ABSTRACT
The reflected lamina of the angular has long been portrayed as a key component in understanding the evolution of the mammalian mandible and middle ear, yet the function of this structure in non-mammalian therapsids remains uncertain. We undertake a broad scale survey of two aspects of the reflected lamina that have been poorly characterized: the ridges and fossae on its lateral surface and the extent of the underlying angular cleft. These two features were visualized in a wide range of therapsids using CT data from the literature and blue light surface scanning. Most species exhibit a clade-specific pattern of surface topography, although dicynodonts are highly variable. A striking similarity in the general number and orientation of fossae and ridges is seen between the otherwise disparate therapsid clades, and we propose homologies in these surface features across Therapsida. These features may serve as useful phylogenetic characters in analyses of the relationships among the major therapsid clades. The angular cleft does not underlie all of what is often referred to as the ‘reflected lamina’ in the literature, and an updated definition of this term is provided. Several therapsid groups independently expanded the angular cleft to further separate the reflected lamina from the angular body. We discuss some functional consequences of our findings and note inconsistency between proposed clade-specific muscle attachment sites and the presence of homologous fossae and ridges across much of Therapsida.
INTRODUCTION
Some of the most dramatic morphological changes that occurred during the evolution of synapsids were the transformations of the mandible: the coronoid eminence heightened into a tall coronoid process, the quadrate-articular jaw joint was replaced by a dentary-squamosal joint, muscle insertions on the postdentary bones shifted onto the dentary, and the postdentary bones completely separated from the dentary to become incorporated into the mammalian middle ear (Crompton, Citation1963; Barghusen, Citation1968; Kermack et al., Citation1973; Meng et al., Citation2018). These changes allowed synapsids to process food more efficiently, which was crucial to support the high-energy lifestyle that eventually became a hallmark feature of mammals (Crompton and Parker, Citation1978; Farmer, Citation2000; Kemp, Citation2007; Hopson, Citation2012). Because of its importance in understanding the assembly of the mammalian body plan, and therefore major evolutionary transitions and the origin of higher taxa, the functional morphology of the synapsid mandible has received detailed attention for over a century (Watson, Citation1912; Adams, Citation1919; Crompton, Citation1963, Citation1972; DeMar and Barghusen, Citation1972; Kermack et al., Citation1973; Bramble, Citation1978; King et al., Citation1989; Lautenschlager et al., Citation2017). Despite this extensive research, the function of the reflected lamina of the angular in the mandible of non-mammalian therapsids remains a glaring paleobiological mystery.
The reflected lamina of the angular is a plate of bone separated medially from the body of the angular by a thin space called the angular cleft. This structure is found in sphenacodontids and therapsids, and embryological studies and the fossil record clearly document its homology with the ectotympanic bone of mammals (Palmer, Citation1913). In most non-eucynodont therapsids, the surface of the reflected lamina features a radiating pattern of ridges and fossae. Despite its distinctiveness and potential importance for the study of the mammalian feeding system and middle ear, the function of the reflected lamina, including its surface topography and associated angular cleft, has never been decisively determined.
