Tribute
While perhaps best be known by outsiders as a coastal geomorphology expert, Dr. Antony Orme was in fact a consummate example of a well-rounded field geomorphologist, as evinced by his wide-ranging publications which focused on topics from tectonism, beaches, and paleoecology to geomorphology history (of the discipline), Pleistocene research, and climatic geomorphology, among others. This field tradition was passed onto many of his students who, in turn, continue to pass on such traditions to their students. While we were fortunate to have many strong mentors along our paths, the encouragement and continued camaraderie by our PhD Advisor (Ron Dorn) nonetheless, and at least partially, stemmed from his advisor, Dr. Antony “Tony” Orme. Without Tony’s own desire to explore the world and nature – and coasts specifically – perhaps we (the authors) would not have had successes in our own field-based research endeavors (Allen, Citation2005, Citation2012, Citation2017, Citation2019; Dorn et al., Citation2013; Paradise, Citation2012, Citation1995, Citation2005, Citation2010). Although we both retain strong inclinations for empirical research and fieldwork, having enjoyed exploring since we were young, without proper guidance, that spirit can easily become extinguished. Had Tony not passed on his love of exploration and adventure to his students, maybe our advisor would not have done so either, leaving many landscapes and landforms undiscovered and unexplained. We feel fortunate to have benefited from his legacy that has allowed us to conduct geomorphic fieldwork in very interesting locations, on very intriguing landforms, and across landscapes at local, regional, and international scales.
As we both continue to explore and guide our own academic children (and grandchildren) just as he did, we continue to perpetuate the legacy and indomitable nature of a great geographer and geomorphologist, Dr. Antony Orme. It is with this spirit of Tony’s intrepidness, we present this article examining two types of relict landforms, selected regionally due to our current respective locales, that highlight some of our recently undertaken field-based research in the US and Caribbean we believe would have intrigued our academic grandfather.
Relict landforms
Though definitions vary across disciplines – from a continual taxon of a once-diverse and extensive population (cf., DuBois & Kapustka, Citation1983; Grandcolas et al., Citation2014; Milne & Abbott, Citation2002) and “ … distinctive populations or species that typically are small in size or severely restricted in geographic range” (Habel et al., Citation2010: 1), to something cosmically old or leftover in physics (cf., Starobinskii, Citation1979; Stuchlík, Citation2005) – in the Earth sciences, Cotton (1945: 396) described these residual (or relict) landforms as, “ … those developed by erosive processes (morphogenetic systems) no longer operating.” He also proposes the German synonym of “fossil” landform, noting that such features rarely exist as an entire landscape in and of themselves, and instead share the geomorphological stage with ongoing landform processes. Stratford (Citation1999) suggested a similar sentiment in their coastal relict landforms literature review centered on ancient Lake Bonneville, and Viles (Citation1987) gave the term a quick mention in her overarching review of coastal landforms. In terms of relict landform research, the majority seems clustered around glaciology and glacial/peri-glacial geomorphology (cf., Clarhäll & Kleman, Citation1999; Fredin & Hättestrand, Citation2002; Leslie & McGlone, Citation1973; Ross et al., Citation2019; Wilson, Citation2004; Wilson et al., Citation2020) and rock glaciers (cf., Colucci et al., Citation2019; Frauenfelder & Kääb, Citation2000; Kofler et al., Citation2020; Selley et al., Citation2019; Wagner et al., Citation2019), with very little of the research centered on coastal relict landforms specifically. Still, Budanov et al. (Citation2018), Winker and Howard (Citation1977), and (Reimnitz & Wolf, Citation1998) have studied non-dune coastal forms, while Buynevich et al. (Citation2007) and Lericolais et al. (Citation2007) focused their research explicitly on coastal dunes.
That said, those studies (and many others) remain focused on the connection between relict landform and climate – and often paleoclimate in particular – which makes sense, given the strong links between glacial regions and climate change studies. As early as the 1970s, geomorphologists understood the importance of linking climate to landforms (and geomorphology, see Derbyshire, Citation1973; Sauchyn, Citation1990; Shaw, Citation1976; Wolman & Gerson, Citation1978), and Tony specifically focused on the geomorphology-climate link in the 1980s (Orme, Citation1980, Citation1988, Citation1989), including an article on relict coastal landforms (Orme & Orme, Citation1991). It has also been noted that at least 95% of landforms found in the mid-latitudes represented relict features, since “The ability of the rocks to withstand erosion does not depend on their physical but rather on their morphological hardness,” which “ … varies according to climate” (Büdel, Citation1973: 203). This means that a specific rock type, for example, granite – “ … one of the hardest rocks in the mid-latitudes … ” – remains “ … soft and sand-like … in the moist tropics, where deep weathering prevails” (Büdel, Citation1973: 203). In other words, rocks found in mid-latitude climates are generally more resistant to decay and subsequent erosion, which means such landscapes host more remnants than other climatic zones.
Even so, in geomorphology it is often easiest to identify the prevalent processes at one or various scales (since most processes and forms within geomorphology are scale independent, cf., Phillips, Citation2001, Citation1999). For example, we are taught early on that in geomorphic systems there occurs a correspondence between the scales of process and forms. However, modern research indicates that the identification and study of nested, hierarchical structures can offer great understanding into the effect of scale on process where “every geomorphic system consists of an array of ever smaller, lower-level systems, and is at the same time part of a sequence of ever larger, higher-level system” (DeBoer, Citation1992: 303). Such is the case in the examination of coastal features. Indeed, ascertaining a dominant force, factor, or influence of a geomorphic feature remains a fairly straightforward effort. The difficulty rests, however, in determining with what magnitude that force is acting, and/or in many cases, at which frequency (and scale) of influence or “interruption” said feature occurs (to use the archaic Davisian notion). That means scrutinizing coastal features can generate insight once we understand that coastal landscapes dominated by high-frequency, low-magnitude events and processes (i.e., subaerial weathering) may often result in landscapes exhibiting medium-sized features, while the low-frequency, high-magnitude processes (i.e., tectonic uplift) may produce distinctive landscapes displaying large/very large-sized features. In short, physical landscape features remain polygenetic precisely because geomorphologic processes, though nested and hierarchical in terms of magnitude and frequency, ultimately remain independent of scale.
The importance of relict landform studies informing interpretation and evaluation of polygenetic landscape features notwithstanding, outside of dune complexes, relict coastal landforms appear disproportionately in the literature – even in the mid-latitudes where they should be/are more prevalent. This seems odd, given the proclivity of coastal features’ often-close association with climate change via sea level change. Still, for our purposes, the term relict refers to landscape features that did not undergo changes as quickly as adjacent or similar lithologies, features, or parts of a landscape. Since many surface process rates change, or have their affecting features destroyed, relict features represent those that are not completely changed, distorted, transformed, or razed since the relict characteristics represent a prior process and/or differing rate of effect. Such relict landscapes have always fascinated geographers, geologists, and other Earth scientists and, to that end, we present two short, somewhat-linked case studies from Northern California and Barbados, centered on two relict landform types we believe Tony Orme would have enjoyed analyzing with us: relict marine (wave-cut) terraces and (inland) relict tidal/wave-cut “mushroom” rocks.
Marine (wave-cut) terraces
Marine terraces and their accompanying broader landscapes are considered to have been less affected by active processes changing its adjoining terrain. Such features, like a wave-scoured plateau sitting high above the sea, are often distinctive on the landscape while existing side-by-side with landforms that are currently more affected by active depositional or erosional processes. Such is the case with the distinctive raised shoreline platforms and terraces that line the Northern California Coast. These marine terraces are found stacked along and above the orogenic belt of the Pacific Coast – an emerging Quaternary landscape – which often represent ancient nascent terraces and exposed shoreline features (i.e. dunes, wave-cut platforms). However, it was their patches of unusual dwarf trees and grey soils that drew attention to further study (Jenny et al., Citation1969). Perched paleosols (podzols), which form under conditions dramatically different from the present climate and topography, support dwarf cypress trees; these were the keys that fueled the investigations into Northern California’s unique relict marine terraces (Jenny et al., Citation1969; Matthews, Citation1972).
