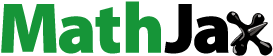
Abstract
Purpose
Higher order ocular aberrations (e.g. entopic scatter) arising from cornea and lens, decreases retinal image contrast by dispersing part of the image-forming optics over a broad retinal surface. Selective filtering of the light that is most susceptible to aberration (high-energy “blue” light) may reduce some of the behavioral effects. This was tested by comparing the performance of a blue-light filtering (BLF) vs a clear intraocular lens implant (IOL).
Methods
52 participants with IOL (BLF: AlconSN60AT; clear: AlconSA60AT; N = 98 test eyes; M = 67.33 7.48 years; 58.8% Female; 25.5% non-White) were recruited. Our outcome measure was based on the minimum resolvable distance between two points of light (two-point thresholds), formed using broadband xenon or isolated short-wave energy (425 nm). Iris color was measured by visual inspection and comparison against standard images.
Results
In the broadband condition, patients with BLF IOL had smaller two-point thresholds (M = 17.17 ± 5.71 mm; F[1,48] = 2.60; p = 0.045) than clear controls (M = 20.93 ± 10.22 mm). Similar improvements were found in the short-wave condition (MBLF=17.02 ± 5.30; Mclear=21.42 ± 10.99; p = 0.04). In the contralateral broadband comparison, eyes with the BLF had significantly smaller two-point thresholds (M = 18.10 ± 10.47 mm; t = −2.90, p < 0.001) than the clear IOL (M = 20.89 ± 10.61 mm). Similar effects were seen in the short-wave condition (MBLF=18.23 ± 9.88; Mclear = 21.06 ± 10.47; p = 0.001). Darker iris color was related to reduced scatter across IOL types, in both shortwave (F[2,48] = 4.62, p = 0.02) and broadband (F[2,48] = 5.27, p = 0.009) conditions.
Conclusions
Anterior screening, be it by a darker iris or a BLF IOL, is directly related to decreases in two-point light thresholds.
Introduction
Many challenges accompany replacing the natural crystalline lens with an intraocular lens implant (IOL). The first is the surgical incision which creates an optical imperfection within the cornea. Ophthalmologists have worked for years to reduce the size of corneal incisions in order to lessen deleterious visual effects that often result (such as induced astigmatismCitation1). The corneal incisions that accompany laser corrections (laser in situ keratomileusis or photorefractive keratectomy) face similar challenges, as do other corneal surgeries such as keratoplasty. One relatively common effect with any surgical intervention to the eye is an increase in intraocular light scatter.Citation2 Depending on the smoothness of the junction between an implant and the remaining lens capsule, for instance, more or less light scatter and other side effects (secondary pigment dispersion, posterior capsule opacification, etc.Citation3) can occur.
Intraocular light scatter is the primary underlying issue in many common visual complaintsCitation4 ranging from glare disability and discomfort to dysphotopsia. Light scatter (and the accompanying visual artifacts), in a relatively linear fashion, even exacerbates losses in normal visual parameters such as contrast sensitivity.Citation5 Increased light scatter tends to accompany both age and refractive stateCitation6 and the scatter, and the visual effects of the scatter, are often the motivation for having cataracts replaced.Citation7 Typically, the summative effect of cataract surgery is that intraocular scatter is improved.Citation8 This, however, is clearly not always the case,Citation9 nor is the improvement uniform. IOLs can be incorrectly placed, scratched during implantation, retain folding marks, or become decentered after surgery.Citation10,Citation11 The design of the IOL, for instance, matters significantly (e.g. glistening from hydrophobic material, diffractive optics, a high index of refraction, truncated edge, etc. are all design features that have been correlated with scatter issuesCitation12).
