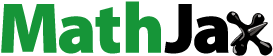
Abstract
Purpose
To evaluate corneal stiffening of porcine corneas induced by corneal crosslinking (CXL) with constant irradiance as a function of total fluence.
Methods
Ninety corneas from freshly enucleated porcine eyes were divided into five groups of 18 eyes. Groups 1–4 underwent epi-off CXL using a dextran-based riboflavin solution and an irradiance of 18 mW/cm2, group 5 served as the control group. Groups 1 to 4 were treated with a total fluence of 20, 15, 10.8, and 5.4 J/cm2, respectively. Thereafter, biomechanical measurements were performed on 5 mm wide and 6 mm long strips using an uniaxial material tester. Pachymetry measurements were performed on each cornea.
Results
At 10% strain, the stress was 76, 56, 52, and 31% higher in groups 1–4, respectively compared to the control group. The Young’s modulus was 2.85 MPa for group 1, 2.53 MPa for group 2, 2.46 MPa for group 3, 2.12 MPa for group 4, and 1.62 MPa for the control group. The difference between groups 1 to 4 and the control group 5 were statistically significant (p = <0.001; p = <0.001; p = <0.001; p = 0.021). In addition, group 1 showed significantly more stiffening than group 4 (p = <0.001), no other significant differences were found. Pachymetry measurements revealed no statistically significant differences among the five groups.
Conclusion
Additional mechanical stiffening can be achieved by increasing the fluence of the CXL. There was no threshold detected up to 20 J/cm2. A higher fluence could compensate the weaker effect of accelerated or epi-on CXL procedures.
Introduction
To date corneal crosslinking (CXL) is the only treatment that halts the progression of keratoconus by increasing the stiffness of the weakened corneal stroma.Citation1 Therefore, riboflavin is applied to the cornea and subsequently irradiated by UV-A light to induce new bonds within the corneal extracellular matrix.Citation2,Citation3 During this process, reactive oxygen species (ROS) are generated, which are crucial for the process, but also harmful to vulnerable keratocytes, corneal endothelial cells, and more posterior structures. Therefore, safety limits, such as minimal corneal thickness and total applied UV-energy were postulated when CXL was introduced. These limits have been valid for many years.Citation4,Citation5 Recent studies, however, showed that the riboflavin concentration at the endothelium level is substantially lower than anticipated in the initial safety calculations.Citation6 Accordingly, the traditional fluence limit of 5.4 J/cm2 has been violated in newer protocols.Citation7–9 Hence, thinner corneas have been treated by adjusting the fluence levels to the minimal thickness, newer epi-on treatments were evaluated,Citation10,Citation11 or customized energy levels inducing a stiffening gradient were used to improve corneal regularization.Citation9 The clinical results of CXL using higher fluence are promising, and to date, no endothelial decompensation has been reported for these protocols.
These studies have shown that higher CXL fluence is clinically safe, but the effect of higher fluence on corneal mechanical stiffness has been only poorly studied so far. To our knowledge, there are only two studies that measure CXL stiffening of CXL for various fluence levels up to 10.8 J/cm2.Citation12,Citation13
To determine the biomechanical response of CXL, uniaxial stress-strain measurements on porcine corneas are used by many groups in the past,Citation14,Citation15 therefore, allowing good comparability of results.
The aim of this study is to analyze the biomechanical stiffening after accelerated epi-off CXL, with a fluence of 5.4 up to 20 J/cm2 in porcine corneas using uniaxial extensiometry.
Methods
Corneal preparation and CXL procedure
Ninety freshly enucleated porcine eyes were collected from the local slaughterhouse. All eyes were examined for integrity and pachymetry (SP-100; Tomey Corporation) was performed to exclude damaged eyes. The eyes were randomly divided into five groups. All eyes were de-epithelialized with a hockey knife. A solution containing 0.1% riboflavin (riboflavin-5-monophosphate in 0.9% NaCl) dissolved in 16% dextran was applied on the corneal surface every 2 min for 30 min. Then, the eyes of group 1–4 were continuously irradiated with UVA-light (λ = 365 nm) (Mosaic, Avedro, Inc., Waltham, MA, USA) at an irradiance of 18 mW/cm2, applying a total fluence of 20, 15, 10.8, and 5.4 J/cm2 in group 1, 2, 3, and 4, respectively. Group five was not irradiated and served as a control group. After the treatment, corneal discs were excised and stored in a 16% dextran bath for 2 h to ensure uniform hydration conditions. Pachymetry was then measured again and 5 mm wide strips were excised along the vertical axis.
