Abstract
Purpose
Age-related macular degeneration (AMD) is the commonest cause of permanent vision loss in the elderly. Traditional Chinese medicine (TCM) has long been used to treat AMD, although the underlying functional mechanisms are not understood. This study aims to predict the active ingredients through screening the chemical ingredients of anti-AMD decoction and to elucidate the underlying mechanisms.
Methods
We collected the prescriptions for effective AMD treatment with traditional Chinese medicine and screened several Chinese medicines that were used most frequently in order to compose "anti-AMD decoction." The pharmacologically active ingredients and corresponding targets in this anti-AMD decoction were mined using the Traditional Chinese Medicine Systems Pharmacology (TCMSP) database. Subsequently, the AMD-related targets were identified through the GeneCards database. Network pharmacology was performed to construct the visual network of anti-AMD decoction-AMD protein-protein interaction (PPI). Further, the Autodock software was adopted for molecular docking on the core active ingredients and core targets. The function of core ingredients against oxidative stress and inflammation in retinal pigment epithelial cells was assessed using biochemical assays.
Results
We screened out 268 active ingredients in anti-AMD decoction corresponding to 258 ingredient targets, combined with 2160 disease targets in AMD, and obtained 129 drug-disease common targets. The key core proteins were predominantly involved in inflammation. Furthermore, molecular docking showed that four potential active ingredients (Quercetin, luteolin, naringenin and hederagenin) had good affinity with the core proteins, IL-6, TNF, VEGFA and MAPK3. Quercetin, luteolin and naringenin demonstrated capacities against oxidative stress and inflammation in human retinal pigment epithelial cells.
Conclusions
The data suggests that anti-AMD decoction has multiple functional components and targets in treating AMD, possibly mediated by suppression of oxidative stress and inflammation.
Introduction
Age-related macular degeneration (AMD), the most common cause of vision loss in the elderly, is a degenerative disease in the central macula area of the neuroretina and the supporting retinal pigment epithelium.Citation1 By 2040, approximately 288 million people worldwide will suffer from AMD, which is also the main cause of blindness in the elderly.Citation2 AMD is a progressive, multi-gene and multi-factorial disease with complex etiology. In addition to the association of innate immune pathway genes with AMD susceptibility, environmental risk factors, such as smoking, obesity and sun exposure are also known to be associated with AMD.Citation3,Citation4 Its main pathological features are abnormal deposits underneath the retinal pigment epithelium (RPE) layer, RPE cell atrophy and progressive photoreceptor cell death.Citation5 However, the exact pathophysiology of AMD has not been fully elucidated, which hampers the possibility of developing efficient therapeutic options.Citation6
Traditional Chinese medicine (TCM) has shown unique advantages in the treatment of AMD from both a holistic and syndrome differentiation perspective.Citation7 In China, many confirmed cases of AMD have received TCM treatment. Some practitioners, on the basis of their own clinical experience, conduct syndrome differentiation in the treatment of AMD patients, formulating a combination of Chinese medicine that achieves more efficacious clinical outcomes.Citation7 Typically the formulae of anti-AMD Decoctions are complicated, and the underlying mechanisms that effectively protect against AMD remain unclear. Therefore, we need to use new approaches that allow further exploration of the ingredient targets and the main mechanisms of anti-AMD decoction.
With the development of bioinformatics, network pharmacology has become a highly effective method for studying TCM, as it can reveal, in a systematic manner, the relationship between the bioactive ingredients of TCM and their potential mechanisms.Citation8 It offers a new approach to studying the multi-targeted mechanisms of TCM in the treatment of complex diseases such as AMD.Citation9 Molecular docking technology can clarify the principle of the efficacy of TCM at the molecular level, study the geometric structure and spatial interaction between drug molecules and target proteins, and make the research of TCM more rigorous and scientific.Citation10
In the present study, an anti-AMD decoction was constructed, comprising ten Chinese medicines that are cited frequently in the relevant literature as clinically effective treatments of AMD. To a certain extent, the decoction represents the common Chinese medicines used in the clinical treatment of AMD. We used network pharmacology and molecular docking technologies to predict the active ingredients and targets of anti-AMD decoction, construct the associated visual, multi-level and multi-dimensional network diagram, and systematically explore the underlying mechanisms. We also validated the predicted ingredients in the anti-AMD decoction using biochemical approaches. The study offers novel methods for identifying functional ingredients and elucidating associated molecular mechanisms, while promoting the development of modern Chinese medicine and the discovery and introduction of new drugs.
Materials and methods
Collecting the clinically effective Chinese medicine formulae
Using "AMD" as the keyword, we searched the clinically effective Chinese medicine formulae in the treatment of AMD in the China National Knowledge Infrastructure Database (CNKI, http://www.cnki.net/; https://cn.bing.com/academic/search?q=%E4%B8%AD%E5%9B%BD%E7%9F%A5%E7%BD%91), WeiPu (VIP, http://www.cqvip.com; https://cn.bing.com/academic/search?q=%E7%BB%B4%E6%99%AE&qs=n&form=QBRE&sp=-1&pq=%E7%BB%B4%E6%99%AE&sc=0-2&sk=&cvid=01277BFB29E142528A6ECBE28433090A&ghsh=0&ghacc=0), WanFang database (http://www.wanfangdata.com.cn/index.html; https://cn.bing.com/academic/search?q=%E4%B8%87%E6%96%B9), Chinese Biomedical Literature Service System (CBM, http://www.sinomed.ac.cn/; https://cn.bing.com/search?q=%e4%b8%ad%e5%9b%bd%e7%94%9f%e7%89%a9%e5%8c%bb%e5%ad%a6%e6%96%87%e7%8c%ae%e6%9c%8d%e5%8a%a1%e7%b3%bb%e7%bb%9f&FORM=HDRSC1), and PubChem (https://www.ncbi.nlm.nih.gov/pubmed/). After assessing the information in the above databases, we identified ten Chinese medicines that were used frequently in Chinese medicine formulae and combined them into an anti-AMD decoction.