Since design clearly matters, one question is are there features that can be added to further reduce the visual effects of these types of higher order ocular aberrations. One strategy could be the one often employed by nature, strategic filtering.Citation13 The human macular (retinal) pigments, for example, do not reduce the amount of scattered light coming from the anterior optics of the eye.Citation14 They clearly, however, reduce the effects of light scatter at the macula (e.g. glare disabilityCitation15). Macular pigment filters short-wave (blue) light and this is likely not incidental. Filtering in the high-energy portion of the visible spectrum may be optimalCitation16 for reducing actinic exposure and improving visual function.Citation17
Filtering the cones within the macula, however, does not address scatter effects outside that small region. Hence, many species filter light in the anterior portion of their optics. For example, fish often have yellow corneas. Diurnal squirrels and prairie dogs have a yellow screening filter between their cornea and retina.Citation13 Humans may employ a similar strategy through age-related filtering of short-wave light by the cornea/crystalline lens and by accumulating melanin in the iris. Darker iridies (extending also behind the sclera as part of the retinal pigment epithelium) have been associated with decreases in intraocular scatter.Citation18 Tinted spectacle and contact lenses employ a similar strategy and have also been shown to reduce the effects of light scatter.Citation19,Citation20 Gray et al.,Citation21 for instance, showed that BLF IOLS improved driving performance by reducing some of the deleterious effects of light scatter.
In this study, we tested whether a blue-light filtering (BLF) IOL would reduce the behavioral effects of higher order intraocular aberrations (like light scatter) when compared to a matched clear IOL across individuals with different levels of iris pigmentation. Our behavioral outcome was based on measuring the distance between two discrete point sources of broadband xenon light. Light spread across the retina (essentially a wider point spread function) necessitates a greater separation before two discrete points of light are distinct (see ). Measuring this distance provides an assessment of the extent of higher order aberrations within the eye.Citation22
Materials and methods
To determine whether anterior screening by a BLF IOL affects two-point thresholds, a case (BLF)-control (clear) design was used. Comparisons were made between-subjects with different lens types and within-subjects who have contralateral implantation (BLF in one eye; clear control lens in the fellow eye). All study procedures and materials were approved by the University of Georgia Institutional Review Board prior to initiating the study. All participants gave both written and verbal informed consent prior to participation, and the tenets of the Declaration of Helsinki were adhered to at all times during the execution of the study.
Subjects
Demographics
Fifty-two participants (M = 67.33 ± 7.48 years; 58.8% Female; 25.5% non-White/non-Caucasian; see for full demographic information) were recruited from a local ophthalmology practice. Eligibility criteria included cataract extraction surgery with IOL implantation in one or both eyes with either the clear control lens (Alcon SA60AT) or the BLF IOL (Alcon SN60AT) in at least one eye (for light transmission curves see Ernest, 2004Citation23) Surgery must have been conducted on the test eye(s) a minimum of 9 weeks prior to enrollment (average time between implantation and research participation was 53.9 weeks, 3.7 days ± 42.0 weeks, 2.0 days). Medical records reviews were conducted by the collaborating ophthalmology practice to ensure that participants with ocular conditions (such as posterior capsular opacification) that would have confounded our measurements were excluded.
Table 1. Demographic and ocular health information for all participants.
Assignment into case and control groups
The referring eye care practice implanted, and consequently referred, more participants with the SN60AT BLF lens. Given the fact that the primary analysis for this study was a between-subjects case control analysis (cases with the BLF and controls with the clear IOL), participants were assigned to case and control groups upon enrollment as follows (see ).
Figure 2. Schematic depicting recruitment, enrollment, assignment, data collection and analysis activities.
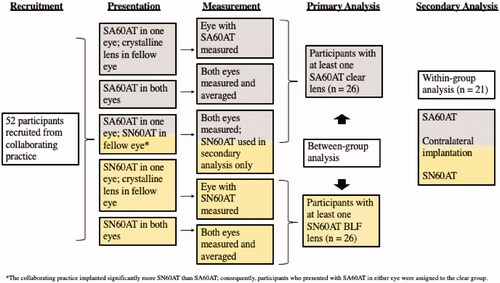
Clear controls (n = 26)
One subject presented with the clear IOL in one eye and their own crystalline lens in the fellow eye. Only the eye with the clear IOL was measured and included in the control group for the primary analysis. Five subjects presented with the clear IOL in both eyes, and both eyes were measured. The two eyes were then averaged into a single data point for each subject, and these five data points were included as controls in the primary analysis. Twenty-one subjects presented with contralateral implantation (BLF case in one eye; clear control in the fellow eye). Both eyes were measured in all 21 subjects. For 20 of the 21 subjects, the data from the clear control eye were included in the primary analysis, and the data from the BLF case eye were retained for the secondary analysis. To ensure equal sample sizes for the primary analysis, one of the contralateral subjects was randomly chosen for inclusion into the case BLF group for the primary analysis. In all 21 contralateral subjects, data from the fellow eye were retained for the secondary analysis.