Several functions have been proposed for this suite of structures. Some have suggested that the angular cleft may have housed a gland (Broom, Citation1932; Watson, Citation1953), but glands rarely occupy bony recesses and likely would not require a dedicated space in which to reside (Janensch, Citation1952; Allin, Citation1975). Several authors have placed an insertion for the pterygoideus (Romer and Price, Citation1940; Watson, Citation1953; Barghusen, Citation1968) or pseudotemporalis (Westoll, Citation1943) muscles within the angular cleft, supposing that these muscles wrapped around the ventral edge of the mandible to reach insertion points on the ventrolateral surface of the angular body, as in the pterygoideus of extant reptiles. Others have placed attachments for various jaw adductor or throat muscles in the fossae on the lateral face of the reflected lamina (Janensch, Citation1952; Barghusen, Citation1968; Kemp, Citation1969, Citation1982; King, Citation1981). The alternating ridges and fossae have also been proposed to strengthen the reflected lamina against muscle strain (Parrington, Citation1955). The angular cleft could represent an air-filled diverticulum of the pharynx that may have been used as a resonating chamber for vocalization (Westoll, Citation1943). A widely accepted idea is that the reflected lamina of non-mammalian therapsids had some role in a rudimentary airborne- or substrate-borne sound reception system involving the postdentary bones, quadrate, and stapes (Allin, Citation1975; Allin and Hopson, Citation1992; Laurin, Citation1998; Laaß, Citation2016). If the reflected lamina was indeed involved in sound reception in early therapsids, then the evolution of the mammalian middle ear was likely the consequence of sustained selection for improved hearing ability rather than simply a side effect of the appearance of the dentary-squamosal joint (Allin, Citation1975; Crompton and Parker, Citation1978). Assessing function in the fossil record is notoriously difficult and, unfortunately, there is little direct evidence supportive of one hypothesis for the function of the angular complex in non-mammalian therapsids.
It is especially difficult to assess the competing hypotheses for the function of the reflected lamina because potentially important systematic variation in this structure remains poorly documented. The external topography of the angular is rarely described in detail in the literature, and there are likely several reasons for this. First, because the reflected lamina is extremely fragile, with sub-millimeter thickness in many specimens, it is often absent or heavily damaged, especially in historic specimens that have not benefitted from modern preparation techniques. The rarity of specimens with a relatively complete, undeformed reflected lamina hinders comparative descriptions of the structure. A second potential reason for the lack of detail in descriptions of the reflected lamina is that its surface topography is highly stereotyped within some clades, meaning that researchers rarely feel the need to do more than affirm that a newly described fossil conforms to the expected morphology of its clade. Descriptions of these stereotyped arrangements have been made for a few clades, which we review below, but comparisons between clades have not been made because the patterns of ridges and fossae in each clade are often so complex and different that it is difficult to recognize homologous features that could be reasonably compared. The angular cleft is also rarely described in detail, as it is largely obscured by the reflected lamina laterally. Intricate fossil preparation can occasionally reveal the morphology of the angular cleft, but in many cases the cleft is deep and thin enough that it can only be visualized by serial sectioning or computed tomographic (CT) scanning. Serial sections have occasionally been used to describe the angular cleft, but the coarse level of detail that can be reasonably obtained by this method makes it difficult to accurately map the shape of this space (Maier and van den Heever, Citation2002). As a result, it is unclear how much of what has been called the ‘reflected lamina’ in the literature is actually separated from the angular body. Further, substantive comparisons of angular cleft morphology have not been made between therapsid clades, which further hinders our understanding of how this feature evolved and its potential function. A broad understanding of morphological variation and homology of the angular complex requires surveying a large number of specimens and making comparisons across a wide taxonomic sample, which has not been undertaken across all of non-mammalian Synapsida.
Here we describe in detail the surface features on the angular across non-mammalian synapsids and make comparisons between the major therapsid clades in order to identify potential homologies and provide a framework to examine the evolution of the reflected lamina. The features we identify may also prove useful in future phylogenetic analyses of therapsids, which historically have suffered from a dearth of characters that can be applied across the highly distinct clades (Hopson and Barghusen, Citation1986; Sidor and Hopson, Citation1998; Rubidge and Sidor, Citation2001; Kemp, Citation2009). We propose a set of anatomical terms to help identify homologous features on the reflected lamina, which should facilitate informative descriptions of angular topography in the future. In addition, we use CT data to map the extent of the angular cleft in several therapsids to clarify how much of what has been called the ‘reflected lamina’ is indeed physically separated from the body of the angular. Finally, we discuss some implications of our findings for hypothesized functions of the angular complex.
