ABSTRACT
Nature-based solutions are seen to benefit both society and biodiversity. However, research into their future resilience is required. Soft capping is a nature-based conservation strategy that mimics the natural colonisation of plants on top of ruined walls and reduces rates of material deterioration. To remain effective, soft capping species must be tolerant of future climatic conditions. We use the Maxent species distribution model to assess the resilience of soft capping species to low and high emission scenarios across Britain and Ireland. We assess the mid- and end-century presence probability of four native and archaeophyte species used in soft capping (Sedum album, S. acre, S. anglicum and Saxifraga granulata). Future presence probabilities were calculated using the climate models HadGEM3-GC31-LL, IPSL-CM6A-LR and MIROC6. Results suggest that current sedum-based soft caps will remain viable until mid-century with additional maintenance (e.g. watering) during droughts, although soft caps predominantly formed of Sedum album may be prone to failure in south-eastern England. In the future, species more resilient to arid conditions may need to be preferentially selected for soft capping to ensure resilience under a warming climate. Species distribution modelling provides a useful way of predicting the future resilience of nature-based solutions.
Introduction
Nature-based solutions (NbS) are increasingly being deployed by environmental managers and policymakers. NbS are actions that work with nature to address societal challenges, and involve the protection, restoration or management of natural and semi-natural ecosystems; the sustainable management of aquatic, agricultural and forest ecosystems, and the creation of novel urban ecosystems (Seddon, Citation2022). Examples of NbS include the creation of forests and parks in urban areas to enhance cooling and reduce flood risk. NbS provide win-win solutions that benefit both society and biodiversity, and have been proposed as being beneficial for the conservation of historic sites (Coombes & Viles, Citation2021). However, to continue being successful, NbS must be resilient to current and future environmental changes. This raises the important question of if, and how, we can future prove NbS over the coming century.
Since the 1980s, the potential for plants to act as potential agents of NbS at historic sites, rather than as sources of deterioration and damage, has been increasingly recognised. In particular, the plants have been utilised at various ruined historic masonry sites, such as abbeys, castles and monasteries, to reduce the rate of material deterioration on the tops of ruined walls (Historic Environment Scotland, Citation2014a; Wood et al., Citation2018). The presence of plants forming a “soft cap” on ruined wall heads has been found to (i) reduce temperature fluctuations and the number of freeze–thaw cycles by providing a thermal blanket, (ii) reduce water ingress into the wall head and (iii) minimise water runoff down the wall face by enhancing absorption capabilities (Hanssen & Viles, Citation2014; Lee et al., Citation2009; Viles & Wood, Citation2007). Soft capping can also enhance local biodiversity at heritage sites and represents one of the first and most successful examples of NbS for heritage conservation (Coombes & Viles, Citation2021; Miller, Citation2019). The comparative cheapness, ease of installation, minimal maintenance requirement and reversibility of this strategy mean that soft capping is now widely recommended, particularly in Britain, as shown in , which depicts documented examples of soft capping trials and projects. Heritage organisations such as Historic England (Wood et al., Citation2018) and Historic Environment Scotland (Historic Environment Scotland, Citation2014a, Citation2014b) advocate this method of conservation at sites where biological growth is not considered to negatively impact the aesthetic value of the site. However, the long-term success of soft capping relies on the environmental conditions being conducive to the survival of the plant species growing in the soft capping.
Figure 1. Map showing the location and nature of 84 documented soft capping projects and trials, with inset map of sites in Britain and Ireland. Two notable natural soft cap growth sites are also included.
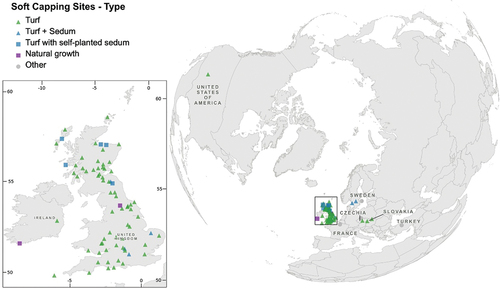
In England, trials of capping using mats of sedum plants (Sedum L.) have had some success in providing a self-sustaining soft capping (e.g. ) following their widespread use in green roofs, and sedum plugs have been widely used around the edges of turf-based soft capping to reduce soil loss down the wall face (e.g. ; Historic Environment Scotland, Citation2014b; Wood et al., Citation2018). Many naturally occurring soft caps, which have grown up over several centuries, have also been found to contain sedums (Historic Environment Scotland, Citation2014b). Sedums are used in green roofs due to their ability to survive severe environmental stress (Durhman et al., Citation2007; Nagase & Dunnett, Citation2010; Rowe et al., Citation2006; Vijayaraghavan, Citation2016); they are able to tolerate drought conditions due to their succulent properties, thick waxy leaves and Crassulacean acid metabolism (CAM) photosynthetic pathway, which minimises water loss. While their physical characteristics mean they may be resilient to warm and dry climates, the tolerance of the sedum species used in soft capping to anthropogenic climate change over medium and long timescales, and how this varies spatially, needs to be better understood. This is essential for informing the design and maintenance of soft caps to ensure long-term resilience.
Figure 2. Sedum-based soft capping on masonry at (a) Godstow Abbey, Wolvercote, Oxford, England (Listed Grade II) installed in 2009 using sedum matting and (b) test walls in Wytham Woods, Oxfordshire, England, where sedum plugs were installed in 2008 around the cap edge (Wood et al., Citation2018). Areas primarily populated with sedums are highlighted with white dashed lines.
