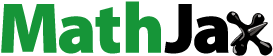
Abstract
Purpose
To improve the stability of the Corvis ST biomechanically-corrected intraocular pressure measurements (bIOP) after refractive surgery and its independence of corneal biomechanics.
Methods
A parametric study was carried out using numerical models simulating the behavior of the eye globe under the effects of IOP and Corvis ST external air pressure and used to develop a new algorithm for bIOP; bIOP(v2). It was tested on 528 healthy participants to evaluate correlations with CCT and age. Its ability to compensate for the geometrical changes was tested in 60 LASIK and 80 SMILE patients with six months follow up. The uncorrected Corvis ST IOP (CVS-IOP) and the two versions of biomechanically corrected IOP; bIOP(v1) and bIOP(v2), were compared.
Results
In the healthy dataset, bIOP(v2) had weak and non-significant correlation with both CCT (R = −0.048, p = .266) and age (R = 0.085, p = .052). For bIOP(v1), the correlation was non-significant with CCT (R = −0.064, p = .139) but significant with age (R = −0.124, p < .05). In both LASIK and SMILE groups, the median change in bIOP(v2) following surgery was below 1 mmHg at follow-up stages and the interquartile range was smaller than both bIOP(v1) and CVS-IOP.
Conclusion
The bIOP(v2) algorithm performs better than bIOP(v1) and CVS-IOP in terms of correlation with CCT and age. The bIOP(v2) also demonstrated the smallest variation after LASIK and SMILE refractive surgeries indicating improved ability to compensate for geometrical changes.
Introduction
Refractive surgeries are increasingly popular globally due to their high success rate and patient safety. Most common laser vision correction (LVC) procedures are Femtosecond laser-assisted in situ keratomileuses (LASIK), Small incision lenticule extraction (SMILE) and Transepithelial Photorefractive keratectomy (PRK). These surgeries affect corneal stiffness to different extents—while all three involve ablating tissue, only LASIK and SMILE also require tissue separation through the creation of a flap and a cap, respectively.Citation1 The flap and the cap also have different characteristics with the LASIK flap being almost completely separated from the residual stroma, and the SMILE cap maintaining a connection with the surrounding stroma except at the location of a short incision.Citation2 These differences in characteristics are expected to have different effects on corneal biomechanics in general, and corneal stiffness in particular.Citation3
Most intraocular pressure (IOP) measurement methods (or tonometry techniques), whether contact or non-contact, are based on a simple concept involving the application of a mechanical pressure to the cornea and relating the resulting deformation to the value of IOP. This concept is applied in contact methods including applanation tonometers (e.g. the Goladmann Applanation Tonometer, GAT),Citation4 and indentation methods such as the Schiotz tonometer.Citation5 It is also adopted in non-contact methods such as the Ocular Response Analyzer (ORA) and the Corvis ST tonometers where air pressure is used to deform the cornea.Citation6,Citation7 In all of these methods, a small deformation, or a high resistance to deformation, is considered an indication of a high IOP, and vice versa.
While this concept simplifies the design and development of tonometers, it ignores the inevitable effect of corneal stiffness on the IOP measurements. Undoubtedly, a cornea with a high stiffness—for instance due to a large thickness or a high material resistance—would experience overestimations of IOP, while a thin or soft cornea would cause IOP underestimations. In several studies using GAT, the reference standard in tonometry, there was a significant disagreement in quantifying the effect of central corneal thickness (CCT) on IOP measurements with estimates ranging between 2 and 7 mmHg per 100 µm variation in CCT.Citation8,Citation9
Glaucoma is a leading cause of irreversible blindness, associated with ganglion cell damage and resulting in a gradual loss of visual field.Citation10,Citation11 Its progression is commonly associated with intraocular pressure (IOP) elevation, and IOP reduction by pharmacological treatment, laser or surgical interventions is the main option available for management of the disease.Citation12,Citation13 Several IOP estimation techniques have been developed over the past 80 years, but the inaccuracies embedded in their operation can affect disease management and are thought to be at least partly responsible for visual acuity and visual field loss while under treatment.Citation14
In an earlier study, an attempt was made to develop biomechanically-corrected IOP (bIOP[v1]) estimates based on corneal deformation under an air puff produced by the Corvis ST (Oculus, Wetzlar, Germany), a non-contact tonometer integrated with an ultra-high-speed Scheimpflug camera that captures 4330 frames per second over an 8.5 mm wide single horizontal slit.Citation15 The bIOP(v1) was assessed in a number of later studies and was shown to be successful in reducing correlation with stiffness parameters, most notably CCT and age, as well as reducing the effect of the biomechanical change caused by refractive surgeries on IOP measurements.Citation16–18 In addition, it was shown to be reliable when compared to monometric IOP values.Citation19
However, despite the success of the bIOP(v1), there is still a need to reduce its dependence on corneal biomechanics further, especially given that the bIOP(v1) has become an integral component of other Corvis ST parameters such as the Stiffness Parameter (SP; strongly associated with overall corneal stiffness) and the Stress Strain Index (SSI; a measure of corneal material stiffness); it is also part of SP at Aplanation 1 in the Corvis Biomechanical Index (CBI; used in early diagnosis of keratoconus).Citation20–22 bIOP(v1) was developed based on numerical models of healthy corneas,Citation15 and despite its success, studies found slight correlation with corneal stiffness (which grows with age)Citation23 and its mean values were slightly lower in glaucoma patients when compared towith GAT, the reference standard.Citation24,Citation25 The bIOP(v1) measurements also underwent some reductions post-refractive surgeries indicating an influence of corneal stiffness changes.Citation26,Citation27 Furthermore, in our unpublished work, we identified small increases in bIOP(v1) after crosslinking procedures, which may have been caused by the associated increases in corneal stiffness. Also in post-refractive surgery the results were not consistent possibly due to biomechanical variations.Citation26,Citation27
For these reasons, this study seeks to optimize the bIOP(v1) algorithm, with the objective of improving its performance and independence of corneal biomechanics. The development of a new algorithm (bIOP[v2]) followed a similar route to that used for bIOP(v1), but adopted improved and more representative numerical modeling followed by clinical validation.
