ABSTRACT
Billfishes include some of the largest pelagic teleostean species, but several aspects about their morphology, paleobiology, and evolution remain ambiguous. Their fossil record is fragmentary and mostly represented by rostral and skull remains. Here, we present a comparative study of the caudal vertebral morphology of extant istiophorid species and we use this information to describe two fossil vertebrae from the Gatun and Chagres formations, both from the late Miocene of Panama. The caudal vertebra from the Gatun Fm. is characterized by the presence of a lateral apophysis and identified as Makaira sp., while the vertebra from the Río Indio Member of Chagres Fm. lacks this structure and its morphology indicates a different genus. The estimated total length of the Makaira sp. specimen from Gatun Fm. is about 5.18 m, the largest size calculated for a marlin, while the Rio Indio specimen was about 2.56 m long. Phylogenies show that the radiation of most living istiophorid species seemingly occurred during the Pliocene. However, our results highlight that the genus Makaira and the taxa lacking the lateral apophyses occurred during the late Miocene, showing that the radiation of istiophorids most likely occurred before the Pliocene (ca. 5 Ma).
INTRODUCTION
Istiophorid billfishes include large oceanic epipelagic predators that occur in tropical and subtropical waters (Nakamura, Citation1983, Citation1985; Pepperell, Citation2010). Elongated upper jaws forming a rostrum and a strong and flexible, highly specialized vertebral column composed of 24 interlocked vertebrae characterize these fishes () (Nakamura et al., Citation1968; Nakamura, Citation1983; Davie, Citation1990). The phylogenetic relationships and the genetics of extant istiophorid species have been extensively studied because of their importance for fisheries and recreational fishing (Graves and McDowell, Citation1995, Citation2012, Citation2015; Hoolihan et al., Citation2004; Collette et al., Citation2006; Little et al., Citation2010; Hanner et al., Citation2011; Bernard et al., Citation2013; Santini and Sorenson, Citation2013; Williams et al., Citation2018). Conversely, morphological studies of extant taxa are proportionally fewer, hampering our understanding of their fossil record and trait evolution (Gregory and Conrad, Citation1937; Morrow and Posner, Citation1957; Nakamura et al., Citation1968; Nakamura, Citation1983, Citation1985; Hebrank et al., Citation1990; Fierstine and Voigt, Citation1996). Fossil istiophorid remains are relatively common in Neogene deposits but most records consist only of rostral fragments and isolated bones that render taxonomic identifications difficult (Fierstine and Applegate, Citation1968; Fierstine and Walters, Citation1968; Fierstine, Citation1974, Citation1990, Citation1999a, Citation1999b; Gottfried, Citation1982; Fierstine and Welton, Citation1988; Fierstine and Voigt, Citation1996; Carnevale et al., Citation2002). However, some specimens from the Pliocene of California and Virginia in the U.S.A., and the Pleistocene of the Philippines were assigned to extant species such as Makaira nigricans, Istiompax indica, Istiophorus platypterus, and Kajikia albida based on diagnostic features of preserved bony elements (Gottfried, Citation1982; Fierstine and Welton, Citation1983; Fierstine, Citation2001). The taxonomy of isolated and fragmentary bones of Miocene specimens nevertheless remains inaccurate and fossils are commonly left in open nomenclature or identified as Makaira sp. and Makaira aff. M. nigricans (Lériche, Citation1926; Fierstine and Applegate, Citation1968; Fierstine, Citation1978, Citation1998, Citation1999a; Fierstine et al., Citation2001; Carnevale et al., Citation2002; Carnevale and Godfrey, Citation2018). Here, we provide a framework to identify fossil istiophorids using isolated caudal vertebrae and we additionally use this information to describe two new fossils from the late Miocene of Panamá. The morphological study presented herein also provides fossil evidence about the time of origin and radiation of extant istiophorids.
FIGURE 1. Regions, structures and variables of measured caudal vertebrae in istiophorid billfishes. A, vertebral column of Istiompax indica showing the regions of the caudal vertebrae; B–C, generalized istiophorid caudal vertebrae showing the variables measured in fossils and extant specimens; B, right lateral view; C, dorsal view; D–G, shape variation of the neural spine in istiophorids in left lateral view with corresponding generalizations on the right side; D, triangular spine of Istiophorus; E, quadrangular spine of Makaira and Istiompax; F, rhomboid spine of Kajikia; G, rectangular spine of Tetrapturus spp; H–I, generalized istiophorid hypural plate; H, left lateral view; I, anterior view; J, left dentary, medial view of the interdentary joint. Modified from Fierstine (Citation2001) and Davie (Citation1990).
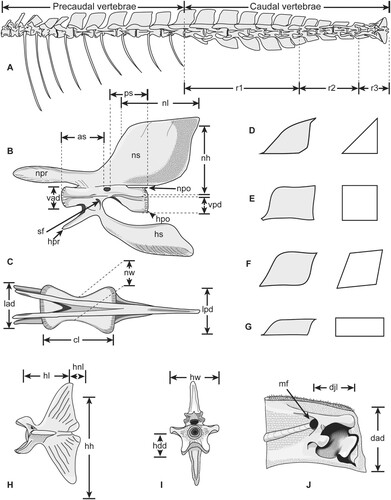
STUDY AREA AND GEOLOGICAL SETTING
Here, we studied fossil remains from two localities in Panama, San Judas Tadeo and Vista Hermosa (). In the San Judas Tadeo quarry (9°21'7.92''N, 79°50'9.24''W; ), the lower Member of the Gatun Formation is exposed () (section No. 10 of Hendy [Citation2013] at stratigraphic meters 160–200 m), which was dated to 10.5–12 Ma (Hendy, Citation2013). It is composed of volcaniclastic conglomerates, pebbly sandstones, fine sandstones, and siltstone with abundant concretions distributed in densely packed horizons and Thalassinoides burrows.
FIGURE 2. Geological maps showing the fossil localities where istiophorid remains were recovered from the Gatun Fm. and Rio Indio Member of Chagres Fm. A, Salud to Miguel de la Borda map of the Caribbean West coast of Panama, displaying the fossil locality at Vista Hermosa quarry; B, Central Colon geological map showing the distribution of the Gatun Fm. and the fossil locality at the San Judas Tadeo quarry in Cativa; C, lithostratigraphy, age, and paleobathymetry of the Chagres and Gatun formations. Modified after Coates et al. (Citation1992), Collins et al. (Citation1996), and Hendy (Citation2013).
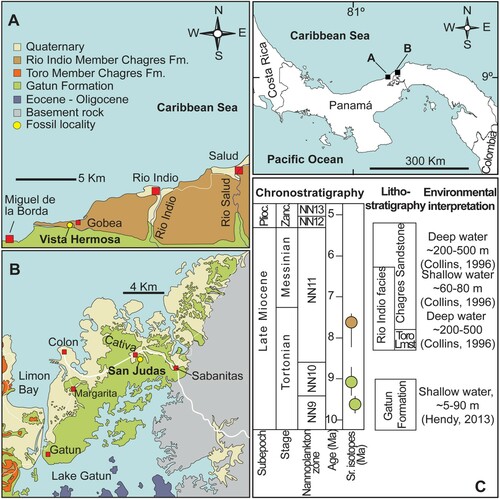
The fossiliferous horizons of the Vista Hermosa locality (9°9'57.60''N, 80°15'28.80''W; ) correspond to the Río Indio Member of the Chagres Formation, stratigraphic section No. 5 (samples 1639–1643) of the Panama Paleontology Project (PPP) that includes the lowermost 25 m of the Río Indio Member and which is dated to ca. 8.3–7.1 Ma (Coates, Citation1999; Collins, Citation1999). The Río Indio Member at this section is characterized by blue-gray claystone and siltstones with abundant mollusks (Coates, Citation1999).