Screening of active ingredients
Data regarding the ingredients of the anti-AMD decoction were mainly collected from the Traditional Chinese Medicine Systems Pharmacology Database and Analysis Platform (TCMSP) (http://lsp.nwu.edu.cn/tcmsp.php; https://old.tcmsp-e.com/load_intro.php?id=40) to identify all the active ingredients.Citation11 At the same time, with oral bioavailability (OB)≥30% and drug likeness (DL)≥0.18 as the filter conditions,Citation12 the active ingredients of anti-AMD decoction were screened.
Identification of potential targets
The target of active ingredients was retrieved by TCMSP database. The Universal Protein database (UniProt, https://www.uniprot.org/) was utilized to convert the protein name of the anti-AMD decoction active ingredients to the gene names.Citation13 The search results were filtered to retain only studies conducted on “Homo sapiens (Human)” so that the names could be standardized and data duplicates deleted.
Prediction of potential targets of anti-AMD decoction
The GeneCards database (https://www.genecards.org/) provides functional information for all known and putative human genes.Citation14 Using the GeneCards database with "AMD" as the keyword, the species was designated as "Homo sapiens (Human)" to obtain the target of AMD, and a Venn diagram drawn with the Venny 2.1 online mapping tool platform (https://bioinfogp.cnb.csic.es/tools/venny/) to obtain an intersection of the two, which would represent a potential target for the treatment of AMD with anti-AMD decoction.
Construction of anti-AMD decoction treatment of AMD related network
The obtained potential targets, their corresponding active ingredients, and the anti-AMD decoction corresponding to the potential active ingredients were imported into Cytoscape 3.7.2 software (http://www.cytoscape.org/) to construct the network of anti-AMD decoction for the treatment of AMD.Citation15
Construction of anti-AMD Decoction-AMD protein-protein interaction (PPI) core network
First, we entered the obtained potential targets into the STRING database (https://string-db.org/), which stores known and predicted protein interaction information, including direct and indirect interactions between proteins.Citation16,Citation17 Only “Homo sapiens” proteins with a confidence score higher than 0.4 were picked out. Next, the data of protein interaction relationship was obtained, and the results were exported in tab-separated value (TSV) format. These data were imported into Cytoscape 3.7.2 software to construct the anti-AMD decoction-AMD PPI network. Three centrality measures were used to filter the data, including degree centrality (DC), closeness centrality (CC), and betweenness centrality (BC). The data were preliminarily processed by the screening criteria of “DC ≥ median DC,” and then secondarily screened as core targets by the screening criteria of “DC, BC, and CC greater than or equal to their median.”
Molecular docking of potential targets and active ingredients
We downloaded the four-dimensional structure diagram of the target proteins with the highest median value of the PPI network in the Protein Data BankCitation18 (PDB, http://www.RCSB.org/) database. AutoDock Tool software (version 1.5.6, http://autodock.scripps.edu/) was used to further process the protein, which mainly involved removing water molecules, separating ligands, hydrogenating and calculating the charge of the target protein, and using it as a receptor macromolecule. We then downloaded the structure diagram of the potential active ingredients of Chinese medicine in the ZINC 15 database (http://zinc.docking.org/),Citation19 used the AutoDock Tool to add charges, assign atom types, confirm rotatable flexible bonds, and used it as the ligand molecules. Finally, the docking results of active compounds and protein targets were visualized with PyMOL software (version 2.2, https://pymol.org/2/).
Cell treatment and cell viability
Human retinal pigment epithelial (RPE) cells (ARPE-19, ATCC CRL-2302™, passage number 4) were cultured in Dulbecco’s Modified Eagle Medium (DMEM)/F-12 medium with 10% fetal calf serum (FCS) and treated with luteolin, naringenin or quercetin (Sigma) at different concentration for 24 h in DMEM/F-12 medium with 1% FCS. Cell viability was determined using an MTT assay according to our previous description.Citation20
Quantitative real-time polymerase chain reaction (qRT-PCR)
ARPE-19 cells were treated with H2O2, quercetin (10 µM), luteolin (10 µM), naringenin (10 µM), or quercetin (10 µM)+luteolin (10 µM)+naringenin (10 µM), or co-treated with H2O2 plus quercetin, naringenin, quercetin or luteolin + naringenin + quercetin in DMEM/F-12 medium with 1% FCS for 24 h. Total RNAs were extracted from the above cells using Trizol reagent (Sigma) following the manufacture’s guidance. cDNA synthesis and qRT-PCR were carried out using commercial kits (Thermo Fisher Scientific) according to the manufacture’s protocols. The primers of targeted genes had 100% amplification efficiency and are listed in Table S1 (Supplementary Data). The relative expression of target genes was calculated based on the 2–ΔΔCT formula.
Biochemical assays
Reactive oxygen species (ROS) in untreated and treated cells was measured using DCFH-DA (6-Carboxy-20,70- Dichlorofluorescin diacetate) following our previous description.Citation20 Catalase (CAT) activity and the levels of glutathione (GSH) and malondialdehyde (MDA) in untreated and treated cells were measured using, respectively, the OxiSelect Catalase Activity Assay Kit (Cell Biolabs, STA-341), the GSSG/GSH assay kit (Cell Biolabs, STA-312) and the TBARS assay kit (Cell Biolabs, STA330), following the manufacturer’s instructions. Secreted cytokines, IL-1β, IL-6, Il-8 and TNFα, from untreated and treated cells were measured using commercial kits (PeproTech) according to the manufacturer’s protocols.
