Abstract
Purpose
MiR-34a-5p is reported to be related with age-related nuclear cataract. This study investigated the mechanism of miR-34a-5p in the regulation of oxidative stress on lens epithelial cells.
Methods
The three candidate miRNAs were screened by CCK-8 assays after transfection of mimics or inhibitor in H2O2-treated HLE-B3 cells. The apoptosis, ROS level and GPX activity of HLE-B3 cells transfected with miR-34a-5p mimics or inhibitor were analysed by flow cytometry, cellular ROS and GPX activity test. The target genes of miR-34a-5p were predicted by proteomic and bioinformatic analysis. The relationship between miR-34a-5p and GPX3 were internally validated by qRT-PCR and Western blot and externally verified by dual-luciferase reporter assay. The effect of miR-34a-5p-GPX3 axis on regulation of oxidative stress in HLE-B3 cells were conducted by overexpression of GPX3 and tested by flow cytometry analysis, cellular ROS and GPX detection.
Results
The viability of H2O2-treated HLE-B3 cells were weakened by up-regulated miR-34a-5p. Cell apoptosis and oxidative damage were also induced by overexpression of miR-34a-5p. GPX3 and SRC were identified as target genes of miR-34a-5p by combined analysis of proteomic and bioinformatics, while GPX3 was selected for further research for its connection with anti-oxidation. Western blot and qRT-PCR tests proved that GPX3 is negatively regulated by miR-34a-5p. Dual-luciferase reporter assay verified that GPX3 is the direct target of miR-34a-5p. The increased oxidative stress induced by transfection of miR-34a-5p mimics in H2O2-treated HLE-B3 cells was attenuated by overexpression of GPX3.
Conclusions
MiR-34a-5p is a negative regulator of oxidative stress on lens epithelial cells and the mechanism is by silencing the expression of GPX3. These data suggest that miR-34a-5p may be a potential novel therapeutic target for the prevention and treatment of age-related cataract.
Introduction
Reactive oxygen species (ROS) is the downstream product of oxidative stress, which is thought to be a major predisposing factor in age-related cataract.Citation1 Hydrogen peroxide (H2O2) is the main source of cellular ROS and oxidative damage in lens epithelial cells (LEC). It is generated through multiple biological processes including aerobic respiration, ultraviolet light, inflammatory stimulus and etc.Citation2,Citation3 Normal lens is protected by the defense system containing antioxidants from oxidative damage through clearance of ROS and reduction of oxidized molecules.Citation4–6 However, the increasing age of lens tip the balance from reduction to oxidation, leading to the formation of cataract.Citation7,Citation8
By far, intraocular lens implantation following phacoemulsification is the most effective treatment of cataract. However, cataract surgery may result in complications such as posterior capsular opacity, glaucoma, endophthalmitis, uveitis, retinal detachment and etc.Citation9 In addition, the economic burden produced by cataract surgery, which is posted on both society and patients, is nonnegligible. Therefore, it is imperative to explore the mechanism of cataractogenesis, which may contribute to the discovery of alternative treatment.
MicroRNAs (miRNAs) are small noncoding RNAs consisting of 22 nucleotide base pairs, which could regulate gene expression at the posttranscriptional level by binding to the 3‘untranslated regions (UTRs) of target mRNAs, resulting in mRNA either translational repression or transcript degradation.Citation10 Evidence also indicates that some miRNAs are involved in the formation of cataract.Citation11,Citation12 We previously reported that miR-34a-5p, miR-630 and miR-335-3p are related to H2O2 induced oxidative stress in HLE-B3 cells.Citation13,Citation14 Whether or how these miRNAs affect the defense system of LEC against oxidative stress remains unclear.
Therefore, the purpose of the present study was to determine the exact miRNA which participate in the regulation of LEC’s antioxidant capacity and reveal the underlying mechanism. Our findings suggest that miR-34a-5p aggravates the H2O2-induced oxidative damage on HLE-B3 cells by directly silencing glutathione peroxidase 3 (GPX3) and may provide a potentially useful therapeutic target for the treatment of age-related cataract.