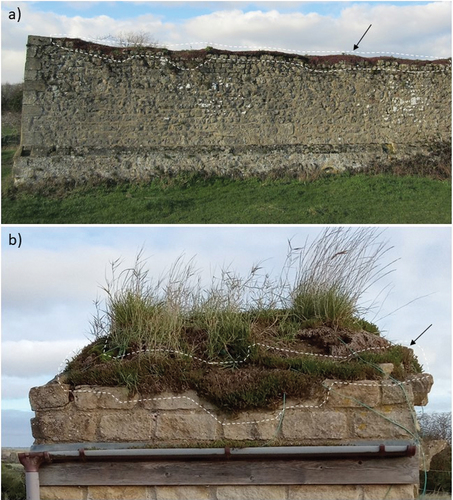
Modelling approaches enable us to assess process interactions over timescales not feasible under field or laboratory conditions (Richards & Brimblecombe, Citation2022b). In particular, species distribution models (SDMs) are commonly used to assess the ability of species to survive under future climate conditions. These models use present-day distribution data with environmental parameters (typically abiotic, such as temperature and moisture) to assess the spatial ranges suitable for species under different climate scenarios (Lissovsky et al., Citation2021). This method has been critiqued due to the challenges of obtaining suitable input data, the model-building process and model evaluation (Araújo et al., Citation2019; Pearson & Dawson, Citation2003; Srivastava et al., Citation2019). However, in the context of this study, SDMs provide a highly useful tool as we are primarily concerned with environmental suitability for given species in specific locations as determined by their fundamental niche, irrespective of (i) natural dispersal mechanisms (as the target species are manually installed as part of soft caps) and (ii) competition factors (due to the selective and periodic manual removal of unfavourable or undesirable colonists, such as woody species). Guides of best practice (Araújo et al., Citation2019) and review articles (Lissovsky et al., Citation2021) have also helped highlight approaches that enhance model quality.
In this study, we used an SDM approach with the aim of assessing the resilience of key sedum species in Britain and Ireland to future climate change over the 21st century under high and low emission scenarios. The importance of interactions between cultural heritage and biodiversity is increasingly being highlighted (Celesti-Grapow & Ricotta, Citation2021; Cicinelli et al., Citation2018; Panitsa et al., Citation2022), with soft capping argued to support the local biodiversity of ruined sites by increasing the diversity of habitats present on site and the provision of resources for pollinators (Wood et al., Citation2018). Therefore, in the first instance, we specifically chose to focus on the potential future presence and survival of selected British native and archaeophyte species (i.e. non-native taxa introduced by humans that naturalised before 1500 CE). Understanding the long-term behaviour of these species will help shape conservation practices at many ruined masonry sites as well as highlight the dynamics and importance of the relationship between cultural heritage and ecology.
Methods
Study area
Our study focused on Britain and Ireland due to the relatively widespread use of, and general interest in, soft capping here. The supra-national scale approach of our study means that outcomes can inform strategic conservation decisions rather than being limited to the management of a single site (Richards & Brimblecombe, Citation2022a). This also allowed us to capture spatial variations in climate across Britain and Ireland with, for example, southern England having a warmer climate than northern Scotland. Our approach could also be applied to other countries and regions, and findings for Britain and Ireland may be indicative of concerns/actions that may need to be considered in future management plans both in these regions and elsewhere. Throughout, we use the term “Ireland” to refer to the Isle of Ireland, including both the Republic of Ireland and Northern Ireland.
Species selection and records
At several ruined sites managed by English Heritage, “The Enviromat” sedum carpet has been used for soft-capping trials (Wood et al., Citation2018). This matting contains native, archaeophyte and non-native sedum species and one saxifrage (https://www.turfonline.co.uk/product/sedum-ground-mat-kit/). Of the 18 species in this matting, two are native (Sedum acre, which is also commonly used for sedum plugs, and Saxifraga granulata) and one is an archaeophyte (Sedum album). While Sedum anglicum is not found in this matting, it is of interest given its native status in the study area and, therefore, was included in our analysis. Sedum album and S. acre are the most widely distributed sedums across Britain and Ireland; S. anglicum is mainly found in coastal areas of Ireland and the west of Britain, and S. granulata has a widespread but patchy distribution (Figure S1, data are provided from the New Atlas of the British and Irish Flora recording scheme (1970–2022) courtesy of the Botanical Society of Britain and Ireland (BSBI)).
Presence records for each of the selected species were obtained from the BSBI and the Global Biodiversity Information Facility (GBIF) (). Criteria for record inclusion were informed by Pescott et al. (Citation2018). We included records that were as follows: (i) observations of in-situ plants; (ii) taken between 1970 and 2022; (iii) located between 30° to 70°N and −15° to 30°E, capturing the European range of the species; (iv) recorded using a reference coordinate associated with a precision of <3 km; and (v) geographically (spatially) unique, which we considered to be within two decimal places of longitude/latitude (approximately 1 km). The rationale for these criteria is outlined in . After the removal of records that did not meet our stated criteria, we retained the following numbers of records for each species: S. album = 28,591; S. acre = 37,190; S. anglicum = 12,944; and S. granulata = 12,276.
Figure 3. Schematic of (a) model inputs, (b) model runs, (c) model outputs and (d) data analysis used in this study to generate modelled presence likelihoods for four soft capping species (Sedum album, S. acre, S. anglicum and Saxifraga granulata).
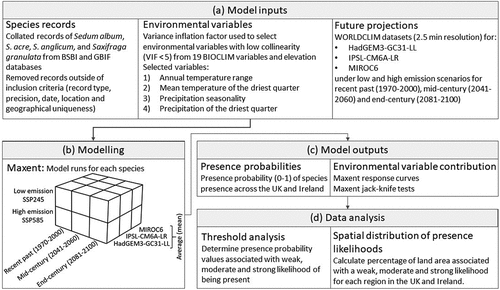
Table 1. Rationale of record inclusion criteria.