Statistical analysis
Data was analyzed using one-way analysis of variance (PRISM graphpad 9, www.graphpad.com) and presented as mean ± SE (standard error). p < 0.05 is recognized as significance.
Results
Screening of effective Chinese medicine formulae
We screened effective Chinese medicine formulae for clinical treatment of AMD in CNKI, VIP, WangFang and PubChem databases. Inclusion criteria were: retrieval time was from the establishment of the database; formulae were complete and there were no missing documents; clinical studies had clear efficacy standards and reported in over 20 cases. Exclusion criteria were: duplicate publications; literature with incomplete data; non-clinical studies; review and systematic evaluation of literature; clinical studies with no clear efficacy criteria. According to the inclusion criteria, we sorted out the Chinese medicine formulae. After deleting the duplicates, we obtained 101 Chinese medicine formulae that contained 167 Chinese medicines (Supplementary Table S2). Of note, 10 Chinese medicines, including Poria Cocos(Schw.) Wolf, Radix Salviae, Atractylodes Macrocephala Koidz, Angelicae Sinensis Radix, Lycii Fructus, Hedysarum Multijugum Maxim, Chuanxiong Rhizoma, Licorice, Fructus Ligustri Lucidi, Rehmanniae Radix Praeparata, were used at a relatively high frequency, being listed in at least 20 formulae. We have therefore defined these 10 herbs as a new collective formula called “anti-AMD decoction.”
Screening of active ingredients of anti-AMD decoction and their targets
A total of 268 active ingredients of anti-AMD decoction satisfying OB ≥ 30% and DL ≥ 0.18 were obtained through the TCMSP database, including fifteen from Poria Cocos (Schw.) Wolf, sixty-five from Radix Salviae, seven from Atractylodes Macrocephala Koidz, two from Angelicae Sinensis Radix, forty-five from Lycii Fructus, twenty from Hedysarum Multijugum Maxim, seven from Chuanxiong Rhizoma, ninety-two from Licorice, thirteen from Fructus Ligustri Lucidi, and two from Rehmanniae Radix Praeparata (Supplementary Tables S3, S4). Among these, thirteen active ingredients were present in at least two of these Chinese medicines, while the quercetin was identified in four Chinese medicines of the anti-AMD decoction, suggesting that functional coordination in some related biological processes may exist in the Decoction (Supplementary Table S5). When searching the TCMSP database, a total of 282 targets were identified for the 268 active ingredients (Supplementary Table S6).
Prediction of potential targets of anti-AMD decoction for AMD
Through searching the GeneCards database, a total of 2160 AMD disease targets were obtained (Supplementary Table S7). The Venny 2.1 online mapping tool was used to obtain 129 common targets after the intersection of the 2160 disease targets and 258 identified active ingredients (Supplementary Figure S1). The results showed that the components of the anti-AMD decoction possibly acted cooperatively in treating AMD through multiple potential targets.
Subsequently, we used Cytoscape 3.7.2 to construct a visual anti-AMD decoction-potential active ingredients-potential targets-AMD network containing 306 nodes and 432 edges (Supplementary Figure S2). From the perspective of ingredients, there were four active ingredients with more than 10 targets; of these, quercetin, luteolin, naringenin and hederagenin interacted with, respectively, 78, 48, 21 and 12 target proteins. We then constructed the anti-AMD decoction-main active ingredients-potential targets-AMD network. Quercetin, luteolin, naringenin and hederagenin were entered into Cytoscape 3.7.2 as the main active ingredients of anti-AMD decoction for network analysis. We observed the phenomenon that some ingredients in anti-AMD decoction could act on multiple targets, while different ingredients could work together on the same target (). Based on these observations, we identified close correlations among the active ingredients in the anti-AMD decoction and AMD-associated disease targets, suggesting that a number of ingredients contained in the anti-AMD decoction might exhibit their therapeutic roles via multiple AMD-associated targets.
Figure 1. Network between the four ingredients of the anti-AMD decoction and AMD-related disease targets. The yellow diamonds represent the potential action targets, pink triangle represents the anti-AMD decoction, light blue triangle represents AMD, and other polygons of different colors represent the main active ingredients in the anti-AMD decoction.

Construction of anti-AMD Decoction-AMD PPI core network
The 129 identified potential targets (Supplementary Figure S1) were entered into the String database, while the protein interaction data were imported into Cytoscape 3.7.2 software to obtain the protein interaction network, consisting of 129 nodes and 2638 edges (). We filtered the information by calculating DC, BC, and CC. The median value of all target protein values was 35, and there were 67 target proteins greater than or equal to the median value. These 67 target proteins can be considered as being of significance in the construction of the network of major targets for anti-AMD decoction, which contained 67 nodes and 1598 edges (). Through further screening, with “DC ≥ median DC, BC ≥ median BC, CC ≥ median CC” as a condition, 29 potential proteins were obtained, including IL-1β, IL-6, TNF and VEGFA, which contribute to AMD pathogenesis ().
Figure 2. Anti-AMD decoction-AMD protein-protein interaction (PPI) core network obtained from STRING. The PPI network containing 129 nodes and 2638 edges (left). The areas highlighted in pink represent the candidate targets by the screening criteria of “DC ≥ median DC.” The PPI network for the candidate targets by the screening criteria of “DC ≥ median DC” contains 67 nodes and 1598 edges. The areas highlighted in yellow represent the candidate targets by the screening criteria of “DC ≥ median DC, BC ≥ median BC, and CC ≥ median CC.” Core-target PPI network by the screening criteria of “DC ≥ median DC, BC ≥ median BC, and CC ≥ median CC” contains 29 nodes and 400 edges. BC: Betweeness Centrality; CC: Closeness Centrality; DC: Degree Centrality (DC).
