ABSTRACT
The osteohistology including bone cross-sectional geometry of skeletal elements such as the limb bones, ribs, and intercentra of three Triassic temnospondyl taxa from India were examined to reveal varied bone microstructure and differing growth patterns. The Early Triassic trematosaurid examined is characterized by a change in tissue type from a well-vascularized fibrolamellar bone early in ontogeny to peripheral lamellar bone and lines of arrested growth later in ontogeny, suggesting a fast initial growth followed by a slow and intermittent growth later in life. In contrast, the Middle Triassic paracyclotosaurid and the Late Triassic chigutisaurid examined had a cyclical, overall slow growth, as evidenced from predominance of lamellar bone in the cortex. It is hypothesized that the rapid growth of the trematosaurids was an attempt to stabilize the ecosystem after the Permo-Triassic extinction event. Fibrolamellar bone tissue is described for the first time in a Triassic temnospondyl. Bone cross-sectional geometry, low-to-average cortical porosity, and other parameters imply that the members of the Early and Middle Triassic taxa recovered from India retained a high level of terrestriality. The high cortical porosity and extensive medullary spongiosa suggest that the Late Triassic chigutisaurids had a semi-aquatic or aquatic life style. The varying cortical thickness of the limb bones in paracyclotosaurids may be attributed to different biomechanical constraints.
INTRODUCTION
The temnospondyls were a large and diverse group of fossil tetrapods that existed from the Early Carboniferous to the Early Cretaceous. They are usually regarded as fossil amphibians that gave rise to some or all of the living lissamphibians (CitationDamiani, 2004). The temnospondyls are known from all continents and play an important role in biostratigraphy and global correlation (CitationDamiani, 2004; CitationRuta et al., 2007). They occupied a great range of ecological niches, from aquatic, semi-aquatic and amphibious, to terrestrial (CitationDefauw, 1989). The size of the Mesozoic temnospondyls varied from about 30 to 8000 mm in length (CitationSteyer and Damiani, 2005) and they can be differentiated from other tetrapods by their distinctive centra, which comprised a much reduced pleurocentrum and an enlarged intercentrum (CitationCarroll, 1988). More than 12 families of temnospondyls are known, including about 90 genera (CitationMilner, 1993; CitationYates and Warren, 2000).
India contains a rich and varied Triassic temnospondyl fauna from different stratigraphic horizons of several discrete Gondwana basins (). This fauna includes the Trematosauridae, Paracyclotosauridae, and Chigutisauridae (CitationBandyopadhyay, 1999; CitationSengupta, 2003). The trematosaurids are known from the Early Triassic Panchet Formation of the Damodar basin (CitationHuxley, 1865; CitationTripathi, 1969), the paracyclotosaurids (sensu CitationSchoch and Milner, 2000) are known from the Middle Triassic Denwa Formation of the Satpura basin (CitationMukherjee and Sengupta, 1998), whereas the chigutisaurids are known from the Late Triassic Maleri Formation of the Pranhita-Godavari basin (CitationSengupta, 1995).
FIGURE 1 Major Gondwana basins of peninsular India and representative vertical lithologs of A, Early Triassic Panchet Formation showing trematosaurid-bearing horizon; B, Middle Triassic Denwa Formation showing paracyclotosaurid-bearing horizon; C, Late Triassic Maleri Formation showing chigutisaurid-bearing horizon; D, Gondwana stratigraphy of the basins (after CitationKutty et al., 1987; CitationChakraborty et al., 2003). Fossil-bearing horizons are marked by asterisks. Inset shows the relative positions of the Gondwana outcrops within India. For the vertical lithologs, the x axis denotes grain size ranging from clay (cl) and silt (s) to various sand sizes such as fine (f), medium (m), coarse (c), and pebbly (p); the y axis denotes height in meters.
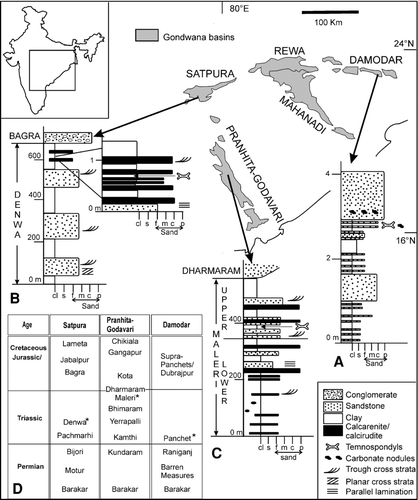
Previous work on temnospondyls from India involved taxonomy, osteology, taphonomy, and biostratigraphic implications (CitationRoyChowdhury, 1965; CitationKutty and Sengupta, 1989; CitationSengupta, 1995, 2002, 2003; CitationBandyopadhyay and Sengupta, 2006). These studies did not infer growth strategies and life habits of the animals and have lead to a rather limited understanding of their bone microstructure. The latter is important because it gives an insight into the growth of the animal under different environmental conditions and also the way the animal survived in different conditions. In general, there is little work on the bone histology of temnospondyls except for those of CitationRicqlès (1975, 1977, 1978, 1979), CitationDutuit and Heyler (1975), CitationDamiani (2000), CitationSteyer et al. (2004), and CitationSanchez et al. (2008). CitationDamiani (2000) examined the femoral bone microstructure of several unidentified temnospondyls and suggested that the animals were adapted to the aquatic medium, whereas CitationSteyer et al. (2004) analyzed the growth strategy of a metoposaurid temnospondyl, Dutuitosaurus, and, based on the femoral bone microstructure of both juveniles and adults, suggested that after a cyclical but high initial growth, there was a decrease in growth rate after attaining sexual maturity. A shift in the way of life from shallow water to deep water was correlated with an ontogenetic change from juveniles to adults for Dutuitosaurus (CitationSteyer et al., 2004).
Bone histology is a well-established technique for examining the paleobiology of extinct animals (e.g., CitationEnlow, 1963, 1969; Ricqlès, 1969, 1972, 1989; CitationChinsamy, 1997; CitationCurry, 1999; CitationHorner et al., 2000, 2001; CitationBotha, 2003). Although organic components such as osteocytes, blood vessels, and lymph vessels are destroyed during fossilization, the bone usually retains its structural organization, making it possible to decipher the tissue microstructure of the fossil animal (CitationFrancillon-Vieillot et al., 1990; CitationRicqlès et al., 1991). Comparing this with the bone microstructure of extant animals, various aspects such as individual age, growth, and life style adaptations can be inferred (e.g., CitationBotha and Chinsamy, 2004). Additional information regarding lifestyle may be obtained from the study of bone cross-sectional geometry because previous work has shown the existence of a direct relationship between an animal's lifestyle and structural design of the bones (e.g., CitationWall, 1983; CitationBotha and Chinsamy, 2004; Ray and CitationChinsamy, 2004; CitationRay et al., 2005; CitationKriloff et al., 2008).
The current study thus aims at a paleobiological study of three temnospondyl families—the Trematosauridae, Paracyclotosauridae, and Chigutisauridae—collected from India based on bone histology and bone cross-sectional geometry to elucidate their growth strategies, ontogenetic changes, and specialized life habits. Even though the work is based on limited samples, it reveals new observations on the bone microstructure and growth patterns that may prove to be characteristic of the three Indian temnospondyl families examined.
Institutional Abbreviations—ISIA, Indian Statistical Institute, Kolkata, India.
Bone Histological Abbreviations—ca, circumferential anastomoses of vascular channels; cor, cortex; flb, fibrolamellar bone; LAG, line of arrested growth; lb, lamellar bone; mr, medullary region; pfb, parallel fibered bone; pmr, perimedullary region; ra, radial anastomoses of vascular channels; RBT, relative bone wall thickness; rc, resorption cavities; trb, trabeculae; vc, vascular channels; wfb, woven fibered bone matrix.
GEOLOGICAL AND TAPHONOMIC BACKGROUND
Trematosauridae
The trematosaurids and an indeterminate taxon were collected from the Early Triassic Panchet Formation of the Damodar Basin (). The fossil locality is at Dumdumi (23° 37′ 57″N: 86° 52′ 55″E) near Asansol, West Bengal. The Panchet sediments dip at low angles (∼10°) towards N-NW at south of the Damodar River but in the reverse direction to the north of the river, indicating the presence of a gentle syncline. The overall paleocurrent trend is from northwest to northeast (CitationVeevers and Tewari, 1995). Lithologically, the Panchet Formation is a coarsening upward sequence () and is divided into three distinct parts (CitationRobinson, 1970). The lower part (50–100 m) consists of micaceous, greenish or olive green–colored, well-laminated siltstone, interbedded with yellow or buff-colored sandstone beds (0.5–4 m), and is dominated by fine interchannel deposits. The middle part (∼200 m), containing lenses of red or chocolate-colored laminated shaly siltstone (0.5–4.2 m) interbedded with buff-colored sandstones (2.5–23 m), is inferred as both channel and interchannel deposits. The sediments of the upper part (300–400 m) of the formation are dominated by buff or pale grey sandstones with thin red shale layers and were probably deposited rapidly by a large, high-energy river channel (CitationRobinson, 1970). Most of the Early Triassic temnospondyls examined were collected from these sandstone bodies () as channel lag deposits in association with smaller quartz pebbles, feldspar chips, and mudclasts.
Paracyclotosauridae
The paracyclotosaurids were collected from the Middle Triassic Denwa Formation of the Satpura basin () and pertain to the locality, Sahavan (22° 38′N: 78° 34′E) in Hoshangabad district, Madhya Pradesh. On the basis of contrasting lithofacies and distinctive sedimentary structures, the Denwa Formation is divided into a sandstone dominated Lower Denwa unit (120 m) and a mudstone dominated Upper Denwa unit (180 m). The paracyclotosaurids were collected from a heterolithic sand-mud facies of the Upper Denwa unit, which has been interpreted as deposited by sandy braided channels with associated flood plains (CitationGhosh et al., 2006).