Methods
Numerical modeling
The present study relied on numerical models simulating the behavior of the eye globe under the effects of IOP and external air pressure. The finite element software package ABAQUS 6.13 (Dassault Syst.mes Simulia Corp., Rhode Island, USA) was used to simulate the Corvis ST testing procedure on models of the human eye. The models included the eye’s outer tunic (cornea and sclera) and internal fluids (aqueous and vitreous), but excluded other components of the orbit. Following a mesh density study, each model was built with 800 15-noded continuum elements (C3D15H) connected by 3606 nodes and organized in one layer, 10 cornea rings and 10 sclera rings (see Supplemental Material - Figure 1).Citation28 The models were generated using a bespoke ocular model generator software developed in house.Citation29 The optic nerve head was omitted as its inclusion was found in the study to have a negligible effect on corneal deformation under both IOP and external air pressure.
The models adopted the geometric features of the ocular globe reported in earlier studies. The corneal shape factor was set at 0.82, the limbal radius at 5.85 mm and the scleral radius at 11.5 mm.Citation30–34 The peripheral corneal thickness (PCT) at the edge of the limbus was assumed to be 150 µm more than CCT, while the sclera equatorial thickness was set at 0.80 PCT and the posterior pole thickness at 1.20 PCT.Citation21,Citation35,Citation36
IOP was defined using a surface-based fluid cavity, the pressure of which can be controlled. The cavity was assumed to be filled with an incompressible fluid with a density of 1000 kg/m3 to represent the vitreous and aqueous humor.Citation37 In all models, rigid-body motion was prevented by restraining the equator nodes in the anterior-posterior direction, and the posterior pole in both the superior-inferior and temporal-nasal directions. The analysis then started with determination of the stress-free form (SFF) of each model that would exist under zero IOP. The SFF was determined using an iterative process outlined in an earlier study,Citation38 and this was followed by the application of IOP and then the Corvis ST air pressure. While IOP was applied as a uniformly-distributed internal cavity pressure, the spatial and temporal distribution of the Corvis ST pressure on the corneal surface followed the results of an earlier study that considered the fluid-structure interaction between the air pressure and the cornea (see Supplemental Material - Figure 2).Citation39
Based on the results of earlier experimental studies,Citation40,Citation41 the cornea was assumed to follow the stress-strain relationship presented in EquationEquation (1)(1)
(1) , with a gradual increase in tangent modulus with age:
(1)
(1)
On the other hand, the sclera was divided into three segments, each with its distinctive stress-strain relationshipsCitation42 (see Supplemental Material - Figure 1 and EquationEquation (2)(2)
(2) ):
(2)
(2)
Parametric study
A numerical parametric study was conducted to assess the effect of geometrical stiffness parameters (CCT, and central anterior corneal radius [R]), material stiffness (represented by age) and IOP on estimations of corneal deformation under the Corvis ST air pressure. The study considered variations in: CCT between 395 and 645 µm, in steps of 50 µm; R between 7.2 and 8.4 mm, in steps of 0.6 mm; age between 30 and 100 years, in steps of 10 years; and IOP between 10 and 35 mmHg, in steps of 5 mmHg. These ranges slightly exceeded the ranges reported in the literature or seen in ophthalmic practice.Citation21,Citation30–35
In addition, models were further modified to adapt realist in healthy and KC corneal geometries. Numerical models had a cone located at apex, and within 1 mm and 2 mm distance from apex. Cones had a height of 75microns and 150 microns and covered an area of 1 mm or 2 mm in radius. The position of the cone was also moved radially at steps of 22.5 degrees as it would influence corneal deformation under airpuff pressure. These parameters were obtained from a previous study where cone size and location was characterized.Citation43
The models were analyzed using the Abaqus nonlinear finite element solver—first under IOP then under external air-puff pressure. Corneal deformation across the whole anterior surface was recorded throughout all loading stages. From these data, deformation along the central horizontal line of the cornea within the middle 8.5 mm zone was used in later analysis to estimate values of the various dynamic corneal response (DCR) parameters commonly provided by the Corvis ST, including those listed in Supplemental Material - Table 1.
bIOP(v2) algorithm development
The process adopted to develop an algorithm for bIOP(v2) started, as explained in the flowchart in , with data collection from the output of numerical simulations, and determination of the values of the DCRs. These DCRs were selected for being those with high repeatability as found in an earlier study as well as being the base parameters used to develop newer DCRs such as SP or CBI.Citation44 A bespoke MATLAB code was developed to consider all possible combinations of these parameters in third-order polynomials, relating the parameters considered in each combination to the IOP estimations. The polynomial combinations included first-order, second-order and third-order parameters, and multiplications of first-order and second-order parameters. With the 11 base parameters considered, 484 combinations were explored.
An optimization process, based on the least-squares method, was adopted to select the parameter coefficients that could minimize the differences between the IOP estimations and the IOP values adopted in the numerical models. The polynomial with the smallest error in IOP estimations (given by the following objective function) was then adopted as the bIOP(v2) algorithm:
(3)
(3)
where
is the IOP value used in each specific numerical model,
is the corresponding IOP value obtained from the algorithm, and n is the total number of models.
Clinical data
The bIOP(v2) algorithm with the best possible performance (or smallest RMSE) was assessed using two clinical datasets of healthy corneas and corneas that have undergone refractive surgeries, respectively. In this exercise, the ability of the algorithm to reduce correlations with CCT and age (relative to bIOP[v1] and uncorrected Corvis ST readings) and maintain stability in IOP measurements after refractive surgery procedures is considered an indication of its success.
Dataset 1 of healthy participants
Database 1 included data obtained from 528 healthy participants enrolled at the Vincieye Clinic in Milan, Italy. The Institutional Review Board at the University of Liverpool ruled that approval was not needed for this record review study. However, ethical approval and participants’ informed and written consent for using the data in research had been secured before the data was collected, anonymized, and used in earlier studies.Citation45 The ethical standards set out in the 1964 Declaration of Helsinki, and its revision in 2000, were observed.