MATERIAL AND METHODS
Descriptive and Analytical Procedure
We compared the caudal vertebral morphology in all extant species of istiophorids (). In Makaira nigricans and Istiompax indica the caudal vertebrae are in positions 12–24, while in Istiophorus, Kajikia spp., and Tetrapturus spp. they are in positions 13–24 (). Descriptions for vertebral caudal morphology in extant istiophorids emphasize three regions and two structures (). The first region includes the vertebral series with similar morphologies from the first caudal vertebra (starting at vertebrae 12 or 13) up to position 17 (). The second region includes vertebral series from position 18–21 and the third region includes the vertebrae forming the caudal peduncle (position 22–24) () and the caudal fin skeleton (Davie, Citation1990). The emphasized structures include the shape of the neural spine () and the presence of the transverse process. The method used to describe istiophorid vertebrae follows a combination of Fierstine (Citation2001) and Nakamura (Citation1983). For caudal vertebrae from the first position up to vertebra 23, eight variables can be measured for fossils and extant specimens (). For the last vertebra (hypural plate), five different variables are measured here ().
The shape of the neural spine in extant species is studied in the first caudal vertebra using the ratios of Nakamura (Citation1983) (). As the vertebrae from region 3 (caudal peduncle) are highly modified and each one has a different shape, we performed three individual PCA analyses for vertebrae 22, 23, and 24 in extant species to compare their morphologies. These analyses detect individual vertebrae patterns from the caudal peduncle that ultimately allow species determination using such measurements () (Nakamura, Citation1983; Fierstine, Citation2001). For the PCA, we used the functions ‘fviz_pca_var’ and ‘fviz_pca_ind’ of the ‘factoextra’ package (Kassambara, Citation2017; Kassambara and Mundt, Citation2017) and the function ‘prcomp’ of the R base statistics (R Core Team, Citation2020) to obtain the percentage of variance explained by the first two principal components (PC). As one fossil individual includes an associated dentary, we included a comparative analysis for two variables in this bone () in four extant marlin species using a one-way ANOVA followed by a Tukey HSD test. All analyses presented herein were made in R and the data are included in the supplemental data (Tables S1–S3).
We employed the Fierstine (Citation2001) method to describe fossil billfishes using vertebral features and the dentary bone (). This method converts the variables (, ) into ratios () to reduce size variations and to enable the analysis of these isolated elements (Fierstine and Voigt, Citation1996; Fierstine, Citation2001). For the descriptions, we employed a combination of the osteological terminology of Gregory and Conrad (Citation1937), Davie (Citation1990) and Rojo (Citation2017). The common names for extant species follow Pepperell (Citation2010). Position of the fossil vertebrae in the vertebral column is assigned according to observed morphologies in extant species (). Accordingly, we first determined the position of the vertebrae within the vertebral column (region; ) based on the general morphology and then, the exact position was established using the shape of the spines, hemal prezygapophysis, and the intervertebral arc (). The systematic scheme for fossil billfishes described here follows that of Nelson et al. (Citation2016).
TABLE 1. Selected variables measured from the first caudal (v 13 and v 14) for five istiophorid species compared with measurements from fossils from Gatun and Chagres Formations.
TABLE 2. Ratios for vertebral variables of five extant istiophorid species compared with fossil vertebrae from Gatun and Chagres formations.
Body Size Estimation
The body size of the fossil specimens was estimated based on the proportion method using the centrum length and the body size of a mounted skeleton of Istiompax indica as constraint (AMS 1.38913–001). The length of the fossil vertebra was compared with the measurement of the equivalent vertebrae in the skeletal specimen with known measurements to estimate the total length (TL) and lower jaw fork length (LJFL). Linear measurements of bones were made to the nearest 0.01 mm with digital calipers or using the digital image measure tool of Adobe Photoshop. Nevertheless, we give the measurements rounded to compensate for any measurement inaccuracies.
Comparative Specimens
Extant Species—Xiphias gladius (swordfish): NHMW, uncataloged completely mounted skeleton in the exhibition hall; USNM 110042, complete skeleton. Istiophorus platypterus (sailfish): NHMW uncataloged, completely mounted skeleton in the exhibition hall, MNHN 6960 complete skeleton, STRI 1628 complete skeleton. Makaira nigricans (blue marlin): LACM 46023–1, vertebral column; USNM 196019, complete skeleton. Istiompax indica (black marlin): AMS 1.38913–001, complete skeleton; LACM 25509, complete skeleton; Kajikia albida (white marlin): USNM 270766 complete skeleton; USNM 3605507, axial skeleton. Kajikia audax (striped marlin): MNHN 6821 complete skeleton, USNM 372777 complete skeleton. Tetrapturus pfluegeri (long bill spearfish): LACM 25462, complete skeleton; UF 208789 vertebral column. Tetrapturus belone: NHMW 93902, complete skeleton; MNHN A-7504, complete skeleton. Tetrapturus angustirostris (short bill spearfish): LACM 25499, complete skeleton. Additional material for extant species is listed in the supplemental material (Table S1).
Fossil Specimens—Field campaigns to the San Judas Tadeo and Vista Hermosa localities were conducted in August 2010 and April 2014, and yielded a single vertebra and two vertebrae, and a dentary fragment, respectively (). The fossils were prepared mechanically with carbon fiber needles, air scribe chisels and rotatory flexible shaft tools, and subsequently chemically stabilized with paraloid PB72 and paleobonds PB40 and PB002. All specimens are housed in the fossil collections of the Museo de Paleontología de Panamá under collection numbers MUPAN-STRI 46871 and MUPAN-STRI 31427.
FIGURE 3. A, right lateral view of the fossil caudal vertebrae of Makaira sp. (MUPAN-STRI 46871) from Gatun Fm.; B, detail of the anterior section of the centrum showing the broken lateral apophysis; C, dorsal view with the approximate reconstruction of the lateral apophysis in gray color; D, anterior surface of the centrum with reconstructed lateral apophysis in gray color; E, posterior surface of the centrum; F, left lateral view of the two articulated caudal vertebrae from Río Indio (MUPAN-STRI 31427) with a small piece of a neural prezygapophysis from a third vertebra attached; G, dorsal view as oriented in F; H, left dentary joint associated with Río Indio specimen in right lateral view; I, left articular associated with the Río Indio fossil vertebrae in right lateral view. Gray marks show the approximate reconstruction for missing parts, arrow indicates the intervertebral arc formed by the hemal spine and hemal prezygapophysis in F. Scale bar equals 5 cm.
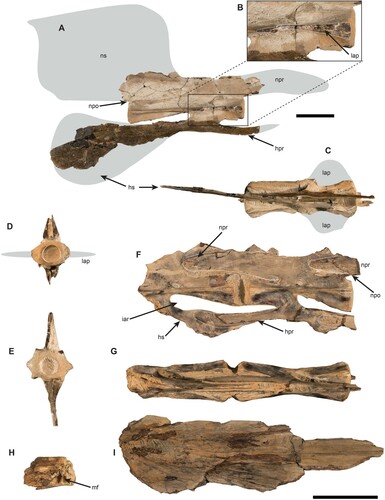
Institutional Abbreviations—AMS, Australian Museum of Natural History, Sydney, Australia; CTPA, Center of Tropical Paleontology and Archaeology of STRI, Panama, City of Panama, Panama; LACM, Natural History Museum of Los Angeles Country, Los Angeles, California, U.S.A.; MNHN, Muséum National d’Histoire Naturelle (Paris), Paris, France; MUPAN, Museo de Paleontología de Panamá, Universidad de Panamá, City of Panama, Panama; NHMW, Natural History Museum Vienna, Vienna, Austria; STRI, Smithsonian Tropical Research Institute, Panama, City of Panama, Panama; UF, Florida Museum of Natural History, Gainesville, Florida, U.S.A.; USNM, Smithsonian National Museum of Natural History, Washington, D.C., U.S.A.