Materials and methods
Cell line and culture
The human lens epithelial cell line HLE-B3 was purchased from the American Type Culture Collection (ATCC, Manassas, VA, USA). HLE-B3 cells were grown as a monolayer in DMEM supplemented with 10% heat-inactivated fetal bovine serum (FBS), penicillin (100 U/ml), and streptomycin (100 µg/ml) at 37 °C in a humidified atmosphere of 5% CO2 and 21% O2. Twenty-four hours before the day of the experiment, cells were switched to hypoxic conditions (1% O2 to mock physiological environmentCitation15). At 85–90% confluence, the cells were treated with 75 µM H2O2 for 24 h as the previous study.Citation13
Proteomic analysis
Isobaric tags for relative and absolute quantification (iTRAQ) combined with nanoscale liquid chromatography-mass spectrometry (NanoLC − MS/MS) were used to identify proteins that were differentially expressed after 24 h incubation with 75 µM H2O2. HLE-B3 cells were harvested and 100 µg of the cell lysates were labeled with iTRAQ labeling reagents 114 (H2O2 treatment) and 115 (negative control) (Applied Biosystems, Foster City, CA). After strong cation exchange and NanoLC − MS/MS analysis, protein identification and iTRAQ quantitation were performed using ProteinPilot4.1 software (AB SCIEX, USA). Two physical replicates were performed. After identification and quantification, the differentially expressed proteins were selected and Gene Ontology (GO) analysis was applied to divide these proteins into specific categories.
Cell transfection
GPX3 expression vector was constructed using a pcDNA 3.1 expression vector (Gene Pharma Co., Ltd. Shanghai, China). Full-length GPX3 coding sequences were PCR amplifed and cloned into a pcDNA 3.1 expression vector according to a standard procedure. MiR-negative control, miR-34a-5p inhibitor and mimics, miR-630 inhibitor and mimics, miR-335-5p inhibitor and mimics were synthesized and purchased from Gene Pharma Co., Ltd (Shanghai, China). Cell transfection was performed with Lipofectamine 2000 (Invitrogen, Carlsbad, CA, USA) following the manufacturer’s instructions. Briefly, HLE-B3 cells were grown on six-well tissue culture plates to 80% confluence. Cells were then washed twice with PBS, miRNA inhibitor or miRNA mimics were mixed with Lipofectamine transfection reagent and transfected into the cells. After 6 h, the supernatant was removed, and fresh medium was added.
RNA extraction and quantitative real-time polymerase chain reaction (qRT-PCR)
The qRT-PCR method has been described in detail previously. In brief, total RNA from HLE-B3 cells were isolated using TRIzol Reagent (Invitrogen, Carlsbad, CA, USA) following the manufacturer’s instructions. To determine the expression of the miRNA and mRNA, we employed the qRT-PCR using the Fast SYBR Green Chemistry (TaKaRa, Japan). The primers are as follows: GPX3 forward primer, TGGTCATTCTGGGCTTTCCC; GPX3 reverse primer, TCCACCTGGTCGGACATACT; miR-34a-5p forward primer, CTGGCAGTGTCTTAGCTGGTTGT; miR-630 forward primer, GCGAGTATTCTGTACCAGGGAAGGT; miR-335-3p forward primer, CGGCGTTTTTCATTATTGCTCCTGACC. Real-time PCR was performed in triplicate on CFX96 Real Time PCR Detection System (Bio-Rad, West Berkeley, CA). The 2−ΔΔCT method was used to determine the relative gene expression, and mature miRNA were normalized to U6-snRNA.
Cell viability
Cell Counting Kit-8 (CCK-8; Dojindo Molecular Technologies, Kumamoto, Japan) was used to detect the viability of HLE-B3 cells according to the manufacturer's protocol. Forty-eight hours after transfection, 5000 cells were seeded into each well of a 96-well plate and incubated. A 10-µl aliquot of CCK-8 was added to quintuplicate wells and incubated for 2 h. To calculate the number of viable cells in each well, the absorbance at 460 nm was measured by a microplate reader (Bio-Rad, West Berkeley, CA).
Target gene prediction and dual luciferase reporter assay
Target genes of the miR-34a-5p were determined by the union of miRNA target predictions from TargetScan 7.1Citation16 and miRanda.Citation17 The picked genes were further analyzed according to the PANTHER classification system.Citation18 The wild-type (WT) and mutant-type (MT) GPX3 luciferase reporter plasmids were obtained from GeneChem Co., Ltd (Shanghai, China), which contain the miR-34a-5p sequences with or without the GPX3 binding sites. To explore the target association between miR-34a-5p and GPX3, Lipofectamine 2000 was used to co-transfect HLE-B3 cells with WT or MT GPX3 luciferase reporter plasmid and miR-34a-5p or NC mimics. Following 48 h, the luciferase activity was measured by the Dual Luciferase Reporter Assay System (Promega Corp., Madison, WI, USA) according to the manufacturer’s protocol.
Western blot
Cells were harvested, rinsed in PBS, and lysed in RIPA buffer containing 5% PMSF for 1 h on ice. After the mixture was centrifuged at 12,000×g for 10 min at 4 °C, insoluble materials were removed. Identical amount (50 µg of protein) of cell lysates were boiled for 5 min, size fractionated by SDS-PAGE, and electrophorectically transfected on to PVDF membranes. After being incubated with blocking solution including 5% powered milk in TBST buffer (10 mM Tris–HCl, 150 mM NaCl, and 0.1% Tween-20) for 1 h at room temperature, the membranes were immunoblotted with primary antibodies (Cell Signaling Technology, USA.). Primary antibodies were identified using HRP-conjugated secondary antibody at a 1:10,000 dilution and visualized by the ECL detection system.
