ABSTRACT
The oldest reported fossil record of Delphinidae is from the late Miocene (11 Ma) of California. Reliable Miocene fossil delphinids, however, are few. Eodelphis kabatensis from the upper Miocene Mashike Formation (8.5-13.0 Ma), Hokkaido, northern Japan, is the oldest described Miocene delphinid including a skull. Therefore, this species is a significant clue to understanding the early evolutionary history of Delphinidae. The original taxonomic assignment of this species within the genus Stenella is questionable; thus, we propose a new combination for the species, Eodelphis kabatensis Horikawa, 1977. Eodelphis is a basal delphinid, and comprehensive morphological cladistic analysis, including molecular topological constraints, supported this taxonomic revision. Paleobiogeographic analyses based on the present morphological cladistic analysis and analysis under the molecular constraints suggest that the origin and early diversification of Delphinidae occurred in the middle Miocene Pacific Ocean or elsewhere, respectively.
SUPPLEMENTAL DATA—Supplemental materials are available for this article for free at http://www.tandfonline.com/UJVP.
INTRODUCTION
Delphinidae (Cetacea, Odontoceti, Delphinoidea), including 36 living species, is not only the most diverse family of cetaceans but also marine mammals as a whole (Committee on Taxonomy, Citation2012). The family includes familiar species such as bottlenose dolphin, spinner dolphin, killer whale, and Risso's dolphin. In addition, an enormous number of fossil species have been described (e.g., Tursiops miocaenus Portis, 1885, and Steno cudmorei Chapman, Citation1917). Most of those species, however, were dubiously assigned to Delphinidae (e.g., Portis, 1885; Chapman, Citation1917). Nowadays, we suggest 12 reliable fossil delphinid species established on the basis of diagnostic skull remains (). Most of these species come from the Pliocene of Italy, although it is thought that the oldest fossil record of Delphinidae is from the late Miocene of California (LACM 52147; Barnes, Citation1977). As pointed out by Ichishima (Citation2005), we cannot determine whether LACM 52147 belongs to Delphinidae or not in a modern sense, because LACM 52147 has not been described. Furthermore, most other fossil records of Miocene delphinids are nomina dubia (e.g., Tursiops miocaenus Portis, 1885, and Steno cudmorei Chapman, Citation1917) or fragmentary (tooth, periotic, or mandible fragment; e.g., Fordyce, Citation1982, Citation1984; Fulgosi and Pilleri, Citation1985; Muizon, Citation1988b; Pilleri, Citation1988). Among those Miocene delphinids, the North Atlantic Ocean and the Mediterranean Sea fossils are no longer regarded as delphinids (Bianucci and Landini, Citation2002). Thus, Miocene delphinids are steeped in mystery, although molecular phylogenetic analyses strongly indicate the diversification of Delphinidae during the late Miocene (e.g., Steeman et al., Citation2009).
In a review of Japanese cetacean fossils, Ichishima (Citation2005) stated that Stenella kabatensis Horikawa, Citation1977, from the upper Miocene Mashike Formation (8.5–13.0 Ma), Hokkaido, Japan, is the only described Miocene delphinid including a skull. Consequently, this species is important to understanding the early evolutionary history of Delphinidae. Nevertheless, this species languished in obscurity outside Japan (but see Archer and Perrin, Citation1999), because the original description was written in Japanese. Horikawa (Citation1977) ascertained that S. kabatensis belonged to Delphinidae (sensu Nishiwaki, Citation1965) and the genus Stenella Gray, Citation1866. Yet there are no adequate grounds for this referral due to the fragmentary nature of the rostrum in the holotype, despite Horikawa (Citation1977:109) stating that “the holotype was a long beaked dolphin based on the morphology of the skull.” Ichishima (Citation2005) questioned the attribution of S. kabatensis to Stenella, and noted the necessity for reexamination of this species, because (1) the suture between palatines and pterygoids in the original description differs from all other delphinids; (2) species for comparison with S. kabatensis are extremely limited; and (3) no diagnostic characters of Stenella were found in the holotype.
Table 1 Valid fossil delphinid species that have been discovered with the skull.
The genus Stenella includes five extant species: S. attenuata Gray, Citation1846; S. clymene Gray, Citation1850; S. coeruleoalba Meyen, Citation1833; S. frontalis Cuvier, Citation1829; and S. longirostris Gray, Citation1828. One extinct species has been named, S. rayi Whitmore and Kaltenbach, Citation2008. As Xiong et al. (Citation2009) noted, however, the monophyly of Stenella has been questioned since the 19th century (True, Citation1889; Hershkovitz, Citation1966). Several morphological studies (e.g., Perrin et al., Citation1981, Citation1987) suggested that the genus probably represents an artificial assemblage, some of which may actually be more closely related to Tursiops or Delphinus than to their congeners. However, a morphological cladistic analysis of Delphinidae at the species level has not been attempted until very recently: Bianucci (Citation2013) performed molecular constraint morphological cladistic analysis of Delphininae. LeDuc et al. (Citation1999) confirmed paraphyly of Stenella and Tursiops based on cytochrome b sequence in nearly all delphinids. Furthermore, the clade Stenella + Tursiops + Delphinus includes two other genera, Sousa and Lagenodelphis (LeDuc et al., Citation1999). These complicated relationships have been called the Stenella-Delphinus-Tursiops complex (Caballero et al., Citation2008) or the Sousa-Delphinus-Tursiops-Stenella complex (Xiong et al., Citation2009); hereafter we refer to these complexes as the Stenella complex. Most molecular phylogenetic analyses corroborate these complexes and polyphyly of Stenella, although branches within the Stenella complex do not reach the consensus (e.g., May-Collado and Agnarsson, Citation2006; McGowen et al., Citation2009; Steeman et al., Citation2009; Slater et al., Citation2010; McGowen, Citation2011). In addition, new extant species of Tursiops, T. australis Charlton-Robb et al., Citation2011, and three extinct species, T. osennae Simonelli, Citation1911, Hemisyntrachelus oligodon (Pilleri and Siber, Citation1989), and Etruridelphis giulii (Lawley, Citation1876), may be included in the complex, although H. oligodon and E. giulii have been removed from Tursiops and Stenella, respectively (Post and Bosselaers, Citation2005; Bianucci et al., Citation2009). These complicated taxonomic issues highlight the need for revision of S. kabatensis in light of a new phylogenetic analysis.
The main explanation for unresolved relationships of the Stenella complex and Delphinidae is their recent rapid radiation (Kingston et al., Citation2009; McGowen, Citation2011) between 2.35 and 5.0 Ma (McGowen et al., Citation2009; Steeman et al., Citation2009; Xiong et al., Citation2009). Yet the age of S. kabatensis is estimated at 8.5–13.0 Ma. This range is not only older than the estimated origin of the Stenella complex but also approximates the putatively earliest true dolphin (11 Ma; Barnes, Citation1977), and the divergence of Delphinidae estimated from molecular evidence (between 9.89 and 12 Ma; Cassens et al., Citation2000; McGowen et al., Citation2009; Steeman et al., Citation2009; Slater et al., Citation2010; Chen et al., Citation2011). If S. kabatensis is included in the genus Stenella or the Stenella complex, it would necessitate reconsideration of the evolutionary history of Delphinidae. Thus, reexamination of the phylogenetic position of S. kabatensis is needed. S. kabatensis was located much more basal than the Stenella complex in previous morphological cladistic analyses (Murakami et al., Citation2012a, Citation2012b). However, those analyses included only one species per each genus of the complex. Consequently, we confirm the phylogenetic position of S. kabatensis by reexamining a cladistic analysis including most species of that complex. At the same time, we redescribe the species and establish a new genus for S. kabatensis.
SYSTEMATIC PALEONTOLOGY
CETACEA Brisson, Citation1762
ODONTOCETI Flower, Citation1867
DELPHINOIDEA Gray, Citation1821
DELPHINIDAE Gray, Citation1821
EODELPHIS, new genus
Type and Only Known Species
Eodelphis kabatensis (new combination).
Diagnosis
As for the type species.
Etymology
From the Ancient Greek ‘Eo,’ for dawn referring to the earliest delphinid; and from Latin ‘delphis,’ for dolphin.
EODELPHIS KABATENSIS (Horikawa, Citation1977), new combination
(Figs. 2–6)
Delphinidae, gen. et sp. indet. Horikawa and Fujita, Citation1972:177, pl. 1.
Stenella kabatensis: Horikawa, Citation1977:98, figs. 2–8, pls. 1, 2.
Delphinidae, gen. indet. Ichishima, Citation2005:11.
Holotype
HMH 68037; partial skull (lacking rostrum and vertex), with right and left mandibles, isolated teeth, right and left tympano-periotics, basihyal, thyrohyals, and stylohyal. Contrary to the original description, the lacrimal, nasal, and supraoccipital are not preserved.
Emended Diagnosis
Differing from all delphinids by having a medially positioned premaxillary foramen, a partially transversely directed suture line between the palatine and pterygoid on the palate, and prominently long and ventrolaterally extended posterior process of the periotic with the attenuating posterior bullar facet. Differing from all delphinids except Orcinus and Hemisyntrachelus spp. by having a wide ascending process of the left premaxilla relative to the right premaxilla at level of the middle of the external nares. Differing from all delphinids except Australodelphis and Hemisyntrachelus oligodon by possessing a right premaxilla with a nearly straight lateral edge from the antorbital notch to the level of the middle of the external nares. Differing from all delphinids except Australodelphis by possessing a left premaxilla that prominently overhangs the maxilla. Differing from all delphinids except Pseudorca, Orcaella, Orcinus, and Sotalia by having a long lateral lamina of palatine extending beyond the level of the anterior margin of the frontal groove. Differing from all delphinids except Astadelphis, Hemisyntrachelus, Lagenorhynchus obliquidens, Orcinus, Pseudorca, Sousa, and Steno by having a dorsoventrally deep mandible.