BLF cases (n = 26)
In addition to the contralateral subject randomized to BLF group for the primary analysis, 7 participants presented with the BLF in one eye and their crystalline lens in the fellow eye. Only the eyes with the BLF IOL were measured and included in the primary analysis. Eighteen participants presented with the BLF in both eyes, and both eyes were measured. The two eyes were then averaged into a single data point for each subject, and these 18 data points were included as controls in the primary analysis.
After the primary analysis was complete, a secondary within-subjects case-control analysis was conducted on the 21 subjects who provided one clear control eye and one BLF case eye for analysis, where each subject served as their own control for comparison.
Apparatus and procedure
Upon arrival to the test site, uncorrected distance visual acuity (UDVA) was measured binocularly and monocularly in each test eye. UDVA of 20:40 or better in the test eye(s) was confirmed by a trained experimenter. Iris color was documented using a visual grading systemCitation24 based on judgements (matching a subject’s eye color to a series of 15 standardized iris images) by a single trained observer. The referents used by the grading system are a series of colored photographs of human irises sequenced according to their hue (CIE L*a*b color space) and measured lightness/darkness.
Details of the apparatus that we used for measuring two-point light thresholds were originally presented by Hammond et al.Citation22 The method is based on measuring the separation of two point sources of light. Individuals with a wider point-spread function require more separation before two small points of light will appear distinct. The Rayleigh criterion method of quantifying the spread of two small point light sources was originally described by Grimes et al.Citation25
Light for all of the measurements was produced by a 1000 W Xenon arc lamp. This white spectrum has been shown to be a good match for sunlight.Citation26 An additional short-wave condition was created using a 425 nm interference filter (18 nm half-bandpass) placed before the two small apertures creating the light points (the 50% transmission point of the BLF filter was 445 nm). An achromatic lens was used to partially collimate the light so that it provided homogeneous back illumination of an opaque light shield with a collapsible baffle (a center piece lowered as the apertures spread to make distinct light points that were physically disconnected). This light shield contained the two small (2 mm) apertures (∼13 min visual angle) that could be brought together into a stimulus that was perceived as a single point, or slowly moved apart into a stimulus that was perceived as two separate points. The brightness of the stimuli was 712 cd/m2 for the white light condition and 52 cd/m2 for the short-wave condition (measured at the plane of the eye using a Model 1153 LumiLens, United Detection Technology, Graseby Optronics, FL). Stabilization of the subject’s head was accomplished by an adjustable chin and forehead rest assembly, with an eye patch covering the non-test eye.
Light from the point source (i.e. light emerging from the 2 mm apertures in the light shield) was focused by a long (220 mm) focal length lens (17.8 cm from the plane of the eye) so that the subject’s eye was in partial Maxwellian view (meaning the eye was in the focusing beam but the plane of the eye was anterior to the final focal point). When the points were maximally close together, the stimulus appeared as a single, bright point of light. The two apertures were slowly moved apart from this “zero point,” and subjects indicated when the spread from each light point did not overlap (e.g. when they first perceived a small black space between the two points). The distance was quantified with a digital micrometer, centered on the apertures, that was part of the physical structure forming the apertures. A total of six trials were completed per test eye: three trials were completed using the broadband stimulus, and three trials were completed using the short-wave test condition. When both eyes were measured, test eye order was randomized.
Photometric calibrations were performed using an ILT 950 spectral radiometer (International Light Technologies, Peabdoy MA). Wedge and neutral density radiometric calibrations were performed using a Graseby Optronics United Detection Technology (UDT) instrument (Orlando, FL). The same UDT instrument was used before every experimental session to ensure that the total light output of the optical system remained constant and consistent throughout the study.