The mean age of the participants was 39.9 ± 16.8 years (7.0–91.0) and mean CCT 537 ± 33 µm (444–635). The gender data was not captured. All participants were free of any ophthalmic disease, and had a Belin/Ambrósio Enhanced Ectasia total deviation index (BAD-D), derived from the Pentacam (OCULUS Optikgeräte GmbH; Wetzlar, Germany), of <1.6 standard deviations (SD) from normative values in both eyes. Patients with previous ocular surgery or disease, myopia < −10 D, concurrent or previous glaucoma, hypotonic therapies or diabetes mellitus were excluded.
All patients were evaluated with a complete ophthalmic examination, including the Corvis ST and Pentacam. All Corvis ST exams were acquired by the same experienced technicians with good quality scores (QS) that enabled calculation of all Corvis DCRs. Moreover, a frame-by-frame analysis of the exams was performed by an independent masked examiner (RV) to ensure the quality of each acquisition. One eye per patient was randomly selected and included in the analysis to avoid the bias of the relationship between bilateral eyes that could influence the analysis results.
Dataset 2 of refractive surgery patients
The medical records of 140 patients submitted to bilateral refractive surgery using LASIK (60 patients) and SMILE (80 patients) between February 2017 and April 2018 at the Eye Hospital of Wenzhou Medical University were retrospectively evaluated. The data collection was approved by the Institutional Review Board of the Hospital. Informed consent was provided by all participants for the use of their data in research before the data were collected. LASIK participants’ mean (range) age was 25 ± 5.2 years (17–37), CCT was 555 ± 22 µm (511–592), and manifest spherical equivalent (MSE) treated was −5.4 ± 1.6 D (−9.5–−1.8), with an optical zone diameter of 6.6 ± 0.4 mm (5.9–7.5) and a maximum ablation depth of 90.4 ± 20.5 µm (35–122). SMILE participants’ mean (range) age was 26.3 ± 4.9 years (17–41), CCT was 557 ± 24 µm (506–635), and manifest spherical equivalent (MSE) treated was −5.3 ± 1.6 D (−8.8–−2.4), with an optical zone diameter of 6.6 ± 0.2 mm (6.0–6.9) and a maximum ablation depth of 104.5 ± 20.1 µm (63–145).
After the procedure, one drop of tobramycin/dexamethasone (Tobradex; Alcon, TX, USA) was instilled at the surgical site. A bandage contact lens (Acuvue Oasys; Johnson & Johnson, FL, USA) was placed on the cornea and kept for one day after FS-LASIK. Fluorometholone 0.1% (Flumetholon; Santen, Osaka, Japan) and topical levofloxacin 0.5% (Cravit; Santen, Osaka, Japan) were applied 4 times a day for 1 week. The fluorometholone dosage was then tapered each subsequent week until it was stopped 1 month after FS-LASIK and SMILE.
In all cases, the Pentacam was used at 1, 3 and 6 month post-surgery to measure corneal anterior and posterior topography. At the same follow-up points, the Corvis ST was used to provide estimates of uncorrected and corrected estimates of IOP (CVS-IOP and bIOP[v1]). As for Dataset 1, the same exclusion criteria were applied, one eye per patient was randomly selected and included in the study. With this dataset, the success of bIOP(v2) was evaluated by the stability in its IOP estimations after the SMILE and LASIK procedures.
Statistical analysis
To analyze the results of the clinical validations, one sample Shapiro-Wilk test was used to check the normality of distribution of the continuous variables. For the healthy cases in Dataset 1, due to the normal distribution of the variables, correlations between IOP estimates and both age and CCT were assessed with Pearson product-moment correlation coefficient. For the refractive surgery data, as normality could not be verified, comparisons between the bIOP(v1), bIOP(v2) and uncorrected Corvis ST IOP (denoted as CVS-IOP) estimates in different postoperative periods were carried out using the Friedman’s test; post hoc pairwise comparisons were made using Nemenyi’s test. Wilcoxon Signed Rank Test was used to compare the pre- and post-operative data in each group. The differences between each postoperative period and the preoperative values were expressed by boxplots, in which the main box contains the median difference and the interquartile range (IQR). Analyses were accomplished using R Core Team (2016), a language and environment for statistical computing (R Foundation for Statistical Computing, Vienna, Austria; https://www.R-project.org/). A p value below .05 was considered statistically significant.
Results
bIOP(v2) algorithm
The polynomial with the best performance had an RMS of 0.3 mmHg and included third-order combinations of the parameters: CCT, AP1, PD, HCR, DeflAmpMax, A1V, DeflAmpA1 and HCT.
Clinical validation—dataset 1
The 528 healthy participants of Dataset 1 had mean IOP values obtained from CVS-IOP, bIOP(v1) and bIOP(v2) were 15.0 ± 2.7 mmHg (6.0–29.0), 14.4 ± 2.3 mmHg (9.1–23.9) and 15.4 ± 2.2 mmHg (10.1–27.8), respectively. There was no significant correlation between bIOP(v2) and either CCT (R = −0.048, p = .266) or age (R = 0.085, p = .052). With bIOP(v1), there was no significant correlation with CCT (R = −0.064, p = .139) but the correlation with age did reach statistical significant (R = −0.124, p < .05) CVS-IOP was significantly correlated with both CCT (R = 0.345, p < .05) and age (R = 0.111, p < .05); see .
Clinical validation—dataset 2
Smile
Comparisons of the preoperative values of the three IOP estimates showed statistically significant differences between the three groups (p < .05) with bIOP(v2) being slightly higher than both bIOP(v1) (14.7 ± 1.7 vs 13.8 ± 1.7 mmHg) and CVS-IOP (14.7 ± 1.7 vs 14.1 ± 1.9 mmHg). On the other hand, post-hoc comparisons showed both CVS-IOP and bIOP(v1) were significantly lower than bIOP(v2) (p < .05). These results are summarized in . The follow-up analysis revealed that the highest reductions occurred in CVS-IOP estimates at all postoperative periods, while significantly less reductions were observed in bIOP(v1) (p < .05) and the smallest variations were seen in bIOP(v2) (p < .05). As observed in , the median reduction in bIOP(v2) was below 1 mmHg at all postoperative periods and the interquartile range was smaller than bIOP(v1) and CVS-IOP.