Stress–strain measurement
Corneal biomechanics was quantified using uniaxial tensile tests. The samples were mounted with two clamps on the testing device equipped with a 1 N load cell (UStrech Cellscale, Ontario, Canada). A preload of 5 × 103 Pa prestress was applied to each specimen for 2 min to ensure uniform tension of the tissue at the beginning of each measurement. The strain was applied at a rate of 0.035 mm/s, similar to previous biomechanical measurements.Citation16–18 The force on the sample was measured during the extension until a strain of 10%. Both force and displacement were recorded every 10 ms during the measurement. The distance between the two clamps was 6 mm at the beginning of the measurement, similar to previous experiments.Citation17,Citation18 The samples were immersed in a bath containing 16% dextran solution to maintain hydration during the measurement. The stress and strain were calculated at each measurement point, while the Young’s modulus was calculated at 6, 8, and 10% strain.
Numerical evaluation
Data was exported to Excel MS Software (Microsoft Corporation) where descriptive analysis was performed. The cross-section area of the samples required to determine the stress was calculated by multiplying the sample thickness measured by pachymetry by its width of 5 mm. The Young’s modulus (E) describing the relationship between stress (σ) and strain (ε) was calculated as:
Then, the mean of the Young’s modulus at 10% strain were compared using the unifactorial ANOVA using SPSS (version 26.0 IBM Corporation). Post-hoc Holm-Bonferroni correction for multiple comparisons was performed. Pachymetry of the five groups was analyzed using Kruskal–Wallis. A p-value of <.05 was considered statistically significant.
Results
Eighty-six (96%) of 90 eyes could be considered for the statistical evaluation. Four eyes of had to be excluded due to a failure of the software of the mechanical testing device during the measurement. Accordingly, 16 corneas from group 3, 17 from groups 2 and 4, and 18 from groups 1 and 5 were included in the data analysis.
The average stress of groups 1–5 at 6, 8, and 10% strain is shown in . The stress at 10% strain was increased by 76% in group 1, 56% in group 2, 52% in group 3, and by 31% in group 4 compared to control group 5. The linear regression analysis at a strain of 10% showed a high correlation between fluence and stress (R2 = 0.955; p = 0.004).
Table 1. Stress (kPa) at the strain of 6, 8, and 10%.
The Young’s modulus at 10% strain was calculated for the five groups and is shown in . The Young’s modulus was compared using post-hoc Bonferroni adjusted Analysis of Variance (ANOVA), resulting p values are shown in . Groups 1–4 showed a statistically significant stiffening compared with group 5 (p = <0.001; p = <0.001; p = <0.001; p = 0.021). In addition, group 1 showed significantly more stiffening than group 4 (p = <0.001), no other significant differences were found.
Figure 1. Young’s modulus at a strain of 10%. Group 1 = corneal crosslinking (CXL) with a fluence of 20 J/cm2, group 2 = CXL with a fluence of 15 J/cm2; group 3 = CXL with a fluence of 10.8 J/cm2; group 4 = CXL with a fluence of 5.4 J/cm2; group 5 = control group untreated corneas.
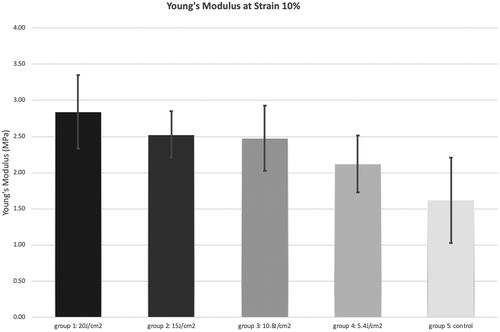
Table 2. p-Values resulting from post-hoc Bonferroni adjusted analysis of variance (ANOVA) between stress (kPa) at the strain of 10% after CXL with different fluences.