Locality and Age
The holotype was collected in a small tributary valley of the Oshirarika River, Uryu Town, Hokkaido, Japan (43°40′40″N, 141°41′10″E; Horikawa and Fujita, Citation1972; ). This area corresponds to the east limb of Nobusha anticline, and Neogene Mashike and Rumoi formations are exposed in ascending order (Sato et al., Citation1964). The Togeshita Formation, underlying the Mashike Formation and partially contemporaneous heterotopic facies with the base of the lower mudstone unit of the Mashike Formation, is not exposed in this area (). The Mashike Formation is divided into the mudstone, the sandstone, and so-called hard shale units in ascending order (Sato et al., Citation1964). The mudstone unit (100–700 m in thickness) mainly consists of dark gray massive soft mudstone and sandy mudstone, although thin beds of gray or blue gray soft fine sandstone are intercalated with the mudstone and sandy mudstone (Sato et al., Citation1964). The sandstone unit (20–250 m in thickness) is composed of dark gray or gray massive medium to fine sandstone bearing white pumice and abundant glauconite (Sato et al., Citation1964). This sandstone unit is correlated to the Ban-nosawa Formation and the sandstone unit of the Kinkomanai Formation, which also yield abundant glauconite (Sagayama, Citation2000; ). The hard shale unit (100–200 m in thickness) is characterized by alternating beds of thin dark gray hard shale, and thin gray siltstone and intercalated andesitic tuff-breccia (Sato et al., Citation1964). The hard shale becomes whitish upon weathering and splits into small angular pieces (Sato et al., Citation1964). This unit is correlated to the Mourai Formation (hard shale; Tsushima et al., Citation1956). The Rumoi Formation, conformably overlies the hard shale unit of the Mashike Formation, which is subdivided into the sandstone unit, the Nobusha volcanic unit, and the diatomaceous mudstone unit (Sato et al., Citation1964). The sandstone unit (200–350 m in thickness) is composed of gray or blue gray massive fine sandstone and locally of massive tuffaceous mudstone intercalated with thin layers of hard shale (Sato et al., Citation1964). The Nobusha volcanic unit and the diatomaceous mudstone unit (dark gray massive soft diatomaceous mudstone and tuffaceous siltstone) are not distributed in the study area ().
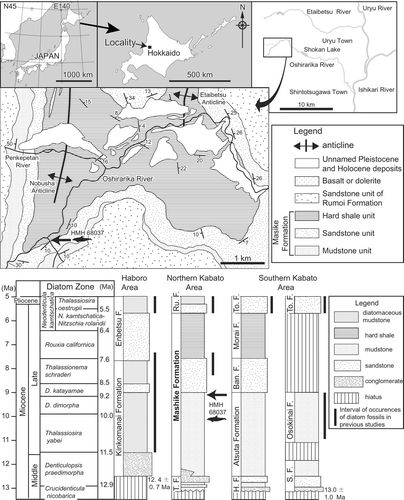
According to Horikawa and Fujita (Citation1972), the holotype was derived from an outcrop near the top of the mudstone unit of the Mashike Formation. The geological structure is simple along the small tributary valley based on the geological map (Sato et al., Citation1964; ). The facies at the type locality (dark gray massive soft sandy mudstone) clearly corresponds to the mudstone unit of the Mashike Formation, and is distinguishable from not only the hard shale unit of the Mashike Formation but also the diatomaceous mudstone unit of the Rumoi Formation. It is difficult to distinguish between the molluscan assemblages of the mudstone unit and other units of the Mashike or Rumoi formations. However, the mudstone unit of the Mashike Formation yields some pre-Pliocene Mollusca such as Portlandia sp. (hayasakai type), Portlandia sp. (watasei type), and Yoldia sagittaria (Kobayashi et al., Citation1957; Sato et al., Citation1964; Amano, Citation1983). These molluscan fossils are not recorded from the Rumoi Formation. These lines of evidence eliminate the possibility that the hard shale unit of the Mashike Formation or the Rumoi Formation are the strata from which the type specimen is derived.
To more rigorously constrain the age of the specimen, we attempted to obtain diatom fossils from the matrix of the holotype and calcareous concretions and mudstone near the type horizon. Unfortunately, none of the samples yielded diatom fossils. According to Watanabe and Yoshida (Citation2000), the upper limit of the sandstone unit and the lower part of the hard shale unit of the Mashike Formation are correlated to the Thalassionema schraderi zone (7.6–8.5 Ma; Neogene North Pacific diatom biohorizons; Yanagisawa and Akiba, Citation1998) and Rouxia californica zone (6.4–7.6 Ma), respectively (). Therefore, the minimum age of Eodelphis is at least 7.6 Ma.
The mudstone unit of the Mashike Formation is correlated to neighboring Neogene marine units based on lithology (e.g., Tsushima et al., Citation1956; Sato et al., Citation1964; Sagayama, Citation2000; ). These Neogene marine deposits are Atsuta (gray or dark gray massive mudstone; Tsushima et al., Citation1956), Osokinai (massive soft siltstone to very fine sandstone; Kakimi and Uemura, Citation1958), and the main part of the Subetsu formations (dark gray mudstone and sandy mudstone; Kakimi and Uemura, Citation1958) of the southern Kabato area. The fission track age of the lower part of the Subetsu Formation is 13.0 ± 1.0 Ma (Tsuji et al., Citation1992). The Osokinai Formation (Sagayama, Citation1989) is correlated to the Thalassiosira yabei zone (10.0–11.5 Ma), Denticulopsis dimorpha zone (9.2–10.0 Ma), and D. katayamae zone (8.5–9.2 Ma). These ages of correlated Neogene marine deposits suggest that the potential age of Eodelphis is between 8.5 and 13.0 Ma. However, the maximum age of Eodelphis may be relatively younger (ca. 9 Ma) within this range, because the holotype came from near the upper limit of the mudstone unit of the Mashike Formation.
DESCRIPTION
Skull
Photographs of the holotype of the skull without drawing suture lines and indices of anatomical characters are given in Supplementary Data 2 (Figs. S1, S2, and S3).
Premaxilla
The rostral parts of the premaxillae anterior to the anterior tips of the prenarial triangle and the distal tip of the nasal processes are missing due to weathering (). The inflection of premaxilla just anterior to, or in line with, the anterior edge of the supraorbital processes of the frontal is absent. The anteromedial, posteromedial, and posterolateral sulci are shallow. The prenarial triangle, which is demarcated by anteromedial and posteromedial sulci, is narrow. One premaxillary foramen exists in both right and left premaxillae and is located medially. Both premaxillary foramina are small and subequal in diameter. The porcelanous part of the premaxilla steeply inclines medially (). The lateral margin of the right premaxilla is nearly straight anteroposteriorly, whereas the left premaxilla arches laterally posterior to the posterolateral sulcus. This part of the left premaxilla is elevated dorsolaterally and prominently overhangs the left maxilla compared with the right premaxilla (, ). The overhanging part extends posteriorly to the level of the midpoint of the external bony nares. The premaxillary eminence is absent. The greatest width of premaxillae is at the level of the postorbital processes; the ratio to width of the maxillae at the level of the postorbital processes is approximately 0.50. The nasal process of the left premaxilla is relatively wide transversely towards the right at level of the middle of the external bony nares: the relative width of the right premaxilla below 150% of the left premaxilla at that point. The external bony nares are U-shaped. Both right and left premaxillae are thick dorsoventrally posterior to the posterolateral sulcus, these parts of the premaxillae steeply incline posteriorly from the rostral part in lateral view (). Consequently, the premaxillary sac fossa is deep. The surface of the premaxillary sac fossa is smooth.
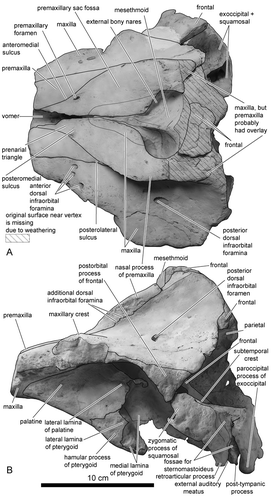
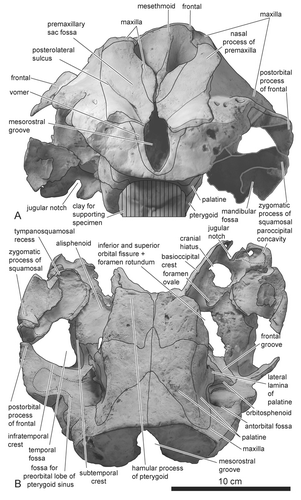
Maxilla
The maxillary crest is convex dorsally (, ). Anterior dorsal infraorbital foramina are preserved: two in the right maxilla and three in the left maxilla. Besides anterior and posterior dorsal infraorbital foramina, additional dorsal infraorbital foramina (at least five in both right and left sides) exist at the suture between the maxilla and the premaxilla (). Both maxillae are exposed widely anteromedial to the edge of the external bony nares and lateral to the posterior part of the mesorostral groove (). There is no posterior dorsal infraorbital foramen on the maxilla near the posterior extremity of the premaxilla. In ventral view, the narrow and little-developed anterior sinus fossa exists anterior to the antorbital fossa (, ). The infraorbital plate of maxilla is absent. The dorsolateral edge of the ventral internal opening of the infraorbital foramen is formed by the maxilla.
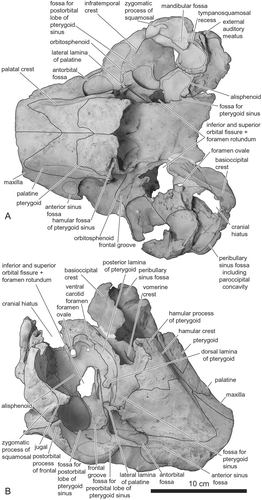
Jugal
The temporal border of the jugal, which is the only preserved part of this bone, is slightly thickened compared with similar-sized delphinids ().
Vomer and Mesethmoid
As in other delphinids, the mesethmoid is wide and well developed dorsally, with its axis skewed towards the left side (, , ). The lateral edges of the mesorostral groove anterior to the level of the premaxillary foramen diverge anteriorly (). Posterior to this level, the joined maxillae converge and cover the mesorostral groove for 13 mm of its length. The vomer does not appear on the preserved portion of the palate in ventral view.
Frontal
The cruciform sculpture on the vertex of the frontals indicates that originally two nasals were present on the vertex in dorsal view (). The vertex of the frontals, as well as the mesethmoid, skews towards the left side (). The postorbital process of the frontal is an isosceles triangle and the apex of the process is directed ventrally in lateral view (). The anterodorsal wall of the braincase is formed by frontals (not indicated in figure).