Anatomical Abbreviations—hpo, hemal postzygapophysis; hpr, hemal prezygapophysis; hs, hemal spine; iar, intervertebral arc; lap, lateral apophysis; mf, maxillary foramen; npo, neural postzygapophysis; npr, neural prezygapophysis; ns, neural spine; r, regions for caudal vertebrae; sf, spinal foramen; v, vertebra.
Variable Abbreviations—as, length from the anterior edge of the centrum to the anterior margin of the spinal foramen; cl, centrum length; dad, interdentary joint length; djl, length of the interdentary joint from the mandibular foramen to the anterior-most denticles; hdd, dorsoventral diameter of the anterior surface of the centrum; hh, length from the dorsal tip of the hypural plate to the ventral tip; hl; length from the anterior edge of the centrum to the hypural notch; hnl, hypural notch length; hw, largest width across the hypurapophyses; lad, lateral diameter of the anterior surface of the centrum; lpd, lateral diameter of the posterior surface of the centrum; nh, neural spine height; nl, neural spine length; nw, narrowest width of the centrum as seen from the ventral surface; ps, length from the posterior edge of the centrum to the posterior margin of the spinal foramen; vad, dorsoventral diameter of the anterior surface of the centrum; vpd, dorsoventral diameter of the posterior surface of the centrum.
SYSTEMATIC PALEONTOLOGY
Subclass NEOPTERYGII Regan, Citation1923
Infraclass TELEOSTEI Müller, Citation1845
Cohort EUTELEOSTOMORPHA Greenwood, Rosen, Weitzman, and Myers, Citation1966
Subcohort NEOTELEOSTEI Rosen, Citation1973
Infracohort EURYPTERYGIA Rosen, Citation1973
Section CTENOSQUAMATA Rosen, Citation1973
Subsection ACANTHOMORPHATA Betancur-R., Broughton, Wiley, Carpenter, López, Holcroft, Arcila, Sanciangco, Cureton II, Zhang, Buser, Campbell, Ballesteros, Roa-Varon, Willis, Borden, Rowley, Reneau, Hough, Lu, Grande, Arratia, and Ortí, Citation2013
Division ACANTHOPTERYGII Johnson and Patterson, Citation1993
Subdivision PERCOMORPHACEAE Betancur-R., Broughton, Wiley, Carpenter, López, Holcroft, Arcila, Sanciangco, Cureton II, Zhang, Buser, Campbell, Ballesteros, Roa-Varon, Willis, Borden, Rowley, Reneau, Hough, Lu, Grande, Arratia, and Ortí, Citation2013
Series CARANGARIA Betancur-R., Broughton, Wiley, Carpenter, López, Holcroft, Arcila, Sanciangco, Cureton II, Zhang, Buser, Campbell, Ballesteros, Roa-Varon, Willis, Borden, Rowley, Reneau, Hough, Lu, Grande, Arratia, and Ortí, Citation2013
Order ISTIOPHORIFORMES Betancur-R., Broughton, Wiley, Carpenter, López, Holcroft, Arcila, Sanciangco, Cureton II, Zhang, Buser, Campbell, Ballesteros, Roa-Varon, Willis, Borden, Rowley, Reneau, Hough, Lu, Grande, Arratia, and Ortí, Citation2013
Family ISTIOPHORIDAE Robins and de Sylva, Citation1960
MAKAIRA Lacépède, Citation1802
, and
Referred Material—Makaira sp. MUPAN-STRI 46871 () is a vertebral element, which was located in the caudal position 13, with well-preserved centrum and partially preserved hemal prezygapophyses, but lacking neural spine and the neural prezygapophyses ().
Locality and Age—The vertebral element was recovered from the San Judas Tadeo quarry, Cativá, Colón Province (9°21'7.92''N, 79°50'9.24''W) on the Caribbean coast of Panama and comes from the lower Member of the Gatun Fm. (), which is of late Miocene (Tortonian) age, ca. 10.5 to 12.0 Ma (Hendy Citation2013).
Description—The vertebral element MUPAN-STRI 46871 is light brown; portions with dark brown are due to structural adhesive PB40, which was applied during preparation. The specimen was partially exposed in the field level with several fractures and a hard-calcareous matrix still is attached to some parts. The vertebral element is well preserved () and its length measures 154 mm. The distance between the anterior margins and the spinal foramen is slightly larger (as = 76 mm) than the distance from the posterior margin to the spinal foramen (ps = 74 mm). The vertebra has a lateral longitudinal fracture on both sides at its anterior end that corresponds with the lateral apophyses, which are missing (). Anteriorly, the centrum is concave and oval being wider (lad = 50 mm) than high (vad = 33 mm) with thickened lateral margins (). The posterior surface of the centrum is hexagonal in outline and wider than high (lpd = 49, vpd = 31; ). The hemal spine is very closely placed to the posterior end of the centrum (). The smallest width of the centrum (nw) is 45 mm resulting in a short spoon appearance in dorsal and ventral views (). The neural spine seems to have been large and likely to be quadrangular according to its preserved base. However, it got lost during transport previous to preparation and is reconstructed here with linear projections (). The hemal spine is nearly complete and has an elongated, oval shape that is diagnostic for the caudal position 13 (). The neural prezygapophysis is only basally preserved, while the hemal prezygapophysis is broken distally but its rounder borders allow reconstructing the shape reconstruction ().
Remarks—The present vertebral element is assigned to Makaira sp., because it displays strongly developed lateral apophyses and has an ovate hemal spine, which is indicative of position 13 (, ) instead of a pointed and rectangular hemal spine as seen in Istiompax (). A species assignment, however, is not possible. Based on the centrum length and using the vertebrae 13 of Istiompax indica as template, the body to fork length is estimated to be ca. 402 cm and the total body length to be ca. 518 cm. The presence of lateral apophyses is a character found only in the largest extant species, Makaira nigricans and Istiompax indica (Nakamura et al., Citation1968; Nakamura, Citation1983, Citation1985; Davie, Citation1990). Fossils of Makaira spp. are reported at least since the middle Miocene (Fierstine, Citation1978, Citation1998, Citation1999b, Citation2006), whereas the fossil record of genus Istiompax extends only back into the early Pliocene (Gottfried, Citation1982; Fierstine, Citation2001). In the discussion section below, this specimen is referred to as the ‘Gatun vertebra.’
ISTIOPHORIDAE gen. et sp. indeterminate
, and
FIGURE 4. Morphology of the caudal vertebrae in istiophorid billfishes. A–D, comparison of the neural spine shape in caudal vertebrae of istiophorid billfishes. A, first and second caudal vertebrae of Makaira nigricans (USNM 196019); B, first and second caudal vertebrae of Istiompax indica (AMS 1.38913–001); C, vertebra 16 of Makaira nigricans (USNM 196019); D, vertebra 15 of Kajikia audax (USNM 372777); E, first and second (v 13–14) caudal vertebra of Kajikia albida (USNM 360507), the v 13 shows the lack of the extended prezygapophysis and both show the reduced hemal spine; F, vertebra 21 of Kajikia albida (USNM 360507) showing the second and big spinal foramen; G, vertebra 14 of Istiophorus platypterus (MNHN 6960); H, vertebra 15 of Tetrapturus angustirostris (LACM 25499). The pink shadow indicates the space formed by the intervertebral arc. Color available only in the online version. Scale bar equals 10 cm in A, C, in B–H scale bar equals 5 cm.