The average pachymetry before stress-strain measurement was 731 ± 57, 725 ± 59, 713 ± 52, 711 ± 62, and 741 ± 62 µm in groups 1–5, respectively. There was no statistically significant difference between the five groups (p = 0.538).
Discussion
The most important finding of this study is the significant dose-response relationship of the stiffening effect after epi-off CXL with increasing applied fluence from 5.4 to 20 J/cm2.
The first studies reporting the biomechanical response after CXL using the Dresden protocol with a total fluence of 5.4 J/cm2 showed an increased stress by a factor of 1.8 at a strain of 10%.Citation3 Previous studies by our group using similar experimental settings and the same mechanical tester showed a similar increased stress of up to 80% at a strain of 10% using an accelerated protocol (9 mW/cm2, 10 min).Citation16,Citation17 The current results of the accelerated treatment show a much smaller increase of stress of only 31% compared to the control group. However, in this study, the standard fluence of 5.4 J/cm2 was induced by an irradiance of 18 mW/cm2 for 5 min, whereas the irradiance of the Dresden protocol is only 3 mW/cm2. Similar findings of reduced stiffening in accelerated protocols were reported by our groupCitation17 and other authors.Citation15 Hammer et al. found an even smaller and non-significant (p = 0.065) stiffening of about 12% in their experiments using 18 mW/cm2 with a standard fluence of 5.4 J/cm2 too.Citation15 This effect can be explained by the hypothesis, that high irradiances are associated with a rapid depletion of oxygen in the anterior layers of the cornea, which limits oxygen availability in the posterior layers, and consequently leads to a more superficial crosslinking effect.Citation19,Citation20 This is consistent with the more superficial demarcation lines after CXL with high irradiance.Citation21
Although higher fluences than 5.4 up to 15 J/cm2 have been used clinically for several years,Citation10,Citation22 to our knowledge, there are only two recently published studiesCitation12,Citation13 reporting biomechanical measurements using fluences up to 10.8 J/cm2 for CXL. Biomechanical investigations for higher fluences do not exist to date but are relevant, as they are used clinically.
We found that doubling the fluence increased the stiffening effect from 31 to 53%, which is comparable to the 22 and 49% stiffening reported by Abrishamchi et al. using a similar setting to measure the corneal biomechanics. However, the CXL protocol used differed from our study as they used an irradiance of 9 mW/cm2 for 10 min to reach the fluence of 5.4 J/cm2 and an irradiation of 18 mW/cm2 for 9 min and 15 s, corresponding to a slightly smaller total fluence of 10 J/cm2 than in our study. The authors also did not specify which riboflavin solution was used in their experiments. The use of a HPMC based solution could explain the somewhat weaker stiffening effect, as dextran-based solutions which were used in our study are known to produce stronger stiffening.Citation16 Boschetti et al. reported more stiffening for higher fluences using inflation tests to measure the biomechanics, what makes a comparison of the results difficult.Citation13
A consequence of the data presented here is that stiffening can be increased by additional treatment time and fluences above 10.8 J/cm2, a significant difference was found between 5.4 and 20 J/cm2. A longer treatment allows more oxygen to diffuse in the cornea and therefore enables more CXL. Based on the present results, we cannot determine which factor has the greatest influence, the additional treatment time or energy. Clinical data of customized CXL report deeper demarcation lines in areas with higher applied fluence and treatment durationCitation9,Citation23 suggesting that some re-diffusion of oxygen in deeper layers, after an initial saturation of the anterior stroma, is responsible for this deepening of the demarcation line. This theory is supported by the slight increase in oxygen after 10 min during continuous UV-irradiation in a depth of 200 microns, found by Kamaev et al. in 2012.Citation24 However, only future oxygen measurements in combination with biomechanical tests can confirm this assumption and determine the upper threshold for induced crosslinks. An increased anaerobic CXLCitation24 might also serve as a potential explanation for the deeper demarcation line and the increased stiffening.