Nestled among California’s famous coastal Redwoods (Sequoia sempervirens) that flank the coast from Monterey north to the Oregon border, and perched hundreds of feet above the coast, one finds remote and perplexing patches of dwarfed trees (Cupressus pigmaea) and endemic vegetation, and sand dunes, sitting atop distinctive grey soils or podzols. These distinct areas of acidic soils and stunted trees are rare along the coastline and represent fragmented mini-biomes perched above the sea on a series of uplifted marine terraces. Not only are these vicariant patches of dwarf trees unusual, but their occurrence drove early discussions and examinations of their odd geomorphic setting: these parallel tiered terrace complexes found along the coast. From Santa Cruz to Mendocino Counties along the western coast, up to five terraces have been identified, having been uplifted over millennia through what are believed to be episodic periods of upheaval, rather than continual forces (Jenny, Citation1973; Schulz et al., Citation2018; see , this article). Since their attributed discovery to Hans Jenny in the 1960s, these relatively en-echelon terraces exhibit an “ecosystem evolution” from the lower terraces covered with humus-rich soils covered by grasses on coastal prairies, to somewhat infertile acidic soils (podzols) supporting endemic and dwarfed vegetation: dwarf pygmy forests (Jenny et al., Citation1969).
Figure 1. Site map of the two marine terrace locations – Albion in Mendocino, County, and Davenport in Santa Cruz County, California. Map by T.R. Paradise.
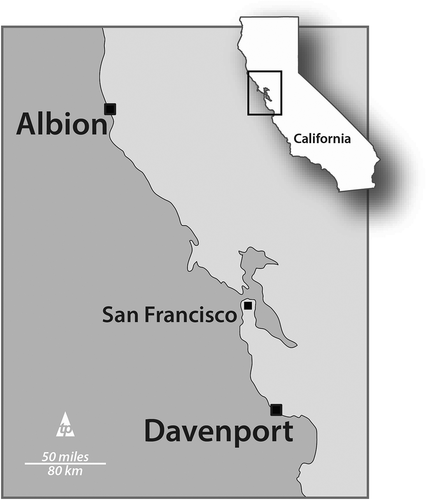
Figure 2. Site map identifying the locations of the pygmy Cypress and ashen-soil (podzolic) ecosystems. The dwarf “forests” are found on the eastern flanks of the second, third, and fourth terraces as mapped by Jenny (Citation1973). It was the occurrence of these dwarf trees on podzols that triggered early research into the geomorphologic origins of these ecologic staircases. Map by T.R. Paradise.
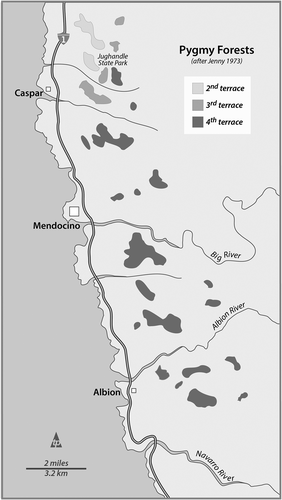
These broad and frequently evenly spaced terraces frequently give the northern California coastline a distinctive look, much like staircases rising from the sea. However, it was only in recent years that their morphology was recognized and explained.
Origins and prior research
These marine terraces have been attributed to the interplay of two processes: tectonic uplift and sea level change. Following the ice ages, as the sea level rose, waves action combined with mobilized submarine sediments to scour and carve off-shore platforms through abrasion, cavitation erosion, and subaerial and submarine mass-wasting. Once the sea level dropped, these erosional platforms were exposed along the coast (Matthews, Citation1972; Schulz et al., Citation2018). Adding to sea level change and subaerial exposure, active seismicity flanking the San Andreas Fault and adjacent to the Mendocino Triple Plate Junction of the North American, Pacific, and Gorda plates, exacerbated crustal deformation in the area causing further uplift (Shobe et al., Citation2020; Simms et al., Citation2016). So, when the uplift was relatively fast enough, these wave-cut platforms were “preserved as marine terraces above the influence of the ocean” (Schulz et al., Citation2018). The highest and most distinctive marine terraces noted in this paper are near Albion (Mendocino County) and Davenport (Santa Cruz County), California where they are elevated above the current sea level (MSL) from 600ʹ (180 m) at Davenport to nearly 1000ʹ (300 m) near Albion.
Mendocino county terraces: Albion, California
The prevalent rock substrate in the region is the Franciscan Formation’s mélange of marine graywacke sandstones (Cretaceous) and comprises the terraces along the Mendocino Coast. In the 1960s, Hans Jenny (Jenny, Citation1973) identified five characteristic terraces along Jug Handle Creek roughly situated above sea level at 30 m (100ʹ), 90 m (300ʹ), 130 m (425ʹ), and 200 m (650ʹ) – the fifth terrace is located above 240 m (800ʹ) but is heavily forested and obscured. Jenny called them the first, second, third, fourth and fifth terraces (). He also coined a “zero terrace” which is presently being hewn and shaped at the low tide stage as a “rough, rubbled bedrock surface” that supports tidepools, sea stacks, skerries, and arches with flanking pocket beaches and cliffs (Jenny et al., Citation1969).
Figure 3. Mendocino County’s marine terraces: first, second, and third. The fourth terrace is somewhat visible with cleared ground above the third. Jenny’s (Citation1973) ‘zero terrace is visible here as the wave-cut platform interspersed with pocket beaches and caves. Image: commons.wikimedia.org/wiki/File:Marine_terraces_California.jpg.
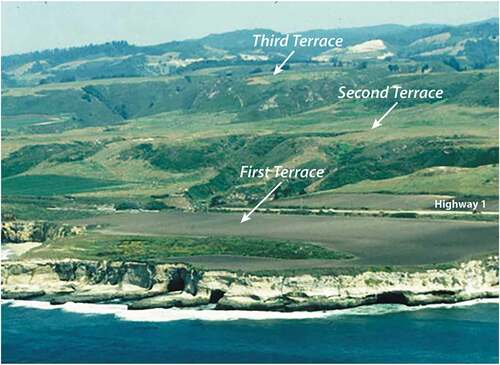
Figure 4. An oblique diagram of the surface and cross-sections of the Jug handle ecological Staircase showing the repeated substrate composition of each terrace: dunal ridge, coastal sediments and hardpan and podzols supporting the dwarf Cypress forests. Diagram by T.R. Paradise after Jenny (Citation1973), Schulz et al. (Citation2018).
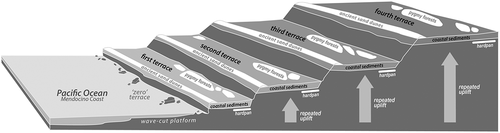
It was Jenny’s early astute observations and fieldwork that first acknowledged these geomorphic staircases through the documentation of the (a) incongruous vegetation, (b) abrupt cliff-faces separated by broad fields, and (c) patches of peculiar grey soils (podzols). He also noted that the humus-covered substrate had a repeated and incongruous composition across each terrace. At the terrace’s western edges, a buried dunal ridge was found, followed by a distal ancient shorefront complex, and further behind (to the east) by perched patches of ashen-grey podzols (). These aluminum-rich soils are prevalent beneath Europe’s Heathlands, and Australia’s Eucalyptus forests, however, in this region they are not a function of the overgrowth but the tilted terrace structure, inconsistent permeability and fertility, and lengthy periods of subaerial weathering (Jenny et al., Citation1969; Schulz et al., Citation2018; see , this article). Observed on each terrace, it was the recurring sand dunes, wave knickpoints, paleo-shorefronts, and patches of podzols that suggested that each terrace had been uplifted during episodes of relatively rapid upheaval: the older terraces located above each other, and farther from the present shoreline (Fox, Citation1976; Jenny, Citation1973).
Figure 5. An orthophotograph and topographic representation of the Mendocino County marine terraces at the confluence of the Navarro River and Pacific Ocean, near Albion, California. Note that the terraces here are relatively similar in dimension and spacing – both laterally and vertically. Jenny’s (Citation1973) wave-cut platform and offshore stacks, arches, tombolos, and skerries represent his “zero” terrace. Image by Google Earth and map by T.R. Paradise.