Environmental parameters
We used WorldClim’s 2.5-min resolution datasets (Fick & Hijmans, Citation2017) for the recent past (1970–2000), mid-century (2041–2060) and end-century (2081–2100) (). The realised environmental conditions experienced by plants are dependent on sub-meter- and sub-centimetre-scale microclimates. Therefore, all data coarser than this represent an averaged approximation of the conditions that plants will be exposed to, which is particularly the case for plants on top of exposed, free-standing walls. As such, none of the WorldClim datasets have a fine enough resolution to capture the exact wall-top microclimates. This is a common challenge and means that models generated using these data likely represent a wider climatic envelope for each target species. Thus, following the principles of model parsimony (Burnham & Anderson, Citation2002), we selected the coarser 2.5-min WorldClim dataset over the finer-scale 30-sec dataset, giving an adequate resolution for assessing local-scale environmental changes while also balancing computational efficiency.
Environmental parameters were assessed using three climate models from the CMIP6 ensemble run under low emission (SSP245) and high emission (SSP585) scenarios to capture a range of potential future pathways. The three climate models, HadGEM3-GC31-LL, IPSL-CM6A-LR and MIROC6, were selected due to their distance from each other with regard to their development, thereby minimising the biases present in any one climate model (). The environmental parameters used to drive each model were selected from the 19 BIOCLIM variables (Busby, Citation1991), which are climatic variables derived from temperature and rainfall parameters commonly used to explain species distributions, along with elevation (). Given the isolated nature of soft caps, we assumed that they are likely to be particularly affected by climatic changes as captured by the BIOCLIM parameters. For example, the positioning of soft caps means that their component species are not connected to groundwater supplies and are planted in controlled soil types, which are commonly relatively shallow. Furthermore, seasonal responses to temperature and rainfall have been noted in soft caps (Wood et al., Citation2018), which indicates their likely sensitivity to future changes in temperature and rainfall seasonality and extremes.
As many of the BIOCLIM variables are highly correlated, we selected variables with low collinearity that influence species survival within soft caps (Koźmińska et al., Citation2019; Nagase & Dunnett, Citation2010). The significance of collinearity was determined using the variance inflation factor (VIF) in a stepwise manner, until the VIF was <5 for all remaining variables (Ecodiv.Earth, Citation2016). Ultimately, we selected the following four BIOCLIM environmental variables as predictors: (1) annual temperature range; (2) mean temperature of the driest quarter; (3) precipitation seasonality (i.e. variation in monthly precipitation); and (4) precipitation in the driest quarter ().
Modelling the climate niche
We used the SDM Maxent Version 3.4.4 to model the relationship between species presence records and climate variables () (Phillips et al., Citation2021). Maxent is one of the most commonly used SDMs that applies the concept of the fundamental niche to define the presence probability of a given species under varying scenarios (Lissovsky & Dudov, Citation2021). We used Maxent as it can effectively determine occurrence or habitat suitability based on the presence of a species (Lissovsky & Dudov, Citation2021).
We trained Maxent using linear, quadratic and product functions so that a smooth response function was produced. For each model run, 25% of the observations were subsampled for testing, and a maximum of 1,000 model iterations were performed. The model performance was assessed using the Area Under the receiver operating characteristic Curve (AUC) method; AUC values range from 0 to 1, with values >0.5 indicating a model performance better than random chance. All model runs were checked to ensure environmental similarity between the training data and the modelled future data across Britain and Ireland.
Maxent was used to calculate the presence probability for each of the four target species in the recent past, mid-century and end-century; under low and high emission scenarios; and using climatic data from the three climate models (). The mean of the three climate models was calculated, and the results of each model run are provided as Supplementary Information (Figures S2–4). To evaluate the contribution of each individual BIOCLIM variable to the MAXENT prediction, we used response curves and the jack-knife test of variable importance, which were calculated within the MAXENT program (). A multivariate environmental similarity surface analysis was also performed by Maxent to assess the accuracy of the predicted distribution of the target species. Across the United Kingdom and Ireland, all projected environmental variables were within the range present in the training data.
Thresholds
The Maxent model calculates the presence probability between 0 and 1. We undertook a threshold analysis to relate these values to weak, moderate or strong likelihoods of a given species being able to survive. For each species, we calculated the distribution of the presence probabilities of terrestrial grid cells across Britain and Ireland where the species have been recorded. These were plotted as cumulative histograms, and the presence probability values associated with the 2nd and 20th percentiles of each dataset were selected as lower and upper thresholds (to the nearest 0.05 presence probability value). These percentiles were determined by visually comparing the model outputs for the recent past to the observation data. Presence probability values below the lower threshold are associated with a weak likelihood of a species being present; values between the two thresholds are associated with a moderate likelihood of being present; and values above the upper threshold are associated with a strong likelihood of being present. The classification of a moderate likelihood of survival acknowledges that soft caps are able to be maintained, and thus with some limited human intervention (e.g. additional watering during drought periods) these caps may be able to withstand environmental conditions that would be too stressful for populations without additional maintenance.
The likelihood values of species presence for past and future scenarios were spatially mapped to assess changes in the distributions of the species. Changes between the past and future scenarios were assessed by comparing (i) the spatial coverage of each likelihood value (e.g. size of the distribution) in England, Wales, Scotland and Ireland and; (ii) the latitudinal and longitudinal distributions of each likelihood value (e.g. location of the distribution).
The longitudinal and latitudinal distributions were presented using box and whisker plots where the box is bounded by the 25th and 75th percentiles, with the median shown with a central line. The whiskers represent all other points, except those that are deemed as outliers, here considered to be any value that lies over 1.5 times the interquartile range below and above the 25th and 75th percentiles. The large sample size of our dataset meant that statistical tests such as Mann Whitney U were overpowered, resulting in differences being found that were of statistical significance but of no practical importance. Therefore, for each likelihood, variations in the latitude and longitude were assessed by comparing the median of the future distribution to the 40th to 60th percentile range from the historic distribution. If the median of the future distribution fell outside of this percentile range, it was deemed to be a notable change.
