Abstract
Purpose: We employed automated analysis of scanning laser ophthalmoscopy (SLO) to determine if mechanical strains imposed on disc, and retinal and choroidal vessels during horizontal duction in children differ from those of adults.
Methods: Thirty-one children aged 11.3 ± 2.7 (standard deviation) years underwent SLO in central gaze, and 35° ab- and adduction. Automated registration with deep learning-based optical flow analysis quantified vessel deformations as horizontal, vertical, shear, and equivalent strains. Choroidal vessel displacements in lightly pigmented fundi, and central disc vessel displacements, were also observed.
Results: As in adults, strain in vessels during horizontal duction was greatest at the disc and decreased with distance from it. Strain in the pediatric disc was similar to published values in young adults,Citation1 but in the peripapillary region was greater and propagated significantly more peripherally to at least three disc radii from it. During adduction in children, the nasal disc was compressed and disc vessels distorted, but the temporal half experienced tensile strain, while peripapillary tissues were compressed. The pattern was similar but strains were less in abduction (p < .001). Choroidal vessels were visualized in 24 of the 62 eyes and shifted directionally opposite overlying retinal vessels.
Conclusions: Horizontal duction deforms the normal pediatric optic disc, central retinal vessels, peripapillary retina, and choroid, shearing the inner retina over the choroid. These mechanical effects occur at the sites of remodeling of the disc, sclera, and choroid associated with typical adult features that later emerge later, including optic cup enlargement, temporal disc tilting, and peripapillary atrophy.
Introduction
The typical healthy child’s eye differs markedly from that of the adult. In young children, the optic cup is typically absent, but emerges and progressively enlarges with age.Citation2 The child’s optic disc is not tilted, but later often tilts temporally with advancing age.Citation3 The child’s peripapillary choroid and retina abut the optic disc, but progressive peripapillary atrophy often develops with age.Citation4 Hyperopia is common, and axial myopia rare, in the infant,Citation5 while progressive axial myopia develops from school age through the teen years with such great frequency as to represent a major public health burden in some ethnic populations.Citation6–8 While the occurrence of these maturational changes in the human eye is widely recognized, the root causes of these features are not only obscure but have rarely even been subjects of speculation. None of the forgoing changes would seem to represent a functional advantage, with most such changes even considered pathological when they advance into older adulthood. It has heretofore been mysterious as to why the optic disc and peripapillary region should change so stereotypically with maturation as they do.
Recent findings suggest a possible mechanical factor that may be an important contributor to these maturational peripapillary changes: biomechanical loading by the optic nerve (ON) loading during eye movement. The ON exerts some drag on the globe during any eye movement in any direction. However, the ON lacks sufficient slack to permit unhindered adduction,Citation9 so that adduction exceeding about 26° causes tethering that stretches the healthy ON,Citation10 but fails to stretch and retracts the globe in primary open-angle glaucoma.Citation11,Citation12 Computational modeling suggests that ON traction in large adduction concentrates mechanical force that compresses and tilts the optic disc and stresses the peripapillary sclera.Citation13 Mechanical effects of ON tethering on the optic disc, peripapillary retina, and choroid have been demonstrated in adults by B-scan optical coherence tomography (OCT)Citation10,Citation14 and most recently by confocal scanning laser ophthalmoscopy (SLO). However, the magnitude of the optic disc and peripapillary deformation during adduction tethering is less in older than younger adults.Citation15 The sclera is recognized to stiffen with age,Citation16–19 yet orbital growth has reached 86% to 96% of adult values for most parameters by age 8 years.Citation20 These observations suggest the possibility that children, with their more pliable ocular tissues, might experience even greater eye movement-related optic disc and peripapillary deformations than young adults. If so, the vast number of ongoing normal eye movements might accumulate to widely deform the posterior eye of the child, and this powerful mechanical phenomenon might be a plausible driver of, or contributor to, the maturational disc and peripapillary changes whose origins have been heretofore unknown.
The present study, therefore, sought to employ automated strain analysis of the optic disc and peripapillary region in children to quantitatively examine tissue deformations arising from static horizontal ductions, and to compare these similarly-obtained data recently published for adults. We tested the prediction that such deformations would be at least as great as those observed in adults but would be more widely distributed in posterior ocular regions in which age-related changes typically emerge later in life.
Methods
Subjects
A total of 31 children (15 boys and 16 girls; mean age, 11.3 ± 2.7 years, standard deviation, SD; range; 8–17 years) were recruited by advertising posted in a pediatric ophthalmology clinic waiting room at the Stein Eye Institute. Subjects gave assent and their parents gave written, informed consent prior to participation according to a protocol approved by the University of California, Los Angeles Institutional Review Board that complied with the Declaration of Helsinki. Subjects underwent ophthalmic history and examination to verify ocular diagnosis and absence of optic neuropathy. Twelve children had history of esotropia, all either corrected to within 10Δ of orthotropia by hyperopic spectacles or strabismus surgery well before time of participation. Seven children had history of exotropia, all but two with intermittent exotropia surgically corrected well before participation. Two children had small-angle intermittent hypertropia. One child each had unilateral high myopia with amblyopia, history of mild retinopathy of prematurity not requiring treatment, and mild abducens paresis. Seven children had normal ophthalmic examinations. Congenital ON anomaly and nystagmus were excluded. Both eyes of all subjects were analyzed. Mean spherical equivalent refractive error was +0.3 ± 2.9 (range, −12 to +8) diopters, including only one high hyperope and one high myope.