Figure 3. Box plot of differences between postoperative and preopertative IOP measurements in the SMILE group (box: interquartile range, bar: median).
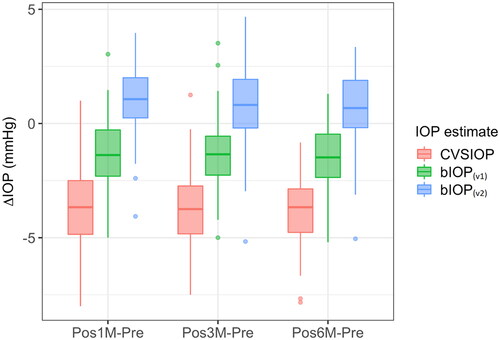
Table 1. Values of CVS-IOP, bIOP(v1), and bIOP(v2) obtained before and 1, 3, and 6 months after SMILE surgery.
Lasik
As with the SMILE group, statistically significant differences were observed between the values of the CVS-IOP, bIOP(v1) and bIOP(v2) estimates obtained before LASIK (p < .05). At this stage, bIOP(v2) was slightly higher than both bIOP(v1) (14.7 ± 1.5 vs 13.8 ± 1.7 mmHg) and CVS-IOP (14.7 ± 1.5 vs 14.0 ± 1.9 mmHg). On the other hand, post-hoc comparisons showed both CVS-IOP and bIOP(v1) were significantly lower than bIOP(v2) (p < .05; ). Follow-up analysis showed that the highest reductions were in CVS-IOP at all postoperative stages, while significantly less reductions were observed in bIOP(v1) (p < .05) and the smallest variations were seen in bIOP(v2) (p < .05). The median reduction in bIOP(v2) was below 1 mmHg at all follow-up stages and the interquartile range was smaller than bIOP(v1) and CVS-IOP ().
Figure 4. Box plot of differences between postoperative and preopertative IOP measurements in the LASIK group (box: interquartile range), bar: median).
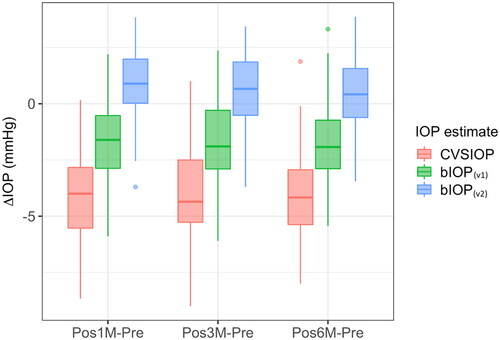
Table 2. Values of CVS-IOP, bIOP(v1), and bIOP(v2) obtained before and 1, 3, and 6 months after LASIK surgery.
Discussion
Patients who undergo refractive surgery were found to have lower IOP by GAT and other tonometers, and the reduction in IOP measurement was correlated to the amount of visual correction and the associated losses in corneal thickness.Citation26,Citation46 This is due to changes in corneal geometry and material stiffness. These findings are significant since IOP plays an important role in the management of glaucoma, and it has been identified as the only modifiable risk factor of the disease.Citation47,Citation48 Current measurement techniques, contact or non-contact, are based on applying a mechanical force on the cornea and relating its resistance to deformation to the value of IOP. However, since the deformation is also affected by corneal biomechanics, which in turn are dominated by corneal thickness and material stiffness, inaccuracies are often present in IOP estimates.Citation28
Attempts have been made in the past to provide more accurate estimations of IOP. Among these attempts, devices such as the Dynamic Contour Tonometer (DCT),Citation49 the Ocular Response Analyzer (ORA)Citation50 and the Corvis STCitation51 have been successful to varying degrees. As GAT is still the reference standard, comparisons of the performance of these tonometers were often held against the GAT. In a study on 39 LASIK patients, GAT IOP was found to reduce significantly after the surgical procedure by 3.0 ± 1.9 mmHg, while corresponding changes in DCT IOP were insignificant at (−0.2 mmHg ± 1.5 mmHg).Citation52 In another comparative study, 39 patients who underwent LASIK had GAT reducing significantly postoperatively (3.0 ± 1.9 mmHg) while no significant changes were found in DCT (−0.2 mmHg ± 1.5 mmHg).Citation53 And other earlier studies on healthy eyes, the Corvis bIOP(v1) was not significantly correlated with changes in CCT and age.Citation15,Citation45
The bIOP(v1) was developed using numerical modeling of healthy corneas through parametric studies considering four main parameters; CCT, age, IOP and corneal anterior radius.Citation15 The resulting bIOP(v1) algorithm performed well, demonstrating reduced influence of the cornea’s geometric parameters, most notably CCT, and the tissue’s material stiffness, which changes with age, relative to other tonometry methodsCitation16,Citation23,Citation24,Citation54–57 (in one of studies, bIOP(v1) was significantly correlated with ageCitation23) Studies found that for every 100 µm change in CCT, bIOP(v1) changed by 0.6 mmHg,Citation58 0.5 mmHg,Citation23 0.7 mmHgCitation16 and 0.9 mmHg.Citation59 In terms of correlation with age, bIOP(v1) change for every 10 years was reported at 0.4 mmHgCitation23 and 0.3 mmHg;Citation59 in the other studies mentioned above, correlation with age was not reported. The bIOP(v1) also provided reasonably stable IOP estimates after refractive surgeries with differences between pre- and post-LASIK limited to 1.04 ± 1.46 mmHg,Citation27 1.7 ± 1.0 mmHg,Citation26 and 0.1 ± 2.1 mmHg.Citation18 Other studies showed differences in bIOP(v1) pre- vs post-SMILE of 2.5 ± 1.39 mmHg,Citation60 0.9 ± 1.7 mmHg,Citation26 and 0.8 ± 1.8 mmHg.Citation18 The differences in these studies’ results may have been caused by using populations with different sizes and racial origins.