Results
Model quality and thresholds
The models’ AUC values were 0.816 (S. album), 0.821 (S. acre), 0.964 (S. anglicum) and 0.864 (S. granulata) suggesting a model quality of good to excellent for all four species (Araújo et al., Citation2005), with a tight relationship between species distribution and the bioclimatic variables. The threshold values associated with weak, moderate or strong likelihoods of the environment being habitable were species dependent (). For S. anglicum, the range of presence probability values associated with a moderate likelihood of being present was notably wider than for the other studied species, even though its AUC suggests an excellent model quality (). This might suggest that at lower presence probability values, other factors, such as habitat availability, could control the distribution of S. anglicum. In addition, with fewer mainland European records, future projections are more likely to be skewed by the distribution of this species in Britain and Ireland. Therefore, we note that the moderate presence probabilities for S. anglicum may have a greater level of uncertainty than those for the other species.
Figure 4. Comparative cumulative distributions of presence probabilities for terrestrial grid cells across Britain and Ireland with records for: (a) Sedum album, (b) S. acre, (c) S. anglicum and (d) Saxifraga granulata. The presence probabilities associated with 2% and 20% of the locations with a record of species presence are indicated with dot-dashed and dashed lines, respectively. Values below the 2% threshold, between 2 and 20% thresholds and above the 20% threshold are associated with a weak, moderate and strong likelihood of survival, respectively.
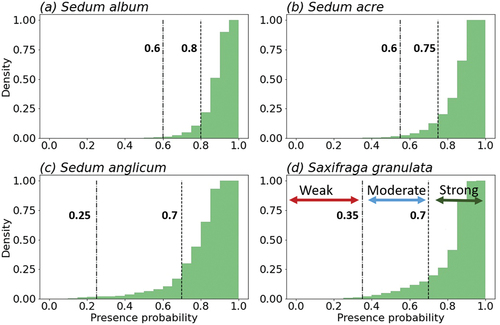
Contributions of environmental variables
We found that the contributions of environmental variables to the predicted probabilities of species presence were similar for S. album and S. acre. High presence probabilities were associated with an annual temperature range of between 15°C and 25°C; a mean temperature in the driest quarter (three-month period) between 5°C and 15°C; 150 mm of precipitation in the driest quarter; and decreasing precipitation seasonality (see Figure S9 for the response curves). In contrast, S. anglicum is able to better tolerate small annual temperature ranges, although the mean temperature in the driest quarter tolerance of this species is narrower than S. album and S. acre, peaking between 9°C and 14°C. The projected response of S. granulata was similar to S. album and S. acre for the mean temperature in the driest quarter, but this species was less tolerant of low ranges in annual temperature.
The Maxent jack-knife tests of variable importance showed that the mean temperature of the driest quarter provided the most information on habitat suitability for S. album, S. acre and S. granulata, while for S. anglicum, annual temperature range was the most important. For all species, annual temperature range contained the most information on habitat suitability that was not present in the other variables.
Species response
Our results show that the modelled habitat suitability for native and archaeophyte sedum and saxifrage species is spatially and temporally dependent (). In the recent past, S. album, S. acre and S. granulata show a strong presence likelihood across much of England, Wales, eastern Scotland and eastern Ireland, while a strong presence likelihood for S. anglicum mainly occurs along the west coasts of Britain and Ireland (). However, the mid-century projections show a shift from strong to moderate presence likelihoods under both low and high emission scenarios. By the end of the century, the likelihood of these species being present is heavily dependent on the emission pathway, with all species having a very weak likelihood of survival under the high emission scenarios.
Figure 5. Habitat suitability for Sedum album, S. acre, S. anglicum and Saxifraga granulata in the recent past (1970–2000) and under low and high emission scenarios for mid- and end-century. Areas are coloured according to whether they have a strong (green), moderate (blue) or weak (red/brown) likelihood of presence.
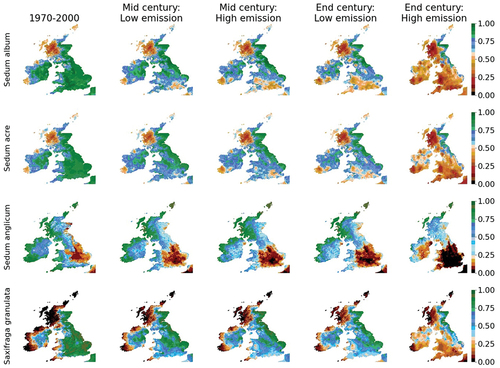
The widespread distribution of S. album across Britain and Ireland is reflected in the high habitat suitability for this species across much of the study area (, 1970–2000). Projections for mid-century show that under the low emission scenario in Wales, Scotland and Ireland, the proportion of land associated with a weak presence likelihood is projected to remain stable, with projected changes ranging from a 7% decrease (Scotland) to a 4% increase (Wales) in spatial coverage (). Under a high emission scenario, there is a notable increase in the size of the moderate and weak presence likelihoods in south England, Wales and Ireland () with strong likelihood distributions showing a notable shift northwards and weak likelihood distributions showing a notable shift south and eastwards ().
Figure 6. Percentage of land area in England (a, e, i, m), Wales (b, f, j, n), Scotland (c, g, k, o) and Ireland (d, h, l, p) with a weak (red), moderate (blue) or strong (green) likelihood for Sedum album (a-d), S. acre (e-h), S. anglicum (i-l) and Saxifraga granulata (m-p) presence in the recent past (1970–2000), by mid-century and by end-century under low and high emission scenarios. Histograms showing the distributions of presence probabilities are provided in Figures S5–S8.
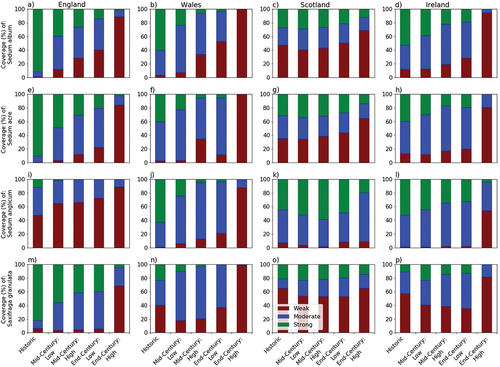
Figure 7. The latitudinal and longitudinal distribution of weak (red), moderate (blue) and strong (green) likelihoods under historic and future scenarios. Future distributions with notable change (as defined in section Thresholds) are shown in bold/opaque colours, while distributions with no notable change are shown with transparent colours.