Scanning Laser ophthalmoscopic imaging
Rather than obtaining 2D tomographic scans by OCT, the infrared confocal SLO mode of a Heidelberg Spectralis scanner (Heidelberg Engineering, Heidelberg, Germany) was used to image the optic disc and peripapillary retina in central gaze, 35° adduction, and 35° abduction sequentially.Citation1 Because this technique is much faster than OCT, it is particularly suitable for imaging a wide extent of the posterior eye in children. As shown in , the subject’s head position was fixed to the head and chin rest using a wide strap and foam wedges to prevent head rotation from happening unconsciously when rotating eyes. For each gaze direction, the scanner head was rotated on its gimbal so that it remained aligned with the visual axis. Since the internal target of the scanner is offset 12° nasally from straight ahead,Citation10 the offset was considered in setting camera rotation for all gaze directions using a custom goniometric scale on the gimbal mount.Citation15 Imaging was completed in less than a minute in each gaze position, with of this time developed to aligning and focusing the scanner. In this manner, we were able to identify and track blood vessels embedded in the optic disc, retina, and sometimes in the choroid during horizontal duction. These strong, readily identified anatomical features were considered as fiducials that were used to infer deformations in the tissues in which they are embedded.
Digital image processing
Digital image processing as described in our previous study of adults was employed, so is only summarized briefly hereCitation1 Images were preprocessed to equalize illumination and correct torsion before registration into pairs consisting of central gaze–abduction, and central gaze–adduction using the “speeded-up robust features” (SURF) algorithmCitation21 implemented in the software MATLAB R2019b (MathWorks, Natick, MA). Local differences between features in image pairs representing displacement caused by eye movement were determined using a deep learning-based optical flow algorithm called the “recurrent all-pairs field transform” (RAFT). Since we found that retinal vessels often moved in the opposite direction from underlying choroidal vessels to cancel the overall displacement in the peripapillary region, we analyzed the motion of retinal vessels only by isolating them for analysis using a convolutional neural network trained to identify disc and retinal blood vessels, excluding choroidal vessels.
Strain analysis
Le et al.Citation15 manually measured displacement of retinal vessel bifurcation points around the optic disc during horizontal duction. Although the manual measurement was tedious, this study clearly demonstrated local displacement of vascular features around the optic disc during duction. However, because displacement could be measured only at the relatively sparse vessel bifurcation points, data were not available in all anatomical regions, and these informative regions varied among individual subjects. Moreover, displacement does not exactly correspond to deformation. The approach employed here converts local displacements to “strain,” a unitless number that normalizes displacement to local size and thus enables comparison among the subjects while being independent of image magnification.
Image analysis
As previously described,Citation1 images were divided into eight zones, comprising four concentric annular regions of one disc radius extent centered on the optic disc, each divided into hemisectors as shown in . Region 1 represents the optic disc. Region 2 constitutes the annular area extending one disc radius beyond the disc border. Region 3 extends an additional disc radius beyond region 2, and region 4 a further disc radius beyond region 3. After masking to exclude choroidal vessels and other features, strain in disc and retinal vessels was averaged for each zone. Positive strain values indicate tension (stretching), and negative values indicate compression. Strain was analyzed separately in horizontal and vertical directions relative to the disc-foveal line. We also measured shear strain, which arises from opposing forces paralleling an object’s surface. Then, the equivalent strain that combines all three strains was calculated for the measurement of total deformation applied to the posterior eye. The error limits of repeatability of strain measurements were validated in a prior studyCitation1 and depicted here as gray error bands in graphs of the current data. Data in children are compared with published data for 22 young adults of average age 26 ± 5 years since it has already been demonstrated that strains in older adults during adduction are greater and more concentrated at the optic disc than in older adults.Citation1
Statistical analysis
Statistical analysis was performed using generalized estimating equations (GEE) in the IBM SPSS statistical package (IBM, West Armonk, NY). This statistical approach compensates for possible correlations between eyes of individual subjects, permitting each eye to contribute data. The GEE models for strain included the effects of the covariate of age and accounted for possible inter-eye correlations within subjects. Statistical significance was set at p < .05, without correction for multiple comparisons since this was an exploratory study performed for the first time in children.
Results
Horizontal strain
The posterior eye of children was deformed by horizontal duction, as in the example in Supplemental Video 1, creating local strain quantified in . In adduction, the greatest horizontal strain occurred in the temporal hemidisc at 0.59 ± 0.21% compression; the second greatest horizontal strain occurred in the nasal hemidisc at 0.57 ± 0.13% tension. also depicts previously published comparable values for adults, which for horizontal strain in adduction do not differ significantly from children.Citation1 In children, horizontal strain during adduction declined with distance from the disc to the periphery in the nasal hemisector by 39% from the optic disc to immediate peripapillary Region 2, then more moderately and uniformly by 17% from Region 2 to 3, and by 16% Region 3 to 4. It is important that even in Region 4, the most peripheral nasal region evaluated, tensile strain during adduction propagated peripherally to remain significantly greater than the repeatability limit. On the other hand, except for the optic disc, there was little horizontal strain at all in temporal hemisectors in children, or as published in adults.Citation1 In children, the largest horizontal strain during abduction was also in the disc, but there was significantly less strain in both hemidiscs than during adduction: the nasal hemidisc showed 0.22 ± 0.10% compression (p < .001), while the temporal hemidisc showed 0.19 ± 0.19% tension (p = .011). During abduction, all regions outside the optic disc (Regions 2, 3, 4), in children were dominated by small compressive horizontal strains averaging about 0.11%, except for nearly zero strain in the temporal hemisector in Region 2.