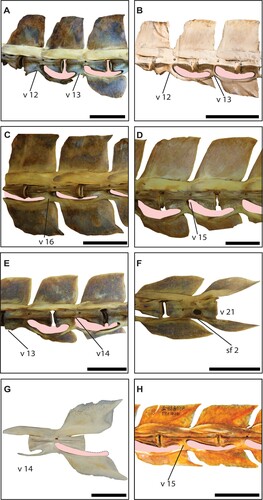
Referred Material—Istiophoridae gen. et sp. indeterminate MUPAN-STRI 31427 () includes two articulated caudal vertebrae, which were found associated with an incompletely preserved left dentary and angulo-articular bones.
Locality and Age—The material was recovered from the ground level in a new site belonging to the Vista Hermosa quarries at the entrance of the road to Vista Hermosa Village in the Donoso district of Colon Province (9°9'57.60''N, 80°15'28.80''W) on the north-western Caribbean coast of Panama (). Here, sediments of the Chagres formation are exposed, which are of late Miocene (Tortonian/Messinian) in age and date to ca. 8.3–7.1 Ma (Collins et al., Citation1996).
Description
Vertebrae—The specimen consists of two articulated vertebrae that were preserved within a calcareous, light-grayish concretion with associated remains of mollusk shells and light-brown bony fragments (). The anterior vertebra comprises a partially preserved centrum, whereas the second one is completely preserved and has a small piece of a neural prezygapophysis from a third vertebra attached (). Measurements for this specimen were taken from the second, complete centrum (). The vertebra has a centrum length of 81.58 mm. The distance from the anterior and posterior margins, respectively, to the spinal foramen are almost the same (as = 39, PS = 37). Both vertebrae lack lateral apophyses (). The anterior and posterior surfaces of the centrum are oval and wider (lad = 29 mm, lpd = 29) than high (vad = 22 mm, vpd = 24 mm) without thickened lateral borders. The narrowest width of the centrum (nw) is 19 mm giving it an hourglass shape (). The neural and hemal spines are completely missing so that it is impossible to establish their shape and size (). The neural and hemal prezygapophyses, conversely, are completely preserved and display the typical pattern of the intervertebral joint for istiophorids (). The vertebra also preserves the neural postzygapophysis, which is relatively short, dorsoventrally high and the spur arises anterior to the posterior end of the centrum ().
Jaw Elements—A poorly preserved left dentary and angulo-articular bone were found associated with the vertebral elements (). The dentary preserves its distal end, the mandibular foramen, and the symphyseal surface (). The anterior tip is wide and high (). The angulo-articular bone is nearly complete, but lacks the ventral and dorsal margins, and the socket-like region for articulation with the quadrate ().
Remarks—The vertebrae are abraded and broken without preserving any diagnostic character for assigning it to any of the extant genera Istiophorus, Kajikia, or Tetrapturus employing the identification scheme of Nakamura (Citation1983, Citation1985). Based on the shape of the intervertebral arc morphology and by having hemal prezygapophysis extended upward widely surpassing the intervertebral joint (), the two vertebrae belong to region 1 of the vertebral column and are likely to have been situated between positions 14–17 () as indicated by those extant istiophorid genera that lack lateral apophyses ().
We calculated the body size using the centrum length of the vertebrae 16 of the extant Istiompax indica as template. Accordingly, we estimated a LJFL of ca. 199 cm and a TL of ca. 256 cm for the fossil specimen. The estimated size for this specimen is well within the range of species that lack lateral apophyses (Nakamura, Citation1985).
The most distal tip of the dentary is preserved with the mandibular foramen (). The size and shape of the dentary and the angulo-articular evidence that the lower jaw is wide, resembling that found in Makaira spp. to some extent. However, the lack of the lateral apophyses in the caudal vertebrae () is a trait observed in species of Istiophorus, Kajikia, and Tetrapturus (), but not in those of Makaira. The wide dentary and vertebrae lacking lateral apophysis in the specimen MUPAN-STRI 31427 are a character combination observed for the first time in the family Istiophoridae (). In the following discussion, this specimen is referred to as the ‘Río Indio vertebra.’
RESULTS AND DISCUSSION
Morphology and Position of the Caudal Vertebrae in Istiophorids
Morphology—Twenty-four highly modified vertebrae with elongated prezygapophyses and laterally flattened neural and hemal spines characterize the vertebral column of an istiophorid (, ; Nakamura Citation1955, Citation1983, Citation1985). Two main groups of istiophorids can be separated using the vertebral formula and the presence of lateral apophysis in the vertebral elements (Nakamura, Citation1983; Davie, Citation1990). The first group comprises the genera Makaira and Istiompax with a vertebral formula 11 + 13, and vertebral elements with developed lateral apophyses and very wide and tall neural spines (, ). The second group includes Istiophorus, Kajikia, and Tetrapturus that is characterized by the formula 12 + 12 and the lack of lateral apophyses (, ) (Nakamura, Citation1955, Citation1983, Citation1985; Davie, Citation1990). The vertebral traits enable to identify extant species in monotypic genera such as Istiophorus, Makaira, and Istiompax. Therefore, differentiation between species of Kajikia and Tetrapturus are more difficult (, ) (Nakamura, Citation1955, Citation1983, Citation1985). The neural and hemal spines are quadrangular and similar morphologically in Makaira and Istiompax species (, , ). Nevertheless, Is. indica can be differentiated, because the hemal spines are more rectangular and lower than the neural spines between positions v 14–17 (, ), the hemal postzygapophyses that arise close to the centrum margin are longer (), and the intervertebral arc is wider since the posterior end of the centrum is not closely situated to the hemal prezygapophysis (). In Makaira nigricans, both neural and hemal spines are similar in size and shape (, ), the hemal postzygapophyses are shorter and originate at the posterior margin of the centrum, and the intervertebral arc is narrower (, ). The neural spines are rhomboid in the white and striped marlins (Kajikia spp.) and the intervertebral arcs are dorsoventrally wider than in any other genera (, ). In sailfish (I. platypterus) the spines are triangular and the intervertebral arc is elongated (, ). In spearfishes (Tetrapturus spp.), the neural spines, conversely, are rectangular (markedly wider than high) and the vertebral centrum is very elongated as well as the intervertebral arc (, , ). The shape variation of the neural spines across different genera was also expressed by plotting the ratios nh/cl and nl/cl for the first caudal vertebrae (, ) (Nakamura, Citation1983). Our morphometrics comparisons show that the shape of the neural spine can be used to discriminate species in monotypic genera and group the species of Kajikia and Tetrapturus together when isolated vertebrae from region 1 are compared (, , ), since the shape of the spines is best expressed in this region (, ). The results obtained for vertebra 14 () are comparable with other vertebrae from region 1 (, , ), because the neural spine does not change in shape along this region (Nakamura, Citation1983; Fierstine, Citation2001).
Figure 5. A–B, vertebrae 12–14 of Makaira nigricans (USNM 196019) showing the transverse process. A, ventral view and B, dorsal view. C–E, comparison of the caudal vertebral series from v 16–21 showing the shape change of the neural spine (note the shape change in the intervertebral arc that reduces its size progressively starting from v 18). C, Makaira nigricans (USNM 196019); D, Istiompax indica (AMS 1.38913–001), E, Tetrapturus angustirostris (LACM 25499). F–J, morphological comparison of the caudal peduncle (v 22–24) in four marlin species, showing the morphological differences not detected in the PCA analysis. F, Makaira nigricans (USNM 196019); G, Kajikia audax (USNM 372777); H, Kajikia albida (USNM 360507); I, Istiophorus platypterus (MNHN 6960); J, Tetrapturus pfluegeri (UF 208789). Dashed line shows the position where neural spine is reduced to place the pterygiophores of the second dorsal fin and the pink shadow indicates the space formed by the intervertebral arc in C–D. Color available only in the online version. Scale bars equal 10 cm in A–C and equal 5 cm in D–J.