Institutional Abbreviations—AMNH, American Museum of Natural History, New York, New York, U.S.A.; BP, Evolutionary Studies Institute, Johannesburg, South Africa; CGP, Council for Geosciences, Pretoria, South Africa; FMNH, Field Museum of Natural History, Chicago, Illinois, U.S.A.; MB.R., Museum für Naturkunde, Fossil Reptile Collection, Berlin, Germany; MCZ, Museum of Comparative Zoology, Harvard University, Cambridge, Massachusetts, U.S.A.; ML, Museu da Lourinhã, Lourinhã, Portugal; NHCC, National Heritage Conservation Commission, Lusaka, Zambia; NMQR, National Museum, Bloemfontein, South Africa; PIN, Paleontological Institute of the Russian Academy of Sciences, Moscow; SAM, Iziko Museums of South Africa, Cape Town, South Africa; TM, Ditsong Museum of Natural History, Pretoria, South Africa; UCMP, University of California Berkeley Museum of Paleontology, Berkeley, California, U.S.A.
MATERIALS AND METHODS
Specimens Examined—A total of 56 fossil synapsid genera were digitized for this study, spanning a wide phylogenetic and temporal range: three pelycosaur-grade genera, four biarmosuchians, six dinocephalians, 23 anomodonts, eight gorgonopsians, 14 therocephalians, and five non-mammalian cynodonts. Specimens that were figured or mentioned directly in the text are listed in . Additional specimens supporting the distribution of surface features described here are listed in Supplemental Data 1.
TABLE 1. Specimens figured and discussed in the text. Additional supporting specimens are listed in Supplementary Data 1.
Digitization Methods—Specimens across all major synapsid clades were surface scanned using an Artec Space Spider blue light scanner with a voxel size of 0.3 mm. The position of cracks, glue, matrix, etc. was noted for each fossil, and these extraneous objects were digitally removed using the smoothing functions in Meshmixer V3.5.474 (©Autodesk, San Rafael, CA, U.S.A.) to produce clean meshes of the mandible. The scans were also retrodeformed using algorithmic symmetrization in Landmark Editor V3.0 (©IDAV) to minimize the effects of taphonomic deformation on the orientation and position of relevant surface features (Wiley et al., Citation2005). The single axis algorithm was used, as this proved to be the most useful method for correcting deformation in the reflected lamina without introducing unrealistic distortion to the relatively flat surface.
The extent of the angular cleft was determined by segmenting CT scans of therapsid mandibles. Surface scans of specimens in which the angular cleft was fully prepared out were also used for this purpose. The CT data were previously published and made available by the authors of their respective studies (Castanhinha et al., Citation2013; Benoit et al., Citation2017; Bendel et al., Citation2018a; Pusch et al., Citation2019a; Huttenlocker and Sidor, Citation2020). The raw CT image stacks for Cynariops and Lycosuchus were downloaded from the Data Repository of the Museum für Naturkunde Berlin (Bendel et al., Citation2018b; Pusch et al., Citation2019b), and the raw image stack for Probainognathus was downloaded from DigiMorph.org. The part of the angular cleft that lies between the reflected lamina and the body of the angular was segmented in the CT scans using Avizo V9.2.0 (© FEI VSG, Hillsboro, OR, U.S.A.), as this portion was the easiest to delineate using surrounding anatomical structures and is the most relevant to visualizing the extent of the reflected lamina. For the surface scans, we manually built a mesh of the space between the reflected and medial laminae using the “draw” sculpting tool in Meshmixer.
DESCRIPTION
Anatomical Terms
We follow Allin’s (Citation1975) detailed terminology of the angular complex () and propose a series of new terms to describe the fossae on its external surface. The angular keel is a thin projection of the body of the angular extending ventral to the margin of the lower jaw. Posterodorsally, the keel is continuous with a sheet of bone that is mediolaterally separated from the body of the angular. This sheet of bone is the reflected lamina, and the portion of the angular body that lies directly medial to it is termed the medial lamina. At its dorsalmost contact with the angular body, the reflected lamina generally curves slightly posteriorly or dorsally, forming a dorsal notch. Posterior to the angular body is a curved rim of bone formed by the surangular and articular. The space between this rim and the posterior edge of the reflected lamina is called the angular gap, and the wide, gentle concavity on the angular body in this area is the external fossa of the angular. The angular cleft is made up by the angular gap and the space between the reflected and medial laminae, although our description focuses mainly on the latter portion (), as its bony medial and lateral boundaries provide ready landmarks during segmentation of the CT scans. Some therapsids also have an oval or triangular space between the dentary and the body of the angular called the external mandibular fenestra ().