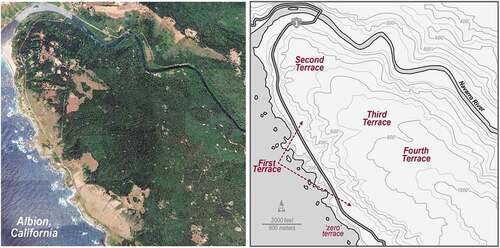
In addition to Jenny’s “ecological staircase” at Jug Handle Creek (now Jug Handle State Park), one of the best examples of marine terraces in Mendocino County can be observed above the confluence of the Navarro River and Pacific Ocean near the town of Albion. As the river/estuary meanders to the north entering the sea, the terraced staircase is visible descending to the confluence (). Like many of the terraces in Northern California, the first terrace is located at 15 m-30 m (50ʹ-100ʹ) above sea level with the zero terrace visible below as the wave-cut platform presently being carved between the low and high tide zones. The first and second terraces are often grassy prairies with patches of assorted cypress (Cupressaceae), Bolander shore pine (Pinus contorta), and introduced gum (Eucalyptus sp.) trees thriving atop fertile mollisols. Occasional coastal redwoods may be found, however, most were cut to supply timber for the expansion of the California railroads, and the rebuilding of San Francisco following the 1906 Earthquake (Crump, Citation1998), since the first terrace was an ideal height for the felled redwoods to be sledged into the sea to be loaded and hauled south shipboard. Most of the coastal development in the area sits atop the first and second terraces.
Figure 6. An orthophotograph and topographic representation of the Santa Cruz County marine terraces near Davenport, California. Note that the terraces here are dissimilar in dimension and spacing where coastal erosion and weathering is causing rapid clii face retreat across the first terrace. Third and fourth terraces are markedly smaller although are estimated to have been exposed 80 K year less. Jenny’s (Citation1973) wave-cut platform and offshore stacks, arches, tombolos, and skerries represent his “zero” terrace. Image by Google Earth and map by T.R. Paradise.
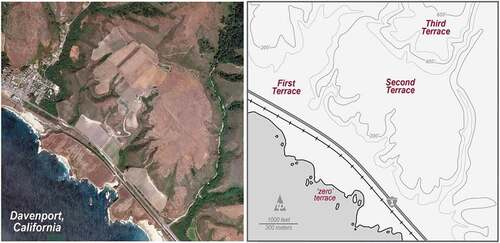
Along transects ascending the stepped terraces, there are distinct changes in vegetation related to the soil development and micro-climatic shifts: Grand fir (Abies grandis), Douglas fir (Pseudotsuga menziesii), Hemlock (Tsuga heterophylla) and redwood (Sequoia sempervirens) are found on the second and third terraces, mixed Bishop pine (Pinus muricata) and redwood groves on the 3rd–4th terraces, and the pygmy cypresses found at the far eastern edges of the third to fifth terraces. Pygmy forests like these are not rare where they can be found in high-latitude permafrost areas where root penetration is constrained, and on mid-latitude saturated nutrient-poor soils. Hans Jenny (Jenny, Citation1973: 15) described these pygmy forests in Mendocino “ … as a class by itself … as the culmination of uninterrupted ecosystem evolution”. The presence of coastal redwoods can be attributed to regional foggy, coastal climates, but these pygmy cypresses occur as a function of edaphic controls (Matthews, Citation1972).
At first, the fifth terraces were estimated to be roughly 1,000,000 years old (Matthews, Citation1972) however, recent studies indicate an uplift rate of <1 m (Simms et al., Citation2016) to 3.5 m/1,000 years (Merritts & Bull, Citation1989) dating the fifth terraces to 150,000 to 300,000 years. Plate flexure and torque during periods of uplift and sea level fluctuations have been attributed to the broad variations in exposure dates as well (Perg et al., Citation2001).
Santa Cruz county terraces: Davenport, California
North of Santa Cruz along scenic coastal Highway 1 sits the town of Davenport, known for its former lime quarry and booming cement industry. Unlike the marine terraces to the north which are a part of the Franciscan mélange, the Davenport terrace complex is underlain by the Santa Cruz Mudstone, a Miocene marine-fluvial diatomaceous rock exhibiting roughly horizontal strata. It is this relatively under formed assemblage that has created a more level step morphology than found in the more unconsolidated Franciscan Formation in Albion where the terraces may dip upslope or toward the sea (). Unlike in Mendocino where the terrace edges and capping fields were shaped by shoreline processes, at Davenport, it is the horizontal strata in tandem with prior shore processes that shaped the relatively flat terraces. However, different the geologic substrates, the subaerial morphologies of the two sites are quite similar. Davenport’s first terrace is located at roughly 15–45 m (50ʹ-150ʹ) above sea level, followed by the second terrace at 60 m (200ʹ), 150 m (500ʹ), and the fourth at 180 m (600ʹ). Like in Albion at the Navarro River confluence, the fifth terraces are heavily wooded and difficult to identify and analyze.
Figure 7. Graph and table representing the estimated ages of the marine terraces in Mendocino and Santa Cruz counties, California as a function of elevation. These findings were based on pedogenic analyses of iron and aluminum (Merritts & Bull, Citation1989) and cosmogenic radionuclide examination (Perg et al., Citation2001). Note that the Mendocino terraces are significantly older (c. 20k-80 K years) and higher (3rd terrace from 600ʹ-800ʹ) than the Santa Cruz terraces (3rd terrace at 400ʹ-600ʹ). Graphics by T.R. Paradise, after Merritts and Bull (Citation1989) and Perg et al. (Citation2001)
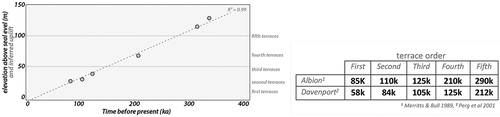
Figure 8. Image looking south in Mendocino County (from Albion to Elk, CA) at the sea stacks and wave-cut platforms on the “zero” terrace. The white structure sits atop the first terrace, and the second and third terraces are visible to the east. The relatively level mountain top to the left/east represents a fourth terrace in this area. Image by T.R. Paradise.
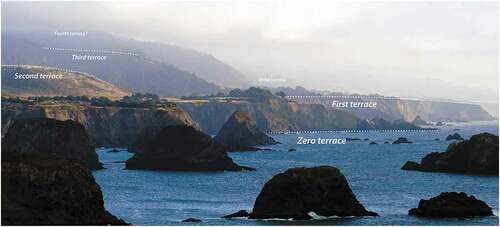
Along the Santa Cruz coastline, the first terrace is mostly built-up and occupied, while the upper second and third terraces are widely used for agricultural purposes: this region is commonly known for artichoke and Brussel sprout crops. In many areas, the first terrace has eroded into gullies or chines that cut through the cliff edge to slope to the sea or a pocket beach. Here, the zero terrace platform is often scattered with sea stacks and arches, skerries, small tombolos, and dividing headlands. Unlike the Albion terraces, the Davenport terraces are not equal in extent; they become smaller from the first to fourth terrace decreasing in extent from 300 m (1000ʹ) at the first and second terraces, to 150 m (500ʹ) at the third terrace. While the lower three Navarro River terraces at Albion are each roughly 600 m (2,000ʹ) in extent, they do not decrease in overall dimension until the fourth and fifth terraces (). In general, however, in both areas the terraces decrease in overall area and horizontality as they ascend from the shore. This can be attributed to their greater exposure, decay, and erosion since uplift. Recent research has indicated that uplift in the Santa Cruz area has been steady over 250,000 years at roughly 1 mm/year, although it underwent a 40 mm uplift from the 1989 Loma Prieta Earthquake – the quake focus was only 19 miles away (Perg et al., Citation2001).