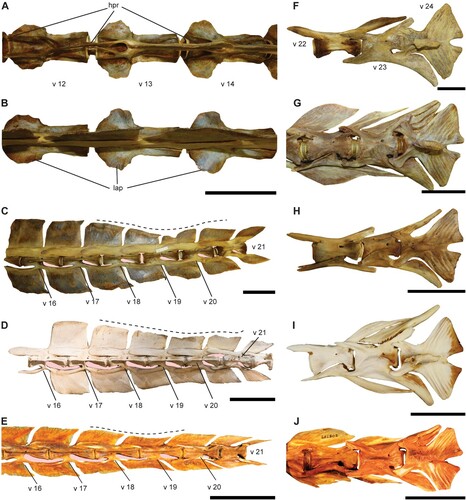
FIGURE 6. A, PCA analysis of caudal vertebra 22; B, PCA plot for caudal vertebra 23; C, PCA plot for the hypural plate (v 24). D, comparison of the ratios of nh/cl and nl/cl in the first caudal vertebra for five marlin and two spearfishes showing the shape variation in the neural spine in different genera; E, Boxplot of the dentary height (DAD) in four marlin species. D has been taken and modified from Nakamura (Citation1983); in E, the numbers of the x-axis correspond with the numbers in parentheses for the species labels.
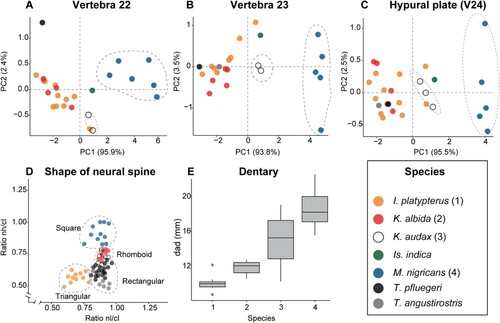
Position—The shapes of the istiophorid caudal vertebrae change according to their position in the vertebral column (, , ). The caudal vertebrae were divided into three regions according to their morphology for comparative purposes (). The first region extends from the first caudal vertebrae (starting at position 12 in Makaira and Istiompax or starting at position 13 in all other genera) up to position 17 (). In region 1, the exact position of the first and second caudal vertebra is easily recognized (). Both have characteristically reduced or slender, elongated to oval hemal spines (). Additionally, the first caudal lacks the elongated hemal prezygapophyses (). For other vertebrae of region 1, the shape is almost constant and it is possible to assign their exact position by using the shape of the intervertebral arc and the neural spine, which, nevertheless, is more difficult (). Region 2 includes the caudal vertebrae between positions 18–21, in which the neural and hemal spines are markedly reduced (, ). Reduction in size of neural spines in region 2 correlates with the position where pterygiophores of the second dorsal fin are placed dorsal to the vertebral column () (Davie Citation1990: fig. 6, page 9). The neural and hemal spines of vertebrae 19–20 have a knife-blade appearance (). Vertebra 21 bears spiniform neural and hemal spine in all species (, ) (Nakamura, Citation1955). With the exception of spearfishes, which have very elongated centra, the vertebrae from region 2 are similar in all species and only the extant K. albida differs from all others in that a large spinal foramen is placed ventrally to vertebra 21 ().
The third region includes the posterior-most four vertebrae (21–24), which are strongly modified forming the caudal peduncle (). Each vertebra from this region has a distinctive shape and its exact position thus can be established beyond doubt (). Previous studies show that species can be differentiated using measurements of isolated vertebrae from the caudal peduncle (Fierstine Citation2001). We tested that here, but the only differences detected were the larger sizes of vertebrae in M. nigricans and Is. indica, and that K. audax has more elongated vertebral centra and its vertebra 22 is proportionally shorter (). Other species did not show any grouping in the PCA results (). Measured variables from isolated vertebrae of the caudal peduncle could best discriminate marlins by size (larger blue and black marlins) (). However, non-measured attributes such as the shape of the neural and hemal spines and the hypural plate, are useful to make specific taxonomic identifications ().
Fossil Vertebrae
The Gatun Vertebra—The Gatun vertebra displays a lateral fracture in the anterior portion indicating that there was a lateral, now broken off apophysis (). The large size of the vertebra and the presence of expanded lateral apophyses (, ) are traits observed in the extant blue and black marlins (Nakamura, Citation1983, Citation1985; Davie, Citation1990). The oval shape of the hemal spine of the vertebra 14 (, ), the neural postzygapophysis with the spur arising backward and the anterior outline of the centrum very close to the hemal spine forming an elongated and slim intervertebral arc () are traits typically found in the blue marlin (, ). The Gatun vertebra is very similar to those found in the extant Makaira nigricans () and its most remarkable trait is the estimated body size of the specimen, which is ca. 518.16 cm. This size is larger than the maximum total length observed for any extant marlin (Nakamura, Citation1985; Allen and Steene, Citation1988; Randall, Citation1995; IGFA, Citation2001). In Makaira nigricans the maximum total body length is 500 cm, with ca. 290–350 cm being the common length in the Pacific and Atlantic oceans, respectively (Allen and Steene, Citation1988; Randall, Citation1995; IGFA, Citation2001). There are two fossil specimens in Panama that can be related to the Gatun vertebra: Makaira panamensis from the Chagres Fm. and Makaira cf. M. nigricans from the Gatun Fm. (Fierstine, Citation1978, Citation1999a). Although both fossils represent very large specimens, they do not preserve associated vertebrae and its body size still cannot be calculated.
The Río Indio Vertebrae—They consist of two articulated vertebrae that lack the lateral apophyses and which are smaller than the Gatun vertebra (, ). The vertebrae preserve the hemal prezygapophyses interlocked with the base of the hemal spine, which results in an elongated intervertebral arc () (Nakamura, Citation1955; Davie, Citation1990). In extant species, the shape of this intervertebral arc changes along the vertebral column (). From the first caudal vertebra up to position 17, the intervertebral arc is larger and elongated, while it closes progressively in posterior to position 17 (). Based on the close shape of the intervertebral arc, the Río Indio vertebrae are assigned to region 1 and their morphology indicates that they represent vertebrae of between positions 15 and 17 (, , ). The lack of well-preserved neural and hemal spines renders any generic level determination difficult, but lack of lateral apophyses occurs in Istiophorus, Kajikia, and Tetrapturus (–2) (Nakamura et al. Citation1968; Nakamura Citation1983, Citation1985; Davie Citation1990). Any relationship of the Río Indio vertebrae with Tetrapturus is discarded here, because members of this genus have considerably smaller vertebrae with lower and more elongated vertebral centra (, ; , ) that hardly can be confused with other genera (Nakamura, Citation1983, Citation1985). Six out of 12 ratios of the Río Indio vertebrae established here are similar to values found in I. platypterus, while two out of 12 ratios are similar to those observed in K. audax and K. albida, respectively (). The shape of the intervertebral arc resembles that found in members of Istiophorus (, , ). However, the Río Indio vertebrae possess a dorsoventrally larger postzygapophysis with a spur originating anterior to the posterior end of the centrum (), which is more similar to the condition found in members of Kajikia ssp. () than of Istiophorus () (Gottfried, Citation1982).