Figure 2. Horizontal, vertical, and shear strains around the optic nerve head during adduction and abduction in current data for children and published data for adults.Citation1 Significance is noted only for strains exceeding the published repeatability limits denoted by gray bands.Citation1 *p < .05. **p < .01. SEM: standard error of mean.
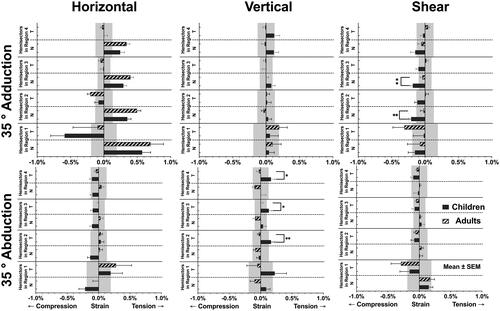
Vertical strain
Adduction produced minimal vertical tensile strain in all regions comparable to the repeatability limit of the technique, as was earlier observed in adultsCitation1 (). Abduction in children induced significant 0.22 ± 0.19% tensile vertical strain in the temporal hemidisc, and average of 0.15% in the peripheral temporal hemisectors, but minimal strain in the peripheral nasal hemisectors, although there was 0.09 ± 0.06% tensile strain in the nasal hemidisc.
Shear strain
Unlike other strains that were maximal in the optic disc, in children the largest shear strain of 0.21 ± 0.04% compression occurred in the nasal hemisector of Region 2 during adduction (). This strain was similar to reported in adults.Citation1 Most regions in the nasal hemisector exhibited compressive shear strain during adduction, and this shear strain propagated nasally to remain significant even in the most peripheral Region 4, being statistically significantly greater than adults in Regions 2 and 3 (). In abduction, the disc experienced the greatest compressive shear strain of 0.15 ± 0.07% in its temporal half, while the greatest tensile shear strain of 0.15 ± 0.15% was in the nasal hemidisc. In abduction children exhibited no significant shear in any region (), generally similar to reported behavior of adults.Citation1
Equivalent strains
Within local regions such as hemisectors, strains in opposite directions can cancel one another when averaged. This cancellation is avoided by computing equivalent strains that combine horizontal, vertical, and shear strains. demonstrates that equivalent strains for both adduction and abduction in the temporal hemidisc of children were similar to one another, measuring 2.46 ± 0.22% and 2.30 ± 0.21%, respectively, and also similar to published values in adults.Citation1 In children, strains in the nasal hemidiscs were lower in both adduction and abduction, but equivalent strain in adduction was about 30% greater at 2.16 ± 0.13% than in abduction at 1.68 ± 0.11% (p = .023). Equivalent strain demonstrated the greatest contrast to adult behavior for both abduction and adduction. In children, equivalent strains in abduction, but not adduction, were greater than for adults in both the nasal (p < .01) and temporal (p < .005) disc hemisectors. The most striking finding of all is that during both abduction and adduction, equivalent strain in all regions and both hemisectors peripheral to the optic disc remained 50% to 100% greater in children than in adults at significance levels generally better than 0.001 ().
Figure 3. Equivalent strains during 35° adduction and 35° abduction in current data for children, and published data for adults.Citation1 Significance of differences from adults is noted. *p < .05. **p < .01. ***p < .001. ****p < .0001. Gray bands denote published repeatability limits.Citation1 SEM: standard error of mean.
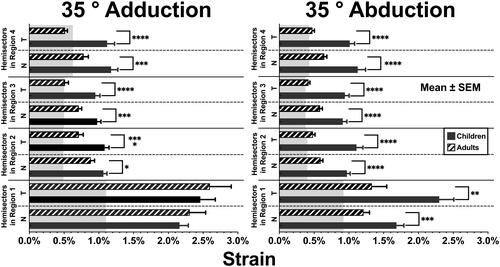
Retinochoroidal shearing
In 24 of the 62 eyes, choroidal vessels were sufficiently visualized through the retina that they could be separately localized by inspection; dense melanin pigmentation obscured choroidal vessels in the remaining eyes. Visualized cases revealed a striking shearing phenomenon during horizontal duction, consisting of directionally opposite movement between overlying retinal vessels, and vessels in the immediately underlying choroid. , better appreciated in Supplemental Video 2, illustrates this shearing in a 10-year-old Caucasian girl who had well-controlled intermittent exotropia and normal corrected visual acuity. Central and adduction images were registered without equalizing illumination for optimal display of choroidal vessels. It is evident in that in adduction, the central vascular trunk of the optic disc moved nasally (A1 and B1, yellow) but the vessels at the nasal disc margin moved temporally (A1 and B1, green). This demonstrates compressive shearing within the optic disc. A second form of shearing occurred concurrently between retina and choroid. Inferonasally located choroidal vessels that were imaged through the overlying retina are demarcated within the red squares in . As seen in , choroidal vessels shifted nasally in adduction relative to overlying retinal vessels. Manual tracing of three vascular points within the choroid demonstrated 30.1 μm mean nasal shift while the nearest overlying retinal vessel shifted 1.0 μm temporally. However, due to the paucity of clearly visible choroidal vessels in most subjects, was not possible to make a quantitative study of the shearing phenomenon.