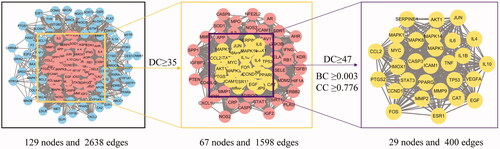
Molecular docking simulation
To further elucidate the molecular mechanism of traditional Chinese medicine against AMD, we performed molecular docking using the main active ingredients (quercetin, luteolin, naringenin and hederagenin) as ligands; based on the combination of the “DC,” “BC” and “CC” values calculated by Cytoscape 3.7.2 software, the predominant target proteins (IL-6, TNF, MAPK3 and VEGFA) had highest overall scores (data not shown), so we chose the four proteins as receptors (). A binding energy value of less than 0 kJ/mol indicates that the ligand can spontaneously bind to the receptor. The docking results showed that the binding energy between the key active ingredients and the core target was less than 0 kJ/mol. Among them, quercetin, luteolin and naringenin had similar binding energy to IL-6, TNF, MAPK3 and VEGFA, respectively (Supplementary Table S8); hederagenin had lower binding energy to the four target proteins, respectively (Supplementary Table S8). The data could suggest that anti-AMD Decoction has good affinity and binding activity for core ingredients and key targets.
Figure 3. Molecular docking analysis of the potential active ingredients of anti-AMD decoction and the core target proteins. (A) Molecular docking mode of quercetin with IL-6, TNF, MAPK3 and VEGFA. (B) Molecular docking mode of luteolin with IL-6, TNFα, MAPK3 and VEGFA. (C) Molecular docking mode of naringenin with IL-6, TNF, MAPK3 and VEGFA. (D) Molecular docking mode of hederagenin with IL-6, TNFα, MAPK3 and VEGFA. Active site amino acid residues are represented as tubes, while the active ingredients are shown as a stick model in green. The yellow dotted lines denote hydrogen bonds with the corresponding bond lengths displayed above. The sticks represent interacting ligands and amino acid residues, with the proteins displayed as secondary structures.
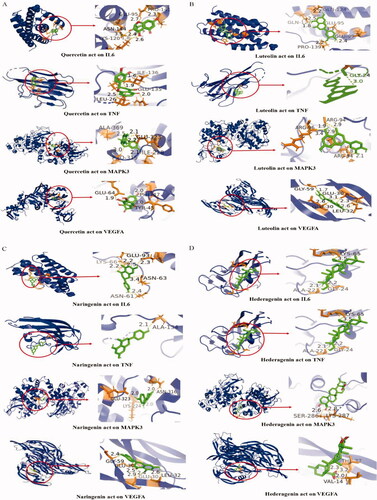
Active ingredients increased anti-oxidative capacity and inhibited inflammation in human RPE cells
We then performed biochemical experiments to verify the bioinformatical findings. We treated human RPE cells (ARPE-19) with quercetin, luteolin or naringenin at concentrations of 0, 10, 20 and 40 µM and found that quercetin significantly decreased cell viability at 20 or 40 µM when compared to the control; however, luteolin or naringenin had no effect on cell viability at 10, 20 or 40 µM (Supplementary Figure S3). Consequently, we used a concentration of 10 µM for the three chemicals in subsequent experiments.
Based on our previous studies,Citation20,Citation21 we treated ARPE-19 cells with H2O2 at 750 µM to induce oxidative stress and inflammation. Consistent with our previous publication, H2O2 treatment markedly increased ROS production, MDA level and expression of proinflammatory cytokines, while decreased catalase activity, GSH level and expression of antioxidant genes (). Co-treatment with quercetin and H2O2 resulted in 67% decrease in ROS generation (p < 0.01), 57% increase in catalase activity (p < 0.05), 19% increase in GSH level (p < 0.05) and 40% decrease in MDA level (p < 0.01) (), compared to cells treated with H2O2 alone; co-treatment with quercetin also increased expression of antioxidant genes—catalase by 138% (p < 0.0001), SOD1 by 96% (p < 0.0001), SOD2 by 53% (p < 0.0001) and GPX1 by 65% (p < 0.0001)—compared to cells exposed to H2O2 alone (). We also examined the effect of quercetin on production of proinflammatory cytokines and found that co-treatment with quercetin decreased expression of cytokines—IL-1β by 32% (protein, p < 0.0001) and 31% (mRNA, p < 0.0001), IL-6 by 52% (protein, p < 0.0001) and by 24% (mRNA, p < 0.0001), IL-8 by 48% (protein, p < 0.0001) and by 27% (mRNA, p < 0.0001), and TNFα by 31% (protein, p < 0.0001) and by 26% (mRNA, p < 0.0001), compared to cells exposed to H2O2 alone (). Similarly, co-treatment with luteolin or naringenin significantly reduced ROS generation, increased catalase activity and GSH level, decreased MDA level, enhanced antioxidant gene expression (mRNA), and inhibited expression of proinflammatory cytokines (both protein and mRNA), compared to cells treated with H2O2 alone ( and ). We also found that co-treatment with a mixture of quercetin, luteolin and naringenin counteracted H2O2-induced effects (). Cells treated with quercetin, luteolin, naringenin or the mixture of these three ingredients demonstrated similar levels of examined biomarkers as that of untreated cells ().
Figure 4. Effects of quercetin (QUE) on oxidative stress and inflammation in the RPE cells. (A) Quercetin counteracted H2O2-induced effects on ROS production, catalase (CAT) activity, GSH level and MDA level. (B) Expression of antioxidant genes was significantly higher in cells treated with quercetin and H2O2 than in cells treated with H2O2 alone. (C) Effect of quercetin on generation of proinflammatory cytokines in H2O2-treated cells. (D) Quercetin regulated expression of proinflammatory cytokine genes in H2O2-treated cells. UNT, untreated cells. NS, no significance; *p < 0.05, **p < 0.01, ***p < 0.001 and ****p < 0.0001.