The classic Dresden protocol with a total fluence of 5.4 J/cm2 applies an uniform energy to the treated area of the cornea, stopping the progression of ectatic corneas with good outcomes and patients safety.Citation25,Citation26 Already a few years after the introduction of CXL, the procedure has been successfully accelerated by increasing the irradiance and varying the riboflavin solutions,Citation27–30 but the stiffening effect, as well as the regularization of the cornea, were not noticeably improved for a long time.Citation31,Citation32 But since the introduction of customized CXL, favorable results have been published regarding the regularization and flattening of the cornea.Citation9,Citation10 According to these protocols, weaker parts of the cornea are treated with a higher fluence to achieve a stronger flattening in these areas. The assumption, that higher fluences cause more stiffening is confirmed by our results.
Currently, the individual amount of the induced crosslinking that is required to stop the progression of an ectatic cornea is not known preoperatively, since the individual biomechanical state of the patient’s cornea cannot be assessed accurately clinically. Therefore, it might be inappropriate to apply more energy in every CXL treatment, as the success rates of current protocols are very high. In addition, clinicians have to be aware that in rare cases, there is ongoing flattening even years after CXL,Citation33 so not all patients will benefit from a very strong CXL.
Though the former fluence threshold of 5.4 J/cm2 is no longer valid and higher radiant exposures can be used,Citation6 fluences higher than 10.8 J/cm2 should be used reluctant, since little data about safety exists.Citation22 In thinner corneas higher fluences are not recommended at all, but a reduction should be considered.Citation11 Epi-on CXL, where the epithelium absorbs additional UV light and probably causes additional safety for the endothelium might be a field of application for higher fluences.
One limitation of this study is the use of porcine corneas. Because they are much more readily available and have similar dimensions to human corneas, porcine specimens are commonly used in experimental CXL studies, although previous studies have found a smaller CXL effect in porcine than in human corneas.Citation3 Nevertheless, it is believed that the results are transferable to human eyes. In our experiments, we used riboflavin dissolved in 16% dextran, as opposed to the 20% dextran used by clinicians and other ex vivo studies. The 16% solution was chosen to minimize corneal thinning which is reduced by using a lower concentration of dextran. The uniaxial stress-strain measurements could be identified as an additional disadvantage. Cutting the strips compromises the integrity of the cornea. In addition, the strips are pulled flat in the extensometer, so that the superficial fibers are stressed less than the deeper layers. However, many other studies have used a similar experimental design, so it is a standard protocol that allows the comparison of results with the existing literature.
In conclusion, the stiffening effect of the accelerated protocol based on 18 mW/cm2 with a standard fluence of 5.4 J/cm2 is lower than earlier reported for the standard CXL that relies on 3 mW/cm2. The biomechanical response after accelerated CXL can be increased by extending the treatment time causing a higher fluence. According to our results, there seems to be no threshold up to 20 J/cm2. A higher fluence could compensate the weaker effect of accelerated or epi-on CXL procedures.
Disclosure statement
Dr. Seiler is a scientific advisor for Avedro/Glaukos and receives material support for research projects. The other authors report no conflicts of interest.
Data availability statement
The data that support the findings of this study are available from the corresponding author, [IF], upon reasonable request.
References
- Seiler T, Spoerl E, Huhle M, Kamouna A. Conservative therapy of keratoconus by enhancement of collagen cross-links. Invest Ophthalmol Vis Sci. 1996;37:1017.
- Santhiago MR, Randleman JB. The biology of corneal cross-linking derived from ultraviolet light and riboflavin. Exp Eye Res. 2021;202:108355. doi:10.1016/j.exer.2020.108355.
- Wollensak G, Spoerl E, Seiler T. Stress-strain measurements of human and porcine corneas after riboflavin-ultraviolet-A-induced cross-linking. J Cataract Refract Surg. 2003;29(9):1780–1785. doi:10.1016/s0886-3350(03)00407-3.
- Wollensak G, Spörl E, Reber F, Pillunat L, Funk R. Corneal endothelial cytotoxicity of riboflavin/UVA treatment in vitro. Ophthalmic Res. 2003;35(6):324–328. doi:10.1159/000074071.
- Wollensak G, Spoerl E, Wilsch M, Seiler T. Endothelial cell damage after riboflavin-ultraviolet-A treatment in the rabbit. J Cataract Refract Surg. 2003;29(9):1786–1790. doi:10.1016/s0886-3350(03)00343-2.