Figure 4. Shearing in 35° adduction by a 15 year old boy. The dotted lines are vertical for reference, and the solid (oblique) lines extend to shifted positions of the corresponding features in adduction. (A, B) Images in primary gaze and adduction after image registration without illumination equalization. (A1, B1) Magnified view of the green square showing displacement of central vascular trunk bifurcation and disc vessel. While major central vascular trunk vessels shifted temporally in adduction, a vessel in the temporal disc shifted nasally (asterisk). (A2, B2) Magnified view of the region of the red square shows that broad vessels in the choroid (gray) moved nasally in adduction, as did the central retinal vascular trunk within the disc.
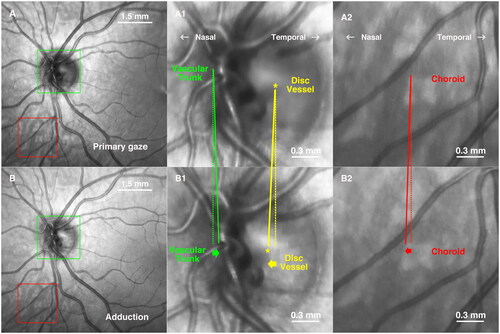
Discussion
The current strain analysis indicates that the optic disc and posterior pole of the child’s eye is structurally dynamic because it deforms during ordinary horizontal eye movements. This finding confirms and extends our earlier report in adults,Citation1 but with some notable differences. Strain during adduction in the temporal hemidisc in children is much larger at 0.6% than reported in younger adults (age 26 ± 5 years) at 0.1%, although similar to the 0.7% strain in older adults (64 ± 10 years). Strain in the nasal hemidisc of children is lower than in younger or older adults and declines more rapidly with distance from the optic disc than in young but not older adults.Citation1 Horizontal strain during abduction in children is much less than in adduction, similar to adults.Citation1 Children exhibit little shear strain in the optic disc or peripapillary region in both ab- and adduction, yet in children, a low level of shear strain in adduction propagates from the optic disc to remain significant even three disc radii from the nasal disc margin ().
It is informative to compare equivalent strain in children with that of adults since this measure of mechanical deformation is not subject to underestimation by oppositely directed behavior in nearby regions. In children, both ab- and adduction produce more than 2% equivalent strain that is maximal within the optic disc, and equivalent strain declines peripherally but remains around half of corresponding values out to the regions 2–3 disc radii from the disc margin; in adults, equivalent strain declined in the periphery to around a third of corresponding optic disc values. Taken together, these findings imply that strain associated with horizontal duction is concentrated in and around the optic disc, but propagates more widely from the disc in children than in adults, so that the force exerted by the ON during horizontal duction is dissipated over a wider extent of the eye than in adults.
The present study also demonstrates that the child’s optic disc is significantly deformed by horizontal duction. In adduction, the central retinal vascular trunk shifts nasally within the disc (), and the temporal disc is horizontally compressed while the nasal disc is stretched. In adduction peripapillary retinal vessels on the nasal side of the optic disc shift temporally, while the underlying choroidal vessels shear by shifting in the opposite direction relative to the overlying retinal vessels. While some of these structural deformations associated with horizontal eye movement have been described in adults,Citation15 disc deformations in children extend broadly into the peripapillary region. In adduction, the pediatric nasal hemidisc undergoes tensile and temporal compressive strain (). To a lesser quantitative extent, the opposite behavior occurs in adduction, where the nasal hemidisc undergoes compressive and temporal hemidisc tensile strain ().
The horizontal eye movements employed in this study are not mere laboratory curiosities. Eye movements such as the 35° add- and abductions employed here are part of everyday life: The normal horizontal duction range is as much as 55°.Citation22 When body and head can both move, 25–45°saccades occur,Citation23 often automatically generated by the vestibulo-ocular reflex.Citation24 Horizontal saccades of more than 40° occur during beverage brewing, searching for objects, ambulation, and catching things.Citation25 About 183 000 saccades are made daily,Citation26 including during sleep.Citation27 Of course, optic disc and peripapillary strains are likely to occur during all eye rotations, no just large ones. The present study did not examine strains during or immediately after saccades, but only during static fixations. Since the sclera, optic nerve, and optic nerve sheath are viscoelastic,Citation28 it is possible the dynamic strains in the disc and peripapillary region following saccades could even be larger than observed here during static fixation.