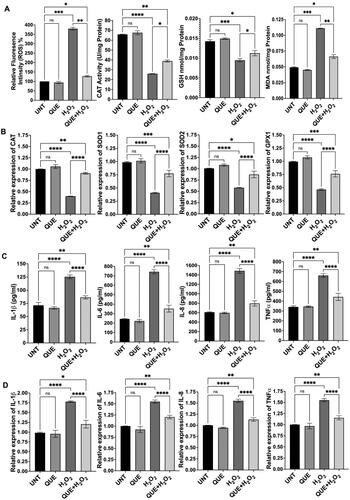
Figure 5. Effects of luteolin (LUT) on oxidative stress and inflammation in the RPE cells. (A) Luteolin counteracted H2O2-induced effects on ROS production, catalase (CAT) activity, GSH level and MDA level. (B) Expression of antioxidant genes was significantly higher in cells treated with luteolin and H2O2 than in cells treated with H2O2 alone. (C) Effect of luteolin on generation of proinflammatory cytokines in H2O2-treated cells. (D) Luteolin regulated expression of proinflammatory cytokine genes in H2O2-treated cells. UNT, untreated cells. NS, no significance; *p < 0.05, **p < 0.01, ***p < 0.001 and ****p < 0.0001.
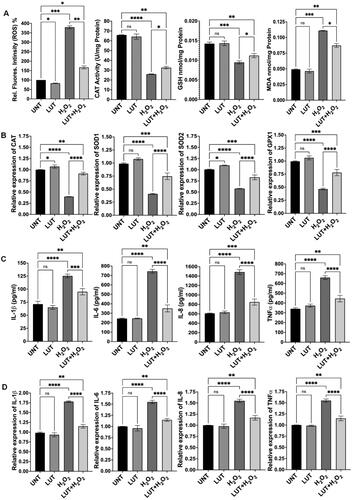
Figure 6. Effects of naringenin (NAR) on oxidative stress and inflammation in the RPE cells. (A) Naringenin counteracted H2O2-induced effects on ROS production, catalase (CAT) activity, GSH level and MDA level. (B) Expression of antioxidant genes was significantly higher in cells treated with naringenin and H2O2 than in cells treated with H2O2 alone. (C) Effect of naringenin on generation of proinflammatory cytokines in H2O2-treated cells. (D) Naringenin regulated expression of proinflammatory cytokine genes in H2O2-treated cells. UNT, untreated cells. NS, no significance; *p < 0.05, **p < 0.01, ***p < 0.001 and ****p < 0.0001.
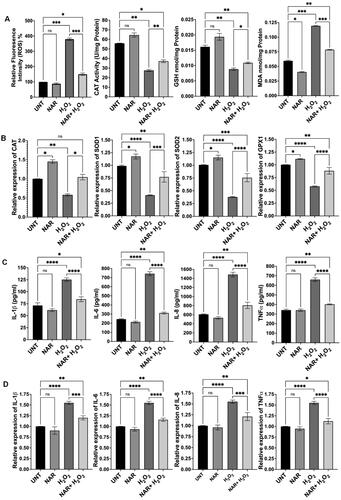
Figure 7. Effects of a mix of naringenin, luteolin and quercetin (NAR + LUT + QUE) on oxidative stress and inflammation of RPE cells. (A) NAR + LUT + QUE counteracted H2O2-induced effects on ROS production, catalase (CAT) activity, GSH level and MDA level. (B) Expression of antioxidant genes was significantly higher in cells treated with NAR + LUT + QUE and H2O2 than in cells treated with H2O2 alone. (C) Effect of NAR + LUT + QUE on generation of proinflammatory cytokines in H2O2-treated cells. (D) NAR + LUT + QUE regulated expression of proinflammatory cytokine genes in H2O2-treated cells. UNT, untreated cells. NS, no significance; *p < 0.05, **p < 0.01, ***p < 0.001 and ****p < 0.0001.
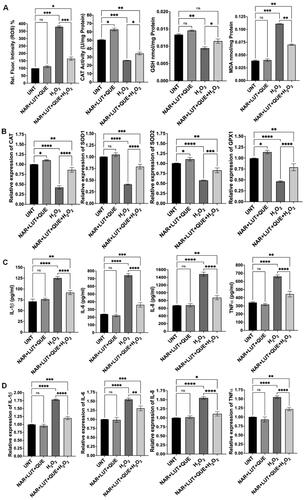
Discussion
The pathogenesis of AMD is complex, involving a number of interrelated aspects such as aging, oxidative stress and inflammatory response.Citation22 Currently, treatment targeting vascular endothelial growth factor (VEGF) can slow the progression of neovascular AMD (wet type, accounting for 10% of patients). However, there is no effective treatment for atrophic AMD (dry type, accounting for 90% of patients). TCM has been shown to have protective effects against both wet and dry AMD.Citation7 Compatibility of TCM usually involves a complex hybrid system of multiple ingredients and targets, affecting different biological processes in order to control symptoms and treat the underlying conditions.Citation23 The Chinese medicine in the anti-AMD decoction in the current study was composed of Poria Cocos (Schw.) Wolf, Radix Salviae, Atractylodes Macrocephala Koidz, Angelicae Sinensis Radix, Lycii Fructus, Hedysarum Multijugum Maxim, Chuanxiong Rhizoma, Licorice, Fructus Ligustri Lucidi, Rehmanniae Radix Praeparata. As indicated by database screening, these are the Chinese medicines with the highest frequency of use in clinical treatment of AMD. In terms of pharmacology, they have unique therapeutic efficacy against the complex pathogenesis of AMD. The complex composition of drugs in the anti-AMD Decoction and the nature of the interactions between the active ingredients are not yet clear. Network pharmacology has been used to study pathways of interaction between active ingredients and TCM mechanisms, and has shown itself to be capable of disentangling complexities in biological systems, in drugs and in diseases.Citation24,Citation25 Therefore, we used network pharmacology to investigate the potential targets involved in anti-AMD decoction treatment of AMD by constructing and analyzing target networks and so revealing the potential mechanisms.