The fossa for the preorbital lobe of the pterygoid sinus is located anterior to the infraorbital crest and extends dorsally into the orbit (, , ). Dorsal development of the fossa for the preorbital lobe of the pterygoid sinus toward the frontomaxillary suture is absent. The fossa for the postorbital lobe of the pterygoid sinus is well developed dorsomedially just posterior to the orbitosphenoid, in ventral and ventrolateral views (, ).
Palatine
The palatine is relatively long. It probably extended anterior to the level of the antorbital notch. Accordingly, anterior level of the pterygoid sinus fossa would also extend beyond the level of the antorbital notch. The ventral edge of the palatine is low-angled to the horizontal plane when viewed laterally (). The suture line between the palatine and the maxilla on the palate extends anterolaterally (). On the other hand, the suture between the palatine and the pterygoid is transversely straight for much of the width of the palatine, although it extends anterolaterally from the midline. The ventral surface of the palatine is not covered by the pterygoid. On the palate, the palatine is wide and medial to the palatal crest is moderately concave (). The lateral surface of the palatine dorsal to the lateral lamina is concave. The palatine forms the medial border of the antorbital fossa. The lateral lamina of the palatine does not fuse to the maxilla. The lateral lamina of the palatine extends posteriorly beyond the level of the frontal groove. The distal tip of the lamina was probably originally longer (at least 5 mm) than indicated by its present condition.
Pterygoid
In lateral view (), the pterygoid shows the typical form of delphinids: the hamular crest is moderately developed and the medial lamina of pterygoid is widely exposed at the level of the hamular process. Contrary to the original description of Horikawa (Citation1977), the left and the right pterygoids are not separated by posteriorly extended palatines: the medial margins of both pterygoids nearly contact each other (, ). The pterygoid is excavated anterior to the choanae by the hamular fossa for the pterygoid sinus (). Fossae for the pterygoid sinus are developed at the dorsal lamina of the pterygoid ().
Parietal
The parietal is moderately bowed laterally and the posterior part of it is not preserved (). The parietal is not fused to any bones.
Exoccipital
The hypoglossal foramen opens at the dorsal-most point, and slightly anterior to the apex, of the jugular notch. The paroccipital concavity for the posterolateral sinus fossa is shallow (). In front of the exoccipital (, ), the cranial hiatus is widely open. The foramen is not infilled by exostosis of any bones.
Orbitosphenoid
The frontal groove on the orbitosphenoid runs anterolaterally from the confluent opening for the inferior and superior orbital fissures and the foramen rotundum ().
Alisphenoid
The fossa for the pterygoid sinus is well developed on the ventrolateral surface of the alisphenoid. This fossa extends anteriorly until just posterior to the orbital fissure, lateral to the sutures with the parietal and the squamosal, and posterior to the anterior margin of the cranial hiatus (the confluent opening for the posterior lacerate foramen, jugular foramen, and the internal acoustic meatus; Mead and Fordyce, Citation2009:19) (). The ventromedial part of the alisphenoid is flat and covered by the well-developed posterior lamina of the pterygoid (). Consequently, the fossa for the basisphenoidal sinus on the alisphenoid is absent. The alisphenoid-squamosal suture lies medial to the anterior edge of the floor of the squamosal fossa, and the foramen ovale is located in the posterior part of the alisphenoid.
Squamosal
The zygomatic process of the squamosal is relatively longer than in extant genera of Delphinidae (): the length of the zygomatic process of the squamosal is 28.4% of the greatest width of the maxilla at the postorbital process. The tip of the process contacts the postorbital process of the frontal. In lateral view, part of the dorsal surface of the zygomatic process and the subtemporal crest are invisible (). The ventral edge of the zygomatic process of squamosal is concave. The retroarticular process of the squamosal is rectangular and its length is moderate. The external acoustic meatus is narrow. The two fossae for the sternomastoideus are shallow. The ventral edge of the retroarticular process is at the same level as that of the posttympanic process.
The subtemporal crest is weak in anteroventral view (). The tympanosquamosal recess is well developed and bifurcated on the ventral surface the squamosal (, ). It forms a large fossa bordering the entire medial edge of the glenoid fossa (). In ventral view, the zygomatic process of the squamosal projects anterolaterally ().
Basioccipital
The basioccipital crest in the holotype is incomplete, and the remaining part with the hyoids are preserved in the counter part of the rock. Accordingly, the width across both basioccipital crests is 110 mm and the depth is 33 mm.
Ear Bones
Periotic
The apex of the anterior process of the periotic curves anteroventrally (). The process shows a rectangular profile anterior to the cochlear portion in both medial and lateral views. The anterior process of the periotic only contacts the outer lip of the tympanic bulla via the accessory ossicle. The shallow anterior incisure for the origin of the tensor tympani lies between the anterior process of the periotic and the cochlear portion. There is no fossa dorsolateral to the dorsal crest at the base of the anterior process. The posterior process of the periotic bends strongly ventrolaterally (–) and the tip of the posterior bullar facet is pointed.
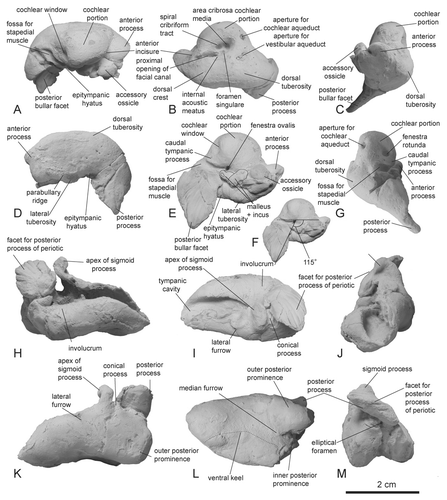
In dorsal view, the cochlear portion is semicircular and the internal acoustic meatus is an inverted pyriform opening (). The fundus of the internal acoustic meatus is funnel-like. The lateral wall of the internal acoustic meatus is low. The aperture for the cochlear aqueduct is smaller than the aperture for the vestibular aqueduct. The lateral margin of the aperture for the vestibular aqueduct is located more lateral than the tractus spiralis foraminosus. The dorsal tuberosity is moderately developed posterolateral to the aperture for the vestibular aqueduct (, ). In cross-section at midlength, the anterior process is ovoid ().
In lateral view, the ventral margin of the anterior process is concave. The peribullary ridge is long and faintly convex laterally. The articular rim is absent. There is not articular surface with squamosal on the anterior process of the periotic (). The dorsal margin of the periotic is straight. A low crest extends to the dorsolateral edge of the posterior process. The posterior process of the periotic was not exposed on the external surface of the skull.
In ventral view, the anteroposterior length of the anterior process of the periotic anterior to the cochlear portion is less than 40% the length of the cochlear portion (). The lateral tuberosity is continuous with the anterior process, there being no anteroexternal sulcus. The accessory ossicle is articulated with the fovea epitubaria. The caudal tympanic process of the periotic is low and its ventral and posterior edges draws smooth curve (, ). The stylomastoid fossa is absent. The posterior bullar facet is long: the greatest length of the posterior process of the periotic as a ratio of the length of the cochlear portion is 93.5%. The ventral surface of the posterior bullar facet bends ventrally (). There are obvious six ridges and seven grooves on the posterior bullar facet. The articular surface for the squamosal or occipital bones on the posterior bullar facet is absent. The angle between the posterior process of the periotic and the long axis of the body of the periotic is approximately 115° (Fig. 5F). In posterior view, the fenestra rotunda is oval and opens at nearly the same level as the fossa for the stapedial muscle (). The aperture for the cochlear aqueduct is located at the dorsomedial edge of the posterior surface of the cochlear potion ().
Tympanic Bulla
The dorsal margin of the involucrum lacks a transverse groove and is concave (). Seven distinct ridges and six grooves occur on the posterior margin of the posterior process, extending parallel with the posterior bullar facet on the posterior process of the periotic (, ). The posterior process of the tympanic bulla does not have the articular surface for the squamosal.
The anterior edge of the tympanic cavity is smoothly convex without an anterior spine (). There is no transverse ridge across the tympanic cavity. The tympanic bulla is transversely narrow (56% the length of the tympanic bulla). In dorsal view, the apex of the sigmoid process of the tympanic bulla is directed laterally (). The dorsal edge of the sigmoid process expands to articulate with the lateral tuberosity of the periotic. The apex of the posterior process is directed dorsolaterally (, , ). The greatest length of the posterior process is much shorter than the anteroposterior length of the tympanic bulla along its long axis.
In lateral view, the ventral margin of the tympanic bulla is concave ventrally (). The anterolateral convexity of the tympanic bulla with the anterolateral notch is absent. The conical process is short, and the lateral furrow of the tympanic bulla is shallow (). The edge of the posterior process of the tympanic bulla is slightly spiny.
The ventral keel of the tympanic bulla extends anteriorly beyond the level of the lateral furrow (). The posterior end of the ventral keel is not directed medially. The median furrow on the posterior side of the tympanic bulla is not divided by a transverse ridge. The posterior edge of the inner posterior prominence is distinctly anterior to the posterior edge of the outer posterior prominence. The patent elliptical foramen can be seen in posterior view ().
Malleus, Incus, and Stapes
The detailed morphology of malleus and the incus are not clear, because these bones cannot be separated from the periotic (). The stapes is lost.
Mandible and Teeth
Mandible
The coronoid processes of both mandibles are preserved. Mandibles are dorsoventrally deep (90 mm high in the right mandible) relative to the greatest width of the maxillae at level of the postorbital processes in Delphinidae, although the coronoid process is low (). The mandibular notch is located between the mandibular condyle and the coronoid process is large and semiovate.
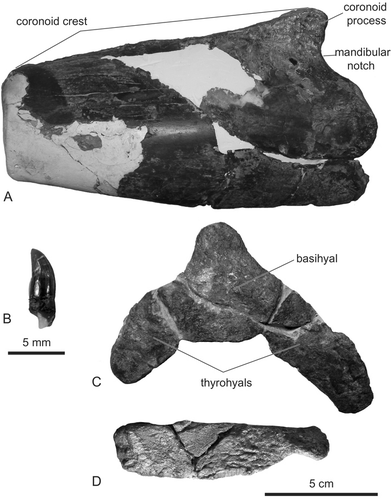
Teeth
Several isolated teeth are preserved around the left mandibular fossa and the fossa for the left pterygoid sinus. Those conical teeth are small but stout: crown height is 4.2 mm and crown width is 2.4 mm (). Tooth enamel is smooth. Horikawa (Citation1977) referred to a tooth whose crown and root heights are 15 mm, respectively, but we could not find this tooth.