Results
Between-subjects analysis
IOL differences
One-way ANOVA was computed to compare the BLF against the clear control lens in both the broadband and short-wave conditions. In the broadband two-point threshold condition, the test eye with the BLF (M = 17.17 ± 5.71 mm) could perceive the split between the points with significantly less distance (F[1,49] = 2.60, p = 0.045) than the test eye with the clear control lens (M = 20.93 ± 10.22 mm). The same effect was seen in the short-wave condition: test eyes with the BLF (M = 17.02 ± 5.30 mm) could perceive two points with significantly less distance between them (F[1,49] = 3.28, p = 0.04) than test eyes with the clear control lens (M = 21.42 ± 10.99 mm).
Effects of Iris color
A one-way ANOVA was computed to determine whether iris color affected two-point thresholds, regardless of IOL type. In both the broadband (F[2,48] = 5.27, p = 0.009) and short-wave (F[2,48] = 4.17, p = 0.015) conditions, participants with dark irides were able to perceive two points with significantly less difference than light irides (pLSD = 0.20) and medium irides (pLSD = 0.003) (see ).
Table 2. Effects of iris color on two-point thresholds.
Modeling the effects of Iris color and IOL type on two-point thresholds
To determine how iris color and IOL type jointly impacted two-point thresholds, a linear regression was conducted with iris color and lens type as independent variables. In the broadband condition, the ANOVA (F[2,48] = 5.15, p = 0.009) for the model was significant, with iris color (ß = −0.357, p = 0.009) accounting for a greater proportion of variance than IOL type (ß = −0.263, p = 0.045). The same effect was seen in the shortwave condition (F[2,48] = 5.62, p = 0.006), with iris color (ß = −0.358, p = 0.009) accounting for a greater proportion of variance than IOL type (ß = −0.290, p = 0.031).
Contralateral analysis
Paired-samples t-tests were used to determine whether the BLF and clear control lens (n = 21) yielded different two-point thresholds within the same subject. In the broadband two-point threshold condition, the test eyes with the BLF (M = 18.10 ± 10.47 mm) perceived two points with significantly less distance between them (t = −2.98, p < 0.005) than the clear control test eyes (M = 20.89 ± 10.61 mm). The same difference was seen in the short-wave condition: the test eyes with the BLF (M = 18.23 ± 9.88 mm) perceived the split between the points with significantly less distance (t = −2.54, p < 0.01) than the test eyes with the clear control lens (M = 21.06 ± 10.47 mm).
Discussion
This study compared the effects of higher order ocular aberrations measured as the distance between two small point sources of light in subjects with BLF vs clear IOL, using narrow-band short-wave and broadband light conditions. For the between subject comparison, we compared 26 subjects with a BLF IOL (in the right or left eye) with 26 subjects with a clear IOL (in the right or left). Twenty-one out of the 52 subjects in the sample, however, also had a clear in one eye and a BLF in the other allowing us to also do a within-subject comparison (albeit with the limitation that one eye had already been analyzed in the between-subject comparison). It should also be noted that experimenter was not blinded to the type of IOL being tested. Notwithstanding these limitations, with both kind of comparisons, the differences were uniformly significant (ranging from about 14% within- and around 20% between-subjects) and favored BLF. This reduction in the deleterious effects of broadband light (emulating the spectrum of mid-day sunlight) was likely due to simple screening since we saw an even larger improvement with darker iris color (an iris effect on scatter is consistent with past studiesCitation18,Citation27) Implanting a clear IOL in one eye and a BLF IOL in the other has been shownCitation28 to have minimal effects on some visual traits like color perception (perceptual phenomena are often more centrally yoked). Our results suggest, however, that some lower level effects (like scatter) may show interocular differences when two different types of lenses are implanted in the same individual.