Mechanical interaction of the intraorbital ON is the likely source of globe deformation during horizontal duction. See-saw tilting of the optic disc during the full range of horizontal duction angles has been demonstrated by OCTCitation10,Citation14,Citation29 that is exaggerated when the ON sheath is distended in papilledema.Citation30–32 Biomechanical modeling suggests that some of this optic disc deformation might be due to drag of the ON within orbital contents.Citation33 However, the consistent finding that optic disc, peripapillary, and choroidalCitation14,Citation34 deformation is greater in adduction than abduction argues that ON tethering contributes to the significantly greater effect of adduction than abduction.Citation9 The typical ON is insufficiently long to permit unhindered adduction, exhausting its sinuous redundancy at an average 26° adduction.Citation10 Beyond 26° adduction, the healthy adult ON stretches to avoid concentrating mechanical force on the disc and peripapillary region, but the stiffer ON in primary open-angle glaucoma fails to stretch during adduction tethering,Citation12 instead retracting the globe.Citation11,Citation12,Citation35 This phenomenon has been suggested by biomechanical modeling to concentrate high stress on the optic disc and temporal peripapillary region.Citation13,Citation36,Citation37
A unifying concept is that pliable ocular tissues, such as those of the children studied here, and the ONs of healthy adults,Citation12 can harmlessly undergo elastic deformation during mechanical perturbations such as associated with eye movements, and then recoil to their normal, unloaded anatomical configurations. The present study demonstrates, during ordinary adduction in healthy children, tension on the nasal disc, and compression of the temporal disc. Resulting tension on the nasal peripapillary retina shifts the central retinal vessels and causes the inner peripapillary retina to shear over underlying choroid, which we have interpreted to reflect retardation of the local translation of the choroid, relative to the retina, due to traction by the ON1. Such deformations are plainly innocuous to the child’s eye in the short term, probably because these deformations propagate widely into the peripapillary region and are associated with little stress in the young, pliable tissues. But as vast numbers of eye movements accumulate during maturity and aging, we suggest that the relentlessly repeated deformations of the disc and peripapillary region may induce downstream vascular and mechanobiological effects, including tissue remodeling,Citation38 collagen synthesis,Citation39 and gene regulationCitation40 in astrocytes,Citation40 oligodendrocytes.Citation41 Optical imaging of the optic disc and peripapillary region in adults of various ages indicates that the eye movement-related deformations decline with progressive age,Citation15 consistent with age-related stiffening that is supported by laboratory studies. Younger people have more elastic peripapillary tissues, including Bruch’s membrane,Citation42 the lamina cribrosa,Citation43,Citation44 and sclera.Citation18,Citation19 Calcification of elastin and increased cross-linked collagen in Bruch’s membrane occur with advancing age.Citation42 Mechanical compliance of the lamina cribrosa declines with age,Citation44 and aging makes older sclera stiffer than younger adult sclera.Citation19 Of course, ocular mechanical properties are likely to vary individually in children, as demonstrated by variation in tensile properties of the eyes of adult eye bank donors.Citation36 Such individual variations would likely alter the local ocular effects of eye movements in children, as suggested by computational modeling,Citation45 as well any resulting long-term remodeling.
We speculate that eye-movement-related mechanical perturbations may be among the causes of maturational changes in the human optic disc and peripapillary region, including emergence and enlargement of the optic cup,Citation2 temporal tilting of the disc, peripapillary atrophy,Citation46 and peripapillary intrachoroidal cavitation.Citation47 All of these phenomena occur at the sites of the substantial tissue deformations during horizontal eye movements. The brief durations of most physiologic eye movements would seemingly require that they be integrated during many repetitions over time in order to be biologically important in driving maturational changes in the eye. We have elsewhere argued that ON tethering in adduction may damage the ON in primary open-angle glaucoma.Citation11,Citation35
The study included Caucasian, Hispanic, and Asian children. In Caucasian children with light ocular pigmentation, choroidal vessels could be visualized through the fair retinal pigment epithelium, revealing the novel phenomenon of relative motion between the inner retina and choroid during adduction, perhaps along Bruch’s membrane, or by shear between the inner and outer retina distributed over its thickness, or shear of retinal vessels over the neural tissue of the retina itself. The central retinal vascular trunk also moved in coordination with the choroid during adduction, suggesting that adduction tethering of the ON shifts the three ocular coats differentially, with greatest deformation at the site of tractional force application on the lamina cribrosa and posterior sclera, less on the choroid, and perhaps least and even in the opposite direction in the ON remote from the disc. We have elsewhere argued that this shear could also be due to greater strain in the relatively soft retina than in the stiffer choroid produced by that same stress associated with eye rotation.Citation1 The real-time shearing phenomenon may be related to the gradual, developmental nasal shift of the lamina cribrosa during axial elongation, in contrast to the relative preservation of the Bruch’s membrane opening and posterior pole.Citation48,Citation49
Strengths and limitations
A strength of this study is its use of machine vision techniques for image analysis that avoid bias and optimize reliability. However, this study has limitations. Although we and others have performed B-scan OCT studies of the optic disc and peripapillary tissues during horizontal ductions by adults, OCT was infeasible in children due to their limited attention spans relative to the prolonged time required to acquire high-resolution OCT, and the very prolonged times required for OCT angiography.Citation50 In adults it is possibleCitation50 but time-consuming to obtain B-scans covering the complete regions imaged by scanning laser ophthalmoscopy in this study. Finally, B-scan OCT is subject to large geometric artifacts in the periphery of widefield images, and to the effects of small, involuntary eye movements across the range of adjacent scans that would compromise reliable image registration and detection of gaze-related deformations at the micron level in three-dimensional reconstructions. It was occasionally impossible to measure the displacement of the central retinal vascular trunk when it was anterior or posterior to the retinal imaging plane. It was often impossible to automatically detect and determine the positions of choroidal vessels despite threshold optimization; this is probably due to light attenuation by overlying tissues such as retinal pigment epithelium and surrounding choroidal pigmentation. Choroidal vessels could not be tracked at all in darkly pigmented individuals. Nevertheless, in some subjects we could document substantial shifts in choroidal vessels relative to the retina ( and Video Clip 2), that are associated with horizontal duction, confirming existence of this novel phenomenon. Finally, due to their low optical contrast even in lightly pigmented children, we could not analyze displacement of choroid vessels and central retinal vascular trunks quantitatively.