Cell apoptosis
HLE-B3 cells transfected with miRNA inhibitor or miRNA mimics were incubated in a six-well plate at a density of 5 × 105 cells per well. After incubation, the cells were washed twice with PBS and harvested by trypsin digestion. Cells were collected and centrifuged at 500 rpm for 5 min, the supernatant was discarded, and 5 µl of Annexin V-FITC and 10 µl of propidium iodide (PI) (Sangon Biotech, Shanghai, China) were added to the cell pellet followed by 15 min incubation in the dark at room temperature. Samples were gently shaken and diluted with binding buffer (140 mM NaCl, 2.5 mM CaCl2, and 10 mM Hepes) and then analyzed by flow cytometry within 30 min of processing.
Intracellular ROS detection
The detection of ROS generation was conducted by measuring and visualising an oxidation-sensitive fluorescein DCFH-DA (BioVision, Shanghai, China). The untreated cells were harvested, washed with PBS two times, and treated with DCFH-DA (10 µM, 37 °C) for 30 min. After the treatment, ROS generation was examined by a microplate reader and a fluorescence microscope (495 nm, 562 nm). All images were observed using fluorescent microscopy (Nikon, Eclipse CI, Tokyo, Japan). Data were expressed as % of control.
GPX activity
The activity of GPX was determined through an indirect method, using 2.0 mM reduced glutathione and 0.042% (w/w) hydrogen peroxide (H2O2) as a substrate. In the presence of glutathione reductase at a concentration 0.75 mU and 0.25 mM of NADPH, the oxidized glutathione was immediately converted to a reduced form with concomitant oxidation of NADPH to NAD+. The decrease in absorbance was then measured at 415 nm and 37 °C. This assay was performed on the supernatant. The necessary enzyme activity to convert one µmol of NADPH to NADP in 1 min was defined as the activity of GPX, and the results were expressed as GPX units/mg protein. The levels of GPX were calculated from standard curves constructed from standard GPX.
Statistical analysis
All the results were presented as means ± SD. Statistical difference were analyzed using SPSS 20.0 statistical software (IBM Corporation, USA). Two-tailed Student’s t-test was used to evaluate experiments with two experimental groups. The results were considered statistically significant when *p < 0.05; **p < 0.01; ***p < 0.001.
Results
miR-34a-5p weakens the viability of H2O2-treated HLE-B3 cells
MiR-34a-5p, miR-630 and miR-335-5p were chosen as candidate miRNAs based on our previous study. In this research, functional screening was applied to compare the three candidate miRNAs’ effect on H2O2-treated HLE-B3 cells. After transfection, the expression levels of miR-34a-5p, miR-630 and miR-335-5p were markedly altered in the HLE-B3 cells compared with miR-NC group, respectively (p < 0.001, p < 0.001, p < 0.001, mimics vs NC; p < 0.05, p < 0.05, p < 0.05, inhibitor vs NC; ). After incubation with 75 µM H2O2 for 24 h, CCK-8 assay was used to test the viability of the transfected cells. MiR-34a-5p (p < 0.01) and miR-630 (p < 0.05) inhibitor significantly improved the viability of HLE-B3 cells, while the influence of miR-34a-5p mimics/inhibitor transfection on cell viability was greater than that of miR-630, respectively (). Meanwhile, the effect of miR-335-5p mimics/inhibitor transfection was insignificant (). Therefore, miR-34a-5p was selected for further experiment.
Figure 1. Effect of miR-34a-5p, miR-630 and miR-335-5p transfection on the viability of HLE-B3 cells. (A–C) The expression levels of miR-34a-5p, miR-630 and miR-335-5p expression were detected by qRT-PCR in the HLE-B3 cell after transfection with miR-34a-5p, miR-630 and miR-335-5p mimics and inhibitor, respectively. (C–F) CCK-8 assay was applied to observe the effect of miR-34a-5p, miR-630 and miR-335-5p mimics/inhibitor transfection on cell viability. *p < 0.05; **p < 0.01; ***p < 0.001.