Multiple individuals of paracyclotosaurids comprising adults, sub-adults, and juveniles along with dipnoan fishes were collected, showing a distinct stacking of their skulls one above the other in a small pocket of about 2 × 6 × 2 m3. Above this fossil-bearing horizon (), there is a thin layer of trough cross-stratified caliche-derived calcirudite unit containing unionid valves. All these features suggest bogging down of the paracyclotosaurids in shallow water (CitationBandyopadhyay et al., 2002). The presence of muddy heterolithic sheets along with wavy laminations in the siltstones, together with the typical fish-temnospondyl assemblage and unionid valves, indicates flood plain ponds (CitationGhosh et al., 2006). It may be noted that the paracyclotosaurids were a skull-dominated assemblage in association with a subordinate amount of disarticulated postcranial elements, suggesting that most of the postcranial elements were winnowed out and the heavier cranial material was preserved as residual assemblage.
Chigutisauridae
The chigutisaurids are from the Late Triassic Maleri Formation of the Pranhita-Godavari basin (). The fossil locality is at Nalapur (19° 13′ 45″N: 79° 29′ 41″E) of Adilabad district, Andhra Pradesh. The Maleri Formation (300–600 m) is characterized by a red mudstone dominated succession followed up by sand-mud heterolithic facies (). This horizon is biochronologically divided into a lower part with a Carnian fauna and an upper part with an Early Norian fauna (CitationKutty and Sengupta, 1989). The lower fauna is characterized by a metoposaurid temnospondyl, a rhyncosaurid, a phytosaurid, a prolacertid, an aetosaur, a coelurosaurid, and a cynodont. Some fossil fishes such as a dipnoan and a xenacanthid are also present. A few members of lower Maleri fauna such as the dicynodont and the aetosaur continue to the Upper Maleri zone. The latter zone contains a new fauna consisting of two chigutisaurid taxa and two phytosaurids.
Lithologically, the Maleri Formation is characterized by sheet-like sand bodies alternating with relatively thicker mudstone units (CitationBandyopadhyay et al., 2002). Other lithological attributes of the formation include multistorey channel-fill sand bodies and caliche-derived peloidal calcirudite/calcarenite. The sandstone and thick mudstone units are considered as channel and interchannel deposits, respectively (CitationSengupta, 1970). These individual channels are rich in mudclasts and occasional bone fragments. However, the chigutisaurid material examined in the present study was collected from the thick red mudstone facies () and was relatively well preserved. A generalized stratigraphy of the three basins showing the temnospondyl-bearing horizons is given in .
MATERIAL AND METHODS
The current work encompasses some skeletal elements (e.g., humerus, femur, tibia, ribs, and intercentra of the three temnospondyl families, the Trematosauridae, Paracyclotosauridae, and Chigutisauridae. In addition, a humerus and several rib fragments of an indeterminate temnospondyl taxon were examined (–L, ). A comprehensive study of bone histology would involve examining multiple specimens of particular elements of each taxon in order to assess ontogenetic, and environmental variation, and growth patterns. Although attempts were made to section various skeletal elements in the current study, the destructive nature of histological analysis and paucity of well-preserved and associated fossil specimens did not allow sectioning of an ideal data set. In only one instance, the intercentrum of Paracyclotosauridae, was it possible to section multiple examples of a particular element in order to account for variation within a taxon (). For this reason we recognize that this is a preliminary study, but one that can be eventually tested by sectioning comparable elements from other individuals of each taxon from Indian and non-Indian deposits.
TABLE 1 Stratigraphic horizon, measured length, calculated cortical thickness, and cortical porosity of the skeletal elements examined.
FIGURE 2 A, Schematic representation of a temnospondyl based on the reconstruction of Mastodonsaurus (not to scale, adapted from CitationSchoch and Milner, 2000) showing the different types of bones (in dark shade) examined for bone histology. Note that the bones examined are not from a single individual. B–L, available skeletal elements studied: B–C, Trematosauridae, B, ISIA181/3, humerus; C, ISIA181/2, femur; D–G, Indeterminate Temnospondyli, D, ISIA181/1, humerus; E, ISIA181/4, rib; F, ISIA181/5, rib; G, ISIA181/6, rib; H–K, Paracyclotosauridae, H, ISIA181/7, humerus; I, ISIA181/8, femur; J, ISIA181/9, tibia; K, ISIA181/10, rib; L, Chigutisauridae, ISIA181/14, intercentrum. Note that not all the elements examined are given in the figure. Scale applies to B–L. M, schematic representation of a transverse section of a bone showing the calculation of cortical thickness (RBT%) and k-values (after Wall, 1983; Curry and Alexander, 1985; CitationChinsamy, 1993). N, field of view of a femoral transverse section showing the calculation of porosity (shaded in grey) as a percentage of total cortical area (after CitationChinsamy, 1993).
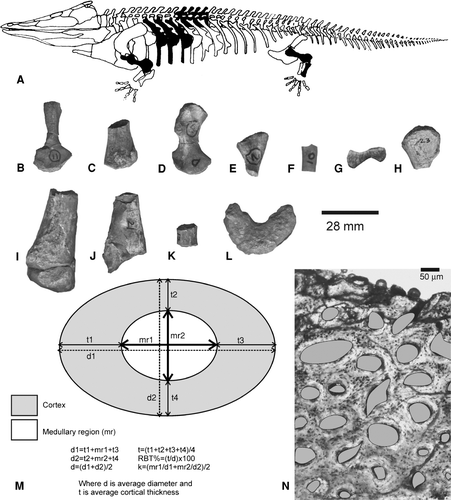
The Early Triassic humerus (ISIA181/3) and the femur (ISIA181/2) were found in association with skull fragments and mandibles of the trematosaurid Gonioglyptus CitationHuxley (1865). The humerus was characterized by a torsion in the mid-shaft region (<90°), and a prominent and wide deltoid crest with a narrow, thin, ridge-like pectoral extension towards the proximal end, whereas the femur contained a distal bifurcation for articulation with the tibia and fibula, and a thin feebly curved shaft. Based on their skeletal association and morphological features, these elements were considered as belonging to the trematosaurids. The other partially preserved humerus (ISIA181/1) and the ribs were collected as isolated specimens about 1.5–2 m away from the fossil locality and are considered here as indeterminate. In contrast, the specimens of Middle Triassic temnospondyls examined in the present work were associated with known taxa. The paracyclotosaurid elements were close to the skull of Parotosuchus (Stanocephalosaurus CitationSchoch and Milner, 2000) crookshanki (CitationMukherjee and Sengupta, 1998). The chigutisaurid humerus, ISIA41, was figured and described as part of Compsocerops cosgriffi by CitationSengupta (1995), whereas the undescribed rib and intercentrum () occurred in association with C. cosgriffi.
The mid-shaft regions of the limb bones were mainly examined because the least modified primary bone tissues are best preserved in these regions. Efforts were made to examine skeletal elements belonging to adult individuals only, and the adult stage was deduced from size-independent criteria such as well-developed muscle scars, ossification of the limb bones, and bone surface texture (Ray and CitationChinsamy, 2004; CitationTumarkin-Deratzian et al., 2006). This ontogenetic stage as deduced from morphological features was later verified from bone microstructure. Before preparing the thin sections, photographs were taken of all the specimens, morphological variations were noted and standard measurements were recorded using digimatic calipers with a precision of 0.01 mm. This was done to preserve an unambiguous record of the morphology of the bones and to compile a photographic record of the end result of the sectioning. Because bone histology is a destructive analysis, efforts were made to section only those skeletal elements that were recovered in multiples. It may be noted that the Early Triassic trematosaurid specimens are smaller in comparison to those of the Middle and Late Triassic taxa ().
Thin-section preparation of the skeletal elements was carried out following the procedures outlined by CitationChinsamy and Raath (1992). The bone slices were cut in the required direction by using a 24-inch LAPRO SLAB. Careful labeling of each resulting segment of the specimen was carried out and a unique number was given for identification and basic cataloging. The cut surface of each bone slice was then ground and polished using a coarse rock grinding machine until the surface was completely flat, smooth, and free from scratch marks. In addition to machine grinding, some manual grinding using an evenly circular motion was done to make the surface smooth. These surfaces were then polished with alundum powder of varying grit sizes (600, 800, 1000 mesh). These polished slices were then washed, dried, mounted, glued to frosted glass slides by araldite hardener and ground down. The final thickness of the slices varied between 120 and 20 μm. These thin sections were then examined in detail under a petrographic polarizing microscope to identify the bone tissue types and other histological characteristics.
Histological terminology and definitions in this paper follow CitationFrancillon-Vieillot et al. (1990) and CitationRicqlès et al. (1991). Growth rings are a common feature of most vertebrates, irrespective of their tissue types, size, and phylogenetic positions. However, not all growth rings are the same in structure or origin (CitationPadian, 1997). The term ‘growth ring’ is used to collectively denote annuli, lines of arrested growth (LAGs) and reversal lines. Annuli represent temporary slow growth whereas LAGs imply complete cessation of growth (CitationFrancillon-Vieillot et al., 1990; CitationRicqlès et al., 1991). A reversal line may form due to erosion of the cortical bone during non-deposition. Deduction of cortical thickness involves measuring relative bone wall thickness (RBT). RBT was calculated as the ratio of the bone wall thickness to the cross-sectional diameter measured across the transverse section following CitationBühler (1986) and CitationBotha (2003), and expressed as a percentage of the diameter (). The optimal k-value, which is the ratio of the internal to the external diameter, as suggested by Curry and Alexander (1985), was also calculated. The thickness of the bone wall and medullary region was measured along two perpendicular axes (), mainly in the diaphyseal sections in case of the long bones, at a magnification of 2.5×.
It may be mentioned here that the shape of bony canals in fossil vertebrates may not bear resemblance to the actual shape of the blood vessels therein (CitationStarck and Chinsamy, 2002). In living animals, along with blood vessels, lymphatic vessels, nerves, and other connective tissues may occupy these channels (CitationStarck and Chinsamy, 2002; Ray and CitationChinsamy, 2004). Hence, as suggested by Ray and CitationChinsamy (2004), cortical porosity implies maximum vascularity () and is quantified as a percentage of the total cortical area (CitationChinsamy, 1993). The procedure for measuring cortical porosity was carried out for several fields of view of each bone section at a magnification of 10× using the software Leica QW in version 3.0. The laminar, reticular and plexiform arrangements of the ‘blood vessels’ (sensu CitationEnlow, 1963; Ricqlés et al., 1991) refer to the arrangements of the channels and not the actual blood vessels.