Comparison of bIOP(v1) to GAT in patients with ocular hypertension and open-angle glaucoma in 122 eyes found that bIOP(v1) was less affected than GAT by corneal biomechanics.Citation61 Another study found bIOP(v1) to have good repeatability in healthy and keratoconic eyes, although the mean value in keratoconic eyes was lower than in the healthy group.Citation24,Citation25 In addition, another study found a good agreement between corrected GAT and bIOP,Citation23 but with a slight negative correlation was observed between bIOP and age. Lower values of bIOP(v1) than GAT in glaucoma patients was also reported.Citation23 In these patients, bIOP(v1) was lower than GAT by 5.1 ± 4.5 mmHg in those with ocular hypertension, by 2.4 ± 4.0 mmHg in groups with primary open-angle glaucoma and hypertension and by 0.8 ± 2.1 mmHg in patients with normal-tension glaucoma.
In our previous research, our strategy was to produce two distinctive algorithms for healthy and soft (keratoconic) corneas, respectively.Citation62 The possible confusion this may cause in clinical practice encouraged adoption of a different strategy where one bIOP algorithm was developed for all cases. For this reason, the numerical models used to develop the bIOP(v2) in this study considered a wide range of corneal geometries that covered both healthy and diseased cases. 864 rotationally symmetric models were used to represent healthy corneas, along with 6912 models representing KC corneas with no rotational symmetry (based on topography analysis of 309 KC corneasCitation43).
In addition to replacing both versions of IOP(v1), IOP(v2) was also intended to improve the independence of IOP measurements from corneal biomechanics, and maintain adequate stability after refractive surgery. In assessing bIOP(v2) in a clinical dataset involving 528 healthy participants, bIOP(v2) changed by −0.3 mmHg for every 100 microns change in CCT while bIOP(v1) changed by −0.4 mmHg and the uncorrected CVS-IOP changed by 2.8 mmHg. Further, bIOP(v2) changed by 0.1 mmHg for every 10 years of age compared with a −0.2 mmHg change in bIOP(v1).
Furthermore, the three IOP measurement techniques were compared in a dataset of patients who underwent SMILE and LASIK surgeries. As the procedures involved removal and separation of corneal tissue, and subsequent reduction in corneal stiffness, it was expected that IOP measurements that are non-corrected for this change in corneal biomechanics, such as the CVS-IOP, would undergo significant reductions in their values.Citation17 This expectation was confirmed by the measurements taken in this study before and after both SMILE and LASIK. In addition, the minimal reduction observed with bIOP(v1), especially after the initial 3 months of the postoperative period in which the steroids effect and stromal edema were typically resolved,Citation63 is an indication that this measurement was less influenced by the corneal alterations caused by the procedures; these results are in line with the findings of previous studies.Citation17,Citation18,Citation64 The present study also showed evidence of smaller post-preoperative IOP measurements with bIOP(v2) (p < .001) and lower variability (50% reduction in IQR) compared with bIOP(v1), suggesting that this new measure was less influenced by the biomechanical changes caused by SMILE and LASIK.
The present study had a number of limitations, which should be noted. The bIOP(v2) measurements could not be compared against corresponding readings by the Goldmann Applanation Tonometer (GAT, the reference standard in tonometry),Citation65 the Dynamic Contour Tonometer (DCT, known to produce IOP estimates that are less influenced by corneal stiffness than GAT)Citation49,Citation66 and the ORA (a non-contact tonometer that produces the cornea-corrected IOP, IOPcc)Citation50,Citation67 as these measurements were not available. Furthermore, the present study is considered the first step in validating the new IOP measure and further validation is required, and is being conducted, in populations with keratoconus, both before and after the cross-linking treatment, after photorefractive keratectomy (PRK) refractive surgery, and in glaucoma and ocular hypertension patients.
In summary, this study aimed to develop a method to reduce the biomechanical effects of the cornea on IOP measurements, and to validate the method in clinical data of healthy participants and of patients undergoing two forms of refractive surgery. The method led to a new algorithm for the biomechanically corrected IOP: bIOP(v2). This algorithm was shown in this study to have reduced dependence on the cornea’s thickness and age compared with the earlier bIOP(v1) algorithm, and to have better stability after SMILE and LASIK surgeries.
Author contributions
AEliasy carried out the study, performed the analysis and acquired the results. AEliasy and BL drafted the manuscript, critically analyzed the results and performed statistical analysis. BL, JW, AA and RV interpretated the data and supported the analysis. RV, PV and FB critically reviewed the manuscript, interpreted the data and contributed in the design of the work. AElsheikh designed and conceptualized the study, supervised the entire project, drafted the manuscript and interpreted the data. All authors have reviewed and approved the submitted version.
Supplemental Material
Download Zip (1.4 MB)Disclosure statement
AElsheikh, RV and PV are consultants for oculus.
Data availability statement
The data that support the findings of this study are available from the corresponding author, AEliasy, upon reasonable request.
Additional information
Funding
References
- Guo H, Hosseini-Moghaddam SM, Hodge W. Corneal biomechanical properties after SMILE versus FLEX, LASIK, LASEK, or PRK: a systematic review and meta-analysis. BMC Opht. 2019;19(1):1–20.
- Sekundo W, Kunert KS, Blum M. Small incision corneal refractive surgery using the small incision lenticule extraction (SMILE) procedure for the correction of myopia and myopic astigmatism: results of a 6 month prospective study. Br J Ophthalmol. 2011;95(3):335–339. doi:10.1136/bjo.2009.174284.
- Khamar P, Shetty R, Vaishnav R, Francis M, Nuijts R, Sinha Roy A. Biomechanics of LASIK flap and SMILE cap: a prospective, clinical study. J Refract Surg. 2019;35(5):324–332. doi:10.3928/1081597X-20190319-01.