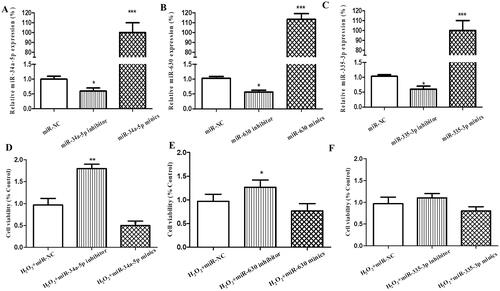
miR-34a-5p accelerates apoptosis and oxidation in H2O2-treated HLE-B3 cells
Apoptosis of HLE-B3 cells transfected with miR-34a-5p mimics or inhibitor were analyzed by flow cytometry after H2O2 treatment. As demonstrated in , overexpression of miR-34a-5p induced more significant cell apoptosis than the miR-NC group (p < 0.05), whereas inhibition of miR-34a-5p suppressed cell apoptosis (p < 0.05). Quantitation of ROS levels using DCFH-DA assay displayed that ROS level was prominently increased after overexpression of miR-34a-5p in HLE-B3 cells (p < 0.01), besides, the amount of ROS was decreased in HLE-B3 cells transfected with miR-34a-5p inhibitor (p < 0.05) ().
Figure 2. Effect of miR-34a-5p on apoptosis and ROS level of HLE-B3 cells. (A) Apoptosis of HLE-B3 cells transfected with miR-34-5p mimics and inhibitor after H2O2 exposure were determined by flow cytometry. (B) DCFH-DA assay was used to detect the ROS level of HLE-B3 cells transfected with miR-34-5p mimics and inhibitor. *p < 0.05; **p < 0.01.
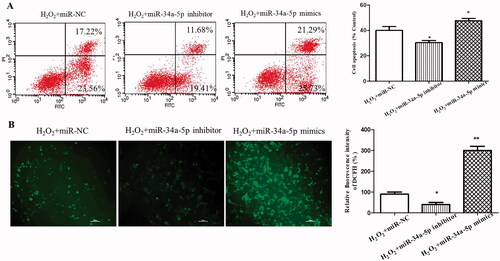
Screening target genes of miR-34a-5p by proteomic and bioinformatic analysis
In this study, by combining iTRAQ reagent labeling followed by NanoLC − MS/MS analysis with miRNA target gene prediction (), we aimed to find out the target genes of miR-34a-5p precisely and efficiently. Using Protein Pilot 4.1, we identified 4836 proteins and quantified 3631 proteins in the two replicates (Supplemental File 1 and Figure S1). Compared with the NC group, 213 proteins were upregulated (the iTRAQ ratio 114:115 > 1.5) and 165 proteins were downregulated (the iTRAQ ratio 114:115 < 0.67) after H2O2 treatment (). The 165 downregulated proteins went through GO analysis and 28 of the 165 hit the “response to stress” category (Supplemental File 2). Meanwhile, target gene prediction of miR-34a-5p was conducted as before and 68 genes were clustered in the response to stress process (Supplemental File 3–4 and Figure S2). After combining the two results, GPX3 and SRC were selected as the targets (). The protein encoded by SRC is a tyrosine-protein kinase and SRC is considered as a proto-oncogene. This gene is closely related with tumorgenesis, whereas the protein encoded by GPX3 is glutathione peroxidase 3, which is a member of the glutathione peroxidase family and catalyze the reduction of organic hydroperoxides and hydrogen peroxide (H2O2) by glutathione, and thereby protect cells against oxidative damage. Therefore, GPX3 was chosen for further research.
Figure 3. Combined analysis of proteomics and bioinformatics for screening target genes of miR-34a-5p. (A) Flow chart of proteomic analysis. (B) Total identified, quantified proteins and differentially expressed proteins. (C) Target gene selection by combining results of proteomic and bioinformatics analysis.
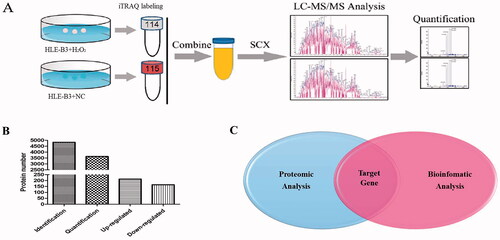
GPX3 Is the direct downstream target of miR-34a-5p
Overexpression of miR-34a-5p in HLE-B3 cells resulted in a significant decrease of GPX3 in both mRNA () and protein () levels. Whereas, knockdown of the endogenous miR-34a-5p by the inhibitor increased GPX3 mRNA and protein levels (). Analysis using 3’untranslated region (UTR) luciferase reporter plasmids containing the miR-34a-5p target sequences (wild-type or mutant) on GPX3 was performed to determine whether GPX3 is a direct target of miR-34a-5p. Expression of miR-34a-5p caused a significant decrease in luciferase activity in the wild-type GPX3 3’ UTR, but not in the mutant form (). Taken together, these results indicate that GPX3 is the direct downstream target of miR-34a-5p in HLE-B3 cells.