Limitations of this study include that it did not measure ocular axial length or keratometry, nor intraocular pressure. Preliminary experiments in adults suggested the absence of IOP changes over the range of eye movements employed here. Moreover, OCT in adults experiencing acute angle closure indicates that even extreme IOP elevation produces only minimal changes in the disc and peripapillary tissues.Citation51 This study investigated only short-term, static lateral gaze fixations on the order of a minute or less. We have found during MRI scanning that adults, but usually not children, can maintain large lateral gaze positions for up to several minutes before limitation by fatigue and involuntary eye movements.Citation52 It is possible that ocular strains could change during prolonged eccentric gaze due to viscoelastic tissue behavior and fluid shifts in the eye, orbital vessels, and optic nerve subarachnoid space. Moreover, disc and peripapillary strains might also vary during dynamic eye movements such as saccades. Such considerations will require future study.
Conclusion
All parts of the child’s posterior eye are significantly deformed during normal horizontal duction. The more widespread propagation of this deformation into the peripapillary region is consistent with greater tissue pliability in children than adults. Particularly in adduction, but also in abduction, the central vascular trunk shifts within the optic disc, the optic disc is horizontally compressed, peripapillary vessels shift, and choroidal vessels experience shearing motion relative to opposite motion of the vessels in the overlying retina. These mechanical effects might contribute to maturational and even pathological remodeling of the optic disc and peripapillary region over the human lifespan.
Supplemental Material
Download Zip (5.1 MB)Disclosure statement
No potential conflict of interest was reported by the author(s).
Additional information
Funding
References
- Park J, Moon S, Lim S, Demer JL. Scanning laser ophthalmoscopy demonstrates disc and peripapillary strain during horizontal eye rotation in adults. Am J Ophthalmol. 2023;254:114–127. doi: 10.1016/j.ajo.2023.06.008.
- Park HJ, Hampp C, Demer JL. Longitudinal study of optic cup progression in children. J Pediatr Ophthalmol Strabismus. 2011;48(3):151–156. doi: 10.3928/01913913-20100719-06.
- Kim TW, Kim M, Weinreb RN, Woo SJ, Park KH, Hwang JM. Optic disc change with incipient myopia of childhood. Ophthalmology. 2012;119(1):21–26.e3. doi: 10.1016/j.ophtha.2011.07.051.
- Tun TA, Wang X, Baskaran M, Nongpiur ME, Tham Y-C, Perera SA, Strouthidis NG, Aung T, Cheng C-Y, Girard MJA, et al. Variation of peripapillary scleral shape with age. Invest Ophthalmol Vis Sci. 2019;60(10):3275–3282. doi: 10.1167/iovs.19-26777.
- Kuo A, Sinatra RB, Donahue SP. Distribution of refractive error in healthy infants. J Aapos. 2003;7(3):174–177. doi: 10.1016/s1091-8531(03)00017-x.
- Lin LL, Shih YF, Tsai CB, Chen CJ, Lee LA, Hung PT, Hou PK. Epidemiologic study of ocular refraction among schoolchildren in Taiwan in 1995. Optom Vis Sci. 1999;76(5):275–281. doi: 10.1097/00006324-199905000-00013.
- Foster PJ, Jiang Y. Epidemiology of myopia. Eye (Lond)). 2014;28(2):202–208. doi: 10.1038/eye.2013.280.
- Saw SM, Katz J, Schein OD, Chew SJ, Chan TK. Epidemiology of myopia. Epidemiol Rev. 1996;18(2):175–187. doi: 10.1093/oxfordjournals.epirev.a017924.
- Demer JL. Optic nerve sheath as a novel mechanical load on the globe in ocular duction. Invest Ophthalmol Vis Sci. 2016;57(4):1826–1838. doi: 10.1167/iovs.15-18718.
- Suh SY, Le A, Shin A, Park J, Demer JL. Progressive deformation of the optic nerve head and peripapillary structures by graded horizontal duction. Invest Ophthalmol Vis Sci. 2017;58:5015–5021.
- Demer JL, Clark RA, Suh SY, Giaconi JA, Nouri-Mahdavi K, Law SK, Bonelli L, Coleman AL, Caprioli J. Optic nerve traction during adduction in open angle glaucoma with normal versus elevated intraocular pressure. Curr Eye Res. 2020;45(2):199–210. doi: 10.1080/02713683.2019.1660371.
- Clark RA, Suh SY, Caprioli J, Giaconi JA, Nouri-Mahdavi K, Law SK, Bonelli L, Coleman AL, Demer JL. Adduction-induced strain on the optic nerve in primary open angle glaucoma at normal intraocular puressure. Curr Eye Res. 2021;46(4):568–578. doi: 10.1080/02713683.2020.1817491.