The selective absorption of short-wave light is likely a good choice for an IOL (matching the normal absorption characteristics of a young crystalline lens). Blue light filtering is particularly prevalent in natureCitation29 and numerous optical mechanisms have been advanced to account for their selective advantage and resultant ubiquity.Citation13,Citation29,Citation30 All of these ideas are predicated on the observation that short-wave light, like many common external elements affecting our biology (e.g. oxygen), is both positive (e.g. broadening color perception or influencing intrinsically photosensitive ganglion cells which are particularly sensitive to short-wave light) and negative, carrying a large potential for harm. Some of this harm is actinic but other deleterious effects have to do directly with visual function. For example, the interaction of short-wave light with other elements of the atmosphere can create a veiling (blue) haze that can significantly limit visual range.Citation17 Mechanisms for accumulating BLFs like macular pigment likely evolved as a means for improving normal vision in reproductively fecund individuals. Darker irises (like dermal melanin) likely evolved to cope with high light stress environments.Citation31 Rayleigh scatter causes a preponderance of blue light backgrounds in the environment that likely favor a BLF.Citation17 Intraocular scatter is likely not Rayleigh dependent,Citation32 at least in the normal healthy eye (although there may be some Rayleigh dependence in the heavily pigmented eyeCitation33) Even if not more prevalent (i.e. more short-wave scatter within the eye compared to longer wavelengths), our data show that filtering short-wave light improves some of the deleterious effects of broad-band light (or narrowband light maximally absorbed by the lens).
Acknowledgements
The authors would like to thank Dr. Lauren Hacker at the Georgia Center for Sight in Athens, GA for her assistance in recruitment, and to Colin Gardner and Dr. Sarah Saint for their assistance in data collection.
Disclosure statement
Author BRH has received consulting fees from Alcon Laboratories. Author LRH is the spouse of author BRH and, thus, has the same conflict as author BRH.
Additional information
Funding
References
- Sonmez S, Karaca C. The effect of tunnel length and position on postoperative corneal astigmatism: an optical coherence tomographic study. Eur J Ophthalmol. 2020;30(1):104–111.
- Van Bree MCJ, Van Verre HP, Devreese MT, Larminier F, Van Den Berg TJTP. Straylight values after refractive surgery: screening for ocular fitness in demanding professions. Ophthalmology. 2011;118(5):945–953.
- Mehta R, Aref AA. Intraocular lens implantation in the ciliary sulcus: challenges and risks. Clin Ophthalmol. 2019;13:2317–2323.
- Van Den Berg TJTP. Importance of pathological intraocular light scatter for visual disability. Doc Ophthalmol. 1986;61(3–4):327–333.
- Zhao J, Xiao F, Zhao H, Dai Y, Zhang Y. Effect of higher-order aberrations and intraocular scatter on contrast sensitivity measured with a single instrument. Biomed Opt Express. 2017;8(4):2138–2147.
- Rozema JJ, van den Berg TJTP, Tassignon MJ. Retinal straylight as a function of age and ocular biometry in healthy eyes. Invest Ophthalmol Vis Sci. 2010;51(5):2795–2799.
- van der Meulen IJE, Gjertsen J, Kruijt B, Witmer JP, Rulo A, Schlingemann RO, van den Berg TJTP. Straylight measurements as an indication for cataract surgery. J Cataract Refract Surg. 2012;38(5):840–848.
- Van Den Berg TJTP, Van Rijn L(R), Michael R, Heine C, Coeckelbergh T, Nischler C, Wilhelm H, Grabner G, Emesz M, Barraquer RI, et al. Straylight effects with aging and lens extraction. Am J Ophthalmol. 2007;144(3):358–363.
- Werner L, Stover JC, Schwiegerling J, Das KK. Effects of intraocular lens opacification on light scatter, stray light, and overall optical quality/performance. Invest Ophthalmol Vis Sci. 2016;57(7):3239–3247.
- Milazzo S, Turut P, Blin H. Alterations to the AcrySof intraocular lens during folding. J Cataract Refract Surg. 1996;22(Supplement 2):1351–1354.
- Durr GM, Ahmed IIK. Intraocular lens complications: decentration, uveitis-glaucoma-hyphema syndrome, opacification, and refractive surprises. Ophthalmology. 2020;22(10):377–381.
- Grzybowski A, Markeviciute A, Zemaitiene R. A narrative review of intraocular lens opacifications: update 2020. Ann Transl Med. 2020;8(22):1547–1547.