Hyoids
The basihyal is not fused to the left and right thyrohyals (Fig. 6C). This feature and the large cranial hiatus may indicate that the holotype individual was physically immature. Both thyrohyals make an acute angle towards each other. The left stylohyal is dorsoventrally deep (24 mm) relative to its length (96 mm; Fig. 6D).
PHYLOGENETIC AND PALEOBIOGEOGRAPHIC ANALYSES
Materials and Methods
Phylogenetic Analysis
The main purpose of the cladistic analysis is to test the revision of the taxonomy of Eodelphis kabatensis (i.e., the phylogenetic position of the taxon). Murakami et al. (Citation2012a, Citation2012b) indicated that Eodelphis is more basal than the Stenella complex in their phylogenetic analyses. However, those analyses included only four species of the Stenella complex. For this reason, we add all species of the complex except Tursiops australis and Sousa teuszii as OTUs (operational taxonomic units) to the matrix of Murakami et al. (Citation2012b). Thus, we considered 30 extinct and extant delphinid species as OTUs in the present cladistic analysis (total 82 ingroup taxa, and the stem cetaceans Georgiacetus and Zygorhiza as outgroup taxa; see Supplementary Data 1). Character data were entered into a character-taxon matrix using MacClade 4.0 (Maddison and Maddison, Citation2000). One of 282 characters is redefined from Murakami et al. (Citation2012b; see Supplementary Data 3, 4, 5).
Previous comprehensive morphological cladistic analyses yielded well-resolved relationships within Delphinidae (Murakami et al., Citation2012a, Citation2012b). In those analyses, Delphinidae were closely related to Phocoenidae, contrary to most comprehensive molecular analyses (e.g., McGowen et al., Citation2009; Steeman et al., Citation2009; Geisler et al., Citation2011). Because of this, we employed morphological cladistic analysis but also this analysis with a constraint tree based on comprehensive molecular phylogenetic analysis. We used the consensus tree of McGowen et al. (Citation2009) as the constrained tree. Cladistic analyses were performed with PAUP 4.0 b10 (Swofford, Citation1998). All characters were treated as unweighted and unordered. The heuristic search and the random stepwise addition with tree bisection-reconnection (TBR) branch swapping algorithm were employed with 100 replicates and 30 trees held at each step during stepwise addition. The decay index (Bremer, Citation1988) for each branch was calculated using MacClade 4.0 (Maddison and Maddison, Citation2000) and PAUP 4.0 b10 (Swofford, Citation1998).
Paleobiogeographic Analysis
We used the parsimony analysis as implemented in Mesquite 2.74 (Maddison and Maddison, Citation2010) to reconstruct ancestral distributions of clades of Delphinidae. Markov k-state 1 parameter model, the single parameter constrained to be equal for all state changes, was used in the analysis. The backbone of phylogenetic trees is the strict consensus tree of the present cladistic analysis and the Adams consensus tree of the constraint cladistic analysis. It is too difficult to subdivide the distribution of modern cetaceans into each area, because many modern cetaceans have extremely wide distribution beyond single ocean basins such as Pacific, Atlantic, Indian, and Arctic. If these are strictly divided into each region, the character status of modern cetaceans becomes problematic. Furthermore, ancestral states become too multistate to reconstruct them. Consequently, we simplified the distribution area of cetaceans. The distribution ranges of extant taxa follow Jefferson et al. (Citation2008): non-Pacific Ocean is coded as 0; Pacific and other oceans, 1; only Pacific Ocean, 2.
Results and Discussion
Morphological Cladistic Analysis
The phylogenetic analysis found 1777 most parsimonious trees with a length of 1841 steps. The general topology of the consensus tree is nearly identical to that of Murakami et al. (Citation2012a, Citation2012b; see their discussion about interfamilial relationship within Delphinoidea). Eodelphis kabatensis forms a clade with Orcinus orca (Linnaeus, Citation1758) and Hemisyntrachelus cortesii Fischer, Citation1829 (). This clade is sister to Orcaella brevirostris + Globicephalinae. Then, that clade forms a clade with all other delphinids. In the Stenella complex, Sousa chinensis forms a clade with Sotalia fluviatilis, and Tursiops aduncus is located outside of Delphininae, unlike molecular phylogenetic analyses (e.g., LeDuc et al., Citation1999; McGowen et al., Citation2009; McGowen, Citation2011). S. rayi, T. osennae, and Etruridelphis giulii are also located outside of Delphininae. Thus, the present morphological cladistic analysis indicates that Eodelphis is more basal than not only any species of Stenella but also the Stenella complex. None of the latter genera (Delphinus, Tursiops, and Stenella) are monophyletic in our phylogenetic hypotheses.
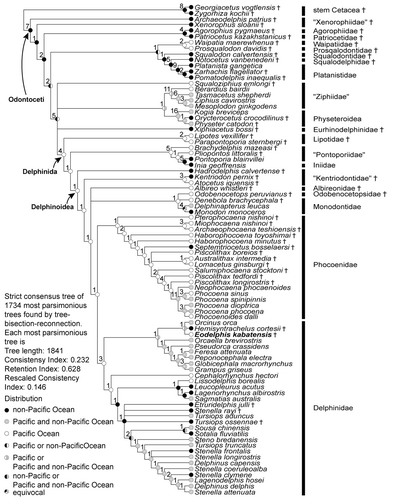
The present cladistic analyses support a relatively basal position for Eodelphis kabatensis among Delphinidae. Eodelphis falls within Delphinidae by sharing the following apomorphies of that clade: presence of a keel affecting the ventral surfaces of the hamular processes [character 139-1; Muizon, Citation1988a]—hereafter, character number and its apomorphic state in the present analysis is indicated by text in square brackets; posterior bullar facet of the periotic with many deep grooves and distinct ridges [192-0; Bianucci, Citation2005]; spiny or irregular edges of the posterior process of the tympanic bulla [206-0; Muizon, Citation1991]; posterior edge of the inner posterior prominence of the involucrum located distinctly anterior to the posterior edge of the outer posterior prominence [209-1; Muizon, Citation1987]; and ventral keel of tympanic bulla terminating approximately at level of the lateral furrow or midpoint of the tympanic bulla [213-1; Geisler and Sanders, Citation2003]. Nine other delphinid synapomorphies cannot be scored in Eodelphis: dorsolateral edge of internal opening of infraorbital foramen formed by maxilla [43-0; Geisler and Sanders, Citation2003]; ventromedial edge of internal opening of infraorbital foramen formed by maxilla and palatine and/or pterygoid [44-1; Geisler and Sanders, Citation2003]; absence of fossa for inferior vestibule on maxilla lateral to external nares or lateral to premaxilla [68-0; Muizon, Citation1988b]; nasals same height as frontals [86-1; Muizon, Citation1988b]; ‘W’- or reversed’U’-shaped nasal-frontal suture line [93-2; Murakami et al., Citation2012a]; nuchal crest rectangular or weakly convex anteriorly or posteriorly in posterodorsal view [116-0; Murakami et al., Citation2012a]; fused atlas and axis [220-1; Murakami et al., Citation2012a]; absence of posterior nasal sacs [251-0; Heyning, Citation1989]; and undivided vestibular sac [257-0; Messenger and McGuire, Citation1998]. Contrary to other delphinids, Eodelphis has a shallow lateral furrow of the tympanic bulla [200-0; Murakami et al., Citation2012a].
Eodelphis does not have synapomorphies of advanced delphinids (‘Delphininae’ + ‘Steninae’ + ‘Lissodelphinae’): thin parabullary ridge of the periotic [171-0; Murakami et al., Citation2012a], and a short posterior process of the periotic [193-1; Murakami et al., Citation2012a]. Two other synapomorphies of the clade cannot be scored: premaxillae narrowly separated by mesorostral groove in the rostrum [13-2; Murakami et al., Citation2012a]; and anterodorsally directed acromion of scapula [240-0; Murakami et al., Citation2012a].
Eodelphis shares three apomorphies with Orcinus and Hemisyntrachelus: weakly asymmetrical premaxillae at level of the midpoint of the external bony nares [73-1; Murakami et al., Citation2012a]; squared off ventral tip of the retroarticular process in lateral view [114-1; Geisler and Sanders, Citation2003]; and angled basihyal and thyrohyals [216-1; Murakami et al., Citation2012a]. One other synapomorphy of this clade cannot be scored in Eodelphis: arched roof of neural canal of atlas [218-0; Murakami et al., Citation2012a]. These three genera are closely related in the present and previous cladistic analysis (Murakami et al., Citation2012a). However, 24 ear bone characters of H. cortesii have not been coded in the analyses, because there are few ear bones associated with the skulls of H. cortesii and they have not been described in detail. If these ear bone characters are coded, the relationship may be considerably altered.
Considering its relatively archaic morphology, it could be argued that Eodelphis represents a non-delphinid delphinoid. Constraining Eodelphis as a stem Monodontidae, requires 12 more steps than the optimal tree. Similarly, constraining Eodelphis as sister to Monodontidae + Phocoenidae requires 25 additional steps to the optimal consensus tree. Thus, we find strong support for inclusion of Eodelphis within Delphinidae.
Molecular Constraint Cladistic Analysis
Under the molecular constraint tree analysis (Fig. 8), several relationships within Delphinoidea are changed (positions of subclades of Kentriodontidae, and Pterophocaena nishinoi Murakami et al., Citation2012a). A lophocetine ‘kentriodontid’ Hadrodelphis + crown Delphinoidea is supported by four synapomorphies: the premaxilla is not compressed mediolaterally at the anterior rostrum [3-0; Murakami et al., Citation2012a]; the anterior sinus fossa is positioned between the anterior extremity of the pterygoid sinus fossa and the posterior extremity of the upper tooth row [17-1; Murakami et al., Citation2012a]; presence of three or more anterior dorsal infraorbital foramina [49-2; Murakami et al., Citation2012a]; and nasals are lower than frontals [86-0; Muizon, Citation1988a].