The Río Indio vertebrae were found associated with a wide dentary (Table S4, ). Previous works indicate that two variables measured from the dentary () have taxonomic significance (Fierstine, Citation2001). However, we found that only one of these variables has taxonomic importance (Table S4, ). The use of ratios between both variables (e.g., DJL = 40.46 mm, dad = 21.84 mm, dad/djl = 0.53) is useful to understand shape changes of the dentary in extant species (, ). The Río Indio dentary bone has a distal tip that is large and quadrangular (). The corresponding angulo-articular also is large (). The shape and size of the dentary and the angulo-articular indicates that the Río Indio specimen had a wide lower jaw similar to those observed in M. nigricans and Is. indica (, ). The combination of a wide lower jaw and absent lateral vertebral apophyses is recognized here for the first time in istiophorids (). Wide lower jaws and developed lateral apophyses in the caudal vertebrae, inter alia, characterize some extant marlins (Nakamura, Citation1983, Citation1985; Habegger et al., Citation2017). The height differences of the lower jaws as shown in are related to the magnitude of the bite force (ABF) and body size in extant species (Nakamura, Citation1983, Citation1985; Habegger et al., Citation2017).
For example, larger dentaries in a robust lower jaw produce higher ABF and are associated with larger body sizes as observed in M. nigricans and Is. indica (Nakamura, Citation1983, Citation1985; Fierstine, Citation2001; Habegger et al., Citation2017). Instead, more slender lower jaws produce lower ABF and are associated with smaller body sizes as in K. albida and I. platypterus (Nakamura, Citation1985; Habegger et al., Citation2017). The exclusive association of larger body size, wide lower jaw and lateral apophyses in caudal vertebrae for extant species (Nakamura et al., Citation1968; Nakamura, Citation1983, Citation1985) shows that the Río Indio specimen could represent a transitional form as indicated by undeveloped lateral apophyses combined with robust lower jaws (). This also indicates that body size represents an important trait in istiophorids as already pointed out (Santini and Sorenson, Citation2013).
Significance of the Lateral Apophysis
Extinct billfish such as members of the Hemingwayidae, Paleorhynchidae, and Blochiidae and new istiophorid specimens from the early Miocene of Austria that currently are under review by the authors, lack the lateral apophyses indicating that the absence of this structure is the plesiomorphic condition (Fierstine and Monsch, Citation2002; Fierstine, Citation2006). The lateral apophyses observed in the Gatun vertebra form a specialized structure for attachment of the posterior oblique tendons that connect the epaxial, hypaxial, and caudal peduncle musculature in extant species (Davie, Citation1990; Hebrank et al., Citation1990). The function of the lateral apophyses is to provide extra flexion and propulsion, because of the attached tendons supporting the caudal musculature and caudal peduncle (Fierstine and Walters, Citation1968; Davie, Citation1990). The lateral apophyses are only observed in larger extant istiophorids (Nakamura et al., Citation1968; Nakamura, Citation1983, Citation1985; Davie, Citation1990) and the large estimated body size for the Gatun specimen (>500 cm) indicates that the pattern is similar in extinct forms (). The observations made in the Gatun and extant specimens shows that the lateral apophyses combined with large quadrangular spines in the caudal vertebrae are adaptations directly associated with the giant body sizes as it provides extra skeletal attachment area for additional muscle fibers (Fierstine and Walters, Citation1968; Davie, Citation1990; Hebrank et al., Citation1990). In contrast, marlins with body size below 400 cm such as I. platypterus, Kajikia ssp., and Tetrapturus ssp. lack the lateral apophyses and possess oval to slim transversal body shapes indicating that they have less axial musculature (Nakamura, Citation1983, Citation1985; Davie, Citation1990; Hebrank et al., Citation1990). In larger marlin species, the muscles used for propulsion supported by the lateral apophyses make up the largest mass of muscles, involving about 57–65% of the total body weight (Davie, Citation1990). Published molecular evidence indicates that gigantism in Makaira and Istiompax evolved convergently and that the genus Makaira represents an evolutionarily older lineage than Istiompax (Graves and McDowell, Citation1995; Santini and Sorenson, Citation2013). The corresponding time-calibrated phylogenies are congruent with the fossil record because the genus Makaira is known since the late Miocene while the record of Istiompax extends only back into the Pliocene (Fierstine, Citation1978, Citation2001, Citation2006). This fossil evidence presented here as well as published phylogenies for extant species suggest that the lateral apophysis is a synapomorphy associated with the extreme gigantism in istiophorids () (Fierstine and Monsch, Citation2002; Santini and Sorenson, Citation2013).
The Río Indio vertebrae represent a smaller individual with morphological and body size similarities to the extant I. platypterus and Kajikia ssp. (Nakamura, Citation1983, Citation1985). The Río Indio specimen displays an unusual combination of traits such as a more quadrangular interdentary joint combined with caudal vertebrae lacking lateral apophyses (). In extant species, a quadrangular interdentary joint is only found in larger blue and black marlins, which also possess caudal vertebrae with lateral apophyses (Nakamura, Citation1983, Citation1985). Published phylogenies indicate that radiation of extant marlins, sailfishes, and spearfishes started during the Early Miocene (ca. 18 Ma) with most of the species having split from their relatives since the Pliocene (ca. 5 Ma) (Santini and Sorenson, Citation2013). Our findings shows that the morphological traits in the vertebral column that characterize extant I. platypterus, Kajikia ssp., and Tetrapturus ssp. (Nakamura et al., Citation1968; Gottfried, Citation1982; Nakamura, Citation1983, Citation1985; Davie, Citation1990) already existed at least since the early Miocene (, ). The morphological evidence from extant and fossil istiophorids indicates that traits such as 13 caudal vertebrae, lateral apophyses, robust lower jaws, and large neural and hemal spines are characters associated with marlin species having body sizes over 400 cm (Nakamura, Citation1983, Citation1985; Davie Citation1990). Our observations support the idea that body size plays an important role in the evolutionary history of istiophorid billfishes, as has been pointed out by a time-calibrated molecular hypothesis, but detailed studies using phylogenies based on combined morphological and molecular data and including living and extinct species still are needed (Santini and Sorenson Citation2013).
CONCLUSIONS
The combination of traits observed in the Río Indio vertebrae indicate that these remains represent an undescribed taxon, but the isolated vertebrae do not provide sufficient information for any accurate description and establishment of a new taxon (). These vertebrae and their associated bones demonstrate that the paleodiversity of istiophorids includes transitional forms () that need to be analyzed in the near future with well-preserved and more complete fossil specimens. We hypothesize that the morphological changes that led to the radiation of istiophorids include evolutionary innovations such as addition of one caudal vertebra and the development of lateral apophyses representing phylogenetic gains. However, a reduced neural spine, narrowing of the lower jaw, and body size reduction seem to represent pleiomorphic traits as evidenced by some species of the extinct billfish families. Phylogenies based on morphological traits and a comprehensive understanding of the fossil record, however, are of utmost importance in order to reconstruct the evolutionary scenario of istiophorid billfishes (see also Nakamura, Citation1983, Citation1985; Davie, Citation1990; Santini and Sorenson, Citation2013).