- Hammond BR. The visual effects of intraocular colored filters. Scientifica. 2012;2012:424965–424918.
- Putnam CM, Bland PJ, Bassi CJ. Influence of macular pigment optical density spatial distribution on intraocular scatter. J Optom. 2017;10(1):63–68.
- Hammond BR, Johnson BA, George ER. Oxidative photodegradation of ocular tissues: Beneficial effects of filtering and exogenous antioxidants. Exp Eye Res. 2014;129:135–150.
- van de Kraats J, van Norren D. Optical density of the aging human ocular media in the visible and the UV. J Opt Soc Am A Opt Image Sci Vis. 2007;24(7):1842–1857.
- Hammond BR, Sreenivasan V, Suryakumar R. The effects of blue light-filtering intraocular lenses on the protection and function of the visual system. Clin Ophthalmol. 2019;13:2427–2438.
- Nischler C, Michael R, Wintersteller C, Marvan P, van Rijn LJ, Coppens JE, van den Berg TJTP, Emesz M, Grabner G. Iris color and visual functions. Graefes Arch Clin Exp Ophthalmol. 2013;251(1):195–202.
- Renzi-Hammond LM, Hammond BR Jr. The effects of photochromic lenses on visual performance. Clin Exp Optom. 2016;99(6):568–574.
- Renzi-Hammond L, Buch JR, Cannon J, Hacker L, Toubouti Y, Hammond BR. A contra-lateral comparison of the visual effects of a photochromic vs. non-photochromic contact lens. Contact Lens Anterior Eye. 2020;43(3):250–255.
- Gray R, Perkins SA, Suryakumar R, Neuman B, Maxwell WA. Reduced effect of glare disability on driving performance in patients with blue light-filtering intraocular lenses. J Cataract Refract Surg. 2011;37(1):38–44.
- Hammond BR, Buch J, Sonoda L, Renzi-Hammond L. The effects of a senofilcon A contact lens with and without a photochromic additive on positive dysphotopsia across age. Eye Contact Lens Sci Clin Pract. 2021;47(5):265–270.
- Ernest PH. Light-transmission-spectrum comparison of foldable intraocular lenses. J Cataract Refract Surg. 2004;30(8):1755–1758.
- Buch J. Iris color scale based on quantitative color analysis. Annual Meeting of the American Academy of Optometry, 2011. 115048. https://www.aaopt.org/detail/knowledge-base-article/iris-color-scale-based-quantitative-color-analysis. Accessed August 21, 2019.
- Grimes DN, Thompson BJ. Two-point resolution with partially coherent light. J Opt Soc Am. 1967;57(11):1330–1334.
- Hammond BR, Bernstein B, Dong J. The effect of the AcrySof natural lens on glare disability and photostress. Am J Ophthalmol. 2009;148(2):272–276.e2.
- van den Berg TJTP, Ijspeert JK, de Waard PWT. Dependence of intraocular straylight on pigmentation and light transmission through the ocular wall. Vision Res. 1991;31(7–8):1361–1367.
- Olson MD, Miller KM. Implanting a clear intraocular lens in one eye and a yellow lens in the other eye: a case series. Am J Ophthalmol. 2006;141(5):957–958.
- Walls GL, Judd HD. The intra-ocular colour-filters of vertebrates. Br J Ophthalmol. 1933;17(11):641–675. http://www.ncbi.nlm.nih.gov/pubmed/18169162. Accessed November 12, 2018.
- Reading VM, Weale RA. Macular pigment and chromatic aberration. J Opt Soc Am. 1974;64(2):231–234.
- Jablonski NG, Chaplin G. The colours of humanity: the evolution of pigmentation in the human lineage. Philos Trans R Soc B Biol Sci. 2017;372:1724.
- Wooten BR, Geri GA. Psychophysical determination of intraocular light scatter as a function of wavelength. Vision Res. 1987;27(8):1291–1298.
- Coppens JE, Franssen L, Van Den Berg TJTP. Wavelength dependence of intraocular straylight. Exp Eye Res. 2006;82(4):688–692.