HISTOLOGICAL DESCRIPTION
General Features
The transverse sections of all the bones examined, irrespective of the skeletal elements, are composed of a well-differentiated central medullary region surrounded by a relatively compact cortex (–7). Most of the ‘vascular channels’ (sensu Ray and CitationChinsamy, 2004), are isolated, discrete, and longitudinally oriented, often varying in shape and orientation, and have radial and circumferential anastomoses. At the regions of muscle insertions such as the deltopectoral crest, cnemial crest, and anterior and posterior ends of the ribs, vascular channels are radially oriented, often running from the inner to the outer cortex. Vascularity, defined in terms of cortical porosity (Ray and CitationChinsamy, 2004), decreases towards the periphery and in most bones results in an avascular outer cortex. The diaphyseal sections of the bones also reveal extensive secondary reconstruction in the form of enlarged resorption cavities and secondary osteons in the perimedullary region. The medullary margin is often uneven and resorptive in nature. The medullary region is filled with a dense network of bony trabeculae of the cancellous bone. Except for ISIA181/3, ISIA181/11, and ISIA41, multiple sections of all other temnospondyl skeletal elements examined are characterized by the absence of growth rings.
FIGURE 3 Early Triassic temnospondyls. A–B, Trematosauridae, ISIA181/3, humerus, showing A, a thick cortex surrounding a large medullary region. Note the presence of a peripheral zone of avascular lamellar bone, which is separated by a prominent LAG (arrow) from the fibrolamellar bone in the inner cortex; B, inset of A at higher magnification showing the LAG (arrow); C–D, Trematosauridae, ISIA181/2, femur, showing C, fibrolamellar bone in the cortex and bony trabeculae in the medullary region. White arrow indicates a reversal line in the perimedullary region; D, inset of C at higher magnification showing fibrolamellar bone tissue and a decrease in cortical vascularity towards the periosteal periphery. White arrows indicate discrete longitudinally oriented primary osteons whereas black arrows indicate globular osteocite lacunae; E–F, Temnospondyli indet., ISIA181/1, cortical region of humerus showing E, fibrolamellar bone in deeper cortex and parallel fibered bone at the periosteal periphery. Arrows indicate primary vascular channels; F, radiating vascular channels (arrows) in the region of the deltopectoral crest. All figures are of transverse sections. Scale bars equal 300 μm.
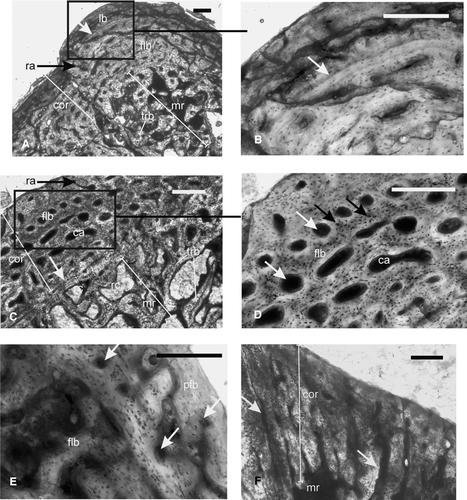
FIGURE 4 Temnospondyli indet, ribs. A–C, ISIA181/5, showing A, a thick compact cortex surrounding a medullary region; B, inset I of A at higher magnification showing woven fibered bone matrix and longitudinally oriented primary vascular channels (arrow); C, inset II of A showing large resorption cavities (arrow) in the perimedullary region; D–F, ISIA181/6, showing D, woven fibered bone matrix with primary vascular channels (arrow) in the cortex; E–F, profuse radially arranged vascular channels; F, inset of E at higher magnification. Arrows indicate two sets of radially arranged vascular channels; one running from the inner to mid cortex and the other from mid to outer cortex. All figures are of transverse sections. Scale bars equal 300 μm.
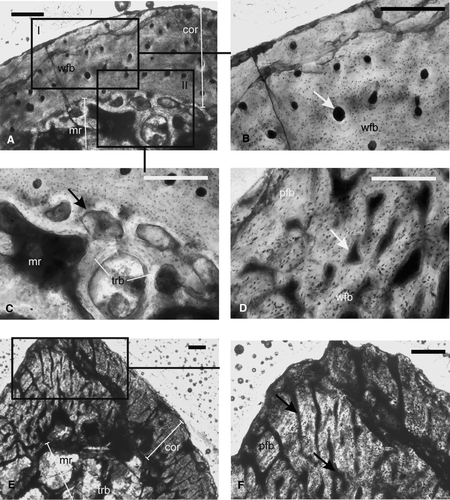
FIGURE 5 Paracyclotosauridae. A–B, ISIA181/7, humerus showing A, lamellar bone as the primary tissue of the cortex; arrow indicates the parallel network of the longitudinally oriented vascular channels; B, asymmetric extension (indicated by a white arrow) of secondary reconstruction towards the deltopectoral crest; C, ISIA181/8, femur showing predominantly lamellar bone in the cortex; note the large resorption cavities in the perimedullary region. All figures are of transverse sections. Scale bars equal 300 μm.
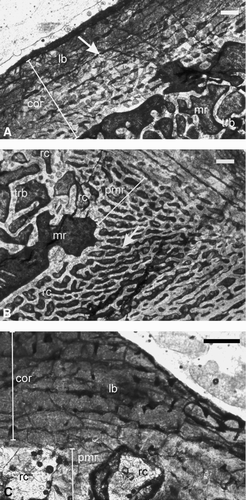
Early Triassic Trematosauridae
Humerus—The humerus (ISIA181/3) has a thick cortex (RBT 23.37%; ) surrounding a large medullary region. At the periosteal periphery, a thick zone of avascular lamellar bone is separated from the rest of the cortex by a prominent LAG (–B). The mid-cortical region () is characterized by a narrow zone of vascular fibrolamellar bone (cortical porosity 7.16%). Vascular channels are longitudinally oriented, discrete, and isolated, often with radial anastomoses. The periphery of the medullary region is smooth and the medullary region is filled with a network of trabeculae of the cancellous bone.
Femur—The femur (ISIA181/2) contains a narrow cortex (RBT 10.12%), and a large medullary region (). The cortex contains woven fibered matrix with numerous globular osteocyte lacunae that are densely packed and randomly oriented (–D). Discrete and isolated primary osteons are longitudinally oriented with few radial and circumferential anastomoses, and together with the woven fibered bone formed the fibrolamellar bone tissue (). Cortical porosity is 7.83% (), and decreases towards the periphery of the cortex (), which makes a narrow peripheral, almost avascular zone. Growth rings are absent. Erosionally enlarged resorption cavities are present (). The medullary region contains a network of loosely packed bony trabeculae of the cancellous bone. The latter is separated from the primary cortical tissue by a prominent reversal line ().
Indeterminate Early Triassic Temnospondyli
Humerus—The humerus (ISIA181/1) has a distinct cortex surrounding a relatively larger medullary region. The cortical thickness is slightly more than 18% (RBT 18.37%) in the humerus (). The diaphyseal section of the bone reveals fibrolamellar bone as the primary bone tissue, which is essentially present in the mid and deeper cortex (). The primary osteons are arranged in a laminar-reticular pattern. The humerus is characterized by the presence of profuse radially arranged vascular channels, which are irregular in shape, and extend from the inner to the outer cortex at the level of the deltopectoral crest (). The porosity of the humerus is 10.3% (). The periosteal periphery of this section preserves a zone of parallel fibered bone tissue (). Growth rings are absent. The medullary region is filled with a dense network of bony trabeculae of the cancellous bone.
Ribs—Transverse sections of three ribs of Early Triassic temnospondyls (ISIA181/4, ISIA181/5, and ISIA181/6) were examined. In ISIA181/4, the transverse section of the proximal end reveals a large medullary region surrounded by a very thin cortex. The matrix of the cortex is woven fibered. There are numerous slightly circular to elliptical osteocyte lacunae, which are densely packed and randomly oriented. Vascularity decreases towards the periphery of the cortex. The medullary region is filled with bony trabeculae.
The transverse section from the middle part of another rib fragment (ISIA181/5; –C) reveals a centrally placed medullary region surrounded by a relatively thick cortex (RBT 16.96%; , ). The primary cortical bone tissue is woven fibered (). Isolated and discrete longitudinally oriented primary vascular channels are present in the cortex (). ISIA181/5 is characterized by a low average cortical porosity (1.23%). In general, the size and density of these primary vascular channels decrease towards the periosteal periphery. The periosteal periphery is smooth. No growth rings have been observed. Large resorption cavities are present in the perimedullary region (). The medullary region is filled with a loose network of bony trabeculae.
FIGURE 6 Paracyclotosauridae. A–B, ISIA181/9, tibia showing A, a narrow cortex composed of lamellar bone surrounding a large medullary region. Note the presence of bony trabeculae in the medullary region. Arrows indicate longitudinally oriented vascular channels. B, radially arranged vascular channels (arrow) at the cnemial crest; C–D, ISIA181/10, rib showing C, a thick compact cortex surrounding the medullary region. Black arrow indicates the irregular periosteal periphery. White arrow indicates radial channels in the deeper cortex. D, peripheral lamellar bone and woven fibered bone in the deeper cortex. E, ISIA181/11, intercentrum showing a compact cortex containing avascular lamellar bone and three LAGs (arrows), surrounding a medullary region. Note the presence of bony trabeculae of the cancellous bone in the medulla. F, ISIA181/12, intercentrum showing Sharpeys’ fibers (arrows) and radially arranged vascular channels in the cortex. All figures are of transverse sections. Scale bars equal 300 μm.