The anti-AMD decoction-potential active ingredients-potential targets-AMD network was constructed using the 268 ingredients and 258 ingredient targets. The results suggest that most ingredients of anti-AMD decoction affected multiple targets. Although the number of putative targets in each Chinese medicine was different, the overlapping targets of different Chinese medicines were numerous. In other words, multiple ingredients of anti-AMD Decoction possibly had the same target, resulting in synergistic effects. For example, quercetin, luteolin, naringenin and hederagenin acted on 78, 48, 21 and 12 targets, respectively, and shared some of these targets. Therefore, these ingredients are likely to be the crucial pleiotropically active ingredients in the anti-AMD decoction. Further molecular docking studies confirmed the interaction between active ingredients and AMD-related potential target proteins at the molecular level, which determined the binding pose of the active ingredients to the target proteins and suggested that the active ingredients might have high binding affinity for AMD-related target proteins. The results showed that the combination of anti-AMD decoction and AMD target binding energy was negative for the four ingredients, indicating that there was binding activity between the ingredient and the target protein. Among these interactions, the most important node was quercetin, which had a high degree of centrality, closeness centrality, and betweenness centrality derived from network analysis, and also had a good affinity with potential target proteins in the molecular docking score.
Quercetin is one of the most significant bioflavonoid ingredients found in the current study. It has been shown to possess strong antioxidant, anti-inflammatory, anti-angiogenic, neuroprotective, and anti-apoptotic properties and demonstrates a significant overall protective effect.Citation26–28 A previous study showed that quercetin could alleviate apoptosis and inflammation via inhibition of NF-kB p65 translocation, C3 activation, and poly ADP-ribose polymerase (PARP) cleavage. It also activated the gene expression of aryl hydrocarbon receptor target genes (CYP1A1, CYP1B1) in tetrachlorodibenzo-p-dioxin (TCDD)-treated retinal pigment epithelium (RPE) cells.Citation29 Another study demonstrated a protective effect of quercetin against VEGF-induced inflammatory response.Citation30 Luteolin is another bioflavonoid ingredient that has a wide spectrum of biological activities, including anti-tumor, anti-bacterial and, in particular, anti-inflammatory effects.Citation31 It suppressed proinflammatory cytokine-induced RPE inflammation via inactivation of the NF-κβ pathway in Interleukin 1β (IL-1β)-stimulated ARPE-19 cells.Citation32 One study found that luteolin demonstrated a promising ability to protect RPE cells from oxidative stress-induced cell death and to potently downregulate inflammatory reactions in these cells by decreasing the activity of the transcription factor cAMP-response element binding protein (CREB) and the mitogen-activated protein kinases (MAPKs) p38 MAPK, c-Jun N-terminal kinase (JNK), and extracellular regulated protein kinases (ERK1/2).Citation33 These findings indicate the potential to use luteolin in the prevention and treatment of retinal inflammatory diseases. Naringenin had a protective effect against NaIO3-induced RPE degeneration in rat eyes, and also prevented laser-induced choroidal neovascularization (CNV) formation.Citation34 Furthermore, it has been demonstrated that the anti-oxidant and anti-apoptotic effects of naringenin may limit neurodegeneration by providing neurotrophic support to prevent retinal damage.Citation35 Here we also confirmed quercetin, luteolin, naringenin, or a combination of the three ingredients, alleviated H2O2-induced oxidative stress by decreasing ROS production and enhancing expression of antioxidant genes; these ingredients inhibited inflammation by decreasing the generation of proinflammatory cytokines, which agrees with the results of network and molecular docking analyses. Quercetin, luteolin and naringenin have been shown to inhibit stress-induced cellular senescence in cell lines and in disease animal models.Citation36–39 In particular, quercetin, as a senolytic drug, has been demonstrated to protect against cellular senescence in clinical trials.Citation40 It is well known that cellular senescence contributes to the pathogenesis of AMD.Citation41 Therefore, it is worthwhile to examine the function of quercetin, luteolin and naringenin against cellular senescence in human RPE (ARPE-19) cells and in AMD mouse models.
The current study screened out only the high-frequency core drugs for the clinical treatment of AMD from the database to form the anti-AMD decoction. In practise, clinical treatment of AMD with TCM is complicated, with Chinese medicines being added to or removed from the decoction based on the patient’s symptoms. Further experimentation and clinical verification are required for the mechanisms of anti-AMD decoction in the treatment of AMD, in order to more systematically and scientifically explain the its mechanisms of action and provide a robust theoretical basis for the clinical treatment of the condition.
Conclusion
Multi-ingredients in the anti-AMD decoction are proposed to have a synergistic and multiplicative effect on multiple AMD-associated disease targets, specifically relating to inflammation and oxidative damage. The active ingredients in the anti-AMD decoction are quercetin, luteolin, naringenin and hederagenin, with these ingredients showing potential for further development as new anti-AMD agents.
Author contributions
X.S. developed the concept. X.Y.L. searched the literatures and performed bioinformatical analyses. Y.C., G.M.T. and X.Z. carried out biochemical analyses. G.M.T. and X.S. analyzed the data. X.L. Z.H., J.R., Z.T. and X.S. drafted the manuscript.
Supplemental Material
Download PDF (3.4 MB)Disclosure statement
No potential conflict of interest was reported by the author(s).
Data availability statement
The authors confirm that the data supporting the findings of this study are available within the article and its supplementary materials.