The fifth terraces in the Santa Cruz area were first estimated to be roughly 500,000 years old; however, recent studies have supported an uplift rate of 0.8–1.4 m/1,000 years (ka) (Perg et al., Citation2001; Shobe et al., Citation2020). Oddly enough sand dune ridges are not found above the second and third terraces in Davenport, unlike the Mendocino sites where dunal ridges have been identified at each terrace edge (Schulz et al., Citation2018; see , this article). Research estimates that the fifth terraces in Mendocino are 50k-100 K years older and 180–240 m (600ʹ-800ʹ asl) higher than their equivalent Davenport terraces to the south at 120–180 m (400ʹ-600ʹ asl) (cf., Merritts & Bull, Citation1989) confirming studies suggesting an active uplift of roughly 1 mm/year for both areas, in addition to tectonic flexures and catastrophic uplift from regional seismicity (Perg et al., Citation2001).
Figure 9. Image looking east, near Davenport, California (Santa Cruz County) showing the location and tiered sequence of the zero through fourth terraces. Note that the first and second terraces are used widely in the area for occupation and agriculture, while the third and fourth terraces are often forested and somewhat inaccessible. Fifth Terraces are often difficult to identify and observe since their greater exposure and increased weathering and erosion has altered their relatively flat morphologies and stair-step appearance. https://commons.wikimedia.org/wiki/File:Marine_terraces_California.jpg.
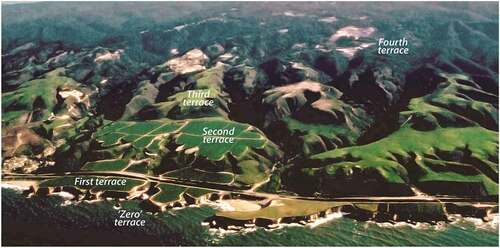
Tidal/wave-cut “mushroom” rocks
Mushroom-shaped rock pedestals (often referred to as “mushroom rocks” by local populations) represent notched, tidal/wave-cut phenomena that occur around the world in various sizes, though they are almost always found within a present-day intertidal and/or wave-action zone (). Notch development is well understood and has been discussed in prior studies (Terefenko & Terefenko, Citation2014; Trenhaile, Citation2014, Citation2015; Moses, Citation2013; Antonioli et al., Citation2015; in P. A. Pirazzoli & Evelpidou, Citation2013, specifically). Likewise, many studies exist on basic deterioration factors and notching processes including Spencer’s (Citation1985) study on Caribbean limestone reefs, Pirazzoli’s (Citation1986) work suggesting a decay rate of 1.0 mm/year in the Mediterranean, Evelpidou and Pirazzoli’s (Citation2016) use of fossil tidal notches in Greece, and Moses et al.'s (Citation2015) long-term study in Thailand. Efforts have also been made to model these processes with varying, yet regional successes, such as P. A. Pirazzoli and Evelpidou (Citation2013) refining a bioerosion model after Torunski’s (Citation1979) work, and Schneiderwind et al.’s (Citation2017) numerical modeling in the Mediterranean. Submerged tidal notch research has also seen a surge in recent years, mainly in the Mediterranean Basin where the majority of tidal/wave-cut notch research seems to occur (cf., Antonioli et al., Citation2018; Benac et al., Citation2004; Evelpidou & Pirazzoli, Citation2014; Evelpidou et al., Citation2015; Vaccher et al., Citation2019), although P. Pirazzoli and Montaggioni (Citation1988) conducted a study in French Polynesia related to sea level change and Trenhaile et al. (Citation2015) pursued research on cliff notches in Baja, Mexico. With respect to the Caribbean specifically, few notch studies have been conducted other than Lorscheid et al.’s (Citation2017) study on small storm-driven beached rocks, and Schubert and Valastro (Citation1976) brief mention of notches occurring off Venezuela’s northern coast.
Figure 10. Different mushroom rocks from around the world. a) Roca Seta, a sandstone mushroom rock along the west coast of Galiano Island, British Columbia, Canada. ~2 m in height. Source: used under creative commons from https://commons.wikimedia.org/wiki/File:Roca_Seta.JPG, photograph by Arrecifazo. b) Limestone mushroom rocks at Yehliu Geopark, Taiwan. Varying sizes from <1 m to >3 m heights. Source: used under creative commons license: https://commons.wikimedia.org/wiki/File:1_yehliu_2015_panorama.jpg. c) Limestone mushroom rocks next to Aoshima Island near Miyazaki, Japan (Kyushu), ~1-1.5 m in height. A tombolo formed on raised and tilted limestone, Aoshima island is also known as the “Ogre’s Washboard” or “Devil’s Washboard”. Photograph by C.D. Allen, Citation2019. d) Kannesteinen Rock off the coast of Vågsøy, Norway. Eclogite (gneiss), ~3 m at its tallest and ~3 m at its widest. Source: used under creative commons license: https://commons.wikimedia.org/wiki/File:Kannesteinen_Rock_Nordfjord.jpg.
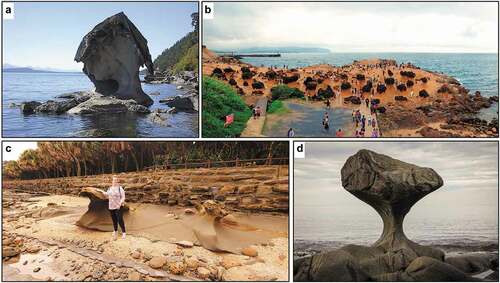
The Barbados link: sea level change and paleoenvironment reconstruction
At first glance, Barbados’ geology seems straightforward: some modern beach deposits and younger, uplifted coral limestone layers (Quaternary) surrounding older, mixed oceanic rocks and jumbled sandstones (Eocene-Oligocene) (). Modern beaches are found mainly along the south and east coasts, with some especially long stretches of beach on the west coast, and a few smaller beaches in the northeast. Although discontinuous cliff and terrace features can be seen around the island, two coral rock terraces are marked by noticeable topographic breaks: the First High Cliff with elevations of approximately 30–60 m above sea level (asl) and dated to ~125,000 years ago, and the Second High Cliff, which stretches in elevation from about 70–335 m asl, with its highest point, Mt. Hillaby (340 m), dated to ~640,000 years ago (Cook & Abbott, Citation2011; Donovan & Haprer, Citation2005). On the eastern side, a triangular-like shaped region called the Scotland District represents a mix of sandstones, shales, and marls of various dates (Eocene to Quaternary), likely formed from deep ocean sediments carried from South America that were subsequently pushed up against the uplifting and already-uplifted limestone (Cook & Abbott, Citation2011; Donovan & Haprer, Citation2005; Poole & Barker, Citation1983).
Figure 11. Map representing the main geologic divisions with mushroom rock locations on Barbados. An uplifted accretionary prism of turbidite, (mostly coral) limestone, and various sandstones, resulted in four main regions: current beaches/coasts (dated to ~82,000 ya), first high-cliff (dated to ~125,000 ya), the second high cliff (~640,000 ya at Mt. Hillaby), and the Scotland District (mixed rocks of mixed ages, mostly non-limestone groups, basement rocks formed in deep ocean ~44 mya), see Schellmann and Radtke (Citation2004), Schellmann et al. (Citation2004), Radtke and Schellmann (Citation2005), and Radtke and Schellmann (Citation2006), and Limonta et al. (Citation2015). Map by K.M. Groom.
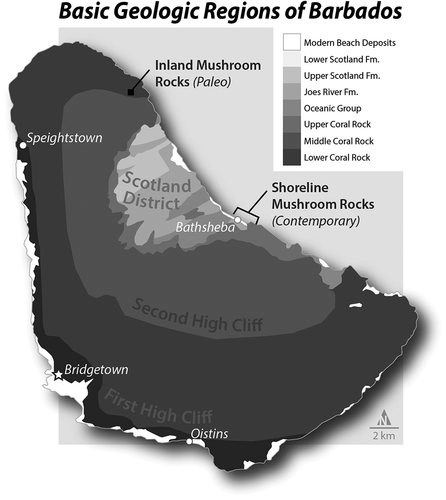
Interestingly, many previous tidal/wave-cut notch studies remain focused on sea level change and paleoclimate reconstruction, something that Pirazzoli and Evelpidou (Citation2013) cautioned against in previous years. Regardless, it is possible for tidal notches to be used as Pleistocene sea level indicators if certain coral (fossil) species are present (Radtke & Schellmann, Citation2005), and several such studies have been conducted in Barbados specifically (Radtke & Schellmann, Citation2006; Schellmann & Radtke, Citation2004; Schellmann et al., Citation2004). In fact, Pleistocene dating of Barbados’ exposed coral reefs – including a few notches, but oddly not mushroom rocks – proved invaluable in helping confirm Milankovich cycle activity, and Barbados remains perhaps one of the best locations “ … to determine the history of sea-level rise and fall during the last 700,000 years” (Cook & Abbott, Citation2011: 54).