Figure 4. GPX3 is the downstream target of miR-34-5p. (A) qRT-PCR was applied to observe the expression of GPX3 in HLE-B3 cells transfected with miR-34-5p mimics and inhibitor. (B) Western blot was used to detect the expression of GPX3 in HLE-B3 cells transfected with miR-34-5p mimics and inhibitor. (C) The complementary binding site of GPX3 and miR-34-5p was predicted by TargetScan. (D) Luciferase reporter assay was performed to confirm the predicted binding of GPX3 and miR-34-5p. *p < 0.05.
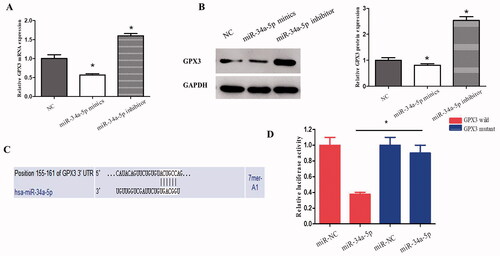
miR-34-5p aggravates apoptosis and oxidative damage of HLE-B3 cells by targeting GPX3
To determine whether the miR-34-5p-GPX3 axis is effective, we transfected the H2O2-treated HLE-B3 cells with miR-34-5p mimics. As shown in , the mRNA and protein levels of GPX3 were significantly decreased. The activity of GPX was attenuated as the same (), even though GPX3 is only a member of the GPX family. Meanwhile, functional experiments demonstrated that cell apoptosis and endogenous ROS level were elevated by the transfection of miR-34-5p mimics ().
Figure 5. Further validation of miR-34-5p-GPX3 axis. (A) The mRNA level of GPX3 in H2O2-treated HLE-B3 cells which were transfected with miR-NC, miR-34a-5p mimics and miR-34a-5p mimics + GPX3 overexpression, respectively. (B) Western blot analysis determining the changes of GPX3 expression level in control, miR-34-5p with/without GPX3 overexpression. (C) GPX activity of the three transfection groups. (D) Percent cell apoptosis of HLE-B3 cells for the aforementioned groups. (E) The amount of cellular ROS in the above mentioned groups. *p < 0.05, **p < 0.01, ***p < 0.001.
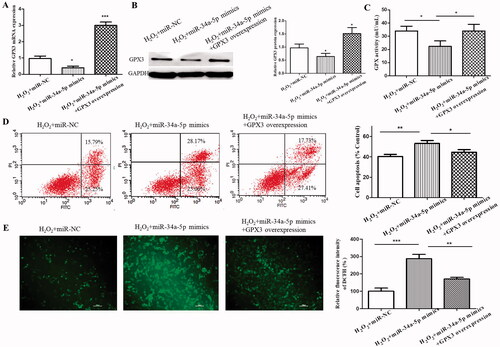
To further verify the involvement of GPX3 in the miR-34-5p-induced cell apoptosis and oxidative stress, the endogenous GPX3 was overexpressed. As a consequence, the expression level of GPX3 () and the activity of GPX () were rescued. More importantly, the increased cell apoptosis and ROS level were alleviated by the incoming GPX3. The data indicate that GPX3 is crucial for miR-34-5p-mediated apoptosis and oxidative damage in HLE-B3 cells.
Discussion
Cataract, the clouding crystalline lens, is most common cause of blindness worldwide and age-related cataract accounts for the largest percentage.Citation19,Citation20 There are various factors related to cataractogenesis, including smoking, ultraviolet (UV) radiation, drug use and other ocular diseases. Notably, oxidative stress is the most important factor of age-related cataract.Citation8 However, the specific molecular mechanism remains unclear. Therefore, it is of great significance to explore the exact molecular mechanism underlying oxidative stress induced cataract, which might contribute to identify new target therapy for cataract.
A large number of miRNAs have been reported to involve in oxidative stress induced diseases.Citation21–23 At the meantime, miRNAs were also found to participate in lens differentiation,Citation24 cataractogenesisCitation25,Citation26 and posterior capaular opacification (PCO).Citation27 Our previous study discovered that miR-34a-5p, miR-630 and miR-335-5p are correlated with age-related nuclear cataract.Citation13 In this study, the three candidates went through functional experiments. The results showed that miR-34a-5p inflicted the most significant impact on the viability of H2O2-treated HLE-B3 cells. Furthermore, apoptosis and ROS level of H2O2-treated HLE-B3 cells were also affected by overexpression or interference of miR-34a-5p. MiR-34a-5p is one of the most explored miRNAs in oxidative stress and cell senescence.Citation28 More than 700 target genes of miR-34a-5p have been discovered and researched.Citation29 However, the mechanism of miR-34a-5p regulating oxidative damage of HLE-B3 cells is still uncertain.