Additional information
Funding
References
- Handa J, Bowes Rickman C, Dick A, Gorin M, Miller J, Toth C, Ueffing M, Zarbin M, Farrer L. A systems biology approach towards understanding and treating non-neovascular age-related macular degeneration. Nat Commun. 2019;10(1):3347. doi:10.1038/s41467-019-11262-1.
- Wong W, Su X, Li X, Cheung C, Klein R, Cheng C, Wong T. Global prevalence of age-related macular degeneration and disease burden projection for 2020 and 2040: a systematic review and meta-analysis. Lancet Glob Health. 2014;2(2):e106-116–e116. doi:10.1016/S2214-109X(13)70145-1.
- Fritsche L, Igl W, Bailey J, Grassmann F, Sengupta S, Bragg-Gresham J, Burdon K, Hebbring S, Wen C, Gorski M, et al. A large genome-wide association study of age-related macular degeneration highlights contributions of rare and common variants. Nat Genet. 2016;48(2):134–143. doi:10.1038/ng.3448.
- Heesterbeek T, Lorés-Motta L, Hoyng C, Lechanteur Y, den Hollander A. Risk factors for progression of age-related macular degeneration. Ophthalmic Physiol Opt. 2020;40(2):140–170. doi:10.1111/opo.12675.
- Zhang X, Alhasani RH, Zhou X, Reilly J, Zeng Z, Strang N, Shu X. Oxysterols and retinal degeneration. Br J Pharmacol. 2021;178(16):3205–3219. doi:10.1111/bph.15391.
- Wu J, Sun X. Complement system and age-related macular degeneration: drugs and challenges. Drug Des Devel Ther. 2019;13:2413–2425. doi:10.2147/DDDT.S206355.
- Li Y, Li X, Li X, Zeng Z, Strang N, Shu X, Tan Z. Non-neglectable therapeutic options for age-related macular degeneration: a promising perspective from traditional Chinese medicine. J Ethnopharmacol. 2022;282:114531. doi:10.1016/j.jep.2021.114531.
- Song Y, Wang H, Pan Y, Liu T. Investigating the multi-target pharmacological mechanism of Hedyotis diffusa willd acting on prostate cancer: a network pharmacology approach. Biomolecules. 2019;9(10):591. doi:10.3390/biom9100591.
- Zhang W, Tao Q, Guo Z, Fu Y, Chen X, Shar P, Shahen M, Zhu J, Xue J, Bai Y, et al. Systems pharmacology dissection of the integrated treatment for cardiovascular and gastrointestinal disorders by traditional chinese medicine. Sci Rep. 2016;6:32400. doi:10.1038/srep32400.
- Jiao X, Jin X, Ma Y, Yang Y, Li J, Liang L, Liu R, Li Z. A comprehensive application: molecular docking and network pharmacology for the prediction of bioactive constituents and elucidation of mechanisms of action in component-based Chinese medicine. Comput Biol Chem. 2021;90:107402. 2021;doi:10.1016/j.compbiolchem.2020.107402.
- Ru J, Li R, Wang J, Zhou W, Li B, Huang C, Li P, Guo Z, Tao W, Yang Y, et al. TCMSP: a database of systems pharmacology for drug discovery from herbal medicines. J Cheminform. 2014;6:13. doi:10.1186/1758-2946-6-13.
- Huang C, Yang Y, Chen X, Wang C, Li Y, Zheng C, Wang Y. Large-scale cross-species chemogenomic platform proposes a new drug discovery strategy of veterinary drug from herbal medicines. PLoS One. 2017;12(9):e0184880. doi:10.1371/journal.pone.0184880.
- Liu J, Li Y, Zhang Y, Huo M, Sun X, Xu Z, Tan N, Du K, Wang Y, Zhang J, et al. A network pharmacology approach to explore the mechanisms of Qishen Granules in heart failure. Med Sci Monit. 2019;25:7735–7745. doi:10.12659/MSM.919768.
- Guo X, Ji J, Feng Z, Hou X, Luo Y, Mei Z. A network pharmacology approach to explore the potential targets underlying the effect of sinomenine on rheumatoid arthritis. Int Immunopharmacol. 2020;80:106201. doi:10.1016/j.intimp.2020.106201.
- Franz M, Lopes C, Huck G, Dong Y, Sumer O, Bader G. Cytoscape.js: a graph theory library for visualisation and analysis. Bioinformatics. 2016;32(2):309–311. doi:10.1093/bioinformatics/btv557.
- Szklarczyk D, Morris J, Cook H, Kuhn M, Wyder S, Simonovic M, Santos A, Doncheva N, Roth A, Bork P, et al. The STRING database in 2017: quality-controlled protein-protein association networks, made broadly accessible. Nucleic Acids Res. 2017;45(D1):D362–D368. doi:10.1093/nar/gkw937.
- Szklarczyk D, Franceschini A, Wyder S, Forslund K, Heller D, Huerta-Cepas J, Simonovic M, Roth A, Santos A, Tsafou K, et al. STRING v10: protein-protein interaction networks, integrated over the tree of life. Nucleic Acids Res. 2015;43(Database issue):D447–D452. doi:10.1093/nar/gku1003.
- Goodsell D, Zardecki C, Di Costanzo L, Duarte J, Hudson B, Persikova I, Segura J, Shao C, Voigt M, Westbrook J, et al. RCSB Protein Data Bank: enabling biomedical research and drug discovery. Protein Sci. 2020;29(1):52–65. doi:10.1002/pro.3730.
- Sterling T, Irwin J. ZINC 15–Ligand discovery for everyone. J Chem Inf Model. 2015;55(11):2324–2337. doi:10.1021/acs.jcim.5b00559.
- Alhasani RH, Biswas L, Tohari AM, Zhou X, Reilly J, He JF, Shu X. Gypenosides protect retinal pigment epithelium cells from oxidative stress. Food Chem Toxicol. 2018;112:76–85. doi:10.1016/j.fct.2017.12.037.