Tidal/Wave-cut notches remain scattered around Barbados, both inland and along the current shoreline (cf., Schellmann & Radtke, Citation2004 and , this article). On the east coast beaches, mushroom rocks are prevalent, with many found near/at the Bathsheba area. Although currently found on the beach, these contemporary mushroom rocks likely have their origins in Hackleton’s Cliff (Second High Cliff edge above Bathsheba, see Cook & Abbott, Citation2011), based on their coral-rich limestone geology (). This means that mushroom rock genesis across Barbados is different than traditional coastal wave-cut and tidal sea stack/stump formation, since they do not represent former coastline and/or terraces. Instead, Barbados’ mushroom rocks likely ended up beachside due to mass wasting events, given that the Scotland District sees high seasonal rainfall and contains soft, oily clays, leaving the region prone to landslides, block glides, and topples (Poole & Barker, Citation1983). Such conditions lead to large limestone blocks detaching from the Second High Cliff (above Bathsheba), and “ … easily sliding down over the greasy oceanic clays” (Poole & Barker, Citation1983: Stratigraphy). Still, regardless of how the rocks ended up on the beach/shore, once there, the processes that turn them into mushroom rocks remain different from “regular” wave-cut notches. Subsequent notching of these rocks was created via different bioerosion processes that include not just wave action, but (mainly) algae-feeding invertebrates (P. A. Pirazzoli & Evelpidou, Citation2013, especially ; Cook & Abbott, Citation2011; Donovan & Haprer, Citation2005).
Figure 12. Mushroom rocks along Barbados east coast: a) large mushroom rock just 20 meters down the hill from Dina’s Bar next to Bathsheba Park, and b) Looking northwest along Long Beach, just south of Bathsheba with many mushroom rocks visible along the shore. Photographs by C.D. Allen, 2018.
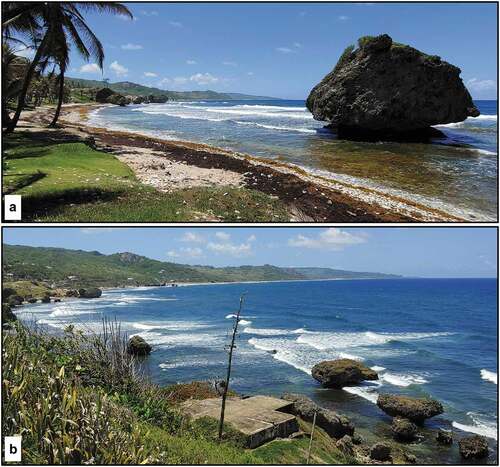
Mushroom rocks on the east coast share similar size, shape, and notch features with the identified inland mushroom rock (see for comparisons of contemporary mushroom rock with paleo-mushroom rock, respectively). Across Barbados, to date, one confirmed inland mushroom rock has been located near the base of the Second High Cliff adjacent to Pie Corner and Cave Hill in St. Lucy Parish (). The area called Cave Hill – not to be confused with Cave Hill on the west coast, north of Bridgetown – derives its name from the abundant (paleo-sea) caves around the base of Second High Cliff, some of which are inland notches (but not mushroom rocks) and further suggest that the inland mushroom rock was once beachside. Indeed, nearby shore areas exhibit similar landforms as those behind the inland mushroom rock, even though the inland mushroom rock sits on a ~ 125,000 year-old terrace set about 20 m higher in elevation and approximately 500 m inland from the current (sea-level) terrace and beaches. Further evidence of this paleo-mushroom rock rests in its orientation to the current shoreline, as clearly visible paleo-wave action indication can be found on only one of its facet junctions (). The search for more examples of paleo-mushroom rocks remains ongoing, as others likely exist – in Barbados and other locations around the world – given their similarities with inland/uplifted wave-cut/tidal notching.
Figure 13. North aspect (a1) and its measurements (a2), West aspect (b1) and its measurements (b2), and Southwest aspect (c1) and its measurements (c2) of representative contemporary mushroom rock at Bathsheba, Barbados. Photographs by C.D. Allen, 2018. Measurement graphics by K.M. Groom.
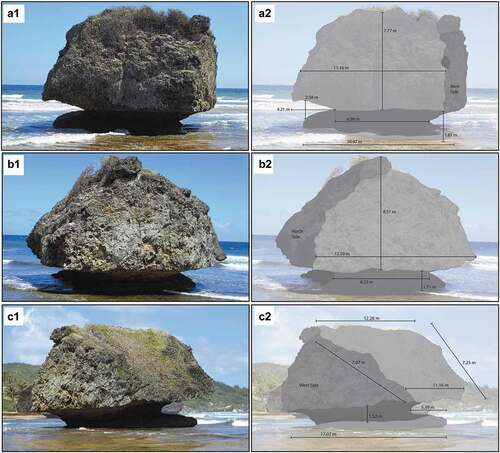
Figure 14. North-northwest aspect (a1) and its measurement (a2), southeast aspect (b1) and its measurement (b2), and west aspect (c1) and its measurments (c2) of paleo-mushroom rock in St. Lucy Parish, Barbados. See for location. Photographs by C.D. Allen, 2018. Measurement graphics by K.M. Groom.
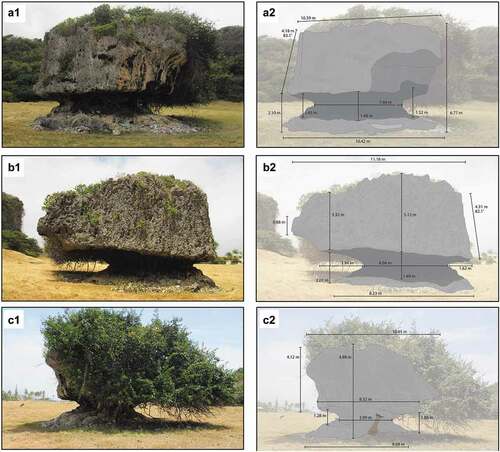
Figure 15. An aerial image showing confirmed inland mushroom rock (“Paleo Mushroom Rock”) and two “Potential Paleo Mushroom Rocks”, with distance from confirmed paleo-mushroom rock to current shoreline. Note the beginning of the Second High Cliff directly south of, and approximately 20 meters higher, than the inland mushroom rocks, which presumably represents the mushroom rocks’ source (i.e., from a rockfall/topple mass wasting event), as the entire land area to the north and east of the Second High Cliff is a wave-cut platform dated to ~125,000 years ago (cf., Cook & Abbott, Citation2011; Radtke & Schellmann, Citation2005, Citation2006; Schellmann & Radtke, Citation2004; Schellmann et al., Citation2004). Image source: Google Earth. Map by K.M. Groom.
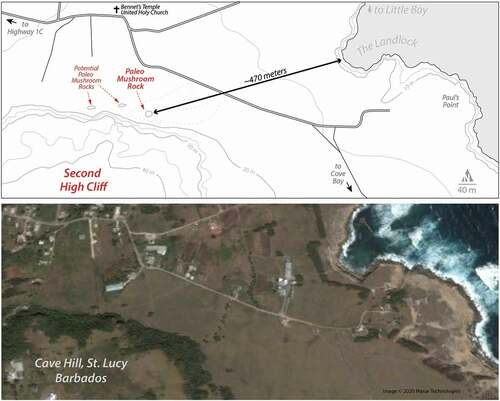
Figure 16. The confirmed inland tidal/wave-cut notch rock (“mushroom rock”) in St. Lucy Parish. This rock would have fallen from the Second High Cliff, perhaps more than 20 m up, just behind it, much like the current coastal mushroom rocks along Long Beach and Bathsheba (see ). The person in the image, roughly 1.5 m in height, is demonstrating the paleo wave-action that, alongside bioerosive processes that include algae-eating invertebrates, helped create this mushroom rock over many centuries. Looking at the underside of this section, clearly etched wave-cut patterns can be seen that line-up azimuthally with the ocean, and where the waves would have crashed into this rock. In other words, wave direction remains clearly visible on this section of the rock. Photograph by C.D. Allen, 2018.