The clade ‘Phocoenidae’ + (Pterophocaena + (Albireonidae + (Odobenocetopsidae + Monodontidae))) is supported by four synapomorphies: presence of the fossa for the inferior vestibule on the maxilla lateral to the external nares or lateral to the premaxilla [68-1; Muizon, Citation1988a]; presence of the frontal boss [95-1; Muizon, Citation1984]; presence of the deep dorsal condyloid fossa [118-2; Geisler and Sanders, Citation2003]; and absence of the elliptical foramen of the tympanic bulla [204-1; Geisler and Sanders, Citation2003]. Pterophocaena is sister to Albireonidae + (Odobenocetopsidae + Monodontidae), although two recent cladistic analyses (Murakami et al., Citation2012a, Citation2012b) and the present morphological cladistic analysis indicate that Pterophocaena is the most archaic taxon within Phocoenidae. However, this clade is supported by only two synapomorphies: the ratio of the greatest width of the premaxillae to the greatest width of the maxillae at the level of the postorbital processes ≥0.50 [74-0; Murakami et al., Citation2012a]; and the roof of the neural canal of the atlas is straight [218-2; Murakami et al., Citation2012a]. Furthermore, if Pterophocaena is forced to the basal-most position of Phocoenidae, the optimal tree is only one step longer. Consequently, we consider this hypothesis to be weakly supported.
The clade Albireonidae + (Odobenocetopsidae + Monodontidae) is supported by six synapomorphies: absence of the anterior sinus fossa [17-0; Muizon, Citation1988a]; in dorsal view, the parietals are visible only as triangular areas, dorsolateral to the supraoccipital, with the non-overlapping supraoccipital separated from and contacting the parietals along the irregular suture [104-3; Geisler and Sanders, Citation2003]; shallow sternomastoid muscle fossa on the zygomatic process of the squamosal [110-1; Geisler and Sanders, Citation2003]; the depth of the pterygoid sinus fossa in the basicranium is deep, excavated dorsally to the level of the foramen ovale [130-1; Fordyce, Citation1994]; absence of the preorbital lobe of the pterygoid sinus fossa [133-0; Fraser and Purves, Citation1960]; and the angle between the posterior bullar facet and the long axis of the cochlear portion is >135° [190-0; Murakami et al., Citation2012a].
Phocoenidae excluding Pterophocaena is supported by five synapomorphies: two anterior dorsal infraorbital foramina [49-1; Murakami et al., Citation2012a]; posterior part of posterolateral sulcus covered by premaxilla in dorsal view [55-2]; presence of the low premaxillary eminence [75-1; Lambert, Citation2008]; presence of the nasal protuberance [87-1; Muizon, 1988]; and medially positioned premaxillary foramina [280-0; Murakami et al., Citation2012b].
Delphinidae is supported by 10 synapomorphies, nine of which are the same as in the present morphological cladistic analysis: dorsolateral edge of internal opening of infraorbital foramen formed by maxilla [43-0]; ventromedial edge of internal opening of infraorbital foramen formed by maxilla and palatine and/or pterygoid [44-1]; nasals at same height as the frontals [86-1]; W- or reversed U-shaped nasofrontal suture [93-2]; nuchal crest rectangular or weakly convex anteriorly or posteriorly in posterodorsal view [116-1]; posterior bullar facet of the periotic with many deep grooves and distinct ridges [192-0]; spiny or irregular edges of the posterior process of the tympanic bulla [206-0]; fused atlas and axis [220-1]; absence of posterior nasal sacs [251-0]; and undivided vestibular sac [257-0]. The 10th (additional) synapomorphy is convex lateral margin of the rostrum anterior to the maxillary flange [7-2; Bianucci, Citation2005]. Among these synapomorphies, Eodelphis possesses only two [192-0, 206-0]. If Eodelphis is forced to the basal-most position of the clade consisting of Pterophocaena nishinoi + (Albireonidae + (Odobenocetopsidae +Monodontidae)), the optimal tree is nine steps longer.
Eodelphis is united in a clade with Orcinus + Hemisyntrachelus. This clade is the earliest-diverging lineage within Delphinidae. Thus, Eodelphis is included in a more basal clade than the Stenella complex, as hypothesized in the present morphological cladistic analysis. The latter clade, which contains three extinct species (Stenella rayi, Tursiops osennae, and Etruridelphis giulii), is included in Delphininae. However, the fossils S. rayi, T. osennae, and E. giulii are posited outside the clade including living species of Stenella, Tursiops, and Delphinus. Synapomorphies of Eodelphis + (Orcinus and Hemisyntrachelus) are weakly asymmetrical premaxillae at the level of the midpoint of the external bony nares [73-1]; a rectangular ventral tip of the retroarticular process in lateral view [114-1]; the lateral lamina of the palatine forms the bony bridge over the orbit [123-1]; and angled the basihyal and thyrohyals [216-1]. Orcinus and Hemisyntrachelus share two synapomorphies to the exclusion of Eodelphis: the posterior process of the periotic is in the same plane as the body of the periotic in lateral view [189-1]; and the mesorostral groove diverging anteriorly posterior to the antorbital notch is absent [279-0].
Comparisons
Here, we compare Eodelphis kabatensis with only Orcinus and Hemisyntrachelus spp., which form a clade in the present morphological cladistic analysis, because it is clear that Eodelphis is more archaic than the Stenella complex. Eodelphis is similar to Orcinus and Hemisyntrachelus spp. in having a wide nasal process of the left premaxilla relative to the right premaxilla at the level of the middle of the external bony nares () and a dorsoventrally deep mandible. The former character varies between individuals of H. cortesii. Eodelphis is similar to H. oligodon in possessing a right premaxilla with a nearly straight lateral margin between the antorbital notch and the level of the middle of the external bony nares. Eodelphis is similar to Orcinus in having a long lateral lamina of the palatine beyond the level of the anterior margin of the frontal groove, although this character also varies between individuals of Orcinus.
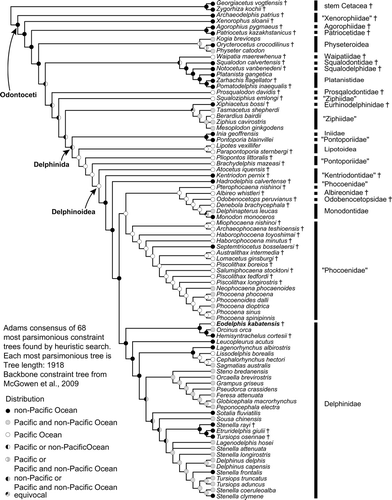
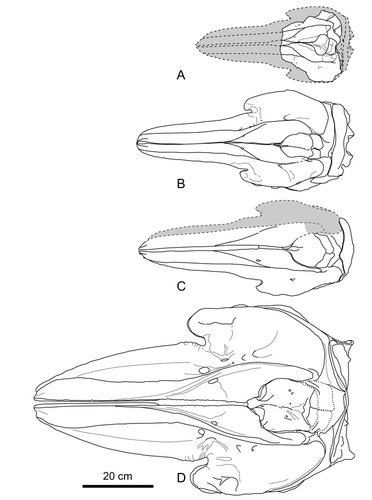
To compare with Orcinus and Hemisyntrachelus spp., the skull of Eodelphis is much smaller: the condylobasal length and greatest width of the maxillae at the level of the postorbital processes are estimated or measured as follows: about 450 mm and 218 mm, respectively, in Eodelphis; 600–730 mm and 272–332 mm, respectively, in Hemisyntrachelus cortesii (N = 7); 625 mm and about 280 mm, respectively, in H. oligodon; and 1040 mm and 561 mm, respectively in Orcinus (NMNS-M 4510). Eodelphis is clearly distinct from those taxa by having a medially positioned premaxillary foramen; the partially transversely directed suture between palatine and pterygoid on the palate; the left premaxilla prominently overhanging the left maxilla, steeply inclined bones around the external bony nares (premaxillae and mesethmoid); and a prominently long and ventrolaterally extended posterior process of the periotic with an attenuated posterior bullar facet. These differences, combined with the results of the cladistic analyses, corroborate the validity of the species Stenella kabatensis, removal of this species from the genus Stenella, and establishment of a new genus Eodelphis for this species.
Stenella Complex
Our comprehensive cladistic analyses indicate that Eodelphis is positioned in an earlier-diverging clade than the Stenella complex. Consequently, this inconsistency is resolved. Two extinct species, Stenella rayi and Tursiops osennae, which have been referred to genera in that complex are located outside this clade in both present analyses. These species may require reassignment from Stenella and Tursiops to new genera of delphinids, respectively. The present morphological cladistic analysis indicates that Delphinus, Tursiops, and Stenella are paraphyletic (). Paraphyletic status of Stenella agrees with all recent molecular phylogenetic analyses (e.g., McGowen et al., Citation2009; Xiong, 2009; McGowen, Citation2011). On the other hand, it is different from all those studies except Bayesian analysis of nuclear DNA only (McGowen, Citation2011) by hypothesizing paraphyly for both Delphinus and Tursiops. Several studies suggest monophyly of both Delphinus and Tursiops (e.g., McGowen et al., Citation2009; McGowen, Citation2011) and most molecular phylogenetic analyses indicate monophyly of Delphinus (e.g., LeDuc et al., Citation1999; Kingston et al., Citation2009). Sousa and T. aduncus are not included in Delphininae, because they lack synapomorphies of Delphininae in the present morphological cladistic analysis: Sousa lacks two and T. aduncus lacks all synapomorphies (i.e., the anterior sinus fossa between the posterior extremity of the upper tooth row and the midpoint of rostrum [17-2; Murakami et al., Citation2012a]; the dorsoventrally long J-shaped postorbital process [47-3; Murakami et al., Citation2012a]; the thin parabullary ridge of the periotic [171-1; Bianucci, Citation2005]; and the posterior end of the ventral keel of the tympanic bulla is protruding and directed medially [214-1; Geisler and Sanders, Citation2003]). In these species, character states of ear bones may be affected by intraspecific variation, because these characters are coded from one individual in each of these species due to limited availability of specimens.