- Seiler TG, Batista A, Frueh BE, Koenig K. Riboflavin concentrations at the endothelium during corneal cross-linking in humans. Invest Ophthalmol Vis Sci. 2019;60(6):2140–2145. doi:10.1167/iovs.19-26686.
- Lang PZ, Hafezi NL, Khandelwal SS, Torres-Netto EA, Hafezi F, Randleman JB. Comparative functional outcomes after corneal crosslinking using standard, accelerated, and accelerated with higher total fluence protocols. Cornea. 2019;38(4):433–441. doi:10.1097/ICO.0000000000001878.
- Shetty R, Pahuja N, Roshan T, Deshmukh R, Francis M, Ghosh A, Sinha Roy A. Customized corneal cross-linking using different UVA beam profiles. J Refract Surg. 2017;33(10):676–682. doi:10.3928/1081597X-20170621-07.
- Seiler TG, Fischinger I, Koller T, Zapp D, Frueh BE, Seiler T. Customized corneal cross-linking: one-year results. Am J Ophthalmol. 2016;166:14–21. doi:10.1016/j.ajo.2016.02.029.
- Mazzotta C, Sgheri A, Bagaglia SA, Rechichi M, Di Maggio A. Customized corneal crosslinking for treatment of progressive keratoconus: clinical and OCT outcomes using a transepithelial approach with supplemental oxygen. J Cataract Refract Surg. 2020;46(12):1582–1587. doi:10.1097/j.jcrs.0000000000000347.
- Hafezi F, Kling S, Gilardoni F, Hafezi N, Hillen M, Abrishamchi R, Gomes JAP, Mazzotta C, Randleman JB, Torres-Netto EA. Individualized corneal cross-linking with riboflavin and UV-A in ultrathin corneas: the Sub400 protocol. Am J Ophthalmol. 2021;224:133–142. doi:10.1016/j.ajo.2020.12.011.
- Abrishamchi R, Abdshahzadeh H, Hillen M, Hafezi N, Torres-Netto EA, Aslanides IM, Chen S, Randleman JB, Hafezi F. High-fluence accelerated epithelium-off corneal cross-linking protocol provides dresden protocol-like corneal strengthening. Transl Vis Sci Technol. 2021;10(5):10. doi:10.1167/tvst.10.5.10.
- Boschetti F, Conti D, Soriano EM, Mazzotta C, Pandolfi A. Experimental in-vitro investigation on epi-off-crosslinking on porcine corneas. PLOS One. 2021;16(4):e0249949. doi:10.1371/journal.pone.0249949.
- Spoerl E, Huhle M, Seiler T. Induction of cross-links in corneal tissue. Exp Eye Res. 1998;66(1):97–103. doi:10.1006/exer.1997.0410.
- Hammer A, Richoz O, Arba Mosquera S, Tabibian D, Hoogewoud F, Hafezi F. Corneal biomechanical properties at different corneal cross-linking (CXL) irradiances. Invest Ophthalmol Vis Sci. 2014;55(5):2881–2884. doi:10.1167/iovs.13-13748.
- Fischinger I, Seiler TG, Wendelstein J, Tetz K, Fuchs B, Bolz M. Biomechanical response after corneal crosslinking with riboflavin dissolved in dextran solution versus hydroxy propyl methyl cellulose. J Refract Surg. 2021;37(9):631–635. doi:10.3928/1081597X-20210610-04.
- Seiler TG, Fischinger I, Senfft T, Schmidinger G, Seiler T. Intrastromal application of riboflavin for corneal crosslinking. Invest Ophthalmol Vis Sci. 2014;55(7):4261–4265. doi:10.1167/iovs.14-14021.
- Lammer J, Laggner M, Pircher N, Fischinger I, Hofmann C, Schmidinger G. Endothelial safety and efficacy of ex-vivo collagen cross linking (CXL) of human corneal transplants. Am J Ophthalmol. 2020;214:127–133. doi:10.1016/j.ajo.2020.02.024.