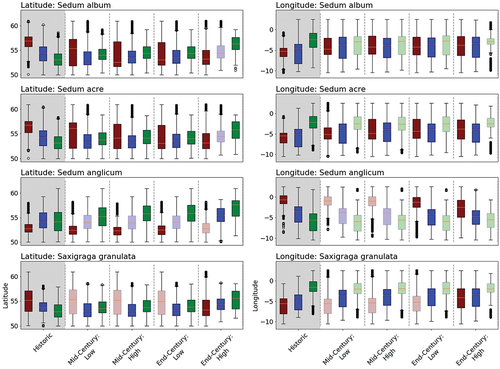
By end-century, under both low and high emission scenarios, a weak presence likelihood is projected for southern England and the majority of Wales (). Elsewhere, there are substantial differences in habitat suitability depending on the emission pathway. For example, under the low emission scenario, 71% of Ireland and 50% of Scotland have a moderate or strong presence probability for S. album, whereas, under the high emission scenario, the presence probability falls to 5% and 31% for Ireland and Scotland, respectively ().
The habitat suitability for S. acre is similar to that of S. album in the recent past (); however, by mid-century, projected areas of weak habitat suitability are more localised for southern England. Under the high emission future, there is a notable northwards shift in the distribution of strong habitat suitability (), resulting in over 80% of Ireland, 84% of England and 99% of Wales being projected to have weak habitat suitability for this species by end-century (). Areas of strong habitat suitability persist only along the east coast of Scotland ().
The modelled habitat suitability for S. anglicum has been more spatially distinct than S. album and S. acre, with areas of strong suitability occurring along the westerly coastlines and the Isles of Scotland, and weak suitability in central England (). By mid-century, strong habitat suitability persists in parts of western Scotland and Ireland, while in central England, there is an expansion in the area of weak habitat suitability (). Similar patterns are projected by end-century under the low emission scenario. Under the high emission scenario, the area of weak habitat suitability notably expands westwards () across the majority of England (88%), Wales (87%) and into central Ireland, with no land in England and Wales, and 4% of land in Ireland, projected to have strong suitability by the end of the 21st century (). Only in the north of Scotland does strong habitat suitability remain for S. anglicum over this timeframe.
The habitat suitability of S. granulata in the recent past shows a strong presence likelihood across central and eastern England, extending up the east coast of Scotland, with areas of weak habitat suitability located in western Ireland and western Scotland (). In contrast to the three sedum species, the percentage coverage of weak habitat suitability decreases in all regions into the mid-century (low and high emission scenarios) and end-century (low emission scenario) (). However, there is a pronounced shift from strong to moderate habitat suitability in England and Wales, with a notable northwards shift areas associated with a strong likelihood suitability (). For example, in England reductions in the coverage of strong habitat suitability are projected to be 26–40% by mid-century and 42–77% by end-century (). Under the high emission scenario, by the end of the 21st century, the only area with strong suitability for S. granulata is located on the east coast of Scotland, with no land in Wales or Ireland projected to have strong habitat suitability ().
Discussion
Sedums are an important plant type for soft capping on ruined heritage sites (Historic Environment Scotland, Citation2014a; Wood et al., Citation2018) and green roofs on buildings due to their hardy nature and ability to withstand extreme climate conditions (Vijayaraghavan, Citation2016). Given future projected increases in the magnitude and frequency of high-temperature events in Britain and Ireland (Gutiérrez et al., Citation2021), it might have been seen as reasonable to assume that this genus would be a resilient nature-based solution for heritage sites over the coming century. However, we have found that soft caps formed of the four species we studied are likely to become increasingly vulnerable if a high emission pathway is followed.
Our results follow a well-established trend (in the northern hemisphere, e.g. Berry et al., Citation2002; Thuiller et al., Citation2005) showing a northward shift in habitat suitability over the 21st century (). In areas with relatively warmer and drier climates (e.g. southern England and Wales), increases in temperature and drought periods may push species to the edges of their environmental niches, whereas in regions with cooler, damper climates today (e.g. northern Scotland), species will likely have greater tolerance of change, while still falling within their established environmental niches. It is also interesting to note that for soft capping in the west of Scotland, S. anglicum is likely to be more resilient to future climate change than S. album, S. acre and S. granulata. As S. anglicum has previously not been included in the brand of commonly used, commercially available sedum matting for soft capping, this could be a useful alternative species for soft capping in this region.
The stability, and even increase, in areas with strong habitat suitability in northern regions of Britain and Ireland suggests that where soft capping is currently successful, it is likely to remain successful until at least the mid-century (). For central and southern regions of Britain and Ireland, the shift from strong-to-moderate habitat suitability suggests that soft capping in these regions is likely to survive but may be vulnerable to external stressors. Consequently, species in areas with moderate habitat suitability many need additional maintenance during periods of high temperature or low precipitation. As altering the temperature of a site can be challenging, such maintenance is likely to involve additional watering during dry periods. Increasing moisture availability through regular watering (Nagase & Dunnett, Citation2010; VanWoert et al., Citation2005), water retention gels (Young et al., Citation2015) and increasing the water-holding capacity of the soil substrate (Farrell et al., Citation2012) have been shown to improve the survival of sedums on green roofs. Without such intervention, environmental conditions at some sites may not be viable for the survival of key soft capping species. In particular, the decrease in the habitat suitability of S. album in southern England suggests that for soft caps in this region, it may be more appropriate to use a different species (e.g. S. granulata or S. acre) to improve the resilience of soft capping into the mid-century. In addition, for areas with moderate habitat suitability, maintaining a diversity of species in the soft capping could be beneficial to improve long-term survivability (Nagase & Dunnett, Citation2010).