Paleobiogeography
The reconstruction of divergence time and geographic distributions of Delphinida are given in and . Evolutionary scenarios are radically different between the morphological cladistic analysis and the molecular constraint morphological cladistic analysis. In the first scenario (), most of the ancestral nodes including Delphinida, Delphinoidea, crown Delphinoidea, and their respective subclades have reconstructed origins and early evolution in the Pacific Ocean. These results confirmed assumptions from the fossil record: appearance of the North Atlantic crown Delphinoidea since the early Pliocene might indicate a Pacific origin for the three families and a subsequent migration across the progressively restricted Panama Seaway (Barnes, Citation1985; Lambert and Gigase, Citation2007). It is strongly suggested that Phocoenidae originated in the Pacific Ocean and diversified by the Serravallian. At least two independent linages in Phocoenidae invaded non-Pacific oceans. It is suggested that Delphinidae also originated in the Pacific Ocean and diversified by the Serravallian, yet so far, Eodelphis is the only described Miocene delphinid. Delphinidae invaded non-Pacific oceans at least twice (the common ancestor between Eodelphis, Orcinus, and Globicephalinae; and the clade excluding Cephalorhynchus hectori).
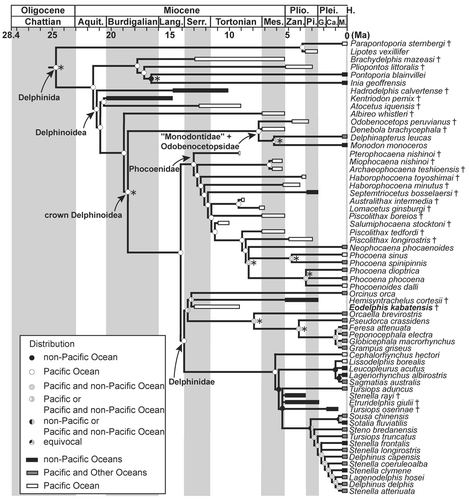
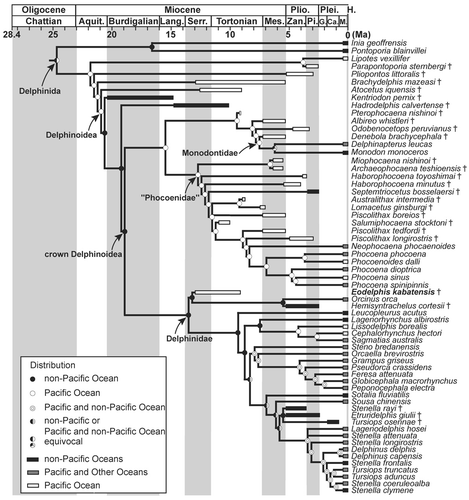
The second (molecular topological constraint) scenario suggests that the origin of crown Delphinoidea occurred outside the Pacific Ocean (). In this scenario, Eodelphis invaded the Pacific Ocean from elsewhere. At least four independent clades within Delphinidae are also hypothesized to have invaded the Pacific Ocean from elsewhere. However, no reliable Miocene delphinids have been discovered from beyond the Pacific Basin (e.g., Bianucci and Landini, Citation2002). A large clade including Phocoenidae, Monodontidae, Odobenocetopsidae, and Albireonidae originated and diversified in the Pacific Ocean. Their early diversification is estimated to have occurred in the Serravallian and Tortonian, although the origin of this clade lies in the Langhian based on molecular studies (e.g., McGowen et al., Citation2009). Thus, the two evolutionary scenarios differ markedly. To determine the origin of Delphinidae, we have to include more fossil delphinid taxa in phylogenetic analyses. In addition, we also have to add more kentriodontids to analyses, because they appear to be the sister group of derived Delphinoidea (including Albireonidae).
MIOCENE DELPHINIDS
As noted in Introduction, most Miocene delphinids are nomina dubia (e.g., Steno cudmorei Chapman, Citation1917) or established on fragmentary fossils (e.g., Fulgosi and Pilleri, Citation1985; Pilleri, Citation1988). Most Miocene delphinid species (e.g., Sinanodelphis izumidaensis Makiyama, Citation1936), which were classified as Delphinidae, have been reassigned to Kentriodontidae, Delphinoidea incertae sedis, or Odontoceti incertae sedis by Barnes (Citation1978) and Fordyce and Muizon (Citation2001). Sinanodelphis was derived from the middle Miocene of Nagano, central Japan. The skull of this taxon has been split along the horizontal line from the tip of the rostrum to the occipital condyle, and the facial plane and the basicranium of the specimen remain buried within matrix. The postcranial skeletons have also been incompletely prepared. The preparation of this taxon is prohibited by the local government, because the taxon have been designated a natural monument of Nagano Prefecture. Thus, we cannot judge whether this taxon belongs to Delphinidae or not.
Anacharsis orbus Bogachev, Citation1956, is a small dolphin, consisting of a nearly complete skeleton (1300 mm long) from the Sarmatian (11.6–12.7 Ma) of Russia. Bogachev (Citation1956) described this long snouted dolphin (condylobasal length = 400 mm, rostrum = 310–330 mm long) as a delphinid. Barnes (Citation1978) and Fordyce and Muizon (Citation2001) did not mention this taxon, but several authors listed Anacharsis as a Miocene delphinid (Carroll, Citation1988; McKenna and Bell, Citation1997; Perrin et al., Citation2002; Sepkoski, Citation2002). Thus, Anacharsis is the only Miocene delphinid referred to in recent publications, although Thewissen and Schneider (Citation2009) removed Anacharsis from Delphinidae. Triangular transverse processes of lumbar vertebrae indicate that Anacharsis belongs to Delphinida, and a short humerus relative to the radius and ulna are apparently similar to Phocoenidae and Delphinidae. Nonetheless, the facial skull of this species is heavily damaged and description of the ventral side of the skull is not sufficient to trace air sinus systems. In addition, ear bones are not preserved in the holotype. Hence, none of the 14 synapomorphies of Delphinidae identified in the present morphological phylogenetic analysis can be scored for Anacharsis. Furthermore, the total number of vertebrae (38) is much fewer than in any known species of Delphinidae. Thus, there is not enough evidence to confirm Anacharsis as a delphinid. Instead, we propose that this species is assigned to Delphinida incertae sedis. As a result, Eodelphis kabatensis is the oldest and only valid Miocene delphinid species. Therefore, this species is significant in the origin, early evolution, and paleobiogeography of Delphinidae and provides stratigraphic calibration of molecular divergence estimates. Nevertheless, further discussion of these implications can only be initiated following the determination of a more highly constrained age of the holotype specimen and more information on several delphinoid taxa from the middle to late Miocene.
CONCLUSIONS
We redescribed the late Miocene delphinid, Stenella kabatensis (8.5–13.0 Ma), from Hokkaido, northern Japan, as a new genus Eodelphis. Both a comprehensive morphological cladistic analysis and this analysis under the constraint tree of molecular phylogenetic analyses support a new combination of the species; i.e., Eodelphis is significantly more archaic than the Stenella complex. Those analyses also indicate that the two extinct species Stenella rayi and Tursiops osennae are not included in the Stenella complex. Eodelphis is significant for understanding the origin, early evolution, and paleobiogeography of Delphinidae, as well as calibration of the molecular divergence estimates: Eodelphis kabatensis is the oldest and only valid Miocene delphinid species yet described. However, paleobiogeographic analyses based on the present phylogenetic analyses suggest different biogeographic scenarios: origin and early diversification in the middle Miocene Pacific Ocean or outside the Pacific Ocean. To resolve the origin of Delphinidae, we need to include more fossil delphinids and kentriodontids in phylogenetic analyses.
Murakami et al Supplemental Data
Download Zip (848.7 KB)ACKNOWLEDGMENTS
We are grateful to C. Argot, L. G. Barnes, G. Bianucci, D. J. Bohaska, E. Cioppi, T. A. Deméré, J. P. Dine, R. E. Fordyce, H. Furusawa, J. Galkin, S. J. Godfrey, P. Holroyd, H. Ichishima, D. S. Janiger, T. Kimura, Y. Koda, N. Kohno, O. Lambert, J. G. Mead, M. Mizushima, C. de Muizon, D. Ormezzano, J. J. Ososky, C. W. Potter, N. D. Pyenson, K. A. Randall, G. Raineri, C. Sarti, S. Sorbi, G. T. Takeuchi, Y. Tajima, Y. Tulu, G. B. Vai, E. Westwig, T. K. Yamada, and R. Zorzin for access to the specimens under their care and valuable suggestions; K. Post for sending photographs of Hemisyntrachelus oligodon; and F. Kono and Y. Mino for CT scanning of the holotype and comparative species. We are also thankful to D. E. Hurlbert, B. Watanabe, the Calvert Marine Museum, and the Clarissa and Lincoln Dryden Endowment for Paleontology at the Calvert Marine Museum for their financial support. We also thank the referees (J. H. Geisler and H. Ichishima) and editor (E. M. G. Fitzgerald) for their helpful comments. This work was supported by the Academic Paper Publication Fee Subsidy of Waseda University.
Handling editor: Erich Fitzgerald
LITERATURE CITED
- Aguirre-Fernández, G., L.G. Barnes, F.J. Aranda-Manteca, and J.R. Fernández-Rivera. 2009. Protoglobicephala mexicana, a new genus and species of Pliocene fossil dolphin (Cetacea; Odontoceti; Delphinidae) from the Gulf of California, Mexico. Boletín de la Sociedad Geológica Mexicana 61:245–265.
- Amano, K. 1983. Palaeontological study of the Miocene Togeshita molluscan fauna in the Rumoi district, Hokkaido. Science Reports of the Institute of Geoscience, University of Tsukuba, Series B 4:1–72.
- Archer, F.I. , II, and W.F. Perrin. 1999. Striped dolphin (Stenella coeruleoalba). Mammalian Species 603:1–9.
- Barnes, L.G. 1977. Outline of eastern North Pacific fossil cetacean assemblages. Systematic Zoology 25:321–343.
- Barnes, L.G. 1978. A review of Lophocetus and Liolithax and their relationships to the delphinoid family Kentriodontidae (Cetacea: Odontoceti). Natural History Museum of Los Angeles County Science Bulletin 28:1–35.
- Barnes, L.G. 1985. Evolution, taxonomy and antitropical distributions of the porpoises (Phocoenidae, Mammalia). Marine Mammal Science 1:149–165.