- Seiler TG, Komninou MA, Nambiar MH, Schuerch K, Frueh BE, Buchler P. Oxygen kinetics during corneal cross-linking with and without supplementary oxygen. Am J Ophthalmol. 2021;223:368–376. doi:10.1016/j.ajo.2020.11.001.
- Richoz O, Hammer A, Tabibian D, Gatzioufas Z, Hafezi F. The biomechanical effect of corneal collagen cross-linking (CXL) with riboflavin and UV-A is oxygen dependent. Transl Vis Sci Technol. 2013;2(7):6. doi:10.1167/tvst.2.7.6.
- Brittingham S, Tappeiner C, Frueh BE. Corneal cross-linking in keratoconus using the standard and rapid treatment protocol: differences in demarcation line and 12-month outcomes. Invest Ophthalmol Vis Sci. 2014;55(12):8371–8376. doi:10.1167/iovs.14-15444.
- Sachdev GS, Ramamurthy S, B S, Dandapani R. Comparative analysis of safety and efficacy of topography-guided customized cross-linking and standard cross-linking in the treatment of progressive keratoconus. Cornea. 2021;40(2):188–193. doi:10.1097/ICO.0000000000002492.
- Cassagne M, Pierne K, Galiacy SD, Asfaux-Marfaing MP, Fournie P, Malecaze F. Customized topography-guided corneal collagen cross-linking for keratoconus. J Refract Surg. 2017;33(5):290–297. doi:10.3928/1081597X-20170201-02.
- Kamaev P, Friedman MD, Sherr E, Muller D. Photochemical kinetics of corneal cross-linking with riboflavin. Invest Ophthalmol Vis Sci. 2012;53(4):2360–2367. doi:10.1167/iovs.11-9385.
- Koller T, Mrochen M, Seiler T. Complication and failure rates after corneal crosslinking. J Cataract Refract Surg. 2009;35(8):1358–1362. doi:10.1016/j.jcrs.2009.03.035.
- Wollensak G, Spoerl E, Seiler T. Riboflavin/ultraviolet-A-induced collagen crosslinking for the treatment of keratoconus. Am J Ophthalmol. 2003;135(5):620–627. doi:10.1016/s0002-9394(02)02220-1.
- Wen D, Li Q, Song B, Tu R, Wang Q, O'Brart DPS, McAlinden C, Huang J. Comparison of standard versus accelerated corneal collagen cross-linking for keratoconus: a meta-analysis. Invest Ophthalmol Vis Sci. 2018;59(10):3920–3931. doi:10.1167/iovs.18-24656.
- Males JJ, Viswanathan D. Comparative study of long-term outcomes of accelerated and conventional collagen crosslinking for progressive keratoconus. Eye. 2018;32(1):32–38. doi:10.1038/eye.2017.296.
- Thorsrud A, Hagem AM, Sandvik GF, Drolsum L. Superior outcome of corneal collagen cross-linking using riboflavin with methylcellulose than riboflavin with dextran as the main supplement. Acta Ophthalmol. 2019;97(4):415–421. doi:10.1111/aos.13928.
- Lenk J, Herber R, Oswald C, Spoerl E, Pillunat LE, Raiskup F. Risk factors for progression of keratoconus and failure rate after corneal cross-linking. J Refract Surg. 2021;37(12):816–823. doi:10.3928/1081597X-20210830-01.
- Rapuano PB, Mathews PM, Florakis GJ, Trokel SL, Suh LH. Corneal collagen crosslinking in patients treated with dextran versus isotonic hydroxypropyl methylcellulose (HPMC) riboflavin solution: a retrospective analysis. Eye Vis. 2018;5:23. doi:10.1186/s40662-018-0116-z.
- Shajari M, Kolb CM, Agha B, Steinwender G, Muller M, Herrmann E, Schmack I, Mayer WJ, Kohnen T. Comparison of standard and accelerated corneal cross-linking for the treatment of keratoconus: a meta-analysis. Acta Ophthalmol. 2019;97(1):e22–e35. doi:10.1111/aos.13814.
- Noor IH, Seiler TG, Noor K, Seiler T. Continued long-term flattening after corneal cross-linking for keratoconus. J Refract Surg. 2018;34(8):567–570. doi:10.3928/1081597X-20180607-01.