- Goldmann H, Schmidt T. [Applanation tonometry]. Ophthalmologica. 1957;134(4):221–242. doi:10.1159/000303213.
- Stamper RL. A history of intraocular pressure and its measurement. Optom Vis Sci. 2011;88(1):E16–E28. doi:10.1097/OPX.0b013e318205a4e7.
- Luce DA. Determining in vivo biomechanical properties of the cornea with an ocular response analyzer. J Cataract Refract Surg. 2005;31(1):156–162. doi:10.1016/j.jcrs.2004.10.044.
- Ambrósio R, Jr Ramos I, Luz A, Faria FC, Steinmueller A, Krug M, Belin MW, Roberts CJ. Dynamic ultra high speed Scheimpflug imaging for assessing corneal biomechanical properties. Rev Brasoftalmol. 2013;72(2):99–102. doi:10.1590/S0034-72802013000200005.
- Realini T, Weinreb RN, Hobbs G. Correlation of intraocular pressure measured with Goldmann and dynamic contour tonometry in normal and glaucomatous eyes. J Glaucoma. 2009;18(2):119–123. doi:10.1097/IJG.0b013e31817d23c7.
- Francis BA, Hsieh A, Lai MY, Chopra V, Pena F, Azen S, Varma R, Los Angeles Latino Eye Study Group. Effects of corneal thickness, corneal curvature, and intraocular pressure level on Goldmann applanation tonometry and dynamic contour tonometry. Ophthalmology. 2007;114(1):20–26. doi:10.1016/j.ophtha.2006.06.047.
- Quigley HA, Broman AT. The number of people with glaucoma worldwide in 2010 and 2020. Br J Ophthalmol. 2006;90(3):262–267. doi:10.1136/bjo.2005.081224.
- Ogden RW. Non-linear elastic deformations. 1997.
- The AGIS Investigators. The Advanced Glaucoma Intervention Study (AGIS): 7. The relationship between control of intraocular pressure and visual field deterioration. Am J Ophthalmol. 2000;130(4):429–440.
- Burr J, Azuara-Blanco A, Avenell A, Tuulonen A. Medical versus surgical interventions for open angle glaucoma. Coch Data Syst Rev. 2012;(9). doi:10.1002/14651858.CD004399.pub3/full.
- Group C-T. Comparison of glaucomatous progression between untreated patients with normal-tension glaucoma and patients with therapeutically reduced intraocular pressures. Am J Ophthalmol. 1998;126(4):487–497.
- Joda AA, Shervin MM, Kook D, Elsheikh A. Development and validation of a correction equation for Corvis tonometry. Comput Methods Biomech Biomed Engin. 2016;19(9):943–953. doi:10.1080/10255842.2015.1077515.
- Matsuura M, Murata H, Fujino Y, Yanagisawa M, Nakao Y, Tokumo K, Nakakura S, Kiuchi Y, Asaoka R. Relationship between novel intraocular pressure measurement from Corvis ST and central corneal thickness and corneal hysteresis. Br J Ophthalmol. 2020;104(4):563–568. doi:10.1136/bjophthalmol-2019-314370.
- Lee H, Roberts CJ, Kim TI, Ambrosio R Jr, Elsheikh A, Yong Kang DS. Changes in biomechanically corrected intraocular pressure and dynamic corneal response parameters before and after transepithelial photorefractive keratectomy and femtosecond laser-assisted laser in situ keratomileusis. J Cataract Refract Surg. 2017;43(12):1495–1503. doi:10.1016/j.jcrs.2017.08.019.
- Chen K-J, Joda A, Vinciguerra R, Eliasy A, Sefat SMM, Kook D, Geraghty B, Roberts CJ, Elsheikh A. Clinical evaluation of a new correction algorithm for dynamic Scheimpflug analyzer tonometry before and after laser in situ keratomileusis and small-incision lenticule extraction. J Cataract Refract Surg. 2018;44(5):581–588. doi:10.1016/j.jcrs.2018.01.023.
- Eliasy A, Chen K-J, Vinciguerra R, Maklad O, Vinciguerra P, Ambrósio R Jr, Roberts CJ, Elsheikh A. Ex-vivo experimental validation of biomechanically-corrected intraocular pressure measurements on human eyes using the CorVis ST. Exp Eye Res. 2018;175:98–102. doi:10.1016/j.exer.2018.06.013.
- Roberts CJ, Mahmoud AM, Bons JP, Hossain A, Elsheikh A, Vinciguerra R, Vinciguerra P, Ambrosio R Jr. Introduction of two novel stiffness parameters and interpretation of air puff-induced biomechanical deformation parameters with a dynamic Scheimpflug analyzer. J Refract Surg. 2017;33(4):266–273. doi:10.3928/1081597X-20161221-03.
- Eliasy A, Chen K-J, Vinciguerra R, Lopes BT, Abass A, Vinciguerra P, Ambrósio R Jr, Roberts CJ, Elsheikh A. Determination of corneal biomechanical behavior in-vivo for healthy eyes using CorVis ST tonometry: stress-strain index. Front Bioeng Biotechnol. 2019;7:105.
- Vinciguerra R, Ambrosio R Jr, Elsheikh A, Roberts CJ, Lopes B, Morenghi E, Azzolini C, Vinciguerra P. Detection of keratoconus with a new biomechanical index. J Refract Surg. 2016;32(12):803–810. doi:10.3928/1081597X-20160629-01.
- Ramm L, Herber R, Spoerl E, Raiskup F, Pillunat LE, Terai N. Intraocular pressure measurement using ocular response analyzer, dynamic contour tonometer, and Scheimpflug analyzer Corvis ST. J Ophthalmol. 2019;2019:1–9. doi:10.1155/2019/3879651.
- Yang K, Xu L, Fan Q, Zhao D, Ren S. Repeatability and comparison of new Corvis ST parameters in normal and keratoconus eyes. Sci Rep. 2019;9(1):1–10.
