophagy. Science. 2011;331(6016):456–61.
- Hardie DG. Amp-activated protein kinase as a drug target. Annu Rev Pharmacol Toxicol. 2007;47:185–210. doi:https://doi.org/10.1146/annurev.pharmtox.47.120505.105304.
- Lee JW, Park S, Takahashi Y, Wang HG. The association of ampk with ulk1 regulates autophagy. PLoS One. 2010;5(11):e15394. doi:https://doi.org/10.1371/journal.pone.0015394.
- Xie Z, Lau K, Eby B, Lozano P, He C, Pennington B, Li H, Rathi S, Dong Y, Tian R, et al. Improvement of cardiac functions by chronic metformin treatment is associated with enhanced cardiac autophagy in diabetic ove26 mice. Diabetes. 2011;60(6):1770–78. doi:https://doi.org/10.2337/db10-0351.
- Efeyan A, Comb WC, Sabatini DM. Nutrient-sensing mechanisms and pathways. Nature. 2015;517(7534):302–10. doi:https://doi.org/10.1038/nature14190.
- Ha J, Guan KL, Kim J. Ampk and autophagy in glucose/glycogen metabolism. Mol Aspects Med. 2015;46:46–62. doi:https://doi.org/10.1016/j.mam.2015.08.002.
- Greer EL, Oskoui PR, Banko MR, Maniar JM, Gygi MP, Gygi SP, Brunet A. The energy sensor amp-activated protein kinase directly regulates the mammalian foxo3 transcription factor. J Biol Chem. 2007;282(41):30107–19. doi:https://doi.org/10.1074/jbc.M705325200.
- Rubinsztein DC, Codogno P, Levine B. Autophagy modulation as a potential therapeutic target for diverse diseases. Nat Rev Drug Discov. 2012;11(9):709–30. doi:https://doi.org/10.1038/nrd3802.
- Sanchez AM, Candau RB, Csibi A, Pagano AF, Raibon A, Bernardi H. The role of amp-activated protein kinase in the coordination of skeletal muscle turnover and energy homeostasis. Am J Physiol Cell Physiol. 2012;303(5):C475–485. doi:https://doi.org/10.1152/ajpcell.00125.2012.
- Meng XH, Chen B, Zhang JP. Intracellular insulin and impaired autophagy in a zebrafish model and a cell model of type 2 diabetes. Int J Biol Sci. 2017;13(8):985–95. doi:https://doi.org/10.7150/ijbs.19249.
- Zhang W, Li X, Wang S, Chen Y, Liu H. Regulation of tfeb activity and its potential as a therapeutic target against kidney diseases. Cell Death Discov. 2020;6:32. doi:https://doi.org/10.1038/s41420-020-0265-4.
- Emery JM, Little JH. Phacoemulsification and aspiration of cataracts: surgical techniques, complications, and results (Mosby). 1979.
- Zhang L, Cheng R, Huang Y. Mir-30a inhibits becn1-mediated autophagy in diabetic cataract. Oncotarget. 2017;8(44):77360–68. doi:https://doi.org/10.18632/oncotarget.20483.
- Li J, Ye W, Xu W, Chang T, Zhang L, Ma J, Pei R, He M, Zhou J. Activation of autophagy inhibits epithelial to mesenchymal transition process of human lens epithelial cells induced by high glucose conditions. Cell Signal. 2020;75:109768.
- Zhou W, Xu J, Wang C, Shi D, Yan Q. Mir-23b-3p regulates apoptosis and autophagy via suppressing sirt1 in lens epithelial cells. J Cell Biochem. 2019;120(12):19635–46. doi:https://doi.org/10.1002/jcb.29270.
- Codogno P, Mehrpour M, Proikas-Cezanne T. Canonical and non-canonical autophagy: variations on a common theme of self-eating? Nat Rev Mol Cell Biol. 2011;13(1):7–12. doi:https://doi.org/10.1038/nrm3249.
- Noda NN, Inagaki F. Mechanisms of autophagy. Annu Rev Biophys. 2015;44:101–22. doi:https://doi.org/10.1146/annurev-biophys-060414-034248.
- Frost LS, Mitchell CH, Boesze-Battaglia K. Autophagy in the eye: implications for ocular cell health. Exp Eye Res. 2014;124:56–66. doi:https://doi.org/10.1016/j.exer.2014.04.010.