- Shin A, Yoo L, Park J, Demer JL. Finite element biomechanics of optic nerve sheath traction in adduction. J Biomech Eng. 2017;139(10):1010101–10101010. doi: 10.1115/1.4037562.
- Chang MY, Shin A, Park J, Nagiel A, Lalane RA, Schwartz SD, Demer JL. Deformation of optic nerve head and peripapillary tissues by horizontal duction. Am J Ophthalmol. 2017;174:85–94. doi: 10.1016/j.ajo.2016.10.001.
- Le A, Chen J, Lesgart M, Gawargious BA, Suh SY, Demer JL. Age-dependent deformation of the optic nerve head and peripapillary retina by horizontal duction. Am J Ophthalmol. 2020;209:107–116. doi: 10.1016/j.ajo.2019.08.017.
- Sigal IA, Flanagan JG, Tertinegg I, Ethier CR. Finite element modeling of optic nerve head biomechanics. Invest Ophthalmol Vis Sci. 2004;45(12):4378–4387. doi: 10.1167/iovs.04-0133.
- Avetisov ES, Savitskaya NF, Vinetskaya MI, Iomdina EN. A study of biochemical and biomechanical qualities of normal and myopic eye sclera in humans of different age groups. Metab Pediatr Syst Ophthalmol. 1983;7(4):183–188.
- Geraghty B, Jones SW, Rama P, Akhtar R, Elsheikh A. Age-related variations in the biomechanical properties of human sclera. J Mech Behav Biomed Mater. 2012;16:181–191. doi: 10.1016/j.jmbbm.2012.10.011.
- Coudrillier B, Tian J, Alexander S, Myers KM, Quigley HA, Nguyen TD. Biomechanics of the human posterior sclera: age- and glaucoma-related changes measured using inflation testing. Invest Ophthalmol Vis Sci. 2012;53(4):1714–1728. doi: 10.1167/iovs.11-8009.
- Escaravage GK, Jr., Dutton JJ. Age-related changes in the pediatric human orbit on CT. Ophthalmic Plast Reconstr Surg. 2013;29(3):150–156. doi: 10.1097/IOP.0b013e3182859716.
- Bay H, Ess A, Tuytelaars T, Van Gool L. Speeded-up robust features (SURF). Comput Vis Image Und. 2008;110(3):346–359. doi: 10.1016/j.cviu.2007.09.014.
- Guitton D, Volle M. Gaze control in humans: eye-head coordination during orienting movements to targets within and beyond the oculomotor range. J Neurophysiol. 1987;58(3):427–459. doi: 10.1152/jn.1987.58.3.427.
- Anastasopoulos D, Ziavra N, Hollands M, Bronstein A. Gaze displacement and inter-segmental coordination during large whole body voluntary rotations. Exp Brain Res. 2009;193(3):323–336. doi: 10.1007/s00221-008-1627-y.
- Anastasopoulos D, Naushahi J, Sklavos S, Bronstein AM. Fast gaze reorientations by combined movements of the eye, head, trunk and lower extremities. Exp Brain Res. 2015;233(5):1639–1650. doi: 10.1007/s00221-015-4238-4.
- Kothari R, Yang Z, Kanan C, Bailey R, Pelz JB, Diaz GJ. Gaze-in-wild: a dataset for studying eye and head coordination in everyday activities. Sci Rep. 2020;10(1):2539. doi: 10.1038/s41598-020-59251-5.
- Robinson DA. Control of eye movements. In TGerjung R (Ed). Comprehensive Physiology. Hoboken (NJ): John Wiley & Sons, Ltd (pp. 1275–1320), 2011.
- Leclair-Visonneau L, Oudiette D, Gaymard B, Leu-Semenescu S, Arnulf I. Do the eyes scan dream images during rapid eye movement sleep? Evidence from the rapid eye movement sleep behaviour disorder model. Brain. 2010;133(Pt 6):1737–1746. doi: 10.1093/brain/awq110.
- Song D, Lim S, Park J, Demer JL. Linear viscoelasticity of human sclera and posterior ocular tissues during tensile creep. J Biomech. 2023;151:111530. doi: 10.1016/j.jbiomech.2023.111530.
- Suh SY, Clark RA, Demer JL. Optic nerve sheath tethering in adduction occurs in esotropia and hypertropia, but not in exotropia. Invest Ophthalmol Vis Sci. 2018;59(7):2899–2904. doi: 10.1167/iovs.18-24305.
- Sibony PA, Hou W. Adduction-induced deformations evoke peripapillary folds in papilledema. Ophthalmology. 2019;126(6):912–914. doi: 10.1016/j.ophtha.2018.12.043.
- Sibony PA. Gaze-evoked deformations of the peripapillary retina and papilledema and ischemic optic neuropathy. Invest Ophthalmol Vis Sci. 2016;57(11):4979–4987. doi: 10.1167/iovs.16-19931.
- Sibony PA, Wei J, Sigal IA. Gaze-evoked deformations in optic nerve head drusen: repetitive shearing as a potential factor in the visual and vascular complications. Ophthalmology. 2018;125(6):929–937. doi: 10.1016/j.ophtha.2017.12.006.