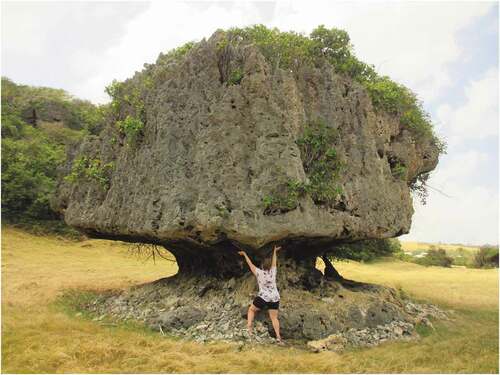
Despite their inherent size and accessibility, however, it seems odd that no specific research yet exists on mushroom rocks in the Caribbean specifically, let alone (large) inland mushroom rocks like the specimen found on Barbados. Though undoubtedly a rare geomorphologic relict landform, inland mushroom rocks could potentially be used as a new/corroborative method for assessing Pleistocene sea level fluctuations – perhaps employing dating techniques such as those Perg et al. (Citation2001) used to enhance understanding of past climates on California’s marine terraces. With any luck, and with more field time to explore, further such studies will yield promising results.
Conclusion
Relict landscapes, like the mushroom rocks of the Barbados (cf., ) or the coastal terraces of Northern California (cf., ), represent features of extraordinary natures and morphologies that remain a function of differing rates of (dis)continuous uplift, erosion, deposition, and/or catastrophic ruin. Around the world, over the past 700,000 years as sea levels fluctuated regionally, and with flexing, torqueing, and rising tectonic plates, coastal wave-cut platforms were lifted and melded into distinctive stair-step sequences that ascend from current coastlines. Relict features on these terraces, such as mushroom rocks or pygmy forests, represent important characteristics that help fuel our ever-growing understanding of coastal geomorphology, sea level fluctuation, paleoclimatology, rock decay (weathering) studies, and geo-tectonics, while twisting and often confounding our knowledge of the interaction of polygenetic landscape development. Relict landform research that focuses on one influence is increasingly difficult to address and often myopic in geomorphic studies, whereas the emergent and exciting research on polygenetic landscape influences and transformation remains driven by our identification, examination, analysis, and passion of these fascinating relict features, phenomena, and landforms.
References
- Allen, C. D. (2005). Micrometeorology of a smooth and rugose biological soil crust near coon bluff, Arizona. Journal of the Arizona-Nevada Academy of Science, 38(1), 21–28. https://doi.org/https://doi.org/10.2181/1533-6085(2005)038[0021:MOASAR]2.0.CO;2
- Allen, C. D. (2012). Los Lavadores: Discovering place in the Andes. Geographical Review, 102(3), 364–371. https://doi.org/https://doi.org/10.1111/j.1931-0846.2012.00157.x
- Allen, C. D. (ed). (2017). Landscapes and Landforms of the Lesser Antilles. Springer.
- Allen, C. D. (2019). Jordan’s forgotten Eastern Desert Qasuur. FOCUS on Geography, 62(2). https://doi.org/https://doi.org/10.21690/foge/2019.62.2p
- Antonioli, F., Ferranti, L., Stocchi, P., Deiana, G., Presti, V. L., Furlani, S., Marino, C., Orru, P., Scicchitano, G., Trainito, E., Anzidei, M., Bonamini, M., Sansò, P., & Mastronuzzi, G. (2018). Morphometry and elevation of the last interglacial tidal notches in tectonically stable coasts of the mediterranean sea. Earth-Science Reviews, 185, 600–623. https://doi.org/https://doi.org/10.1016/j.earscirev.2018.06.017
- Antonioli, F., Presti, V. L., Rovere, A., Ferranti, L., Anzidei, M., Furlani, S., Mastronuzzi, G., Orru, P. E., Scicchitano, G., Sannino, G., Spampinato, C. R., Pagliarulo, R., Deiana, G., De Sabata, E., Sansò, P., Vacchi, M., & Vecchio, A. (2015). Tidal notches in mediterranean sea: A comprehensive analysis. Quaternary Science Reviews, 119, 66–84. https://doi.org/https://doi.org/10.1016/j.quascirev.2015.03.016
- Benac, Č., Juračić, M., & Bakran-Petricioli, T. (2004). Submerged tidal notches in the Rijeka Bay NE Adriatic sea: Indicators of relative sea-level change and of recent tectonic movements. Marine Geology, 212(1–4), 21–33. https://doi.org/https://doi.org/10.1016/j.margeo.2004.09.002
- Budanov, L., Sergeev, A. Y., Neevin, I., & Moskovtsev, A. 2018. Study of modern and relict coastal landforms in the Eastern part of Gulf of Finland with GPR. Paper read at Engineering and Mining Geophysics 2018.
- Büdel, J. (1973). Climatogenetic Geomorphology. In E. Derbyshire, (Ed.), Climatic geomorphology (pp. 202–227). UK: Macmillan Education.
- Buynevich, I. V., Bitinas, A., & Pupienis, D. (2007). Lithological anomalies in a relict coastal dune: Geophysical and paleoenvironmental markers. Geophysical Research Letters, 34(9). https://doi.org/https://doi.org/10.1029/2007GL029767
- Clarhäll, A., & Kleman, J. (1999). Distribution and glaciological implications of relict surfaces on the Ultevis plateau, northwestern Sweden. Annals of Glaciology, 28, 202–208. https://doi.org/https://doi.org/10.3189/172756499781821599
- Colucci, R. R., Forte, E., Žebre, M., Maset, E., Zanettini, C., & Guglielmin, M. (2019). Is that a relict rock glacier? Geomorphology, 330, 177–189. https://doi.org/https://doi.org/10.1016/j.geomorph.2019.02.002
- Cook, T., & Abbott, L. (2011). Barbados: Ascending the seal-level staircase. EARTH Magazine, 56(12), 54–59.
- Crump, S. (1998). Redwoods, iron horses, and the Pacific: The story of the California Western Skunk Railroad (5th ed.). California Western Railroad.
- DeBoer, D. H. (1992). Hierarchies and spatial scale in process geomorphology: A review. Geomorphology, 4(5), 303–318. https://doi.org/https://doi.org/10.1016/0169-555X(92)90026-K
- Derbyshire, E. D. (1973). Climatic geomorphology. Macmillan International Higher Education.