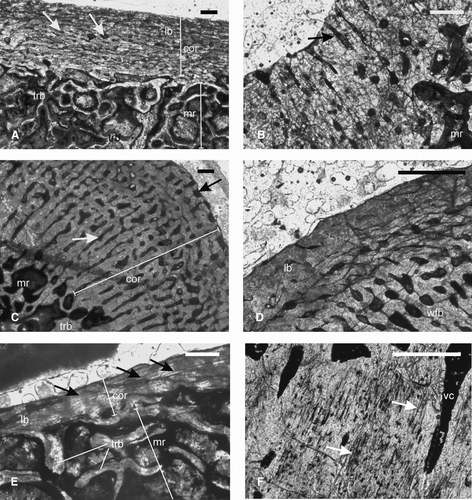
FIGURE 7 Chigutisauridae. A–B, ISIA41, humerus, showing A, lamellar bone as the primary cortical tissue with three LAGs (arrows) and resorption cavities in the perimedullary region; B, extensive secondary reconstruction as evidenced by large resorption cavities and secondary osteon (arrow); C, ISIA181/13, a rib showing lamellar bone in the outer cortex and woven fibered bone in the inner cortex. Note the irregular periosteal periphery (black arrow) and large erosion cavities (white arrow) in the perimedullary region. D, ISIA181/14, intercentrum showing a narrow cortex containing lamellar bone. All figures are of transverse sections. Scale bars equal 300 μm.
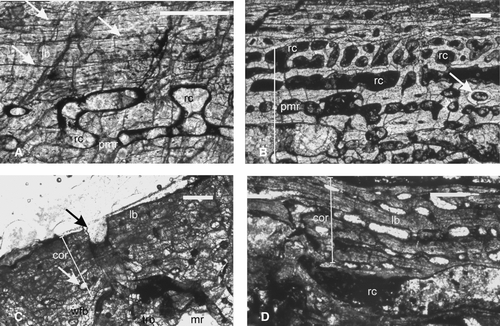
The transverse section of the proximal region of another rib (ISIA181/6) shows a thick, well-defined cortex (RBT 20.44%). The cortex contains woven fibered matrix () similar to that seen in ISIA181/5, and surrounds a distinct and large medullary region (). The vascular channels are mostly irregular in shape, longitudinally oriented (though it varies from place to place), and do not show any concentric deposition of lamellated bone, implying that these are primary channels (). A distinctive feature of this rib is the profusion of radially arranged vascular channels, one set extending from the inner to the mid cortex and the other from the mid to outer cortex (–F). The cortical porosity of this element is higher than that of ISIA181/5 (4.26%; ). Along the periosteal periphery there is a narrow zone of parallel fibered tissue (). No growth rings are seen. The medullary region is partially filled with loosely packed bony trabeculae.
Middle Triassic Paracyclotosauridae
Humerus—A diaphyseal section of the humerus (ISIA181/7; ) has a centrally placed medullary region surrounded by a thick rim of cortex (RBT 25.89%), which is much higher than that of the studied trematosaurids (). The primary bone tissue of the cortex is identified as lamellar () because the matrix shows alternating parallel fine and dark bands under the crossed nicol. The vascular channels are longitudinally oriented and with circumferential anastomoses, resulting in a densely parallel network (cortical porosity 7.81%). Growth rings are absent. The medullary region is partially filled with a network of bony trabeculae (). In the perimedullary region, there are extensive resorption cavities (), with an asymmetric extension towards the deltopectoral crest.
Femur—A diaphyseal section of the femur (ISIA181/8) contains a large medullary region surrounded by a relatively thin cortex (RBT 6.25%; ). The primary cortical tissue is predominantly lamellar bone () of low vascularity. There is no free medullary cavity, and the region is completely filled with a dense network of bony trabeculae. The margin of the medullary region is resorptive and irregular in nature. Some large irregular resorptive cavities are present in the perimedullary region ().
Tibia—The tibia (ISIA181/9) is characterized by a narrow cortex (RBT 8.01%; ) surrounding a large medullary region (). The cortex is predominantly composed of lamellar bone tissue, as shown by the linearly arranged multiple layering of the bone fibers. Osteocyte lacunae are rare. Isolated longitudinally oriented vascular channels are present. At the cnemial crest, radially arranged vascular channels are present (). Growth rings are absent. The medullary region is filled with bony trabeculae of the cancellous bone ().
Rib—The rib (ISIA181/10) shows a thick cortex (RBT 20.07%) surrounding a comparatively smaller centrally located medullary region. The periosteal periphery is irregular (). The predominant primary cortical bone is woven fibered, though there is a peripheral zone of lamellar bone in the outer cortex (). The vascular channels are primary and longitudinally oriented, with few extending up to the periosteal periphery. Radially arranged primary vascular channels are present along the anterior and posterior ends of the rib (). Although the cortical porosity is high (11.51%), the vascularity decreases towards the outer cortex. As in the tibia, ISIA181/9, osteocyte lacunae are rare in this specimen. The medullary region is filled with bony trabeculae.
Intercentra—Transverse sections of the intercentra (ISIA181/11, ISIA181/12) show a narrow cortex. The vascular channels are mostly parallel to the length of the intercentra. In ISIA181/11, the narrow cortex is composed of avascular lamellar bone tissue (). Three LAGs are found () in the mid-cortical region of this bone.
Similarly in ISIA181/12, the narrow cortex is characterized by lamellar bone with few longitudinal vascular channels. Most of these vascular channels are diagenetically altered. Abundant Sharpey's fibers are seen throughout the cortical region (). Growth rings are absent in this intercentrum. Resorption cavities showing endosteal bone deposition delineate the extensive medullary spongiosa from the thinner outer cortex in ISIA181/12, whereas in ISIA181/11, there is gradual transition between the cortex and the medullary region. Both the thin sections preserve a large medullary region completely filled with cancellous bone ().
Late Triassic Chigutisauridae
Humerus—A distal diaphyseal section (ISIA41) was examined to reveal a thin cortex (RBT 16.97%), which is similar to that of the forelimb bones of the examined trematosaurids. The primary bone tissue is identified as lamellar (). Three LAGs are found in the outer cortex (). Other features indicate extensive secondary reconstruction. Almost 90% of the cortex is composed of erosionally enlarged resorption cavities (–B), resulting in a high cortical porosity (21.53%; ). Few well-developed secondary osteons are seen in the perimedullary region (). The large medullary region is partially filled with a network of bony trabeculae of the cancellous bone.
Rib—The rib fragment (ISIA181/13) studied here was diagenetically altered and relatively poorly preserved. A transverse section of this specimen shows a thin cortex surrounding the medullary region. The mid to inner cortex contains woven fibered matrix, whereas the outer cortex shows lamellar bone (). The periosteal periphery is irregular (), with vascularity increasing away from it. Secondary reconstruction in the form of large erosional cavities is present (). The medullary region is filled with bony trabeculae of the cancellous bone.
Intercentrum—A transverse section of the intercentrum (ISIA181/14) shows a narrow cortex composed of lamellar bone tissue (). Most of the vascular channels are erosionally enlarged. Growth rings are absent. The intercentrum is characterized by an extensive medullary spongiosa.
DISCUSSION
Growth Patterns
Early Triassic Trematosauridae—Study of the bone microstructure of two different skeletal elements of Early Triassic trematosaurids from India reveals a predominance of woven fibered bone matrix, longitudinally oriented primary osteons, and fibrolamellar bone tissue. The presence of fibrolamellar bone tissue indicates rapid osteogenesis (CitationAmprino, 1947; CitationMargerie et al., 2002; Ray and CitationChinsamy, 2004) and suggests a fast growth for the trematosaurids (). However, in the humerus (ISIA181/3), growth stopped and then continued with a slow growth rate, as evidenced by the thick peripheral avascular lamellar bone that follows after a prominent LAG (). This slow growth after a fast initial growth possibly indicates the onset of sexual maturity (CitationSander, 2000; CitationBotha, 2003). A LAG is also seen in the mid to inner cortex, indicating periodic cessation of growth. The smooth outline of the medullary region suggests that the medullary expansion was complete and that the humerus belonged to an adult individual.
TABLE 2 Histological characteristics and growth patterns of the Triassic temnospondyls examined.
Similar initial fast growth followed by slow growth is also seen in the femur of the trematosaurid examined in the present work. Although parallel fibered bone or lamellar bone is absent along the periosteal periphery, the outer femoral cortex is nearly avascular, implying the onset of slow growth. In addition, the perimedullary region shows endosteal bone deposition in the form of compacted coarse cancellous bone, suggesting that the femur examined is also not of a juvenile animal, but possibly indicates an intermediate (sub-adult) stage of ontogeny. This is corroborated by the well-ossified morphological features, suggesting that the femur belonged to an adult or sub-adult individual. Lack of growth rings implies sustained growth throughout ontogeny without any periodic interruptions, though there was a distinct variation in the rate of growth. Thus, the growth pattern of the Early Triassic trematosaurid studied involved a fast but continuous initial growth (early in ontogeny) that became considerably slower and periodic in the sub-adult and adult stages (CitationMukherjee et al., 2008).
Early Triassic Indeterminate Temnospondyli—Similar to the trematosaurid skeletal elements, the humerus (ISIA181/1) of the indeterminate temnospondyl shows a predominance of fibrolamellar bone in the cortex, indicating an initial fast growth. This fibrolamellar bone tissue is mainly present in the mid to inner cortical region, whereas the outer cortex shows a peripheral parallel fibered bone, suggesting a decrease in periosteal osteogenesis. This is further corroborated by a decrease in cortical porosity and a more organized pattern of primary osteons (Ray and CitationChinsamy, 2004). It is evident from the bone microstructure that after an initial fast growth, there was a period of slow growth later in ontogeny (CitationFrancillon-Vieillot et al., 1990). There is also a profusion of radial vascular channels in the mid- to inner cortical region. Presence of parallel fibered bone, organized pattern of primary osteons, and a smooth periosteal periphery imply that the humerus belonged to an adult individual. However, growth was continuous, as evident from the lack of external circumferential lamellae.
Several ribs of Early Triassic temnospondyls were examined and reveal a predominance of woven fibered bone tissue in the cortex. In addition, there is a narrow zone of parallel fibered bone at the periosteal periphery. As in other skeletal elements, this feature of the bone microstructure suggests rapid osteogenesis and initial fast growth followed by considerably slow growth (). The ribs also show the onset of secondary reconstruction in the form of large resorption cavities. Endosteal bone deposition is noted in the perimedullary region. Because secondary osteons are not present, and there is a very narrow zone of parallel fibered bone, an intermediate (sub-adult) ontogenetic stage is proposed for the temnospondyl ribs examined.