By regulating the expression of associated genes, miRNAs regulate a wide range of biological functions, including cell proliferation, migration, differentiation and apoptosis. As reported, a single miRNA can regulate hundreds of mRNAs, and a single mRNA might be regulated by multiple miRNAs.Citation30 In order to select the target genes of miR-34a-5p, we applied iTRAQ method and bioinformatic analysis in this study. Currently, iTRAQ has unique advantages over other conventional proteomics techniques for its high throughput and accuracy.Citation31 Moreover, the iTRAQ approach has also been successfully employed for the identification of miRNA targets.Citation32,Citation33 In our research, after H2O2 treatment, 378 proteins were identified as differentially expressed proteins, among which 165 were downregulated compared with NC group. Twenty eight of the 165 clustered in the category of “response to stress” after GO analysis. Together with the 68 genes picked from bioinformatic analysis, GPX3 and SRC stood out as the predicted target genes of miR-34a-5p by both approach. SRC is known as a proto-oncogene for its involvement in tumorgenesis,Citation34 metastasisCitation35 and EMT.Citation36 Meanwhile, the function of GPX3 is closely related with anti-oxidation. Recent study showed that increased GPX3 inhibited lens epithelium cell apoptosis via protecting cells from oxidative stress.Citation37,Citation38 In all, GPX3 is a better matched target gene in our research.
Then, solid proof is required to prove the relationship between miR-34a-5p and GPX3. After transfection of miR-34a-5p mimics/inhibitor, the expression levels of GPX3 in HLE-B3 cells were inversely changed. This is evidence of the internal regulation of miR-34a-5p on GPX3. To complete the evidence chain, we need to validate the regulation externally. Dual-luciferase reporter assay showed that GPX3 is a direct target of miR-34a-5p in HLE-B3 cells. This is the first time that GPX3 was reported as the downstream target of miR-34a-5p.
In the functional experiments, we noticed that upregulation of miR-34a-5p may impair HLE-B3 cells’ defense against oxidative damage and the consequences are increased cell apoptosis and accumulated endogenous ROS. Intriguingly, we also found that miR-34a-5p could reduce the activity of GPX, even though the GPX family consists of 8 members. On the contrary, all these phenotypes could be rescued by the overexpression of GPX3. These findings demonstrate that miR-34a-5p regulates the oxidative stress of HLE-B3 cells by negatively regulating GPX3 and the miR-34a-5p-GPX3 axis is crucial for the survival of HLE-B3 cells under oxidative stress.
This research was conducted on a lens epithelia cell line, which may limit the conclusion since the absence of follow up in primary lens epithelia or whole lens culture. However, the current study still provides new insights regarding the importance of miR-34a-5p in keeping the balance of redox in HLE-B3 cells. MicroRNAs are believed to have potential therapeutic value for many diseases. These data indicate that miR-34a-5p may have important clinical consequences in the treatment of age-related cataract by directly silencing GPX3.
Author contributions
Wang Song: Conceptualization, Validation, Resources, Investigation, Data Curation, Writing – Original Draft
Yu Mengsi: Conceptualization, Formal analysis, Investigation, Visualization
Liu Jun: Resources, Investigation, Data Curation
Guo Chenjun: Investigation, Data Curation
Yan Hong: Conceptualization, Writing – Review & Editing, Supervision, Project administration, Funding acquisition
Supplemental Material
Download Zip (2.3 MB)Disclosure statement
No potential conflict of interest was reported by the author(s).
Additional information
Funding
References
- Truscott RJ. Age-related nuclear cataract-oxidation is the key. Exp Eye Res. 2005;80(5):709–725. doi:10.1016/j.exer.2004.12.007.
- Babizhayev MA. Generation of reactive oxygen species in the anterior eye segment. Synergistic codrugs of N-acetylcarnosine lubricant eye drops and mitochondria-targeted antioxidant act as a powerful therapeutic platform for the treatment of cataracts and primary open-angle glaucoma. BBA Clin. 2016;6:49–68. doi:10.1016/j.bbacli.2016.04.004.
- Sacca SC, Cutolo CA, Ferrari D, Corazza P, Traverso CE. The eye, oxidative damage and polyunsaturated fatty acids. Nutrients. 2018;10(6):668. doi:10.3390/nu10060668.
- Lou MF. Redox regulation in the lens. Prog Retin Eye Res. 2003;22(5):657–682. doi:10.1016/S1350-9462(03)00050-8.
- Tsang CK, Liu Y, Thomas J, Zhang YJ, Zheng XF. Superoxide dismutase 1 acts as a nuclear transcription factor to regulate oxidative stress resistance. Nat Commun. 2014;5:3446. doi:10.1038/ncomms4446.