- Wang X, Fisher LK, Milea D, Jonas JB, Girard MJ. Predictions of optic nerve traction forces and peripapillary tissue stresses following horizontal eye movements. Invest Ophthalmol Vis Sci. 2017;58(4):2044–2053. doi: 10.1167/iovs.16-21319.
- Chen JY, Le A, De Andrade LM, Goseki T, Demer JL. Compression of the choroid by horizontal duction. Invest Ophthalmol Vis Sci. 2019;60(13):4285–4291. doi: 10.1167/iovs.19-27522.
- Demer JL, Clark RA, Suh SY, Giaconi JA, Nouri-Mahdavi K, Law SK, Bonelli L, Coleman AL, Caprioli J. Magnetic resonance imaging of optic nerve traction during adduction in primary open-angle glaucoma with normal intraocular pressure. Invest Ophthalmol Vis Sci. 2017;58(10):4114–4125. doi: 10.1167/iovs.17-22093.
- Park J, Shin A, Jafari S, Demer JL. Material properties and effect of preconditioning of human sclera, optic nerve, and optic nerve sheath. Biomech Model Mechanobiol. 2021;20(4):1353–1363. doi: 10.1007/s10237-021-01448-2.
- Jafari S, Lu Y, Park J, Demer JL. Finite element model of ocular adduction by active extraocular muscle contraction. Invest Ophthalmol Vis Sci. 2021;62(1):1. doi: 10.1167/iovs.62.1.1.
- Liu B, McNally S, Kilpatrick JI, Jarvis SP, O'Brien CJ. Aging and ocular tissue stiffness in glaucoma. Surv Ophthalmol. 2018;63(1):56–74. doi: 10.1016/j.survophthal.2017.06.007.
- van Geemen D, Driessen-Mol A, Baaijens FP, Bouten CV. Understanding strain-induced collagen matrix development in engineered cardiovascular tissues from gene expression profiles. Cell Tissue Res. 2013;352(3):727–737. doi: 10.1007/s00441-013-1573-2.
- Walker A, Kim J, Wyatt J, Terlouw A, Balachandran K, Wolchok J. Repeated in vitro impact conditioning of astrocytes decreases the expression and accumulation of extracellular matrix. Ann Biomed Eng. 2019;47(4):967–979. doi: 10.1007/s10439-019-02219-y.
- Tsai E, Casaccia P. Mechano-modulation of nuclear events regulating oligodendrocyte progenitor gene expression. Glia. 2019;67(7):1229–1239. doi: 10.1002/glia.23595.
- Booij JC, Baas DC, Beisekeeva J, Gorgels TG, Bergen AA. The dynamic nature of Bruch’s membrane. Prog Retin Eye Res. 2010;29(1):1–18. doi: 10.1016/j.preteyeres.2009.08.003.
- Albon J, Karwatowski WS, Avery N, Easty DL, Duance VC. Changes in the collagenous matrix of the aging human lamina cribrosa. Br J Ophthalmol. 1995;79(4):368–375. doi: 10.1136/bjo.79.4.368.
- Albon J, Karwatowski WS, Easty DL, Sims TJ, Duance VC. Age related changes in the non-collagenous components of the extracellular matrix of the human lamina cribrosa. Br J Ophthalmol. 2000;84(3):311–317. doi: 10.1136/bjo.84.3.311.
- Park J, Shin A, Demer JL. Finite element modeling of effects of tissue property variation on human optic nerve tethering during adduction. Sci Rep. 2022;12(1):18985. doi: 10.1038/s41598-022-22899-2.
- Jonas JB, Spaide RF, Ostrin LA, Logan NS, Flitcroft I, Panda-Jonas S. IMI-nonpathological human ocular tissue changes with axial myopia. Invest Ophthalmol Vis Sci. 2023;64(6):5. doi: 10.1167/iovs.64.6.5.
- Ehongo A, Bacq N. Peripapillary intrachoroidal cavitation. J Clin Med. 2023;12(14):4712. doi: 10.3390/jcm12144712.
- Lee KM, Kim M, Oh S, Kim SH. Position of central retinal vascular trunk and preferential location of glaucomatous damage in myopic normal-tension glaucoma. Ophthalmol Glaucoma. 2018;1(1):32–43. doi: 10.1016/j.ogla.2018.05.003.
- Lee KM, Choung HK, Kim M, Oh S, Kim SH. Positional change of optic nerve head vasculature during axial elongation as evidence of lamina cribrosa shifting: boramae myopia cohort study report 2. Ophthalmology. 2018;125(8):1224–1233. doi: 10.1016/j.ophtha.2018.02.002.
- Lim S, Tran A, Garcia SE, Demer JL. Optical coherence tomography angiography demonstrates strain and volume effects on optic disc and peripapillary vasculature caused by horizontal duction. Curr Eye Res. 2023;48(5):518–527. doi: 10.1080/02713683.2023.2172185.
- Wang YX, Jiang R, Wang NL, Xu L, Jonas JB. Acute peripapillary retinal pigment epithelium changes associated with acute intraocular pressure elevation. Ophthalmology. 2015;122(10):2022–2028. doi: 10.1016/j.ophtha.2015.06.005.
- Demer JL, Clark RA, Kono R, Wright W, Velez F, Rosenbaum AL. A 12-year, prospective study of extraocular muscle imaging in complex strabismus. J Aapos. 2003;6(6):337–347. doi: 10.1067/mpa.2002.129040.