Middle Triassic Paracyclotosauridae—Several elements of the Middle Triassic paracyclotosaurids examined here show striking differences in bone microstructure and inferred growth patterns with respect to that of the studied Early Triassic temnospondyls. Both the fore and hind limb bones show predominance of lamellar bone tissue in the cortex (), thereby implying an overall slow growth throughout ontogeny (CitationFrancillon-Vieillot et al., 1990). However, woven fibered bone tissue is present in the deeper cortex of the humerus (ISIA181/7) and the rib (ISIA181/10), indicating that there was relatively fast growth early in ontogeny. The femur on the other hand does not show any woven fibered matrix. Instead, the primary tissue of the cortex is entirely lamellar, suggesting that the overall growth was slow. An abundance of Sharpey's fibers in the intercentrum (ISIA 181/12; ) suggests well-developed musculature (CitationFrancillon-Vieillot et al., 1990; CitationRicqlès et al., 1991; CitationChinsamy-Turan, 2005) similar to that seen in large dicynodonts (CitationBotha, 2003; CitationBotha and Angielczyk, 2007). Sharpey's fibers have not been observed in the other elements examined in the present study.
Late Triassic Chigutisauridae—Only a single rib fragment (ISIA181/13) exhibits presence of woven fibered matrix locally in the mid to inner cortex. The rest of the cortex shows a predominance of lamellar zonal bone as the primary cortical tissue. In addition, the humerus (ISIA41) and the intercentrum show lamellar zonal bone in the cortex. Although a presence of woven fibered matrix in the rib indicates locally restricted fast growth, the overall cortical tissue pattern of all the specimens examined implies slow and periodic growth of the chigutisaurids in contrast to the other Triassic temnospondyl taxa examined. Moreover, the skeletal elements show extensive secondary reconstruction that occupies almost 90% of the cortex. This comprises profuse erosionally enlarged vascular channels. In the humerus, few secondary osteons are seen in the inner cortical region. In addition, near the periosteal periphery three LAGs are observed (), suggesting that growth stopped periodically. Presence of such peripheral rest lines and extensive secondary reconstruction indicates that the skeletal elements examined here belonged to adult individuals.
Synthesis
It is evident that there were striking differences in the growth patterns of the three Triassic temnospondyl taxa studied. Growth of the Early Triassic temnospondyls involved fast initial growth followed by a slowing down of growth later in ontogeny. In contrast, the Middle Triassic paracyclotosaurid and the Late Triassic chigutisaurid showed an overall slow growth, as evidenced by the predominance of lamellar bone. However, in all the taxa examined, the initial growth was rapid in comparison to later growth as seen in most extant and extinct tetrapods. This rapid growth in the juveniles may have helped to limit predation on the juvenile individuals (CitationWiffen et al., 1995; CitationSteyer et al., 2004; CitationCooper et al., 2008). Although lamellar bone has been previously recorded in fossil and extant amphibians (CitationDamiani, 2000; CitationErismis et al., 2002; CitationSteyer et al., 2004), fibrolamellar bone tissue is noted here for the first time. In general, fibrolamellar bone tissue is seen in higher tetrapods such as the dicynodonts and theriodont therapsids (e.g., Chinsamy and Rubidge, 1993; CitationBotha, 2003; Ray and CitationChinsamy, 2004; CitationBotha and Chinsamy, 2004, 2005; CitationRay et al., 2004, 2005; CitationChinsamy and Hurum, 2006) as well as in non-avian dinosaurs (e.g., CitationRicqlès et al., 1991; CitationCurry, 1999; CitationHorner et al., 2000, 2001). However, presence of fibrolamellar bone has been reported in young crocodilians such as Crocodylus and Gavialis (Chinsamy and Hillenius, 2004), and Alligator (CitationTumarkin-Deratzian, 2007).
The Early Triassic temnospondyls of the Panchet Formation, especially the trematosaurids (skull length ∼100 mm) were of small body size (CitationShishkin et al., 1996) and constituted a dominant member of the Panchet fauna (CitationTripathi, 1969). Small size was also noted for the Early Triassic dicynodont Lystrosaurus, another member of the Panchet fauna (CitationRay, 2005). The Damodar basin records a δ13C drop at the Permian-Triassic boundary, which can be correlated with a pronounced climatic and tectonic change (CitationSarkar et al., 2003). The surviving fauna of the Early Triassic Damodar basin may have undergone a similar reduction in size or ‘Lilliput effect’ to that found by CitationUrbanek (1993) in Upper Silurian graptoloids. Reduction in body size of several invertebrate and vertebrate taxa in the immediate aftermath of the end-Permian extinction was also documented by CitationTwitchett (2007). Small body size characterized the Early Triassic temnospondyls from Russia (CitationTverdokhlebov et al., 2002). In addition, the Early Triassic ecosystem of South Africa was dominated by small tetrapods (CitationSmith, 1995). Because the current study shows that the small-sized trematosaurid examined here had a fast growth, it is proposed that this fast growth could be linked to the invasion of the varied but empty Panchet ecological niches.
Another feature noted in most of the Early Triassic temnospondyls (including the trematosaurid) and the Middle Triassic paracyclotosaurids examined here, is the absence of LAGs (except in the humerus ISIA181/3 and ISIA181/11), implying a continuous growth pattern for both the families examined. This again contrasts with that seen in the extant lissamphibians where distinct zonation of the cortex by multiple LAGs constitutes its characteristic feature (CitationLaurin et al., 2004). Although there were multiple annuli suggesting periodic slowing down of growth characterized the Triassic temnospondyls from Australia, no LAGs were reported (CitationDamiani, 2000), indicating that growth never stopped. In contrast, the humerus (ISIA41) of the Indian chigutisaurid is characterized by multiple LAGs in the outer cortex similar to that observed in the femur of Dutuitosaurus (CitationSteyer et al., 2004), implying that growth was intermittent. LAGs and annuli are considered as genetically controlled, as well as strongly synchronized and reinforced by seasonality (CitationEsteban et al., 1999). They have been associated with environmental stress, as suggested by their occurrence in extant crocodilians (CitationHutton, 1986), some rodents (CitationKlevezal, 1996), and Arctic polar bears (CitationChinsamy et al., 1998). In contrast, a weak expression of growth rings has been found in frogs from areas of less continental climate (CitationEsteban et al., 1999). Hence, the presence of growth rings in a single element of each of the three taxa examined, namely the trematosaurid (ISIA181/3), paracyclotosaurid (ISIA 181/11), and chigutisaurid (ISIA41), may represent an individual response to a particularly stressful, unfavorable environmental stimulus. Such a stimulus was hypothesized in the non-mammalian cynodont Thrinaxodon where growth rings were absent apart from an annulus in a single individual (CitationBotha and Chinsamy, 2005).
Lifestyles
A widespread consensus is that an organism's lifestyle is reflected in the skeleton at various levels ranging from gross morphology to histological structure (CitationLaurin et al., 2004; CitationKriloff et al., 2008). External morphological features such as a streamlined body, presence of sensory line canals, paddle-like limbs, and an elongated and laterally flattened tail are suggestive of an aquatic lifestyle (CitationCarroll, 1988). A study on the cortical thickness (RBT%) of the extant mammals has shown that there is a direct relationship between thickness of the bone wall (cortex) and lifestyle adaptations (CitationWall, 1983). A 30% RBT was considered as a threshold to determine life habits; limb bones with greater than 30% RBT suggested an aquatic or a semi-aquatic lifestyle. CitationMagwene (1993) examined the cortical thickness of several crocodilian, lizard, non-mammalian therapsid, and mammalian femora and showed that the majority of the semi-aquatic animals examined had a cortical thickness of more than 30%. A similar study of extant and fossil reptiles also corroborated this hypothesis (e.g., CitationBotha, 2003), though Ray and CitationChinsamy (2004) showed convincingly that there are differing cortical thicknesses in the limb bones of a single individual depending on the functional constraints of the limb bones. A study by CitationKriloff et al. (2008) had shown that the high bone compactness (or cortical thickness) is usually found in aquatic taxa and is associated with a reduction in buoyancy because of increasing bone density. This high bone compactness is more apparent in fully aquatic taxa than amphibious taxa.
The ratio of the internal to the external diameter of the tubular bones (k-values, ) allows the bone mass to be minimized and depends on whether the bone is selected for yield or fatigue strength, for ultimate strength, for impact strength, or for stiffness (CitationCurrey and Alexander, 1985). Aquatic tetrapods that are not deep divers (e.g., alligator and manatee) have low values of k relative to terrestrial tetrapods. In addition, certain special features of the bone microstructure such as extensive medullary spongiosa and high cortical porosity (as in cetaceans), massive pachyostosis (mesosaurs, the dicynodont Lystrosaurus, sirenians), and the presence of calcified cartilage may suggest an aquatic lifestyle (CitationRicqlès, 1977; CitationDamiani, 2000; CitationSteyer et al., 2004; CitationRay et al., 2005).
Traditionally the evolution of the Mesozoic temnospondyls from their Paleozoic ancestors was accompanied by a change from a terrestrial to a more aquatic lifestyle (CitationCarroll, 1988). Gross modifications in the skeleton such as a flattened skull, decreased bone ossification, the presence of lateral line sensory canals, and an increase in the number of pre-sacral vertebrae were considered to support this hypothesis (CitationDefauw, 1989; cf. CitationDamiani, 2000). In general, the skeleton design of Triassic temnospondyls is found to be conservative, consisting of a large skull with a low/flattened lateral profile, a low body line with sprawling limb posture, and a long tail (CitationDamiani and Yates, 2003). An osteohistological study on some unidentified temnospondyls from the Early Triassic Arcadia Formation of Australia by CitationDamiani (2000) showed the absence of a free medullary cavity (osteosclerosis), extensive medullary spongiosa, and retention of calcified cartilage, and suggested an aquatic lifestyle.