- Egan DF, Shackelford DB, Mihaylova MM, Gelino S, Kohnz RA, Mair W, Vasquez DS, Joshi A, Gwinn DM, Taylor R, et al. Phosphorylation of ulk1 (hatg1) by amp-activated protein kinase connects energy sensing to mitophagy. Science. 2011;331(6016):456–61. doi:https://doi.org/10.1126/science.1196371.
- Kim J, Kundu M, Viollet B, Guan K-L. Ampk and mtor regulate autophagy through direct phosphorylation of ulk1. Nat Cell Biol. 2011;13(2):132–41. doi:https://doi.org/10.1038/ncb2152.
- Mammucari C, Milan G, Romanello V, Masiero E, Rudolf R, Del Piccolo P, Burden SJ, Di Lisi R, Sandri C, Zhao J, et al. Foxo3 controls autophagy in skeletal muscle in vivo. Cell Metab. 2007;6(6):458–71.
- Huang J, Manning BD. A complex interplay between akt, tsc2 and the two mtor complexes. Biochem Soc Trans. 2009;37(Pt 1):217–22. doi:https://doi.org/10.1042/BST0370217.
- Zhao Y, Hu X, Liu Y, Dong S, Wen Z, He W, Zhang S, Huang Q, Shi M. Ros signaling under metabolic stress: cross-talk between ampk and akt pathway. Mol Cancer. 2017;16(1):79. doi:https://doi.org/10.1186/s12943-017-0648-1.
- Towns R, Kabeya Y, Yoshimori T, Guo C, Shangguan Y, Hong S, Kaplan M, Klionsky DJ, Wiley JW. Sera from patients with type 2 diabetes and neuropathy induce autophagy and colocalization with mitochondria in sy5y cells. Autophagy. 2005;1(3):163–70. doi:https://doi.org/10.4161/auto.1.3.2068.
- Rosa MD, Distefano G, Gagliano C, Rusciano D, Malaguarnera L. Autophagy in diabetic retinopathy. Curr Neuropharmacol. 2016;14(8):810–25. doi:https://doi.org/10.2174/1570159X14666160321122900.
- Ding Y, Choi ME. Autophagy in diabetic nephropathy. J Endocrinol. 2015;224(1):R15–R30. doi:https://doi.org/10.1530/JOE-14-0437.
- Yang X, Pan X, Zhao X, Luo J, Xu M, Bai D, Hu Y, Liu X, Yu Q, Gao D. Autophagy and age-related eye diseases. Biomed Res Int. 2019;2019:5763658. doi:https://doi.org/10.1155/2019/5763658.
- Bjørkøy G, Lamark T, Brech A, Outzen H, Perander M, Overvatn A, Stenmark H. Johansen T. P62/sqstm1 forms protein aggregates degraded by autophagy and has a protective effect on huntingtin-induced cell death. J Cell Biol. 2005;171(4):603–14. doi:https://doi.org/10.1083/jcb.200507002.
- Li D, Liu GQ, Lu PR. High glucose: activating autophagy and affecting the biological behavior of human lens epithelial cells. Int J Ophthalmol. 2019;12(7):1061–66. doi:https://doi.org/10.18240/ijo.2019.07.02.
- Li J, Ye W, Xu W, Chang T, Zhang L, Ma J, Pei R, He M, Zhou J. Activation of autophagy inhibits epithelial to mesenchymal transition process of human lens epithelial cells induced by high glucose conditions. Cell Signal. 2020;75:109768. doi:https://doi.org/10.1016/j.cellsig.2020.109768.
- Liu X, Zhao X, Cheng R, Huang Y. Autophagy attenuates high glucose-induced oxidative injury to lens epithelial cells. Biosci Rep. 2020;40(4 BSR20193006).
- Steinberg GR, Kemp BE. Ampk in health and disease. Physiol Rev. 2009;89(3):1025–78. doi:https://doi.org/10.1152/physrev.00011.2008.
- Janku F, McConkey DJ, Hong DS, Kurzrock R. Autophagy as a target for anticancer therapy. Nat Rev Clin Oncol. 2011;8(9):528–39. doi:https://doi.org/10.1038/nrclinonc.2011.71.
- Wang B, Zhong Y, Li Q, Cui L, Huang G. Autophagy of macrophages is regulated by pi3k/akt/mtor signalling in the development of diabetic encephalopathy. Aging (Albany NY). 2018;10(10):2772–82. doi:https://doi.org/10.18632/aging.101586.