Furthermore, our results point to the importance of maintaining intraspecific genetic variation in the soft capping to enhance resilience to climatic changes. This variation is likely to be limited if soft capping installation uses cultivars from a limited number of commercial suppliers, unless these suppliers expand the genetic variation in their products. The use of commercial sedum matting for soft capping further extends the spread of cultivars across Britain and Ireland. Therefore, the use of plants derived from locally sourced material could help increase genetic diversity of soft capping species but may increase labour and financial implementation costs.
Our study adds to the vast body of literature showing the substantial impact that a high emission scenario will have on the Earth System. Indeed, by the end of the century under a high emission scenario, areas with suitable climatic conditions for current soft capping species substantially decline, with only the east coast of Scotland maintaining suitability for the examined species (). This suggests that if we are not meeting the targets for a low emission pathway by mid-century, soft capping is likely to need to be planted with more arid-tolerant species in all areas apart from the east coast of Scotland, unless current species show adaptions to new climatic conditions. For example, the use of perennial grasses or arid-tolerant succulents and cacti has been trialled by Lim et al. (Citation2013). Alternatively, non-native sedums such as S. spurium, S. ochroleucum and S. sediforme are more drought resistant than S. album (Koźmińska et al., Citation2019).
If we follow a high emission scenario to the end of the 21st century, many planetary boundaries and tipping points are likely to be crossed (Gutiérrez et al., Citation2021). In this study, we have modelled changes in average climate. Local conditions such as aspect, exposure and soil moisture retention properties will influence the resilience of species in soft caps (Young et al., Citation2015). As such, when relating our regional scale results to specific soft capped sites, if the site is located in a particularly exposed environment (e.g. on the top of an exposed, windy hill), our results might have overestimated the future viability of soft capping species; while at more sheltered sites, the soft caps may be more resilient than the model would suggest. Nevertheless, the direction and magnitude of change provides a clear indicator that heritage managers can incorporate in future-decision making.
In addition to changes in average climate, Britain and Ireland will also likely experience an increase in extreme events such as severe storms (e.g. MET office “amber” or “red” warnings), and the risk of wildfire is projected to increase in continental Europe (Gutiérrez et al., Citation2021), with grass fires occurring in the United Kingdom during the July 2022 heatwave where temperatures exceeded 40°C for the first time in England. Single periods of extreme drought or high temperature may have substantial impacts on habitat suitability and directly influence the effectiveness of soft capping at minimising deterioration processes. Furthermore, if a high emission pathway is realised, soft capping may be less effective at dampening deterioration processes associated with more extreme and variable climate conditions. Therefore, in order to develop effective long-term conservation strategies, further research should be conducted to establish the climatic limits of soft capping (under both future mean and extreme conditions) alongside other forms of NbS for vulnerable heritage.
Given the growing interest in, and opportunities for, adopting NbS for heritage conservation (e.g. Coombes & Viles, Citation2021), our study provides one of the first examples of how a modelling-based approach can inform the design, implementation and maintenance of these conservation strategies. It enabled us to investigate the likely ability of individual sedum and saxifrage species to tolerate future climatic conditions, with the results highlighting the variability in the predicted response between individual species across Britain and Ireland (). This kind of projection-based analysis is crucial for predicting and evaluating the long-term climate resilience of heritage sites both with respect to materials and structures and the ecological communities they support (Coombes & Viles, Citation2021; Luciani & Del Curto, Citation2018; Orr et al., Citation2021; Richards & Brimblecombe, Citation2022b; Viles & Cutler, Citation2012). However, by assessing individual species, our approach does not account for the role of diverse plant communities on the presence probabilities. As diverse green roof communities have been found to have greater survivability compared to monocultures (Nagase & Dunnett, Citation2010), the studied species, located in diverse soft caps, may have a slightly greater tolerance to climatic changes than our predictions. Even if this is the case, the magnitude of future change in our modelled presence probabilities indicates that the future climate will have a substantial impact on the survival of the studied species, particularly in the southern regions of Britain and Ireland ().
Conclusions
Natural-based conservation strategies are increasingly being seen as an important mechanism to address societal challenges in a way that also benefits the environment. For these strategies to remain effective into the future, they need to be able to withstand future environmental conditions. We focus on the NbS of soft capping to assess the extent to which these methods are future-proof. Soft capping is a widely recommended nature-based conservation strategy for ruined masonry in Britain and Ireland. We used species distribution modelling to assess the habitat suitability of four native and archaeophyte soft capping species over the 21st century. Our results indicate that sedum-based soft caps that are already successfully installed and functioning at heritage sites will likely remain viable till the mid-century, although soft caps predominantly formed of S. album may be more prone to failure in south-eastern England. In southern and central regions of Britain and Ireland, increased and additional maintenance, such as watering, may be required during extended periods of drought or in heatwaves. Beyond the mid-century, the management of soft capping is more uncertain and will be dependent on which emission pathway we follow. Under a high emission pathway, we found that only the east coast of Scotland maintained suitability for the studied soft capping species. Alongside much-needed evaluations of other NbS for heritage conservation, future work should assess the climate resilience of other plant groups commonly used in soft capping (e.g. grasses). In conjunction with further modelling-based research, environmental chamber studies and microcosm experiments could be used to investigate the tolerance of key species to moderately suitable habitat conditions as well as extreme events, both in monoculture and species-diverse soft caps. This would help assess the long-term climate sensitivity of species across the wider limits of their environmental niche as well as the functional resilience of soft caps for heritage conservation.