The Triassic sediments of India were considered to have been deposited in a hot and arid climate with seasonal rainfall (CitationRobinson, 1970). This seasonal rainfall and aridity forced the temnospondyls to concentrate in certain pockets having water during the dry spells (CitationRobinson, 1971). Sedimentological and taphonomic data of the Indian temnospondyls examined in the present study suggest that these belonged to a lowland fauna inhabiting river banks, pond margins, and extensive flood plains. The trematosaurids were preserved in channel lag deposits and hence the possibility of them suffering a short transportation cannot be ruled out. In contrast, the paracyclotosaurids were collected from a lacustrine environment whereas the chigutisaurids constituted a dominant flood plain component. Within this broad picture of a lowland fauna, bone microstructure of the taxa examined reveals their occupation of more specific ecological niches.
The bone microstructure of the Triassic temnospondyls examined reveals a narrow cortex surrounding a distinct medullary region (–). In the Indian trematosaurid examined, the RBT of the humerus and femur is 23.37% and 10.12%, respectively, showing that these values are much less than the 30% threshold. Similar low RBT values are also shown by the indeterminate Early Triassic temnospondyls, the Middle Triassic paracyclotosaurids, and the Late Triassic chigutisaurid ().
Although calcified cartilage has not been identified in the skeletal elements, the absence of a free medullary cavity is noted in all the elements examined. However, this alone does not suggest an aquatic life style because the presence of an extensive medullary spongiosa has been noted in terrestrial dicynodonts (CitationBotha, 2003; CitationRay et al., 2005). Moreover, a gradual transition between the cortical compacta and medullary spongiosa characterizes aquatic taxa (CitationSteyer et al., 2004), which is not seen in the trematosaurid and paracyclotosaurid material examined here. In these taxa, the transverse sections show a distinct differentiation between the cortical compacta and medullary spongiosa. In addition, the studied taxa, except for the chigutisaurids (21.53%), have an average cortical porosity of about 6%, which contrasts with the high cortical porosity and cancellous appearance of the bone microstructure of the aquatic vertebrates. For example, the cortical porosity for a femoral mid-shaft of the Early Triassic aquatic dicynodont Lystrosaurus is 23%, giving it an almost cancellous appearance (CitationRay et al., 2005). Moreover, the average k-value is 0.64 in the Early and Middle Triassic temnospondyls examined, though the k-values of the femora (ISIA181/2 and ISIA181/8) were not considered because these were measured from metaphyseal sections. On the other hand, the humeral k-value of the chigutisaurid examined is 0.72; the k-value of the rib head (ISIA181/13) is not considered. A comparison of these k-values shows that the k-values of the Early and Middle Triassic temnospondyls studied in the present work are comparable with that of the land mammals that were examined by Curry and Alexander (1985). Hence, contrary to the earlier contention that all Triassic temnospondyls were essentially aquatic (CitationDamiani, 2000), we propose that the members of the Early Triassic taxa and the Middle Triassic paracyclotosaurid from India used to spend a considerable amount of time on land. It may be hypothesized that in the semi-arid Triassic environment with high seasonal rainfall, the temnospondyls examined were dependent on shallow pools or lakes and rivers, as well as retaining a high level of terrestriality to enable movement from one habitat to another, as suggested by CitationPawley and Warren (2005) for Lydekkerina huxleyi. This finding, based on bone microstructure, contrasts with the skeletal designs of the trematosaurids and paracyclotosaurids (CitationSchoch and Milner, 2000; CitationSteyer, 2002). However, CitationLaurin et al. (2004) in a recent study on Paleozoic temnospondyls have shown that these animals were terrestrial, and were secondarily adapted to water. Moreover, CitationDamiani et al. (2000) and CitationDamiani and Welman (2001) suggested that trematosaurids occupied varied ecological niches ranging from near shore regions to fluvial settings.
The humerus (ISIA41) of the chigutisaurid examined here shows a higher k-value in comparison to the other forms, indicating a large medullary region, which is completely filled with bony trabeculae of the cancellous bone. In addition, ISIA41 exhibits a high cortical porosity (21.53%) because of extensive secondary reconstruction, where about 90% of the cortex contains erosionally enlarged vascular channels. Similar cancellous appearance of the cortex is seen in aquatic animals such as the delphinid cetaceans (Buffrénil and Schoevaert, 1988), marine turtles (CitationRhodin, 1985), and mosasaurs (CitationSheldon, 1997), implying that the chigutisaurids had a similar semi-aquatic or aquatic lifestyle.
FIGURE 8 Comparative bar diagram showing cortical thickness (RBT%) in different skeletal elements of the temnospondyls.
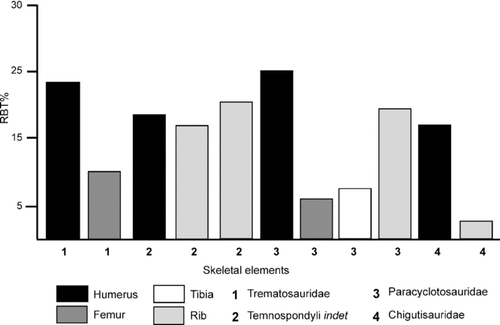
Hence, the bone microstructure reflects ecological differentiation of the Triassic temnospondyls examined, where the trematosaurids and paracyclotosaurids are considered to retain a high degree of terrestriality, contrary to earlier belief, and the chigutisaurids had a semi-aquatic or aquatic habitat. However, an interesting feature noted in the paracyclotosaurids is the relatively low RBT values of the hind limb bones (femur and tibia) in comparison to the humerus (). This feature may be attributed to differential biomechanical constraints, especially during walking on land. These giant predators (CitationSchoch and Milner, 2000) walked in water by touching the ground with their digits (CitationWatson, 1958) and had neutral buoyancy; the limbs did not need to provide any postural support. The paracyclotosaurids had a long skull (skull length [SL]:skull width [SW]:skull height [SH] = 5:3.2:1; CitationMukherjee and Sengupta, 1998) in comparison to those of the trematosaurids (small, SL of a few centimeters, SW:SH = 4.5:1; CitationDamiani and Yates, 2003) and the chigutisaurid Compsocerops cosgriffi (medium-sized, SL:SW:SH = 2.3:2.88:1; CitationSengupta, 1995). The long skull in paracyclotosaurids must have resulted in the forward displacement of the center of gravity when the walking mode on land is considered (CitationParrington, 1967). Hence, skeletal design, bone microstructure (e.g., Sharpey's fibers in the intercentrum), and lifestyle suggest that the forelimbs of the paracyclotosaurids possibly had to provide more postural support to keep the anterior part of the body well off the ground in comparison to the hind limbs, resulting in a relatively thicker humeral cortex.
CONCLUSIONS
-
Preliminary but new observations on the bone microstructure of the Indian Triassic temnospondyls reveal differing growth patterns. However, the number of taxa and specimens examined is too small in comparison to the known diversity of Mesozoic temnospondyls and more work needs to be done for verification of the hypotheses addressed in the present work.
-
In the Early Triassic trematosaurid, the presence of fibrolamellar bone tissue, which indicates rapid osteogenesis and an overall fast growth, is noted. The overall growth strategy of this trematosaurid involved fast initial growth in the juvenile stage, which decreased in the sub-adult and adult stages, as implied by the presence of peripheral lamellar bone in the outer cortex. Growth became intermittent as evidenced by the presence of LAGs, although in the indeterminate taxa examined growth was sustained.
-
The Middle Triassic paracyclotosaurids showed a predominance of lamellar bone, suggesting an overall slow growth strategy. However, relatively fast growth in the early stage of ontogeny is implied by the presence of woven fibered bone in the humerus and rib. The examined Late Triassic chigutisaurids are characterized by the predominance of lamellar bone and the presence of multiple LAGs, indicating slow growth and a periodic cessation of growth, respectively.
-
Although their body plan suggests that the temnospondyls were essentially aquatic, cortical thickness, extent of the medullary region (k-values), absence of calcified cartilage, and low-to-moderate cortical porosity indicate that the trematosaurids and paracyclotosaurids examined retained a high level of terrestriality. In contrast, the relatively high cortical porosity and extensive medullary spongiosa point towards a semi-aquatic or aquatic lifestyle for the chigutisaurids.
-
The relatively high cortical thickness of the forelimb with respect to the hind limb of the paracyclotosaurids is attributed to differing biomechanical constraints. A long and massive skull suggests an anterior shift in the center of gravity, whereby the forelimbs were probably used as struts for holding the anterior part of the body well off the ground during walking. This resulted in a relatively thicker humeral cortex in comparison to the cortices of the hind limbs.
ACKNOWLEDGMENTS
We thank K. Sen, Wadia Institute of Himalayan Geology, India, and the late U. Sarkar for technical assistance. The constructive criticisms by J. Sébastian Steyer, Museum National d’Histoire Naturelle, France, two anonymous reviewers, and A. Warren, La Trobe University, Australia, are gratefully acknowledged. The Sponsored Research and Industrial Consultancy, Indian Institute of Technology, Kharagpur, Indian Statistical Institute, Kolkata, and Department of Science and Technology, New Delhi, provided financial support and infrastructural facilities.
LITERATURE CITED
- Amprino , R. 1947 . La structure du tissu osseux envisagée comme expression de différences dans la vitesse de l’ccroissement . Archives de Biologie , 58 : 315 – 330 .
- Bandyopadhyay , S. 1999 . Gondwana vertebrate faunas of India . Proceedings of the Indian National Science Academy , 65A : 285 – 313 .
- Bandyopadhyay , S. and Sengupta , D. P. 2006 . “ Vertebrate faunal turnover during the Triassic Jurassic transition: an Indian scenario; pp ” . In The Triassic-Jurassic Terrestrial Transition , Edited by: Harris , J. , Lucas , S. G. , Spielmann , J. A. , Lockley , M. G. , Milner , A. R. C. and Kirkland , J. I. 77 – 85 . New Mexico Museum of Natural History and Science Bulletin 37 .
- Bandyopadhyay , S. , RoyChowdhury , T. K. and Sengupta , D. P. 2002 . Taphonomy of some Gondwana vertebrate assemblages of India . Sedimentary Geology , 147 : 219 – 245 .