Supplemental Material
Download Zip (40.5 KB)ACKNOWLEDGMENTS
This study resulted in part from a project, which is funded by the program for young researchers (Grant No. APY–NI10–016A) and the Doctoral and Postdoctoral Scholarships Program of the National Secretary of Science and Technology of Panama (Grant No. BIP–2018–004) as well as the Ricardo Perez S.A and STRI-Tupper Paleontological Fund. Our thanks also go to the Dirección de Recursos Minerales of Panamá for collection permits. We acknowledge the CTPA–STRI paleontological crew for their support during fieldwork, J. Aleman for photography of fossil specimens, and A. Aguirre for help to find rare bibliographic material. We are grateful to J. Clayton, J. Williams, and M. Brett-Surman from the USNM for granting access to the osteological and paleontological collections under their care. Thanks also to P. Béarez from the MNHN and R. Cooke from STRI for access to osteological material of I. platypterus; to M. McGrouther and A. Hay from the AMNH for their valuable help in providing a complete specimen of Is. Indica; to A. Palandačić from the NHMW to grant access to a mounted skeleton of T. belone; and to A. Osorio, J. Martinez, and J. Lezcano for their help during fieldwork in Miguel de la Borda. We dedicate this work to the late Harry Fierstine (1932–2021), who generously shared unpublished data as well his experience and knowledge about istiophorid morphology during the earliest years of the academic formation of C.D., which made this study possible. He will be missed.
LITERATURE CITED
- Allen, G. R., and R. C. Steene. 1988. Fishes of Christmas Island Indian Ocean. 197 pp.
- Bernard, A. M., M. S. Shivji, R. R. Domingues, F. H. V. Hazin, A. F. de Amorim, A. Domingo, F. Arocha, E. D. Prince, J. P. Hoolihan, and A. W. S. Hilsdorf. 2013. Broad geographic distribution of roundscale spearfish (Tetrapturus georgii) (Teleostei, Istiophoridae) in the Atlantic revealed by DNA analysis: implications for white marlin and roundscale spearfish management. Fisheries Research 139:93–97.
- Betancur-R., R., R. E. Broughton, E. O. Wiley, K. Carpenter, J. A. López, C. Li, N. I. Holcroft, D. Arcila, M. Sanciangco, J. C. Cureton II, F. Zhang, T. Buser, M. A. Campbell, J. A. Ballesteros, A. Roa-Varon, S. Willis, W. C. Borden, T. Rowley, P. C. Reneau, D. J. Hough, G. Lu, T. Grande, G. Arratia, and G. Ortí. 2013. The Tree of Life and a New Classification of Bony Fishes. PLOS Currents 5. Available at 10.1371/currents.tol.53ba26640df0ccaee75bb165c8c26288. Accessed April 1, 2021.
- Carnevale, G., and S. J. Godfrey. 2018. Miocene bony fishes of the Calvert, Choptank, St. Marys and Eastover Formations, Chesapeake Group, Maryland and Virginia; pp. 161–198 in S. J. Godfrey (ed.), The Geology and Vertebrate Paleontology of Calvert Cliffs, Maryland, USA. Smithsonian Contributions to Paleobiology 100.
- Carnevale, G., C. Sorbini, W. Landini, and A. Varola. 2002. Makaira cf. M. nigricans Lacépède, 1802 (Teleostei: Perciformes: Istiophoridae) from the Pietra Leccese, late Miocene, Apulia, southern Italy. Palaeontographia Italica 88:63–75.
- Coates, A. G. 1999. Lithostratigraphy of the Neogene strata of the Caribbean coast from Limon, Costa Rica, to Colon; pp. 17–38. In L. S. Collins and A. G. Coates (eds.), A Paleobiotic Survey of the Caribbean Faunas from the Neogene of the Isthmus of Panama. Bulletins of American Paleontology 357.
- Coates, A. G., J. B. C. Jackson, L. S. Collins, T. M. Cronin, H. S. Dowsett, L. M. Bybell, P. Jung and J. Obando. 1992. Closure of the Isthmus of Panama: the Near-Shore Marine Record of Costa Rica and Western Panama. Geological Society of America Bulletin 104:814–828.
- Collette, B. B., J. R. McDowell, and J. E. Graves. 2006. Phylogeny of recent billfishes (Xiphioidei). Bulletin of Marine Science 79:455–468.
- Collins, L. S. 1999. The Miocene to recent diversity of Caribbean benthic foraminifera from the Central American Isthmus. Bulletins of American Paleontology. 357:91–105.
- Collins, L. S., A. G. Coates, W. A. Berggren, M.-P. Aubry, and J. Zhang. 1996. The late Miocene Panama isthmian strait. Geology 24:687–690.
- Davie, P. S. 1990. Pacific Marlins: Anatomy and Physiology. Simon Print, Palmerston North, New Zealand, 88 pp.
- Fierstine, H. L. 1974. The paleontology of billfish - the state of the art; pp. 34–44 in R. S. Shomura and F. Williams (eds.), Part 2. Review and Contributed Papers: Proceedings of the International Billfish Symposium, Kailua-Kona, Hawaii, 9–12 august 1972. Part 2. Contributed papers. United States Department of Commerce, NOAA Technical Report NMFS SSRF-675.
- Fierstine, H. L. 1978. A new marlin, Makaira panamensis, from the late Miocene of Panama. Copeia 1978:1–11.
- Fierstine, H. L. 1990. A paleontological review of three billfish families (Istiophoridae, Xiphiidae, and Xiphiorhynchidae); pp. 11–19 in R. H., Stroud (ed.), Planning the Future of Billfishes. Research and Management in the 90s and Beyond. National Coalition for Marine Conservation, Savannah, Georgia.
- Fierstine, H. L. 1998. Makaira sp., cf. M. nigricans Lacépède, 1802 (Teleostei: Perciformes: Istiophoridae) from the Eastover Formation, late Miocene, Virginia, and a reexamination of †Istiophorus calvertensis Berry, 1917. Journal of Vertebrate Paleontology 18:30–42.
- Fierstine, H. L. 1999a. Makaira sp., cf. M. nigricans Lacépède, 1802 (Teleostei: Perciformes: Istiophoridae) from the late Miocene, Panama, and its probable use of the Panama seaway. Journal of Vertebrate Paleontology 19:430–437.
- Fierstine, H. L. 1999b. A new shortbilled marlin of the genus Makaira, from the Yorktown Formation, (Early Pliocene), eastern North Carolina at Lee Creek Mine, USA. Tertiary Research 19:71–77.
- Fierstine, H. L. 2001. Analysis and new records of billfish (Teleostei: Perciformes: Istiophoridae) from the Yorktown Formation; pp. 21–69 in C. E. Ray and Bohaska, D. J. (eds), Geology and Paleontology of the Lee Creek Mine, North Carolina, III. Smithsonian Contributions to Paleobiology 90.
- Fierstine, H. L. 2006. Fossil history of billfishes (Xiphioidei). Bulletin of Marine Science 79:433–453.
- Fierstine, H. L., and S. P. Applegate. 1968. Billfish remains from southern California with remarks on the importance of the predentary bone. Bulletin of the Southern California Academy of Sciences 67:29–39.
- Fierstine, H. L. And K. A. Monsch. 2002. Redescription and phylogenetic relationships of the family Blochiidae (Perciformes: Scombroidei), middle Eocene, monte Bolca, italy. Studi e Ricerche sui Giacimenti Terziari di Bolca, Museo Civico di Storia Naturale di Verona 9: 121–163.
- Fierstine, H. L., and N. L. Voigt. 1996. Use of rostral characters for identifying adult billfishes (Teleostei: Perciformes: Istiophoridae and Xiphiidae). Copeia 1996:148–161.
- Fierstine, H. L., and V. Walters. 1968. Studies in locomotion and anatomy of scombroid fishes. Memoirs of the Southern California Academy of Sciences 6:1–31.
- Fierstine, H. L., and B. J. Welton. 1983. A black marlin, Makaira indica, from the Early Pleistocene of the Philippines and the zoogeography of istiophorids billfishes. Bulletin of Marine Science 33:718–728.
- Fierstine, H. L., and B. J. Welton. 1988. A late Miocene marlin, Makaira sp. (Perciformes, Osteichthyes) from San Diego County, California, USA. Tertiary Research 10:13–19.