Author contributions
Conception of study: JR, MC; Design of study: JR, JJ, EC; Data acquisition: JR, JJ; Analysis and interpretation of data: JR; JJ, MC, EC, HV; Manuscript drafting and editing: JR, JJ, HV, EC, MC
Acknowledgments
We would like to acknowledge the environmental data used in this study, provided freely by WorldClim. Species data were provided from the New Atlas of the British and Irish Flora recording scheme, courtesy of the Botanical Society of Britain and Ireland (BSBI) and the Global Biodiversity Information Facility (GBIF).
We would like to thank Kevin Walker and Richard Grenyer for their input to this project, and Alice Goudie for producing .
The authors report there are no competing interests to declare. Data are available from the authors on a reasonable request.
Disclosure statement
No potential conflict of interest was reported by the author(s).
Additional information
Funding
References
- Araújo, M. B., Anderson, R. P., Barbosa, A. M., Beale, C. M., Dormann, C. F., Early, R., Garcia, R. A., Guisan, A., Maiorano, L., Naimi, B., O’Hara, R. B., Zimmermann, N. E., & Rahbek, C. (2019). Standards for distribution models in biodiversity assessments. Science Advances, 5(1), 4858–4874. https://doi.org/10.1126/sciadv.aat4858
- Araújo, M. B., Pearson, R. G., Thuiller, W., & Erhard, M. (2005). Validation of species–climate impact models under climate change. Global Change Biology, 11(9), 1504–1513. https://doi.org/10.1111/J.1365-2486.2005.01000.X
- Berry, P. M., Dawson, T. P. T. P., Harrison, P. A., & Pearson, R. G. (2002). Modelling potential impacts of climate change on the bioclimatic envelope of species in Britain and Ireland. Global Ecology & Biogeography, 11(6), 453–462. https://doi.org/10.1111/J.1466-8238.2002.00304.X
- Burnham, K. P., & Anderson, D. R. (2002). Model selection and multimodel inference: A practical information-theoretic approach (2nd ed.). Springer-Verlag.
- Busby, J. R. (1991). BIOCLIM: A bioclimate analysis and prediction system. Plant Protection Quarterly, 6(1), 8–9.
- Celesti-Grapow, L., & Ricotta, C. (2021). Plant invasion as an emerging challenge for the conservation of heritage sites: The spread of ornamental trees on ancient monuments in Rome, Italy. Biological Invasions, 23(4), 1191–1206. https://doi.org/10.1007/s10530-020-02429-9
- Cicinelli, E., Salerno, G., & Caneva, G. (2018). An assessment methodology to combine the preservation of biodiversity and cultural heritage: The San Vincenzo al Volturno historical site (Molise, Italy). Biodiversity and Conservation, 27(5), 1073–1093. https://doi.org/10.1007/S10531-017-1480-Z
- Coombes, M. A., & Viles, H. A. (2021). Integrating nature-based solutions and the conservation of urban built heritage: Challenges, opportunities, and prospects. Urban Forestry & Urban Greening, 63, 127192. https://doi.org/10.1016/J.UFUG.2021.127192
- Durhman, A. K., Rowe, D. B., & Rugh, C. L. (2007). Effect of substrate depth on initial growth, coverage, and survival of 25 succulent green roof plant taxa. HortScience, 42(3), 588–595. https://doi.org/10.21273/HORTSCI.42.3.588
- Ecodiv.Earth. (2016). VIF stepwise variable selection. Ecostudies. https://pvanb.wordpress.com/2016/04/25/vif-stepwise-variable-selection/
- Farrell, C., Mitchell, R. E., Szota, C., Rayner, J. P., & Williams, N. S. G. (2012). Green roofs for hot and dry climates: Interacting effects of plant water use, succulence and substrate. Ecological Engineering, 49, 270–276. https://doi.org/10.1016/J.ECOLENG.2012.08.036
- Fick, S. E., & Hijmans, R. J. (2017). WorldClim 2: New 1km spatial resolution climate surfaces for global land areas. International Journal of Climatology, 37(12), 4302–4315. https://doi.org/10.1002/joc.5086
- Gutiérrez, J. M., Jones, R. G., Narisma, G. T., Alves, L. M., Amjad, M., Gorodetskaya, I. V., Grose, M., Klutse, N. A. B., Krakovska, S., Li, J., Martínez-Castro, D., Mearns, L. O., Mernild, S. H., Ngo Duc, T., van den Hurk, B., & Yoon, J. -H. (2021). Atlas. In climate change 2021: The physical science basis. Contribution of working group i to the sixth assessment report of the intergovernmental panel on climate change. http://interactive-atlas.ipcc.ch/
- Hanssen, S. V., & Viles, H. A. (2014). Can plants keep ruins dry? A quantitative assessment of the effect of soft capping on rainwater flows over ruined walls. Ecological Engineering, 71, 173–179. https://doi.org/10.1016/j.ecoleng.2014.07.028
- Historic Environment Scotland. (2014a). Soft Capping in Scotland: The context and potential of using plants to protect masonry. Volume 1. https://issuu.com/hspubs/docs/soft_capping_in_scotland_vol_1/3
- Historic Environment Scotland. (2014b). Soft Capping in Scotland: The context and potential of using plants to protect masonry. Volume 2: Case Studies. https://issuu.com/hspubs/docs/soft_capping_in_scotland_vol_2/1
- Koźmińska, A., Al Hassan, M., Wiszniewska, A., Hanus-Fajerska, E., Boscaiu, M., & Vicente, O. (2019). Responses of succulents to drought: Comparative analysis of four sedum (Crassulaceae) species. Scientia horticulturae, 243, 235–242. https://doi.org/10.1016/J.SCIENTA.2018.08.028
- Lee, Z., Viles, H. A., & Wood, C. H. (2009). Soft capping historic walls: A better way of conserving ruins? London: English Heritage. Retrieved May 9, 2023, from https://www.geog.ox.ac.uk/research/landscape/rubble/swc/swc-report.pdf