- Bianucci, G. 2005. Arimidelphis sorbinii, a new small killer-like dolphin from the Pliocene of Marecchia River (Central eastern Italy) and a phylogenetic analysis of the Orcininae (Cetacea: Odontoceti). Rivista Italiana di Paleontologia e Stratigrafia 111:329–344.
- Bianucci, G. 2013. Septidelphis morii, n. gen. et sp., from the Pliocene of Italy: new evidence of the explosive radiation of true dolphins (Odontoceti, Delphinidae). Journal of Vertebrate Paleontology 33:722–740.
- Bianucci, G., and W. Landini. 2002. Change in diversity, ecological significance and biogeographical relationships of the Mediterranean Miocene toothed whale fauna. Geobios Mémoire Special 24:19–28.
- Bianucci, G., S.C. Vaiani, and S. Casati. 2009. A new delphinid record (Odontoceti, Cetacea) from the Early Pliocene of Tuscany (central Italy): systematics and biostratigraphic considerations. Neues Jahrbuch für Geologie und Paläontologie Abhandlungen 254:275–292.
- Bogachev, V.V. 1956. Sarmatskii delfin Anacharsis orbus iz okrestnostei g Stavropolya Kavkazskogo. Materialy po Izucheniyu Stavropol'skogo kraya 8:237–254.
- Brandt, J.F. 1874. Ergänzungen zu den fossilen cetaceen Europa's. Memoires de l’Académie Impériale des Sciences de St.-Pétersbourg, VII Série 21:1–54.
- Bremer, K. 1988. The limits of amino acid sequence data in angiosperm phylogenetic reconstruction. Evolution 42:795–803.
- Brisson, M.J. 1762. Le regnum animale in classes IX distributum, sive synopsis methodica sistens generalem animalium distributionem in classes IX, & duarum primarum classium, quadrupedum scilicet & cetaceorum, particularem dibvisionem in ordines, sectiones, genera & species. T. Haak, Paris, 296 pp.
- Caballero, S., J. Jackson, A.A. Mignucci-Giannoni, H. Barrios-Garrido, S. Beltrán-Pedreros, M.G. Montiel, K. Robertson, and C.S. Baker. 2008. Molecular systematics of South American dolphins Sotalia: sister taxa determination and phylogenetic relationships, with insights into a multi-locus phylogeny of the Delphinidae. Molecular Phylogenetics and Evolution 46:252–268.
- Capellini, G. 1883. Di un’orca fossile scoperta a Cetona in Toscana. Memorie della Regia Accademia delle Scienze dell’Instituto di Bologna 4:665–687.
- Carroll, R.L. 1988. Vertebrate Paleontology and Evolution. W. H. Freeman and Company, New York, 698 pp.
- Cassens, I., S. Vicario, V.G. Waddell, H. Balchowsky, D. Van Belle, W. Ding, C. Fan, R.S. Lal Mohan, P.C. Simões-Lopes, R. Bastida, A. Meyer, M.J. Stanhope, and M.C. Milinkovitch. 2000. Independent adaptation to riverine habitats allowed survival of ancient cetacean lineages. Proceedings of the National Academy of Sciences of the United States of America 97:11343–11347.
- Chapman, F. 1917. New or little-known Victorian fossils in the National Museum. Proceedings of the Royal Society of Victoria 30:32–43.
- Charlton-Robb, K., L.-A. Gershwin, R. Thompson, J. Austin, K. Owen, and S. McKechnie. 2011. A new dolphin species, the Burrunan dolphin Tursiops australis sp. nov., endemic to southern Australian coastal waters. PLoS ONE 6:e24047.
- Chen, Z., S. Xu, K. Zhou, and G. Yang. 2011. Whale phylogeny and rapid radiation events revealed using novel retroposed elements and their flanking sequences. BMC Evolutionary Biology 11:314.
- Committee on Taxonomy. 2012. List of marine mammal species and subspecies. Society for Marine Mammalogy. Available at www.marinemammalscience.org. Accessed July 9, 2012.
- Cuvier, G. 1829. Le Règne Animal, Volume 1. Déterville, Paris, France, 584 pp.
- Fischer, J.B. 1829. Synopsis Mammalium: Addenda, Emendanda et Index ad Synopsis. Sumtibus J. G. Cottae, Stuttgart, Germany, 752 pp.
- Flower, W.H. 1867. Description of the skeleton of Inia geoffrensis and of the skull of Pontoporia blainvillei with remarks on the systematic position of these animals in the order Cetacea. Transactions of the Zoological Society of London 6:87–116.
- Fordyce, R.E. 1982. A review of Australian fossil Cetacea. Memoirs of the National Museum of Victoria 43:43–58.
- Fordyce, R.E. 1984. Preliminary report on cetacean bones from Chatham Rise (New Zealand). Geologisches Jahrbuch, Series 4 65:117–120.
- Fordyce, R.E. 1994. Waipatia maerewhenua, new genus and new species (Waipatiidae, new family), an archaic Late Oligocene dolphin (Cetacea: Odontoceti: Platanistoidea) from New Zealand. Proceedings of San Diego Society of Natural History 29:147–176.
- Fordyce, R.E., and C. de Muizon. 2001. Evolutionary history of cetaceans: a review; pp. 169–233 in J.M. Mazin and V. Buffréenil (eds.), Secondary Adaptations of Tetrapods to Life in Water. Verlag Dr. Friedrich Pfeil, Munich, Germany.
- Fordyce, R.E., P.G. Quilty, and J. Daniels. 2002. Australodelphis mirus, a bizarre new toothless ziphiid-like fossil dolphin (Cetacea: Delphinidae) from the Pliocene of Vestfold Hills, East Antarctica. Antarctic Science 14:37–54.
- Fraser, F.C., and P.E. Purves. 1960. Hearing in cetaceans: evolution of the accessory air sacs and the structure and function of the outer and middle ear in recent cetaceans. Bulletin of the British Museum (Natural History) of the Zoology 7:1–140.
- Fulgosi, F.C., and G. Pilleri. 1985. The lower Serravallian cetacean fauna of Visiano (Northern Apennines, Parma, Italy). Investigations on Cetacea 17:55–93.
- Geisler, J.H., and A.E. Sanders. 2003. Morphological evidence for the phylogeny of Cetacea. Journal of Mammalian Evolution 10:23–124.
- Geisler, J.H., M.R. McGowen, G. Yang, and J. Gatesy. 2011. A supermatrix analysis of genomic, morphological, and paleontological data from crown Cetacea. BMC Evolutionary Biology 11:112.
- Gray, J.E. 1821. On the natural arrangement of vertebrose animals. London Medical Repository 15:296–310.
- Gray, J.E. 1828. Spicilegia Zoologica: Original Figures and Short Systematic Descriptions of New and Unfigured Animals, Part 1. Treüttel, Würtz and Co., London, U.K., 8 pp.
- Gray, J.E. 1846. On the cetaceous animals; pp. 13–53 in J. Richardson and J.E. Gray (eds.), The Zoology of the Voyage of H.M.S. Erebus and Terror, Under the Command of Captain Sir James Clark Ross, R. N., F. R.S., During the Years 1839 to 1843. Volume 1: Mammalia, Birds. E. W. Janson, London, U.K.
- Gray, J.E. 1850. Catalogue of the specimens of Mammalia in the collection of the British Museum, Part I. Cetacea. Trustees of the British Museum, London, U.K., 153 pp.
- Gray, J.E. 1866. Notes on the skulls of dolphins or bottlenosed whales, in the British Museum. Proceedings of the Zoological Society, London 1866:211–216.
- Hershkovitz, P. 1966. Catalog of living whales. Bulletin of the United States National Museum 246:1–259.
- Heyning, J.E. 1989. Comparative facial anatomy of beaked whales (Ziphiidae) and a systematic revision among the families of extant Odontoceti. Contributions in Science, Los Angeles County Museum of Natural History 405:1–64.
- Horikawa, H. 1977. [A new species of fossil dolphin from the Miocene deposits of Kabato Mountainland, Hokkaido, Japan]. Earth Sciencee 31:97–114. . [Japanese]
- Horikawa, H., and I. Fujita. 1972. [A fossil dolphin from the Miocene deposits of Kabato Mountainland, Hokkaido, Japan]. Earth Sciencee 26:176–177. . [Japanese]
- Ichishima, H. 2005. [A re-evaluation of some Japanese cetacean fossils]. Memoir of the Fukui Prefecture Dinosaur Museumm 4:1–20. . [Japanese]
- Ichishima, H., and M. Kimura. 2000. A new fossil porpoise (Cetacea; Delphinoidea; Phocoenidae) from the Early Pliocene Horokaoshirarika Formation, Hokkaido, Japan. Journal of Vertebrate Paleontology 20:561–576.
- Jefferson, T.A., M.A. Webber, and R.L. Pitman. 2008. Marine Mammals of the World: A Comprehensive Guide to Their Identification. Academic Press, San Diego, California, 573 pp.
- Kakimi, T., and T. Uemura, 1958. [Geology of the Atsuta District, Sapporo no. 4]. Geological Survey of Japan, Kawasaki, Japan, 54 pp. . [Japanese]
- Kasuya, T. 1973. Systematic consideration of recent toothed whales based on the morphology of the tympano-periotic bone. Scientific Reports of the Whales Research Institute 25:1–103.
- Kingston, S.E., L.D. Adams, and P.E. Rose. 2009. Testing mitochondrial sequences and anonymous nuclear markers for phylogeny reconstruction in a rapidly radiating group: molecular systematics of the Delphininae (Cetacea: Odontoceti: Delphinidae). BMC Evolutionary Biology 9:245.
- Kobayashi, I., T. Kakimi, T. Uemura, and M. Hata, 1957. [Geology of the Takikawa District, Asahikawa no. 52]. Hokkaido Development Agency, Sapporo, Japan, 36 pp. . [Japanese]
- Lambert, O. 2008. A New Porpoise (Cetacea, Odontoceti, Phocoenidae) from the Pliocene of the North Sea. Journal of Vertebrate Paleontology 28:863–872.
- Lambert, O., and P. Gigase. 2007. A monodontid cetacean from the Early Pliocene of the North Sea. Bulletin de l’Institut Royal des Sciences Naturelles de Belgique, sér. Sciences de la Terre 77:197–210.