- Ivanov IV, Mappes T, Schaupp P, Lappe C, Wahl S. Ultraviolet radiation oxidative stress affects eye health. J Biophotonics. 2018;11(7):e201700377. doi:10.1002/jbio.201700377.
- Giblin FJ. Glutathione: a vital lens antioxidant. J Ocul Pharmacol Ther. 2000;16(2):121–135. doi:10.1089/jop.2000.16.121.
- Beebe DC, Holekamp NM, Shui YB. Oxidative damage and the prevention of age-related cataracts. Ophthalmic Res. 2010;44(3):155–165. doi:10.1159/000316481.
- Toda J, Kato S, Oshika T, Sugita G. Posterior capsule opacification after combined cataract surgery and vitrectomy. J Cataract Refract Surg. 2007;33(1):104–107. doi:10.1016/j.jcrs.2006.09.018.
- He L, Hannon GJ. MicroRNAs: small RNAs with a big role in gene regulation. Nat Rev Genet. 2004;5(7):522–531. doi:10.1038/nrg1379.
- Wu CR, Lin HT, Wang QL, Chen W, Luo HH, Chen WR, Zhang H. Discrepant expression of microRNAs in transparent and cataractous human lenses. Invest Ophthalmol Vis Sci. 2012;53(7):3906–3912. doi:10.1167/iovs.11-9178.
- Chen XY, Xiao W, Chen WR, Liu XL, Wu MX, Bo Q, Luo Y, Ye SB, Cao YH, Liu YZ. MicroRNA-26a and -26b inhibit lens fibrosis and cataract by negatively regulating Jagged-1/Notch signaling pathway. Cell Death Differ. 2017;24(8):1431–1442. doi:10.1038/cdd.2016.152.
- Wang S, Guo CJ, Yu MS, Ning XN, Yan B, Zhao J, Yang AG, Yan H. 2018. Identification of H2O2 induced oxidative stress associated microRNAs in HLE-B3 cells and their clinical relevance to the progression of age-related nuclear cataract. BMC Ophthalmol. 18(1):93–104. doi:10.1186/s12886-018-0766-6.
- Mei L, Yan H, Wang S, Guo CJ, Zheng XL, Yan B, Zhao J, Yang AG. Upregulation of miR-630 induced by oxidative damage resists cell migration through targeting ALCAM in human lens epithelium cells. Curr Eye Res. 2020;45(2):153–161. doi:10.1080/02713683.2019.1656748.
- Neelam S, Brooks MM, Cammarata PR. Lenticular cytoprotection. Part 1: the role of hypoxia inducible factors-1alpha and -2alpha and vascular endothelial growth factor in lens epithelial cell survival in hypoxia. Mol Vis. 2013;19:1–15.
- Bartel DP. MicroRNAs: target recognition and regulatory functions. Cell. 2009;136(2):215–233. doi:10.1016/j.cell.2009.01.002.
- Betel D, Koppal A, Agius P, Sander C, Leslie C. Comprehensive modeling of microRNA targets predicts functional non-conserved and non-canonical sites. Genome Biol. 2010;11(8):R90. doi:10.1186/gb-2010-11-8-r90.
- Mi H, Muruganujan A, Casagrande JT, Thomas PD. Large-scale gene function analysis with the PANTHER classification system. Nat Protoc. 2013;8(8):1551–1566. doi:10.1038/nprot.2013.092.
- Chew MC, Lim LW, Tan CS. Multimedia interventions on the informed consent process for cataract surgery. Indian J Ophthalmol. 2014;62(11):1102–1103. doi:10.4103/0301-4738.146732.
- West S. Epidemiology of cataract: accomplishments over 25 years and future directions. Ophthalmic Epidemiol. 2007;14(4):173–178. doi:10.1080/09286580701423151.
- Nosalski R, Siedlinski M, Denby L, McGinnigle E, Nowak M, Cat AN, Ruiz LM, Cantini M, Skiba D, Wilk G, et al. 2020. T-cell-derived miRNA-214 mediates perivascular fibrosis in hypertension. Circ Res. 126(8):988–1003. doi:10.1161/CIRCRESAHA.119.315428.
- Zeng J, Zhu L, Liu J, Zhu T, Xie ZH, Sun XO, Zhang H. Metformin protects against oxidative stress injury induced by ischemia/reperfusion via regulation of the lncRNA-H19/miR-148a-3p/Rock2 axis. Oxid Med Cell Longev. 2019;2019(12):8768327. doi:10.1155/2019/8768327.
- Zhuang XY, Li RS, Maimaitijiang A, Liu RC, Yan FY, Hu HB, Gao XF, Shi HM. miR-221-3p inhibits oxidized low-density lipoprotein induced oxidative stress and apoptosis via targeting a disintegrin and metalloprotease-22. J Cell Biochem. 2019;120(4):6304–6314. doi:10.1002/jcb.27917.