- Tohari AM, Alhasani RH, Biswas L, Patnaik SR, Reilly J, Zeng Z, Shu X. Vitamin D attenuates oxidative damage and inflammation in retinal pigment epithelial cells. Antioxidants. 2019;8(9):341. doi:10.3390/antiox8090341.
- Datta S, Cano M, Ebrahimi K, Wang L, Handa J. The impact of oxidative stress and inflammation on RPE degeneration in non-neovascular AMD. Prog Retin Eye Res. 2017;60:201–218. doi:10.1016/j.preteyeres.2017.03.002.
- Li Y, Zhou H, Xie J, Hou AM, Xu Z, Zhang Y. A Novel method for evaluating the cardiotoxicity of traditional chinese medicine compatibility by using support vector machine model combined with metabonomics. Evid Based Complement Alternat Med. 2016;2016:6012761.
- Boezio B, Audouze K, Ducrot P, Taboureau O. Network-based approaches in pharmacology. Mol Inform. 2017;2017:36.
- Zhou W, Wang Y, Lu A, Zhang G. Systems pharmacology in small molecular drug discovery. Int J Mol Sci. 2016;17(2):246. doi:10.3390/ijms17020246.
- Anand David A, Arulmoli R, Parasuraman S. Overviews of biological importance of Quercetin: a bioactive flavonoid. Pharmacogn Rev. 2016;10(20):84–89. doi:10.4103/0973-7847.194044.
- Salvamani S, Gunasekaran B, Shaharuddin N, Ahmad S, Shukor M. Antiartherosclerotic effects of plant flavonoids. Biomed Res Int. 2014;2014:480258. doi:10.1155/2014/480258.
- Zhao Y, Chen B, Shen J, Wan L, Zhu Y, Yi T, Xiao Z. The beneficial effects of quercetin, curcumin, and resveratrol in obesity. Oxid Med Cell Longev. 2017;2017:1459497. doi:10.1155/2017/1459497.
- Kim J, Jin H, Jang D, Jeong K, Choung S. Quercetin-3-O-α-l-arabinopyranoside protects against retinal cell death via blue light-induced damage in human RPE cells and Balb-c mice. Food Funct. 2018;9(4):2171–2183. doi:10.1039/c7fo01958k.
- Lee M, Yun S, Lee H, Yang J. Quercetin mitigates inflammatory responses induced by vascular endothelial growth factor in mouse retinal photoreceptor cells through suppression of nuclear factor Kappa B. Int J Mol Sci. 2017;18(11):2497. doi:10.3390/ijms18112497.
- Boeing T, de Souza P, Speca S, Somensi L, Mariano L, Cury B, Ferreira Dos Anjos M, Quintão N, Dubuqoy L, Desreumax P, et al. Luteolin prevents irinotecan-induced intestinal mucositis in mice through antioxidant and anti-inflammatory properties. Br J Pharmacol. 2020;177(10):2393–2408. doi:10.1111/bph.14987.
- Hytti M, Szabó D, Piippo N, Korhonen E, Honkakoski P, Kaarniranta K, Petrovski G, Kauppinen A. Two dietary polyphenols, fisetin and luteolin, reduce inflammation but augment DNA damage-induced toxicity in human RPE cells. J Nutr Biochem. 2017;42:37–42. doi:10.1016/j.jnutbio.2016.12.014.
- Hytti M, Piippo N, Korhonen E, Honkakoski P, Kaarniranta K, Kauppinen A. Fisetin and luteolin protect human retinal pigment epithelial cells from oxidative stress-induced cell death and regulate inflammation. Sci Rep. 2015;5:17645. doi:10.1038/srep17645.
- Shen Y, Zhang W, Chiou G. Effect of naringenin on NaIO(3)-induced retinal pigment epithelium degeneration and laser-induced choroidal neovascularization in rats. Int J Ophthalmol. 2010;3:5–8.
- Gopinath B, Liew G, Kifley A, Lewis J, Bondonno C, Joachim N, Hodgson J, Mitchell P. Association of dietary nitrate intake with the 15-year incidence of age-related macular degeneration. J Acad Nutr Diet. 2018;118(12):2311–2314. doi:10.1016/j.jand.2018.07.012.
- Jiang YH, Jiang LY, Wang YC, Ma DF, Li X. Quercetin attenuates atherosclerosis via modulating oxidized LDL-induced endothelial cellular senescence. Front Pharmacol. 2020;11:512.
- Zoico E, Nori N, Darra E, Tebon M, Rizzatti V, Policastro G, De Caro A, Rossi AP, Fantin F, Zamboni M. Senolytic effects of quercetin in an in vitro model of pre-adipocytes and adipocytes induced senescence. Sci Rep. 2021;11(1):1–3. doi:10.1038/s41598-021-02544-0.
- Zhu RZ, Li BS, Gao SS, Seo JH, Choi BM. Luteolin inhibits H2O2-induced cellular senescence via modulation of SIRT1 and p53. Korean J Physiol Pharmacol. 2021;25(4):297–305. doi:10.4196/kjpp.2021.25.4.297.
- Da Pozzo E, Costa B, Cavallini C, Testai L, Martelli A, Calderone V, Martini C. The citrus flavanone naringenin protects myocardial cells against age-associated damage. Oxid Med Cell Longev. 2017;2017:9536148. doi:10.1155/2017/9536148.
- Gerdes EO, Misra A, Netto JM, Tchkonia T, Kirkland JL. Strategies for late phase preclinical and early clinical trials of senolytics. Mech Ageing Dev. 2021;200:111591. doi:10.1016/j.mad.2021.111591.
- Blasiak J. Senescence in the pathogenesis of age-related macular degeneration. Cell Mol Life Sci. 2020;77(5):789–805. doi:10.1007/s00018-019-03420-x.