- Botha , J. 2003 . Biological aspects of the Permian dicynodont Oudenodon (Therapsida: Dicynodontia) deduced from bone histology and cross-sectional geometry . Palaeontologia Africana , 39 : 37 – 44 .
- Botha , J. and Angielczyk , K. D. 2007 . An integrative approach to distinguishing the Late Permian dicynodont species Oudenodon bainii and Tropidostoma microtrema (Therapsida, Anomodontia) . Palaeontology , 50 : 1175 – 1209 .
- Botha , J. and Chinsamy , A. 2004 . Growth and life habits of the Triassic cynodont Trirachodon, inferred from bone histology . Acta Paleontologica Polonica , 49 : 619 – 627 .
- Botha , J. and Chinsamy , A. 2005 . Growth patterns of Thrinaxodon liorhinus, a non-mammalian cynodont from the Lower Triassic of South Africa . Palaeontology , 48 : 385 – 394 .
- Buffrénil , V. de and Schoevart , D. 1988 . On how the periosteal bone of the delphinid humerus becomes cancellous: ontogeny of a histological specialization . Journal of Morphology , 198 : 149 – 164 .
- Bühler , P . 1986 . Das Vogelskellet—hochentwickelter knochen-leichtbau . Arcus , 5 : 221 – 228 .
- Carroll , R. L. 1988 . Vertebrate Paleontology and Evolution , 698 New York : Freeman .
- Chakraborty , C. , Mandal , N. and Ghosh , S. 2003 . Kinematics of the Gondwana basins of peninsular India . Tectonophysics , 377 : 299 – 324 .
- Chinsamy , A. 1993 . Image analysis and the physiological implications of the vascularization of femora in archosaurs . Modern Geology , 19 : 101 – 108 .
- Chinsamy , A. 1997 . Assessing the biology of fossil vertebrates through bone histology . Palaeontologia Africana , 33 : 29 – 35 .
- Chinsamy-Turan , A. 2005 . The Microstructure of Dinosaur Bone: Deciphering Biology with Fine-Scale Techniques , 195 Baltimore : The Johns Hopkins University Press .
- Chinsamy , A. 2004 . “ Physiology of non-avian dinosaurs; pp ” . In The Dinosauria, second edition , Edited by: Weishampel , D. B. , Dodson , P. , Osmólska , H. and Hillenius , W. J. 643 – 659 . Berkeley, , California : University of California Press .
- Chinsamy , A. and Hurum , J. H. 2006 . Bone microstructure and growth patterns of early mammals . Acta Paleontologica Polonica , 51 : 325 – 338 .
- Chinsamy , A. and Raath , M. A. 1992 . Preparation of fossil bone for histological examination . Palaeontologia Africana , 29 : 39 – 44 .
- Chinsamy , A. and Rubidge , B. S. 1993. Dicynodont (Therapsida) bone histology: phylogenetic and physiological implications . Palaeontologia Africana , 30 97 – 102 .
- Chinsamy , A. , Rich , T. and Vickers-Rich , P. 1998 . Polar dinosaur bone histology . Journal of Vertebrate Paleontology , 18 : 385 – 390 .
- Cooper , L. N. , Lee , A. H. , Taper , M. L. and Horner , J. R. 2008 . Relative growth rates of predator and prey dinosaurs reflect effects of predation . Proceeding of the Royal Society B , 275 : 2609 – 2615 .
- Curry , K. A. 1999 . Ontogenetic histology of Apatosaurus (Dinosauria: Sauropoda): new insights on growth rates and longevity . Journal of Vertebrate Paleontology , 19 : 654 – 665 .
- Currey , J. D. and Alexander , R. M. 1985 . The thickness of tubular bones . Journal of Zoology , 206 : 453 – 468 .
- Damiani , R. J. 2000 . Bone histology of some Australian Triassic temnospondyl amphibians: preliminary data . Modern Geology , 24 : 109 – 124 .
- Damiani , R. J. 2004 . Temnospondyls from the Beaufort Group (Karoo Basin) of South Africa and their biostratigraphy . Gondwana Research , 7 : 165 – 173 .
- Damiani , R. J. and Welman , J. 2001 . A long-snouted trematosaurid amphibian from the Early Triassic of South Africa . South African Journal of Science , 97 : 318 – 319 .
- Damiani , R. J. and Yates , A. M. 2003 . The Triassic amphibian Thoosuchus yakovlevi and the relationships of the Trematosauroidea (Temnospondyli: Stereospondyli) . Records of the Australian Museum , 55 : 331 – 342 .
- Damiani , R. J. , Neveling , J. , Hancox , J. and Rubidge , B. S. 2000 . First trematosaurid temnospondyl from the Lystrosaurus Assemblage Zone of South Africa and its biostratigraphic implications . Geological Magazine , 137 : 659 – 665 .
- Defauw , S. L. 1989 . Temnospondyl Amphibians: a new perspective on the last phases in the evolution of the Labyrinthodontia . Michigan Academician , 21 : 7 – 22 .
- Dutuit , J. M. and Heyler , D. 1975 . Présence de cellules d’évidement dans les os de l’arrière-crâne de deux Stégocéphales triasiques . Colloques Centre National de la Researche Scientifique, Problèmes Actuels de Paléeontologie; Évolution des Vertébrés , 218 : 331 – 336 .
- Enlow , D. H. 1963 . Principles of Bone Remodelling , 123 Illinois : Charles C. Thomas, Springfield .
- Enlow , D. H. 1969 . “ The bones of reptiles; pp ” . In Biology of the Reptilia 1 , Edited by: Gans , C. 45 – 80 . New York : Academic Press .
- Erismis , U. C. , Kaya , U. and Arikan , H. 2002 . Observations on the histomorphological structure of some long bones of the water frog (Rana bedriagae) from the Izmir area . Turkish Journal of Zoology , 26 : 213 – 216 .
- Esteban , M. , García-Paris , M. , Buckley , D. and Castanet , J. 1999 . Bone growth and age in Rana saharica, a water frog living in desert environment . Annales Zoologici Fennici , 36 : 53 – 62 .
- Francillon-Vieillot , H. , de Buffrénil , V. , Castanet , J. , Gerandie , J. , Meunier , F. J. , Sire , J. Y. , Zylberberg , L. L. and Ricqlès , A. 1990 . “ Microstructure and mineralization of vertebrate skeletal tissues; pp ” . In Skeletal Biomineralization: Patterns, Process and Evolutionary Trends 1 , Edited by: Carter , J. G. 471 – 530 . New York : Van Nostrand Reinhold .
- Ghosh , P. , Sarkar , S. and Maulik , P. 2006 . Sedimentology of a muddy alluvial deposit: Triassic Denwa Formation, India . Sedimentary Geology , 191 : 3 – 36 .
- Horner , J. R. , Padian , K. and de Ricqlès , A. 2001 . Comparatve osteohistology of some embryonic and perinatal archosaurs: developmental and behavioral implications for dinosaurs . Paleobiology , 27 : 39 – 58 .
- Horner , J. R. , de Ricqlès , A. and Padian , K. 2000 . Long bone histology of the hadrosaurid dinosaur Maiasaura peeblesorum: growth dynamics and physiology based on an ontogenetic series of skeletal elements . Journal of Vertebrate Paleontology , 20 : 115 – 129 .
- Hutton , J. M. 1986 . Age determination of living Nile crocodiles from the cortical stratification of bone . Copeia , 1986 : 332 – 341 .
- Huxley , T. H. 1865 . On vertebrate fossils from the Panchet rocks near Raniganj, Bengal . Paleontologica Indica , 4 : 1 – 24 .
- Klevezal , G. A. 1996 . Recording Structures of Mammals , 274 Brookfield, Vermont : A. A. Balkema Publishers .
- Kriloff , A. , Germain , D. , Canoville , A. , Vincent , P. , Sache , M. and Laurin , M. 2008 . Evolution of bone microanatomy of the tetrapod tibia and its use in paleobiological inference . Journal of Evolutionary Biology , 21 : 807 – 826 .
- Kutty , T. S. and Sengupta , D. P. 1989 . The Late Triassic formations of the Pranhita-Godavari valley and their vertebrate succession—a reappraisal . Indian journal of Earth Sciences , 16 : 189 – 206 .
- Kutty , T. S. , Jain , S. L. and RoyChowdhury , T. 1987 . Gondwana sequence of the northern Pranhita-Godavari valley; its stratigraphy and vertebrate faunas . Paleobotanist , 36 : 214 – 229 .
- Laurin , M. , Girondot , M. and Loth , M. M. 2004 . The evolution of long bone microstructure and lifestyle in lissamphibians . Paleobiology , 30 : 589 – 613 .
- Magwene , P. M. 1993 . What's bred in the bone: histology and cross sectional geometry of mammal-like reptile long bones-evidence of changing physiological and biomechanical demands , 93 Cambridge, Massachusetts : Unpublished M.Sc. thesis, Harvard University .
- Margerie , E. de , Cubo , J. and Castanet , J. 2002 . Bone typology and growth rate: testing and quantifying ‘Amprino's rule’ in the mallard (Anas platyrhynchos) . Les Comptes Rendus de l’ Académie des sciences, Série Biologies , 325 : 221 – 230 .
- Milner , A. R . 1993 . The Paleozoic relatives of lissamphibians . Herpetological Monographs , 7 : 8 – 27 .
- Mukherjee , R. N. and Sengupta , D. P. 1998 . New capitosaurid amphibians from the Middle Triassic Denwa Formation of Satpura Gondwanas, Central India . Alcheringa , 22 : 317 – 327 .
- Mukherjee , D. , Ray , S. and Sengupta , D. P. 2008 . Differing growth patterns and inferred life habits of the Triassic temnospondyls from India; p. 75 in Program and Abstracts of International Conference on Geology: Indian Scenario and Global Context . 7–11 January 2008, Kolkata, India.
- Padian , K. 1997 . “ Growth lines ” . In Encyclopedia of Dinosaurs , Edited by: Currie , P. J. and Padian , K. 288 – 291 . San Diego, , California : Academic Press .