- Herber R, Vinciguerra R, Lopes B, Raiskup F, Pillunat LE, Vinciguerra P, Ambrósio R. Jr Repeatability and reproducibility of corneal deformation response parameters of dynamic ultra-high-speed Scheimpflug imaging in keratoconus. J Cat Ref Sur. 2020;46(1):86–94.
- Cao K, Liu L, Yu T, Chen F, Bai J, Liu T. Changes in corneal biomechanics during small-incision lenticule extraction (SMILE) and femtosecond-assisted laser in situ keratomileusis (FS-LASIK). Lasers Med Sci. 2020;35(3):599–609. doi:10.1007/s10103-019-02854-w.
- Bao F, Huang W, Zhu R, Lu N, Wang Y, Li H, Wu S, Lin H, Wang J, Zheng X, et al. Effectiveness of the Goldmann Applanation Tonometer, the Dynamic Contour Tonometer, the Ocular Response Analyzer and the Corvis ST in measuring intraocular pressure following FS-LASIK. Curr Eye Res. 2020;45(2):144–152. doi:10.1080/02713683.2019.1660794.
- Eliasy A. In vivo measurement of corneal stiffness and intraocular pressure to enable personalised disease management and treatment [Doctoral thesis]. 2020.
- Wang J. Numerical simulation of corneal refractive surgery based on improved reconstruction of corneal surface: University of Liverpool; [Doctoral thesis]. 2015.
- Ambrósio R Jr, Alonso RS, Luz A, Velarde LGC. Corneal-thickness spatial profile and corneal-volume distribution: tomographic indices to detect keratoconus. J Cataract Refract Surg. 2006;32(11):1851–1859. doi:10.1016/j.jcrs.2006.06.025.
- Avitabile T, Marano F, Uva MG, Reibaldi A. Evaluation of central and peripheral corneal thickness with ultrasound biomicroscopy in normal and keratoconic eyes. Cornea. 1997;16(6):639–644.
- Gilani F, Cortese M, Ambrósio RR Jr, Lopes B, Ramos I, Harvey EM, Belin MW. Comprehensive anterior segment normal values generated by rotating Scheimpflug tomography. J Cataract Refract Surg. 2013;39(11):1707–1712. doi:10.1016/j.jcrs.2013.05.042.
- Belin MW, Khachikian SS. New devices and clinical implications for measuring corneal thickness. Clin Exp Ophthalmol. 2006;34(8):729–731. doi:10.1111/j.1442-9071.2006.01395.x.
- Dubbelman M, Weeber HA, Van Der Heijde RG, Völker‐Dieben HJ. Radius and asphericity of the posterior corneal surface determined by corrected Scheimpflug photography. Acta Ophthalmol Scand. 2002;80(4):379–383. doi:10.1034/j.1600-0420.2002.800406.x.
- Elsheikh A, Geraghty B, Alhasso D, Knappett J, Campanelli M, Rama P. Regional variation in the biomechanical properties of the human sclera. Exp Eye Res. 2010;90(5):624–633. doi:10.1016/j.exer.2010.02.010.
- Kotecha A, Elsheikh A, Roberts CR, Zhu H, Garway-Heath DF. Corneal thickness-and age-related biomechanical properties of the cornea measured with the ocular response analyzer. Invest Ophthalmol Vis Sci. 2006;47(12):5337–5347. doi:10.1167/iovs.06-0557.
- Villamarin A, Roy S, Hasballa R, Vardoulis O, Reymond P, Stergiopulos N. 3D simulation of the aqueous flow in the human eye. Med Eng Phys. 2012;34(10):1462–1470. doi:10.1016/j.medengphy.2012.02.007.
- Elsheikh A, Whitford C, Hamarashid R, Kassem W, Joda A, Buchler P. Stress free configuration of the human eye. Med Eng Phys. 2013;35(2):211–216. doi:10.1016/j.medengphy.2012.09.006.
- Maklad O, Eliasy A, Chen K-J, Theofilis V, Elsheikh A. Simulation of air puff tonometry test using arbitrary Lagrangian–Eulerian (ALE) deforming mesh for corneal material characterisation. IJERPH. 2019;17(1):54. doi:10.3390/ijerph17010054.
- Elsheikh A, Wang D, Brown M, Rama P, Campanelli M, Pye D. Assessment of corneal biomechanical properties and their variation with age. Curr Eye Res. 2007;32(1):11–19. doi:10.1080/02713680601077145.
- Elsheikh A, Geraghty B, Rama P, Campanelli M, Meek KM. Characterization of age-related variation in corneal biomechanical properties. J R Soc Interface. 2010;7(51):1475–1485. doi:10.1098/rsif.2010.0108.
- Elsheikh A, Geraghty D, Alhasso D, Rama P. Regional biomechanical behaviour of the human sclera and its variation with age. Inv Opht Vis Sci. 2009;50:E-Abstract 3948.
- Eliasy A, Abass A, Lopes BT, Vinciguerra R, Zhang H, Vinciguerra P, Ambrósio R, Roberts CJ, Elsheikh A. Characterization of cone size and centre in keratoconic corneas. J R Soc Interface. 2020;17(169):20200271. doi:10.1098/rsif.2020.0271.
- Lopes BT, Roberts CJ, Elsheikh A, Vinciguerra R, Vinciguerra P, Reisdorf S, Berger S, Koprowski R, Ambrósio R. Repeatability and reproducibility of intraocular pressure and dynamic corneal response parameters assessed by the Corvis ST. J Ophthalmol. 2017;2017:1–4. doi:10.1155/2017/8515742.
- Vinciguerra R, Elsheikh A, Roberts CJ, Ambrosio R Jr, Kang DS, Lopes BT, Morenghi E, Azzolini C, Vinciguerra P. Influence of pachymetry and intraocular pressure on dynamic corneal response parameters in healthy patients. J Refract Surg. 2016;32(8):550–561. doi:10.3928/1081597X-20160524-01.
- Chang DH, Stulting RD. Change in intraocular pressure measurements after LASIK: the effect of the refractive correction and the lamellar flap. Ophthalmology. 2005;112(6):1009–1016. doi:10.1016/j.ophtha.2004.12.033.