- Wolf L, Gao CS, Gueta K, Xie Q, Chevallier T, Podduturi NR, Sun J, Conte I, Zelenka PS, Padan RS, et al. Identification and characterization of FGF2-dependent mRNA: microRNA networks during lens fiber cell differentiation. G3. 2013;3(12):2239–2255. doi:10.1534/g3.113.008698.
- Varma SD, Kovtun S, Hegde K, Yin J, Ramnath J. Effect of high sugar levels on miRNA expression: studies with galactosemic mice lenses. Mol Vis. 2012;18:1609–1618.
- Varma SD, Kovtun S. Protective effect of caffeine against high sugar-induced transcription of microRNAs and consequent gene silencing: a study using lenses of galactosemic mice. Mol Vis. 2013;19:493–500.
- Hoffmann A, Huang Y, Suetsugu-Maki R, Ringelberg CS, Tomlinson CR, Del Rio-Tsonis K, Tsonis PA. Implication of the miR-184 and miR-204 competitive RNA network in control of mouse secondary cataract. Mol Med. 2012;18:528–538. doi:10.2119/molmed.2011.00463.
- Ito T, Yagi S, Yamakuchi M. MicroRNA-34a regulation of endothelial senescence. Biochem Biophys Res Commun. 2010;398(4):735–740. doi:10.1016/j.bbrc.2010.07.012.
- Chou C-H, Chang N-W, Shrestha S, Hsu S-D, Lin Y-L, Lee W-H, Yang C-D, Hong H-C, Wei T-Y, Tu S-J, et al. miRTarBase 2016: updates to the experimentally validated miRNA-target interactions database. Nucleic Acids Res. 2016;44(D1):D239–D247. doi:10.1093/nar/gkv1258.
- Bartel DP. MicroRNAs: genomics, biogenesis, mechanism, and function. Cell. 2004;116(2):281–297. doi:10.1016/s0092-8674(04)00045-5.
- Evans C, Noirel J, Ow SY, Salim M, Pereira-Medrano AG, Couto N, Pandhal J, Smith D, Pham TK, Karunakaran E, et al. An insight into iTRAQ: where do we stand now. Anal Bioanal Chem. 2012;404(4):1011–1027. doi:10.1007/s00216-012-5918-6.
- Li C, Xiong Q, Zhang J, Ge F, Bi LJ. Quantitative proteomic strategies for the identification of microRNA targets. Expert Rev Proteomics. 2012;9(5):549–559. doi:10.1586/epr.12.49.
- Ou M, Zhang X, Dai Y, Gao JY, Zhu MS, Yang XC, Li YC, Yang T, Ding M. Identification of potential microRNA-target pairs associated with osteopetrosis by deep sequencing, iTRAQ proteomics and bioinformatics. Eur J Hum Genet. 2014;22(5):625–632. doi:10.1038/ejhg.2013.221.
- Han T, Jiang S, Zheng H, Yin Q, Xie MY, Little MR, Yin X, Chen M, Song SJ, Beg AA, et al. Interplay between c-Src and the APC/C co-activator Cdh1 regulates mammary tumorigenesis. Nat Commun. 2019;10(1):3716. doi:10.1038/s41467-019-11618-7.
- Zhang J, Wang S, Jiang B, Huang LH, Ji ZL, Li XT, Zhou HM, Han AD, Chen A, Wu YN, et al. c-Src phosphorylation and activation of hexokinase promotes tumorigenesis and metastasis. Nat Commun. 2017;8:13732. doi:10.1038/ncomms13732.
- Huang RY, Wong MK, Tan TZ, Kuay KT, Ng AH, Chung VY, Chu YS, Matsumura N, Lai HC, Lee YF, et al. An EMT spectrum defines an anoikis-resistant and spheroidogenic intermediate mesenchymal state that is sensitive to e-cadherin restoration by a src-kinase inhibitor, saracatinib (AZD0530). Cell Death Dis. 2013;4(11):e915. doi:10.1038/cddis.2013.442.
- Tu YY, Li LL, Qin B, Wu J, Cheng TY, Kang LH, Guan HJ. Long noncoding RNA glutathione peroxidase 3-antisense inhibits lens epithelial cell apoptosis by upregulating glutathione peroxidase 3 expression in age-related cataract. Mol Vis. 2019;25(25):734–744.
- Reddy AT, Lakshmi SP, Banno A, Reddy RC. Role of GPx3 in PPARγ-induced protection against COPD-associated oxidative stress. Free Radic Biol Med. 2018;126:350–357. doi:10.1016/j.freeradbiomed.2018.08.014.