- Donovan, S. K., & Haprer, D. A. T. (2005). The geology of Barbados: A field guide. Caribbean Journal of Earth Science, 38, 21–33. https://www.mona.uwi.edu/geoggeol/JamGeolSoc/CJES%20Web%20page/CJESpdf/CJES%2038-3%20-%20Donovan.pdf
- Dorn, R. I., Gordon, S. J., Allen, C. D., Cerveny, N., Dixon, J. C., Groom, K. M., Hall, K., Harrison, E., Mol, L., Paradise, T. R., Sumner, P., Thompson, T. J., & Turkington, A. V. (2013). The role of fieldwork in rock-decay research: Case studies from the Fringe. Geomorphology, 200, 59–74. https://doi.org/https://doi.org/10.1016/j.geomorph.2012.12.012
- DuBois, J. D., & Kapustka, L. A. (1983). Biological nitrogen influx in an Ohio relict prairie. American Journal of Botany, 70(1), 8–16. https://doi.org/https://doi.org/10.1002/j.1537-2197.1983.tb12426.x
- Evelpidou, N., & Pirazzoli, P. A. (2014). Holocene relative sea-level changes from submerged tidal notches: A methodological approach. Quaternaire. Revue de l’Association française pour l’étude du Quaternaire, 25(4), 313–320. https://doi.org/https://doi.org/10.4000/quaternaire.7688
- Evelpidou, N., & Pirazzoli, P. A. (2016). Estimation of the intertidal bioerosion rate from a well-dated fossil tidal notch in Greece. Marine Geology, 380, 191–195. https://doi.org/https://doi.org/10.1016/j.margeo.2016.04.017
- Evelpidou, N., Pirazzoli, P. A., & Spada, G. (2015). Origin and Holocene evolution of a slightly submerged tidal notch in the NE Adriatic. Journal of Coastal Research, 31(2), 255–264. https://doi.org/https://doi.org/10.2112/JCOASTRES-D-14-00016.1
- Fox, W. W. (1976). Pygmy forest: An ecologic staircase. California Geology, 29(1), 3–7. http://www.jughandlecreekfarm.org/wp-content/uploads/2010/07/JHC_staircase_article.pdf
- Frauenfelder, R., & Kääb, A. (2000). Towards a palaeoclimatic model of rock-glacier formation in the Swiss Alps. Annals of Glaciology, 31, 281–286. https://doi.org/https://doi.org/10.3189/172756400781820264
- Fredin, O., & Hättestrand, C. (2002). Relict lateral moraines in northern Sweden—evidence for an early mountain centred ice sheet. Sedimentary Geology, 149(1–3), 145–156. https://doi.org/https://doi.org/10.1016/S0037-0738(01)00249-4
- Grandcolas, P., Nattier, R., & Trewick, S. (2014). Relict species: A relict concept? Trends in Ecology & Evolution, 29(12), 655–663. https://doi.org/https://doi.org/10.1016/j.tree.2014.10.002
- Habel, J. C., Assmann, T., Schmitt, T., & Avise, J. C. (2010). Relict species: From past to future. In J. C. Habel & T. Assmann (Eds.), Relict species: Phylogeography and conservation biology (pp. 1–5). Springer.
- Jenny, H. (1973). Pygmy forest, an ecologic staircase: Description and interpretation. University of California.
- Jenny, H., Arkley, R. J., & Schultz, A. (1969). The pygmy forest-podsol ecosystem and its dune associates of the Mendocino coast. Madroño, 20(2), 60–74. https://www.jstor.org/stable/41423345
- Kofler, C., Steger, S., Mair, V., Zebisch, M., Comiti, F., & Schneiderbauer, S. (2020). An inventory-driven rock glacier status model (intact vs. relict) for South Tyrol, Eastern Italian Alps. Geomorphology, 350, 106887. https://doi.org/https://doi.org/10.1016/j.geomorph.2019.106887
- Lericolais, G., Popescu, I., Guichard, F., & Popescu, S. M. (2007). A Black Sea lowstand at 8500 yr BP indicated by a relict coastal dune system at a depth of 90 m below sea level. Special Papers-Goelogical Society of America, 426, 171–188. https://doi.org/https://doi.org/10.1130/2007.2426(12)
- Leslie, D., & McGlone, M. (1973). Relict periglacial landforms at clarks junction, Otago. New Zealand Journal of Geology and Geophysics, 16(3), 575–583. https://doi.org/https://doi.org/10.1080/00288306.1973.10431380
- Limonta, M., Garzanti, E., Resentini, A., Andò, S., Boni, M., & Bechstädt, T. (2015). Multicyclic sediment transfer along and across convergent plate boundaries (Barbados, Lesser Antilles). Basin Research, 27(6), 696–713.
- Lorscheid, T., Felis, T., Stocchi, P., Obert, J. C., Scholz, D., & Rovere, A. (2017). Tides in the last interglacial: Insights from notch geometry and palaeo tidal models in Bonaire, Netherland Antilles. Scientific Reports, 7(1), 1–9. https://doi.org/https://doi.org/10.1038/s41598-017-16285-6
- Matthews, R. A. (1972). Mendocino’s Pygmy forest, a priceless and unique portion of California’s ecology. In E.M. Moores & R.A. Matthews, (Eds.), Geologic guide to the Northern Coast Ranges: Lake, Mendocino and Sonoma Counties, California Annual Field Trip Guide Book, (pp. 57–70). Geological Society of Sacramento.
- Merritts, D., & Bull, W. B. (1989). Interpreting quaternary uplift rates at the Mendocino triple junction, northern California, from uplifted marine terraces. Geology, 17(11), 1020–1024. https://doi.org/https://doi.org/10.1130/0091-7613(1989)017<1020:IQURAT>2.3.CO;2
- Milne, R. I., & Abbott, R. J. 2002. The origin and evolution of Tertiary relict floras. Elsevier.
- Moses, C., Robinson, D., Kazmer, M., & Williams, R. (2015). Towards an improved understanding of erosion rates and tidal notch development on limestone coasts in the Tropics: 10 years of micro‐erosion meter measurements, Phang Nga Bay, Thailand. Earth Surface Processes and Landforms, 40(6), 771–782. https://doi.org/https://doi.org/10.1002/esp.3683
- Moses, C. A. (2013). Tropical rock coasts: Cliff, notch and platform erosion dynamics. Progress in Physical Geography: Earth and Environment, 37(2), 206–226. https://doi.org/https://doi.org/10.1177/0309133312460073
- Orme, A. J., & Orme, A. R. (1991). Relict barrier beaches as paleoenvironmental indicators in the California desert. Physical Geography, 12(4), 334–346. https://doi.org/https://doi.org/10.1080/02723646.1991.10642437
- Orme, A. R. (1980). Marine terraces and quaternary tectonism, Northwest Baja California, Mexico. Physical Geography, 1(2), 138–161. https://doi.org/https://doi.org/10.1080/02723646.1980.10642196
- Orme, A. R. (1988). Coastal dunes, changing sea level, and sediment budgets. Journal of Coastal Research, 3, 127–129. https://www.jstor.org/stable/40928739
- Orme, A. R. (1989). The nature and rate of alluvial fan aggradation in a humid temperate environment, northwest Washington. Physical Geography, 10(2), 131–146. https://doi.org/https://doi.org/10.1080/02723646.1989.10642373
- Paradise, T. R. (1995). Sandstone weathering thresholds in Petra, Jordan. Physical Geography, 16(3), 205–222. https://doi.org/https://doi.org/10.1080/02723646.1995.10642550
- Paradise, T. R. (2005). Petra revisited: An examination of sandstone weathering research in Petra, Jordan. Geological Society of America Special Papers, 390, 39–49.
- Paradise, T. R. (2010). Sandstone chamber humidity and tourism in Petra, Jordan. Journal of Architectural Conservation, 16(2), 65–81. https://doi.org/https://doi.org/10.1080/13556207.2010.10785070
- Paradise, T. R. (2012). The great flood of Petra: Evidence for a 4th-5th AD century catastrophic flood. Annual of the Jordan Department of Antiquities, 56(1), 143–158. http://publication.doa.gov.jo/uploads/publications/45/ADAJ_2011_55-43-56.pdf
- Perg, L., Anderson, R., & Finkel, R. (2001). Use of a new 10Be and 26Al inventory method to date marine terraces, Santa Cruz, California, USA. Geology, 29(10), 879–882. https://doi.org/https://doi.org/10.1130/0091-7613(2001)029<0879:UOANBA>2.0.CO;2
- Phillips, J. D. (1999). Earth surface systems: Complexity, order, and scale. Blackwell.
- Phillips, J. D. (2001). Methodology, scale and the field of dreams. Annals of the Association of American Geographers, 91(4), 754–760. https://doi.org/https://doi.org/10.1111/0004-5608.00175
- Pirazzoli, P. (1986). Marine notches. In O. van de Plassche, (Ed.), Sea-level research (pp. 361–400). Springer. https://doi.org/https://doi.org/10.1007/978-94-009-4215-8_12
- Pirazzoli, P., & Montaggioni, L. (1988). Holocene sea-level changes in French Polynesia. Palaeogeography, Palaeoclimatology, Palaeoecology, 68(2–4), 153–175. https://doi.org/https://doi.org/10.1016/0031-0182(88)90037-5
- Pirazzoli, P. A., & Evelpidou, N. (2013). Tidal notches: A sea-level indicator of uncertain archival trustworthiness. Palaeogeography, Palaeoclimatology, Palaeoecology, 369, 377–384. https://doi.org/https://doi.org/10.1016/j.palaeo.2012.11.004
- Poole, E. G., & Barker, L. H. (1983). Geology of Barbados. Ordnance Survey.