- Lim, A. B., Matero, F., & Henry, M. C. (2013). Greening deterioration: Soft capping as preventive conservation for masonry ruin sites on JSTOR. APT Bulletin: The Journal of Preservation Technology, 44(2/3), 53–61. https://www.jstor.org/stable/41982405?seq=3
- Lissovsky, A. A., & Dudov, S. V. (2021). Species-Distribution modeling: Advantages and limitations of its application. 2. MaxEnt. Biology Bulletin Reviews, 11(3), 265–275. 2021 11:3. https://doi.org/10.1134/S2079086421030087
- Lissovsky, A. A., Dudov, S. V., & Obolenskaya, E. V. (2021). Species-Distribution modeling: Advantages and limitations of its application. 1. General approaches. Biology Bulletin Reviews, 11(3), 254–264. 2021 11:3. https://doi.org/10.1134/S2079086421030075
- Luciani, A., & Del Curto, D. (2018). Towards a resilient perspective in building conservation. Journal of Cultural Heritage Management and Sustainable Development, 8(3), 309–320. https://doi.org/10.1108/JCHMSD-07-2016-0040
- Miller, N. F. (2019). Historic landscape and site preservation at Gordion, Turkey: An archaeobotanist’s perspective. Vegetation History and Archaeobotany, 28(3), 357–364. https://doi.org/10.1007/S00334-018-0689-4
- Nagase, A., & Dunnett, N. (2010). Drought tolerance in different vegetation types for extensive green roofs: Effects of watering and diversity. Landscape and Urban Planning, 97(4), 318–327. https://doi.org/10.1016/J.LANDURBPLAN.2010.07.005
- Orr, S. A., Richards, J., & Fatorić, S. (2021). Climate change and cultural heritage: A systematic literature review (2016–2020). The Historic Environment: Policy & Practice, 12(3–4), 434–477. https://doi.org/10.1080/17567505.2021.1957264
- Panitsa, M., Trigas, P., Kontakos, D., Valli, A. T., & Iatrou, G. (2022). Natural and cultural heritage interaction: Aspects of plant diversity in three east Peloponnesian castles (Greece) and conservation evaluation. Plant Biosystems - an International Journal Dealing with All Aspects of Plant Biology, 156(2), 538–552. https://doi.org/10.1080/11263504.2021.1889701
- Pearson, R. G., & Dawson, T. P. (2003). Predicting the impacts of climate change on the distribution of species: Are bioclimate envelope models useful? Global Ecology & Biogeography, 12(5), 361–371. https://doi.org/10.1046/J.1466-822X.2003.00042.X
- Pescott, O. L., Humphrey, T. A., & Walker, K. J. (2018). A short guide to using British and Irish plant occurrence data for research. v.1.4. https://nora.nerc.ac.uk/id/eprint/520609/1/N520609CR.pdf
- Phillips, S. J., Dudík, M., & Schapire, R. E. (2021). Maxent software for modeling species niches and distributions (Version 3.4.4). http://biodiversityinformatics.amnh.org/open_source/maxent/.
- Richards, J., & Brimblecombe, P. (2022a). Moisture as a driver of long-Term threats to timber heritage—Part I: Changing heritage climatology. Heritage, 5(3), 1929–1946. Ahead of print. https://doi.org/10.3390/heritage5030100
- Richards, J., & Brimblecombe, P. (2022b). The transfer of heritage modelling from research to practice. Heritage Science, 10(1), 1–10. https://doi.org/10.1186/s40494-022-00650-4
- Rowe, D. B., Monterusso, M. A., & Rugh, C. L. (2006). Assessment of Heat-expanded slate and fertility requirements in green roof substrates. HortTechnology, 16(3), 471–477. https://doi.org/10.21273/HORTTECH.16.3.0471
- Seddon, N. (2022). Harnessing the potential of nature-based solutions for mitigating and adapting to climate change. Science, 376(6600), 1400–1416. https://doi.org/10.1126/science.abn9668
- Srivastava, V., Lafond, V., & Griess, V. C. (2019). Species distribution models (SDM): Applications, benefits and challenges in invasive species management. CAB Reviews: Perspectives in Agriculture, Veterinary Science, Nutrition & Natural Resources, 2019, 14. https://doi.org/10.1079/PAVSNNR201914020
- Thuiller, W., Lavorel, S., Araújo, M. B., Sykes, M. T., & Prentice, I. C. (2005). Climate change threats to plant diversity in Europe. Proceedings of the National Academy of Sciences of the United States of America, 102(23), 8245–8250. https://doi.org/10.1073/PNAS.0409902102
- VanWoert, N. D., Rowe, D. B., Andresen, J. A., Rugh, C. L., & Xiao, L. (2005). Watering regime and green roof substrate design affect sedum plant growth. HortScience, 40(3), 659–664. https://doi.org/10.21273/HORTSCI.40.3.659
- Vijayaraghavan, K. (2016). Green roofs: A critical review on the role of components, benefits, limitations and trends. Renewable & Sustainable Energy Reviews, 57, 740–752. https://doi.org/10.1016/J.RSER.2015.12.119
- Viles, H. A., & Cutler, N. A. (2012). Global environmental change and the biology of heritage structures. Global Change Biology, 18(8), 2406–2418. https://doi.org/10.1111/J.1365-2486.2012.02713.X
- Viles, H. A., & Wood, C. (2007). Green walls?: Integrated laboratory and field testing of the effectiveness of soft wall capping in conserving ruins. Geological Society Special Publication, 271(1), 309–322. https://doi.org/10.1144/GSL.SP.2007.271.01.29
- Wood, C., Cathersides, A., & Viles, H. A. (2018). Soft capping on ruined masonry walls. https://research.historicengland.org.uk/Report.aspx?i=16216
- Young, T. M., Cameron, D. D., & Phoenix, G. K. (2015). Increasing green roof plant drought tolerance through substrate modification and the use of water retention gels. Urban Water Journal, 14(6), 551–560. https://doi.org/10.1080/1573062X.2015.1036761