- Li X-Y, Wang -S-S, Han Z, Han F, Chang Y-P, Yang Y, Xue M, Sun B, Chen L-M. Triptolide restores autophagy to alleviate diabetic renal fibrosis through the mir-141-3p/pten/akt/mtor pathway. Mol Ther Nucleic Acids. 2017;9:48–56. doi:https://doi.org/10.1016/j.omtn.2017.08.011.
- Xu J, Deng Y, Wang Y, Sun X, Chen S, Fu G. Spag5-as1 inhibited autophagy and aggravated apoptosis of podocytes via spag5/akt/mtor pathway. Cell Prolif. 2020;53(2):e12738. doi:https://doi.org/10.1111/cpr.12738.
- Mammucari C, Milan G, Romanello V, Masiero E, Rudolf R, Del Piccolo P, Burden SJ, Di Lisi R, Sandri C, Zhao J, et al. Foxo3 controls autophagy in skeletal muscle in vivo. Cell Metab. 2007;6(6):458–71. doi:https://doi.org/10.1016/j.cmet.2007.11.001.
- Zhao E, Czaja MJ, Groszmann RJ, Iwakiri Y, Taddei TH. Transcription factor eb: a central regulator of both the autophagosome and lysosome. Hepatology. 2012;55(5):1632–34. doi:https://doi.org/10.1002/hep.25619.
- Madhavi YV, Gaikwad N, Yerra VG, Kalvala AK, Nanduri S, Kumar A. Targeting ampk in diabetes and diabetic complications: energy homeostasis, autophagy and mitochondrial health. Curr Med Chem. 2019;26(27):5207–29. doi:https://doi.org/10.2174/0929867325666180406120051.
- Winder WW. Amp-activated protein kinase: possible target for treatment of type 2 diabetes. Diabetes Technol Ther. 2000;2(3):441–48. doi:https://doi.org/10.1089/15209150050194305.
- Guigas B, Sakamoto K, Taleux N, Reyna SM, Musi N, Viollet B, Hue L. Beyond aica riboside: in search of new specific amp-activated protein kinase activators. IUBMB Life. 2009;61(1):18–26. doi:https://doi.org/10.1002/iub.135.
- Li -Y-Y, Yu L-F, Zhang L-N, Qiu B-Y, Su M-B, Wu F, Chen D-K, Pang T, Gu M, Zhang W, et al. Novel small-molecule ampk activator orally exerts beneficial effects on diabetic db/db mice. Toxicol Appl Pharmacol. 2013;273(2):325–34. doi:https://doi.org/10.1016/j.taap.2013.09.006.
- Ducommun S, Ford RJ, Bultot L, Deak M, Bertrand L, Kemp BE, Steinberg GR, Sakamoto K. Enhanced activation of cellular ampk by dual-small molecule treatment: aicar and a769662. Am J Physiol Endocrinol Metab. 2014;306(6):E688–E696. doi:https://doi.org/10.1152/ajpendo.00672.2013.
- Jung HS, Chung KW, Won Kim J, Kim J, Komatsu M, Tanaka K, Nguyen YH, Kang TM, Yoon KH, Kim JW, et al. Loss of autophagy diminishes pancreatic beta cell mass and function with resultant hyperglycemia. Cell Metab. 2008;8(4):318–24. doi:https://doi.org/10.1016/j.cmet.2008.08.013.
- Yao J, Tao ZF, Li CP, Li XM, Cao GF, Jiang Q, Yan B. Regulation of autophagy by high glucose in human retinal pigment epithelium. Cell Physiol Biochem. 2014;33(1):107–16. doi:https://doi.org/10.1159/000356654.
- Lee J, Giordano S, Zhang J. Autophagy, mitochondria and oxidative stress: cross-talk and redox signalling. Biochem J. 2012;441(2):523–40. doi:https://doi.org/10.1042/BJ20111451.
- Kawakami T, Gomez IG, Ren S, Hudkins K, Roach A, Alpers CE, Shankland SJ, D’Agati VD, Duffield JS. Deficient autophagy results in mitochondrial dysfunction and fsgs. J Am Soc Nephrol. 2015;26(5):1040–52. doi:https://doi.org/10.1681/ASN.2013111202.