- Parrington , F. R. 1967 . The vertebrae of early tetrapods . Colloques International du Centre National de la Researche Scientifique , 163 : 1 – 269 .
- Pawley , K. and Warren , A. 2005 . A terrestrial stereospondyl from the Lower Triassic of South Africa: the postcranial skeleton of Lydekkerina huxleyi (Amphibia: Temnospondyli) . Palaeontology , 48 : 281 – 298 .
- Ray , S. 2005 . Lystrosaurus (Therapsida, Dicynodontia) from India: taxonomy, relative growth and cranial dimorphism . Journal of Systematic Palaeontology , 3 : 203 – 221 .
- Ray , S. and Chinsamy , A. 2004 . Diictodon feliceps (Therapsida, Dicynodontia): bone histology, growth and biomechanics . Journal of Vertebrate Palaeontology , 24 : 180 – 194 .
- Ray , S. , Botha , J. and Chinsamy , A. 2004 . Bone histology and growth patterns of some nonmammalian therapsids . Journal of Vertebrate Paleontology , 24 : 634 – 648 .
- Ray , S. , Chinsamy , A. and Bandyopadhyay , S. 2005 . Lystrosaurus murrayi (Therapsida, Dicynodontia): bone histology, growth and lifestyle adaptations . Palaeontology , 48 : 1 – 17 .
- Rhodin , A. G. J. 1985 . Comparative chondro-osseous development and growth of marine turtles . Copeia , 3 : 752 – 771 .
- de Ricqlès , A. 1969 . Recherches paléohistologiques sur les os longs des Tétrapodes. II, quelques observations sur la structure des longs des Thériodontes . Annales de Paléontologie (Vertébrés) , 55 : 1 – 52 .
- Ricqlès , A. de . 1972 . Recherches paléohistologiques sur les os longs des Tétrapodes. III, Titanosuchiens, Dinocéphales et Dicynodontes . Annales de Paléontologie (Vertébrés) , 58 : 17 – 60 .
- Ricqlès , A. de . 1975 . Quelques remarques paléohistologiques sur le problème de la néoténie chez les Stégocéphales . Colloqium Internationale Centre National de la Researche Scientifique , 218 : 351 – 363 .
- Ricqlès , A. de . 1977 . Recherches paléohistologiques sur les os longes des tétrapodes. VII. Sur la classification, la signification fonctionnelle et l’histoire des tissues osseus des tétrapodes (Deuxième partie suite.) . Annales de Paléontologie (Vertébrés) , 63 : 33 – 56 .
- Ricqlès , A. de. 1978 . Recherches paléohistologiques sur les os longs de tétrapodes: VII. Sur la classification, la signification fonctionnelle et l’histoire des tissus osseux des tétrapodes. Part. 3: Evolution et bibliographie analytique . Annales de Paléontologie , 64 : 5 – 111 .
- Ricqlès , A. de. 1979 . Relations entre structures histologiques, ontogenèses, stratégies démographiques et modalités évolutives: le cas des Reptiles Captorhinomorphes et Stégocéphales temnospondyles . Comptes rendus de l’Académie des Sciences, Paris , 288 : 1147 – 1150 .
- Ricqlès , A. de. 1989 . Les mécanismes hétérochroniques dans le retour des tétrapodes au milieu aquatiue . Geobios mémoire spécial , 12 : 337 – 348 .
- Ricqlès , A. de , Meunier , F. J. , Castanet , J. and Francilon-Vieillot , H. 1991 . “ Comparative microstructure of bone; pp ” . In Bone 3: Bone Matrix and Bone Specific Products , Edited by: Hall , B. K. 1 – 77 . Boca Raton, , Florida : CRC Press Inc. .
- Robinson , P. L. 1970 . The Indian Gondwana formations–a review; pp . Proceedings of 1st IUGS International Symposium on Gondwana Stratigraphy and Paleontology , : 201 – 268 . September 1967, Ar Del Plata, Argentina.
- Robinson , P. L. 1971 . A problem of faunal replacement on Permo-Triassic continents . Palaeontology , 14 : 131 – 153 .
- RoyChowdhury , T. K. 1965 . A new metoposaurid amphibian from the upper Triassic Maleri Formation of Central India . Philosophical transactions of the Royal Society of London B , 250 : 1 – 52 .
- Ruta , M. , Pisani , D. , Llyod , G. T. and Benton , M. J. 2007 . A supertree of Temnospondyli:cladogenetic patterns in the most species-rich group of early tetrapods . Proceedings of the Royal Society of London B , 274 : 3087 – 3095 .
- Sarkar , A. , Yoshioka , H. , Ebihara , M. and Naraoka , H. 2003 . Geochemical and organic carbon isotope studies across the continental Permo–Triassic boundary of Raniganj Basin, eastern India . Palaeogeography, Palaeoclimatology, Palaeoecology , 191 : 1 – 14 .
- Sanchez , S. , Klembara , J. , Castanet , J. and Steyer , J. S. 2008 . Salamander-like development in a seymouriamorph revealed by paleohistology . Biology Letters , 4 : 411 – 414 .
- Sander , P. M. 2000 . Long bone histology of the Tendaguru sauropods: an implication for growth and biology . Paleobiology , 26 : 466 – 488 .
- Schoch , R. R. and Milner , A. R. 2000 . “ Stereospondyli ” . In Encyclopedia of Paleoherpetology , Edited by: Wellnhofer , P. 203 München : Verlag Dr. Friedrich Pfeil .
- Sengupta , D. P. 1995 . Chigutisaurid temnospondyls from the Late Triassic of India and a review of the family Chigutisauridae . Palaeontology , 38 : 313 – 339 .
- Sengupta , D. P. 2002 . Indian Metoposaurid Amphibians revised . Paleontological Research , 6 : 41 – 65 .
- Sengupta , D. P. 2003 . Triassic temnospondyls of the Pranhita-Godavari basin, India . Journal of Asian Earth Sciences , 21 : 655 – 662 .
- Sengupta , S. M. 1970 . Gondwana sedimentation around Bheemaram (Bhimaram), Pranhita-Godavari valley, India . Journal of Sedimentary Petrology , 40 : 140 – 170 .
- Sheldon , M. A . 1997 . “ Ecological implications of mosasaur bone microstructure; pp ” . In Ancient Marine Reptiles , Edited by: Callaway , J. M. and Nicholls , E. L. 333 – 354 . New York : Academic Press .
- Shishkin , M. A. , Rubidge , B. S. and Kitching , J. W. 1996 . A new lydekkerinid (Amphibia, Temnospondyli) from the Lower Triassic of South Africa: implications for evolution of the early capitosauroid cranial pattern . Philosophical Transactions of the Royal Society of London B , 351 : 1635 – 1659 .
- Smith , R. M. H. 1995 . Changing fluvial environments across the Permian-Triassic boundary in the Karoo basin, South Africa, and possible causes of tetrapod extinctions . Paleogeography, Paleoclimatology, Paleoecology , 117 : 81 – 104 .
- Starck , J. M. and Chinsamy , A. 2002 . Bone microstructure and developmental plasticity in birds and other dinosaurs . Journal of Morphology , 254 : 232 – 246 .
- Steyer , J. S. 2002 . The first articulated trematosaur ‘amphibian’ from the Lower Triassic of Madagascar: implications for the phylogeny of the group . Palaeontology , 45 : 771 – 793 .
- Steyer , J. S. and Damiani , R. J. 2005 . A giant brachyopoid temnospondyl from the Upper Triassic or Lower Jurassic of Lesotho . Bulletin de la Société Géologique de France , 176 : 243 – 248 .
- Steyer , J. S. , Laurin , M. , Castanet , J. and de Ricqlès , A. 2004. First histological and skeletochronological data on temnospondyl growth: paleogeological and paleoclimatological implications . Paleogeography, Paleoclimatology, Paleoecology , 206 193 – 201 .
- Tripathi , C. 1969 . Fossil labyrinthodonts from the Panchet Series of the Indian Gondwanas . Palaeontologia Indica , 38 : 1 – 45 .
- Tumarkin-Deratzian , A. R. 2007 . Fibrolamellar bone in wild adult . Alligator mississippiensis. Journal of Herpetology , 41 : 341 – 345 .
- Tumarkin-Deratzian , A. R. , Vann , D. R. and Dodson , P. 2006 . Bone surface texture as an ontogenetic indicator in long bones of the Canada goose Branta canadensis (Anseriformes: Anatidae) . Zoological Journal of the Linnean Society , 148 : 133 – 168 .
- Tverdokhlebov , V. P. , Tverdokhlebov , G. I. , Surkov , M. V. and Benton , M. J. 2002 . Tetrapod localities from the Triassic of the SE of European Russia . Earth Science Reviews , 60 : 1 – 66 .
- Twitchett , R. J. 2007 . The Lilliput effect in the aftermath of the end-Permian extinction event . Paleogeography, Paleoclimatology, Paleoecology , 252 : 132 – 144 .
- Urbanek , A. 1993 . Biotic crisis in the history of the Upper Silurian graptoloids: a paleobiological model . Historical Biology , 7 : 29 – 50 .
- Veevers , J. J. and Tewari , R. C. 1995 . Gondwana master basin of peninsular India between Tethys and the interior of the Gondwanaland province of Pangea . Geological Society of America Memoir , 187 : 1 – 72 .
- Wall , W. P. 1983 . The correlation between high limb bone density and aquatic habits in recent mammals . Journal of Paleontology , 57 : 197 – 207 .
- Watson , D. M. S. 1958 . A new labyrinthodont (Paracyclotosaurus) from the Upper Trias of New South Wales . Bulletin of the British Museum of Natural History , 3 : 233 – 263 .
- Wiffen , J. , de Buffrénil , V. , de Ricqlés , A. and Mazin , J. M. 1995. Ontogenetic evolution of bone structure in Late Cretaceous Plesiosauria from New Zealand . Geobios , 28 625 – 640 .
- Yates , A. M. and Warren , A. A. 2000 . The phylogeny of the “higher” temnospondyls (Vertebrata: Choanata) and its implications for the monophyly and origins of Stereospondyla . Zoological Journal of the Linnean Society , 128 : 77 – 121 .