- Lawley, R. 1876. Nuovi Studi Sopra ai Pesci ed Altri Vertebrati Fossili delle Colline Toscane. Tipografia dell’Arte della Stampa, Florence, Italy, 122 pp.
- LeDuc, R.G., W.F. Perrin, and A.E. Dizon. 1999. Phylogenetic relationships among the delphinid cetaceans based on full cytochrome b sequences. Marine Mammal Science 15:619–648.
- Linnaeus, C. 1758. Systema naturae per regna tria naturae secundum classes, ordines, genera, species, cum characteribus, differentiis, synonymis, locis, Editio decima, reformata. Salvius, Stockholm, Sweden, 824 pp.
- Maddison, D.R., and W.P. Maddison. 2000. MacClade 4.0. Sinaeur Associates, Sunderland, Massachusetts.
- Maddison, W.P., and D.R. Maddison. 2010. Mesquite: A Modular System for Evolutionary Analysis, version 2.74. . http://mesquiteproject.org. Accessed May 28, 2011.
- Makiyama, J. 1936. Sinanodelphis izumidaensis, a new Miocene dolphin of Japan. Memoirs of the College of Science, Kyoto Imperial University, Series B 11:115–134.
- May-Collado, L.M., and I. Agnarsson. 2006. Cytochrome b and Bayesian inference of whale phylogeny. Molecular Phylogenetics and Evolution 38:344–354.
- McGowen, M.R. 2011. Toward the resolution of an explosive radiation—a multilocus phylogeny 3 of oceanic dolphins (Delphinidae). Molecular Phylogenetics and Evolution 60:345–357.
- McGowen, M.R., M. Spaulding, and J. Gatesy. 2009. Divergence date estimation and a comprehensive molecular tree of extant cetaceans. Molecular Phylogenetics and Evolution 53:891–906.
- McKenna, M.C., and S.K. Bell. 1997. Classification of Mammals above the Species Level. Columbia University Press, New York, 640 pp.
- Mead, J.G., and R.E. Fordyce. 2009. The therian skull: a lexicon with emphasis on the Odontocetes. Smithsonian Contributions to Zoology 627:1–248.
- Messenger, S.L., and J.A. McGuire. 1998. Morphology, molecules, and the phylogenetics of cetaceans. Systematic Biology 47:90–124.
- Meyen, F.J. F. 1833. Beitrage zur Zoologie gesammelt auf einer Reise um die Erde. Saugethiere. Nova Acta Academiae Caesareae Leopoldino-Carolinae 16:551–610.
- Muizon, C.de. 1984. Les vertébrés fossiles de la Formation Pisco (Pérou). Deuxième partie: les odontocètes (Cetacea, Mammalia) du Pliocène inférieur de Sud Sacaco. Institut Français D’Études Andines Mémoire 50:1–188.
- Muizon, C.de. 1987. The affinities of Notocetus vanbenedeni, an Early Miocene platanistoid (Cetacea, Mammalia) from Patagonia, southern Argentina. American Museum Novitates 2904:1–27.
- Muizon, C.de. 1988a. Les relations phylogénétiques des Delphinida (Cetacea, Mammalia). Annales de Paléontologie 74:159–227.
- Muizon, C.de. 1988b. Les vertébrés fossiles de la Formation Pisco (Pérou). Troisiéme partie: les odontocétes (Cetacea, Mammalia) du Miocéne. Institut Français D’Études Andines Mémoire 78:1–244.
- Muizon, C.de. 1991. A new Ziphiidae (Cetacea) from the Early Miocene of Washington State (USA) and phylogenetic analysis of the major groups of odontocetes. Bulletin du Muséum National d’Histoire Naturelle, Paris 4 série 12:279–326.
- Murakami, M., C. Shimada, Y. Hikida, and H. Hirano. 2012a. A new basal porpoise, Pterophocaena nishinoi (Cetacea, Odontoceti, Delphinoidea), from the upper Miocene of Japan and its phylogenetic relationships. Journal of Vertebrate Paleontology 32:1157–1171.
- Murakami, M., C. Shimada, Y. Hikida, and H. Hirano. 2012b. Two new extinct basal phocoenids (Cetacea, Odontoceti, Delphinoidea), from the upper Miocene Koetoi Formation of Japan and their phylogenetic significances. Journal of Vertebrate Paleontology 32:1172–1185.
- Nishiwaki, M. 1965. [Whales and Pinnipeds]. University of Tokyo Press, Tokyo, Japan, 439 pp. . [Japanese]
- Perrin, W.F. 1975. Variation of spotted and spinner porpoise (Genus Stenella) in the Eastern Pacific and Hawaii. Bulletin of the Scripps Institution of Oceanography 21:1–206.
- Perrin, W.F., B. Würsig, and J.G. M. Thewissen. 2002. Encyclopedia of Marine Mammals. Academic Press, San Diego, California, 1414 pp.
- Perrin, W.F., E.D. Mitchell, J.G. Mead, D.K. Caldwell, and P.J. H. Van Bree. 1981. Stenella clymene, a rediscovered tropical dolphin of the Atlantic. Journal of Mammalogy 62:583–598.
- Perrin, W.F., E.D. Mitchell, J.G. Mead, D.K. Caldwell, M.C. Caldwell, Bree, P.J. H. Van Bree, and W.H. Dawbin. 1987. Revision of the Spotted dolphins, Stenella spp. Marine Mammal Science 3:99–170.
- Pilleri, G. 1988. Pre- and post-Messinian Cetacea in the Mediterranean Tethys and the Messinian “Salinity Crisis”; pp. 19–40 in G. Pilleri (ed.), Contributions to the Paleontology of some Tethyan Cetacea and Sirenia (Mammalia) I. Brain Anatomy Institute, University of Berne, Ostermundigen, Switzerland.
- Pilleri, G., and H.J. Siber. 1989. Neuer delphinid (Cetacea, Oddontoceti) aus der Pisco-Formation Perus; pp. 166–191 in G. Pilleri (ed.), Beiträge zur paläontologia der Cetacean Perus. Hirnanatomisches Institut der Universität Bern, Ostermundigen, Switzerland.
- Portis, A. 1886. Catalogo descritivo dei Talassoterii rinvemuti nei terreni terziari del Piemonte e della Liguria. Memorie Reale Accademia delle Scienze Trino, Series II 37:245–365.
- Post, K., and M, Bosselaers. 2005. Late Pliocene occurrence of Hemisyntrachelus (Odontoceti, Delphinidae) in the southern North Sea. Deinsea 11:29–45.
- Sagayama, T. 1989. [Diatom Zones of the Neogene around the Ishikari Lowland, central Hokkaido]. Report of the Geological Survey of Hokkaidoo 60:177–186. . [Japanese]
- Sagayama, T. 2000. [Middle Miocene to Pliocene stratigraphy and movement of sedimentary basin in Neogene of Hokkaido, Japan]. Report of the Geological Survey of Hokkaidoo 71:59–102. . [Japanese]
- Sato, H., M. Hata, I. Kobayashi, S. Yamaguchi, and M. Ishida. 1964. [Geology of the Kokuryo District, Asahikawa no. 46]. Geological Survey of Japan, Sapporo, Japan, 55 pp. . [Japanese]
- Sepkoski, J.J. 2002. A compendium of fossil marine animal genera. Bulletins of American Paleontology 363:1–560.
- Simonelli, V. 1911. Anzi di “Tursiops” del Pliocene Senese. Memorie della R. Accademia delle Science dell’Instituto di Bologna Classe di Scienze Fisiche 8:249–260.
- Slater, G.J., S.A. Price, F. Santini, and M.E. Alfaro. 2010. Diversity versus disparity and the radiation of modern cetaceans. Proceedings of the Royal Society of London, Series B 277:3097–3104.
- Steeman, M.E., M.B. Hebsgaard, R.E. Fordyce, S.Y. W. Ho, D.L. Rabosky, R. Nielsen, C. Rahbek, H. Glenner, M.V. Sørensen, and E. Willerslev. 2009. Radiation of extant cetaceans driven by restructuring of the oceans. Systematic Biology 58:573–585.
- Swofford, D.L. 1998. PAUP 4.0. Sinauer Associates, Sunderland, Massachusetts.
- Thewissen, J.J. M., and B.J. Schneider. 2009. Marine mammal evolution; pp. 692 in W.F. Perrin, B. Würsig, and J.G. M. Thewissen (eds.), Encyclopedia of Marine Mammals, second edition. Academic Press, San Diego, California.
- True, F.W. 1889. Contributions the natural history of cetaceans, a review of the family Delphinidae. Bulletin of the United States National Museum 36:1–197.
- Tsuji, T., Y. Saito, Y. Ikuji, T. Ichinoseki, A. Obuse, K. Kai, and Y. Yanagimoto. 1992. [Sedimentary environments and sequences of the Ponsubetsu and Subetsu formations of the Minenobu Gas Field, northern Ishikari plain, central Hokkaido]. Research Reports of the Japan Petroleum Exploration Research Center 8:1–26. . [Japanese]
- Tsushima, K., T. Kakimi, and T. Uemura. 1956. [Geology of the Atsuta District, Sapporo no. 3]. Geological Survey of Japan, Kawasaki, Japan, 24 pp. . [Japanese]
- Whitmore, F.C. Jr., and J.A. Kaltenbach. 2008. Neogene Cetacea of the Lee Creek Phosphate Mine, North Carolina; pp. 181–269 in C.E. Ray, D.J. Bohaska, I.A. Koretsky, L.W. Ward, and L.G. Barnes (eds.), Geology and Paleontology of the Lee Creek Mine, North Carolina, IV. Virginia Museum of Natural History Special Publication 14.
- Watanabe, M., and F. Yoshida. 2000. [Geology of the Ebishima District, Asahikawa no. 42]. Geological Survey of Japan, Tsukuba, Japan, 61 pp. . [Japanese]
- Xiong, Y., M.C. Brandley, S. Xu, K. Zhou, and Y. Guang. 2009. Seven new dolphin mitochondrial genomes and a time-calibrated phylogeny of whales. BMC Evolutionary Biology 9:20.
- Yanagisawa, Y., and Akiba, F. 1998. Refined Neogene diatom biostratigraphy for the northwest Pacific around Japan, with an introduction of code numbers for selected diatom biohorizons. Journal of the Geological Society of Japan 104:395–414.