- Matlach J, Bender S, Konig J, Binder H, Pfeiffer N, Hoffmann EM. Investigation of intraocular pressure fluctuation as a risk factor of glaucoma progression. Clin Ophthalmol. 2019;13:9–16. doi:10.2147/OPTH.S186526.
- Pascolini D, Mariotti SP. Global estimates of visual impairment: 2010. Br J Ophthalmol. 2012;96(5):614–618. doi:10.1136/bjophthalmol-2011-300539.
- Kanngiesser HE, Kniestedt C, Robert YC. Dynamic contour tonometry: presentation of a new tonometer. J Glau. 2005;14(5):344–350. doi:10.1097/01.ijg.0000176936.16015.4e.
- Montard R, Kopito R, Touzeau O, Allouch C, Letaief I, Borderie V, Laroche L. Ocular response analyzer: feasibility study and correlation with normal eyes. J Fran D'opht. 2007;30(10):978–984. doi:10.1016/S0181-5512(07)79273-2.
- Hong J, Xu J, Wei A, Deng SX, Cui X, Yu X, Sun X. A new tonometer—the Corvis ST tonometer: clinical comparison with noncontact and Goldmann applanation tonometers. Invest Ophthalmol Vis Sci. 2013;54(1):659–665. doi:10.1167/iovs.12-10984.
- Choudhari NS, Jadhav V, George R, Vijaya L. Variability in the calibration error of the Goldmann applanation tonometer. J Glaucoma. 2011;20(8):492–496. doi:10.1097/IJG.0b013e3181f464b8.
- Buckley DH, Swikert M, Johnson RL. Friction, wear, and evaporation rates of various materials in vacuum to 10 − 7 mm Hg. ASLE Trans. 1962;5(1):8–23. doi:10.1080/05698196208972448.
- Ma J, Wang Y, Hao W, Jhanji V. Comparative analysis of biomechanically corrected intraocular pressure with corneal visualization Scheimpflug technology versus conventional noncontact intraocular pressure. Int Ophthalmol. 2020;40(1):117–124. doi:10.1007/s10792-019-01159-9.
- Fu D, Li M, Knorz MC, Wei S, Shang J, Zhou X. Intraocular pressure changes and corneal biomechanics after hyperopic small-incision lenticule extraction. BMC Ophth. 2020;20:1–6.
- Sedaghat M-R, Momeni-Moghaddam H, Yekta A, Elsheikh A, Khabazkhoob M, Ambrósio R Jr, Maddah N, Danesh Z. Biomechanically-corrected intraocular pressure compared to pressure measured with commonly used tonometers in normal subjects. Clin Optom. 2019;11:127–133. doi:10.2147/OPTO.S220776.
- Lee S-h, Moon J-i, Jung YH. Comparison of intraocular pressures measured by the Corvis ST and other tonometers in normal eyes. J Korean Ophthalmol Soc. 2019;60(12):1250–1256. doi:10.3341/jkos.2019.60.12.1250.
- Lee H, Roberts CJ, Ambrósio R, Elsheikh A, Kang DSY, Kim T-I. Effect of accelerated corneal crosslinking combined with transepithelial photorefractive keratectomy on dynamic corneal response parameters and biomechanically corrected intraocular pressure measured with a dynamic Scheimpflug analyzer in healthy myopic patients. J Cataract Refract Surg. 2017;43(7):937–945. doi:10.1016/j.jcrs.2017.04.036.
- Kenia VP, Kenia RV, Pirdankar OH. Age-related variation in corneal biomechanical parameters in healthy Indians. Indian J Ophthalmol. 2020;68(12):2921–2929. doi:10.4103/ijo.IJO_2127_19.
- Abd El-Fattah EA, El Dorghamy AA, Ghoneim AM, Saad HA. Comparison of corneal biomechanical changes after LASIK and F-SMILE with CorVis ST. Eur J Ophthalmol. 2021;31(4):1762–1770. doi:10.1177/1120672120945664.
- Ye Y, Yang Y, Fan Y, Lan M, Yu K, Yu M. Comparison of biomechanically corrected intraocular pressure obtained by Corvis ST and Goldmann applanation tonometry in patients with open-angle glaucoma and ocular hypertension. J Glau. 2019;28(10):922–928.
- Chen K-J, Eliasy A, Vinciguerra R, Abass A, Lopes BT, Vinciguerra P, Ambrósio R Jr, Roberts CJ, Elsheikh A. Development and validation of a new intraocular pressure estimate for patients with soft corneas. J Cataract Refract Surg. 2019;45(9):1316–1323. doi:10.1016/j.jcrs.2019.04.004.
- Dos Santos VA, Schmetterer L, Stegmann H, Pfister M, Messner A, Schmidinger G, Garhofer G, Werkmeister RM. CorneaNet: fast segmentation of cornea OCT scans of healthy and keratoconic eyes using deep learning. Biomed Opt Express. 2019;10(2):622–641. doi:10.1364/BOE.10.000622.
- Chen S, Lopes BT, Huang W, Zheng X, Wang J, Zhu R, Vinciguerra R, Li Y, Wang Q, Li H, et al. Effectiveness of four tonometers in measuring intraocular pressure following femtosecond laser-assisted LASIK, SMILE and transepithelial PRK. J Cat Ref Surg. 2020;46(7):967–974.
- Moses RA. The Goldmann applanation tonometer. Am J Ophthalmol. 1958;46(6):865–869. doi:10.1016/0002-9394(58)90998-X.
- Kotecha A, White E, Shewry J, Garway-Heath D. The relative effects of corneal thickness and age on Goldmann applanation tonometry and dynamic contour tonometry. Br J Ophthalmol. 2005;89(12):1572–1575. doi:10.1136/bjo.2005.075580.
- Goebels SC, Seitz B, Langenbucher A. Precision of ocular response analyzer. Curr Eye Res. 2012;37(8):689–693. doi:10.3109/02713683.2012.660592.