- Radtke, U., & Schellmann, G. (2005). Timing and magnitude of sea level change during MIS 5 derived from Barbados coral reef terraces: A critical literature review and new data. Journal of Coastal Research, (42), 52–62. https://www.jstor.org/stable/pdf/25736973.pdf
- Radtke, U., & Schellmann, G. (2006). Uplift history along the clermont nose traverse on the west coast of Barbados during the last 500,000 years—Implications for Paleo–Sea level reconstructions. Journal of Coastal Research, 22(2 (222)), 350–356. https://doi.org/https://doi.org/10.2112/05-0439.1
- Reimnitz, E., & Wolf, S. C. (1998). Are North Slope surface alluvial fans pre-Holocene relicts? US Government Printing Office.
- Ross, N., Brabham, P., & Harris, C. (2019). The glacial origins of relict ‘pingos’, Wales, UK. Annals of Glaciology, 60(80), 138–150. https://doi.org/https://doi.org/10.1017/aog.2019.40
- Sauchyn, D. J. (1990). A reconstruction of holocene geomorphology and climate, western Cypress Hills, Alberta and Saskatchewan. Canadian Journal of Earth Sciences, 27(11), 1504–1510. https://doi.org/https://doi.org/10.1139/e90-160
- Schellmann, G., & Radtke, U. (2004). A revised morpho- and chronostratigraphy of the late and middle pleistocene coral reef terraces on Southern Barbados (West Indies). Earth-Science Reviews, 64(3–4), 157–187. https://doi.org/https://doi.org/10.1016/S0012-8252(03)00043-6
- Schellmann, G., Radtke, U., Potter, E.-K., Esat, T., & McCulloch, M. (2004). Comparison of ESR and TIMS U/Th dating of marine isotope stage (MIS) 5e, 5c, and 5a coral from Barbados—implications for palaeo sea-level changes in the Caribbean. Quaternary International, 120(1), 41–50. https://doi.org/https://doi.org/10.1016/j.quaint.2004.01.005
- Schneiderwind, S., Boulton, S., Papanikolaou, I., Kázmér, M., & Reicherter, K. (2017). Numerical modeling of tidal notch sequences on rocky coasts of the Mediterranean Basin. Journal of Geophysical Research: Earth Surface, 122(5), 1154–1181. https://doi.org/https://doi.org/10.1002/2016JF004132
- Schubert, C., & Valastro, S., Jr. (1976). Quaternary geology of La Orchila Island, central Venezuelan offshore, Caribbean sea. Geological Society of America Bulletin, 87(8), 1131–1142. https://doi.org/https://doi.org/10.1130/0016-7606(1976)87<1131:QGOLOI>2.0.CO;2
- Schulz, M. S., Lawrence, C., Muhs, D. R., Prentice, C. S., & Flanagan, S. (2018). Landscapes from the waves—Marine terraces of California. US Geological Survey.
- Selley, H., Harrison, S., Glasser, N., Wündrich, O., Colson, D., & Hubbard, A. (2019). Rock glaciers in central Patagonia. Geografiska Annaler: Series A, Physical Geography, 101(1), 1–15. https://doi.org/https://doi.org/10.1080/04353676.2018.1525683
- Shaw, B. D. (1976). Climate, environment and prehistory in the Sahara. World Archaeology, 8(2), 133–149. https://doi.org/https://doi.org/10.1080/00438243.1976.9979659
- Shobe, C., Bennet, G., Tucker, G., Roback, K., Miller, S., & Roering, J. (2020). Boulders as a lithologic control on river and landscape response to tectonic forcing at the Mendocino triple junction. Geological Society of America (GSA) Bulletin, 133(3-4), 647-662. https://doi.org/https://doi.org/10.1130/B35385.1
- Simms, A. R., Rouby, H., & Lambeck, K. (2016). Marine terraces and rates of vertical tectonic motion: The importance of glacio-isostatic adjustment along the Pacific coast of central North America. Geological Society of America (GSA)Bulletin, 128(1–2), 81–93. https://doi.org/https://doi.org/10.1130/B31299.1
- Spencer, T. (1985). Weathering rates on a Caribbean reef limestone: Results and implications. Marine Geology, 69(1–2), 195–201. https://doi.org/https://doi.org/10.1016/0025-3227(85)90142-2
- Starobinskii, A. (1979). Spectrum of relict gravitational radiation and the early state of the universe. JETP Letters, 30(11), 682–685. http://www.astrosurf.com/luxorion/Physique/starobinsky-inflation-jetp-1979.pdf
- Stratford, V. C. (1999). Coastal landforms and relict features of Lake Bonneville-A literature review. In C. J. Allen & L. E. Spangler (Eds.), Geology of Northern Utah and Vicinity (pp. 367–377). Utah Geological Association.
- Stuchlík, Z. (2005). Influence of the relict cosmological constant on accretion discs. Modern Physics Letters A, 20(8), 561–575. https://doi.org/https://doi.org/10.1142/S0217732305016865
- Terefenko, P., & Terefenko, O. (2014). Determining the role of exposure, wave force, and rock chemical resistance in marine notch development. Journal of Coastal Research, 70, 706–711. https://doi.org/https://doi.org/10.2112/SI70-105a.1
- Torunski, H. (1979). Biological erosion and its significance for the morphogenesis of limestone. Senckenbergiana Maritima 11(3–6): 193–265.
- Trenhaile, A. S. (2014). Modelling tidal notch formation by wetting and drying and salt weathering. Geomorphology, 224, 139–151. https://doi.org/https://doi.org/10.1016/j.geomorph.2014.07.014
- Trenhaile, A. S. (2015). Coastal notches: Their morphology, formation, and function. Earth-Science Reviews, 150, 285–304. https://doi.org/https://doi.org/10.1016/j.earscirev.2015.08.003
- Trenhaile, A. S., Gagnon, J., Prestanski, K. J., & Porter, N. I. (2015). Cliff notch formation along the sub-tropical La Paz Peninsula, Southern Baja California, Mexico. Marine Geology, 368, 15–24. https://doi.org/https://doi.org/10.1016/j.margeo.2015.06.009
- Vaccher, V., Muro, F., Antonioli, F., Taviani, M., Dean, S., De Sabata, E., Biolchi, S., & Furlani, S. 2019. Tidal notches on the rock coast of the Ansedonia promontory (Tyrrhenian Sea, Italy). Paper read at Geophysical Research abstracts.
- Viles, H. (1987). Coastal landforms. Progress in Physical Geography: Earth and Environment, 11(3), 398–405. https://doi.org/https://doi.org/10.1177/030913338701100306
- Wagner, T., Pauritsch, M., Mayaud, C., Kellerer-Pirklbauer, A., Thalheim, F., & Winkler, G. (2019). Controlling factors of microclimate in blocky surface layers of two nearby relict rock glaciers (Niedere Tauern Range, Austria). Geografiska Annaler: Series A, Physical Geography, 101(4), 310–333. https://doi.org/https://doi.org/10.1080/04353676.2019.1670950
- Wilson, P. (2004). Relict rock glaciers, slope failure deposits, or polygenetic features? A re‐assessment of some Donegal debris landforms. Irish Geography, 37(1), 77–87. https://doi.org/https://doi.org/10.1080/00750770409555830
- Wilson, P., Matthews, J. A., Mourne, R. W., Linge, H., & Olsen, J. (2020). Interpretation, age and significance of a relict paraglacial and periglacial boulder-dominated landform assemblage in Alnesdalen, Romsdalsalpane, southern Norway. Geomorphology, 369, 107362. https://doi.org/https://doi.org/10.1016/j.geomorph.2020.107362
- Winker, C. D., & Howard, J. D. (1977). Plio-pleistocene paleogeography of the Florida Gulf Coast interpreted from relict shorelines (1). Transactions of Gulf Coast Association of Geological Societies, 27, 409–420.
- Wolman, M. G., & Gerson, R. (1978). Relative scales of time and effectiveness of climate in watershed geomorphology. Earth Surface Processes, 3(2), 189–208. https://doi.org/https://doi.org/10.1002/esp.3290030207