- Fierstine, H. L., S. P. Applegate, G. Gonzalez-Barba, T. Schwennicke, and L. Espinosa-Arrubarrena. 2001. A fossil blue marlin (Makaira nigricans Lacépède) from the middle facies of the Trinidad Formation (upper Miocene to upper Pliocene), San Jose del Cabo Basin, Baja California Sur, Mexico. Bulletin of the Southern California Academy of Sciences 100:59–73.
- Gottfried, M. D. 1982. A Pliocene sailfish Istiophorus platypterus (Shaw and Nodder, 1791) from Southern California. Journal of Vertebrate Paleontology 2:151–153.
- Graves, J. E., and J. R. McDowell. 1995. Inter-ocean genetic divergence of istiophorid billfishes. Marine Biology 122:193–203.
- Graves, J. E., and J. R. McDowell. 2012. Inter-annual variability in the proportion of roundscale spearfish (Tetrapturus georgii) and white marlin (Kajikia albida) in the western North Atlantic Ocean. Collective Volume of Scientific Papers ICCAT 68:1543–1547.
- Graves, J. E., and J. R. McDowell. 2015. Population structure of istiophorid billfishes. Fisheries Research 166:21–28.
- Greenwood, P. H., D. E. Rosen, S. H. Weitzman, and G. S. Myers. 1966. Phyletic studies of teleostean fishes, with a provisional classification of living forms. Bulletin of American Museum of Natural History 131:341–345.
- Gregory, W. K., and G. M. Conrad. 1937. The comparative osteology of the swordfish (Xiphias) and the sailfish (Istiophorus). American Museum Novitates 952:1–25.
- Habegger, M. L., D. H. Huber, M. J. Lajeunesse, and P. J. Motta. 2017. Theoretical calculations of bite force in billfishes. Journal of Zoology 303:15–26.
- Hanner, R., R. Floyd, A. Bernard, B. B. Collette, and M. S. Shivji. 2011. DNA barcoding of billfishes. Mitochondrial DNA 22:27–36.
- Hebrank, J. H., M. R. Hebrank, J. H. Jr. Long, B. Block, and S. A. Wainwright. 1990. Backbone mechanics of the blue marlin Makaira nigricans (Pisces, Istiophoridae). Journal of Experimental Biology 148:449–459.
- Hendy, A. J. W. 2013. Spatial and stratigraphic variation of marine paleoenvironments in the middle-upper Miocene Gatun Formation, Isthmus of Panama. Palaios 28:210–227.
- Hoolihan, J. P., J. Premanandh, M.-A. D’Aloia-Palmieri, and J. A. H. Benzie. 2004. Intraspecific phylogeographic isolation of Arabian Gulf sailfish Istiophorus platypterus inferred from mitochondrial DNA. Marine Biology 145:465–475.
- IGFA. 2001. Database of International Game Fish Association. IGFA, Fort Lauderdale, Florida, United States of America. Available at: www.igfa.org. Accessed July 10, 2021.
- Johnson, D. G., and C. Patterson. 1993. Percomorph phylogeny: a survey of acanthomorphs and a new proposal. Bulletin of Marine Science 52:554–626.
- Kassambara, A. 2017. Practical Guide to Principal Component Methods in R: PCA, M (CA), FAMD, MFA, HCPC, Factoextra (vol 2). Sthda. Available at: www.sthda.com. Accessed August 30, 2020.
- Kassambara, A., and F. Mundt. 2017. Factoextra: extract and visualize the results of multivariate data analyses. R Package Version 1:337–354.
- Lácépede, B. 1802. Histoire Naturelle des Poissons, vol 4. Chez Plassan, Paris, xliv + 728 pp.
- Lériche, M. 1926. Famile des Xiphiidae; pp. 443–446 in M. Lériche (ed.). Les Poissons Néogènes de Ia Belgique. Bulletin de La Société Belge de Géologie, de Paléontologie et d’Hydrologie, Procés-Verbaux 32.
- Little, A. G., S. C. Lougheed, and C. D. Moyes. 2010. Evolutionary affinity of billfishes (Xiphiidae and Istiophoridae) and flatfishes (Plueronectiformes): independent and trans-subordinal origins of endothermy in teleost fishes. Molecular Phylogenetics and Evolution 56:897–904.
- Morrow, J. E., and G. S. Posner. 1957. On the morphology of the pectoral girdle in the genus Makaira. Bulletin of the Bingham Oceanographic Collection, Peabody Museum of Natural History, Yale University 26:88–105.
- Müller, J. 1845. Über den Bau und die Grenzen der Ganoiden und über das natürliche System der Fische. Physikalische Und Mathematische Abhandlungen der Königlichen Akademie Der Wissenschaften in Berlin 1:117–216.
- Nakamura, H. 1955. Report of an Investigation of the Spearfishes of Formosan Waters. Special Scientific Report Fisheries No. 153. US Department of the Interior, Fish and Wildlife Service, Washington, 46 pp.
- Nakamura, I. 1983. Systematics of the Billfishes (Xiphiidae and Istiophoridae). Seto Marine Biological Laboratory, Kyoto, 396 pp.
- Nakamura, I. 1985. FAO Species Catalogue Vol. 5. Billfishes of the World. An Annotated and Illustrated Catalogue of Marlins, Sailfishes, Spearfishes and Swordfishes Known to Date. FAO Fish Biological Synopses 125, Vol. 5. University of Kyoto Mauzuru, Kyoto, 65 pp.
- Nakamura, I., T. Iwai, and K. Matsubara. 1968. A Review of the Sailfish, Spearfish, Marlin and Swordfish of the World. Misaki marine biological Institute, Kyoto University, Kyoto, 95 pp.
- Nelson, J. S., T. C. Grande, and M. V. H. Wilson. 2016. Fishes of the World, 4th ed. John Wiley and Sons Inc., Hoboken, New Jersey, 624 pp.
- Pepperell, J. G. 2010. Fishes of the Open Ocean: A Natural History and Illustrated Guide. University of Chicago Press, Chicago, 272 pp.
- R Core Team. 2020. R: A Language and Environment for Statistical Computing. R Foundation for Statistical Computing, Vienna, Austria. Available at: https://www.R-project.org/. Accessed August 30, 2020.
- Randall, J. E. 1995. Coastal Fishes of Oman. University of Hawaii Press, Hawaii, 439 pp.
- Regan, C. T. 1923. The skeleton of Lepidosteus, with remarks on the origin and evolution of the lower neopterygian fishes. Proceedings of the Zoological Society of London 93:445–461.
- Robins, C. R., and D. P. de Sylva. 1960. Description and relationships of the longbill spearfish, Tetrapturus belone, based on western North Atlantic specimens. Bulletin of Marine Science 10:383–413.
- Rojo, A. L. 2017. Dictionary of Evolutionary Fish Osteology. CRC Press, Boca Raton, Florida, 282 pp.
- Rosen, D. E. 1973. Interrelationships of Higher Euteleostean Fishes; pp. 397–513 in P. H. Greenwood, R. S. Miles, and C. Patterson (eds.), Interrelationships of fishes. Academic Press, London.
- Santini, F., and L. Sorenson. 2013. First molecular timetree of billfishes (Istiophoriformes: Acanthomorpha) shows a late Miocene radiation of marlins and allies. Italian Journal of Zoology 80:481–489.
- Williams, S. M., J. R. McDowell, M. Bennett, J. E. Graves, and J. R. Ovenden. 2018. Analysis of whole mitochondrial genome sequences increases phylogenetic resolution of istiophorid billfishes. Bulletin of Marine Science 94:73–84.