FIGURE 1. Schematic therapsid lower jaw illustrating the terminology of the angular complex and other relevant structures (anterior is to the left). A, left mandible in lateral view with horizontal section taken between dotted lines; B, right mandible in medial view. Abbreviations: ab, body of the angular; ac, angular cleft; ag, angular gap; ak, angular keel; angf, angular fossa; cpe, coronoid process/eminence; d, dentary; dn, dorsal notch; emf, external mandibular fenestra; fo, fossa; ja, jaw articulation surface; ml, medial lamina of the angular; pra, prearticular; ri, ridge; rl, reflected lamina of the angular.
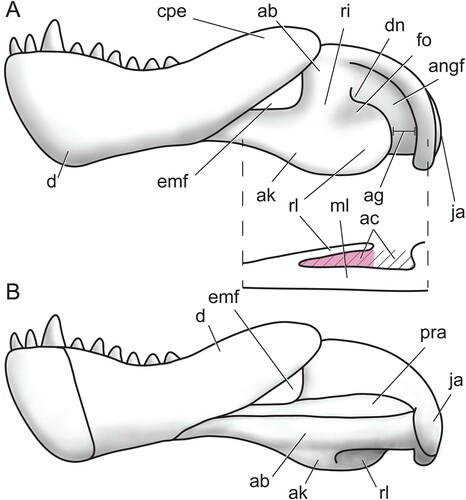
FIGURE 2. Morphological diversity of the therapsid mandible. A, photo of the biarmosuchian Biarmosuchus tener (PIN 1758/2); B, photo of the dinocephalian Syodon biarmicum (PIN 157/2); C, photo of the anomodont Emydops arctatus (BP/1/1307, mirrored); D, photo of the therocephalian Tetracynodon darti (SAM-PK-K10800); E, photo of the eucynodont Trirachodon berryi (BP/1/4658). Solid black lines mark the posterior edge of the preserved reflected lamina. Abbreviation: rl, reflected lamina of the angular. Scale bars equal 1 cm.
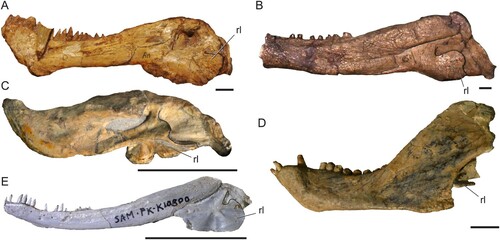
Systematic Review
Pelycosaur-Grade Synapsids—The lateral surface of the angular lacks ridges and fossae in all non-therapsid synapsids (). Moreover, in the earliest-diverging forms (e.g., caseasaurs, varanopids, ophiacodontids) the ventral surface of the angular is broadly rounded (Reisz, Citation1986). A subtle angular keel is first seen in edaphosaurids, and in later-diverging ‘haptodonts,’ the posterior edge of the keel becomes mediolaterally separated from the angular body, resulting in a reflected lamina of the angular that is separated from the body of the angular by a small angular cleft. The relationship of ‘haptodonts’ to other synapsids is unclear (Spindler, Citation2016; Angielczyk and Kammerer, Citation2018), but they are likely basal sphenacodontians (Huttenlocker et al., Citation2021). The reflected lamina of the angular is present in all members of Sphenacodontidae, the sister group of Therapsida (Reisz et al., Citation1992; Sidor and Hopson, Citation1998). In sphenacodontids, the angular keel is separated from the body of the angular only at its most posterior edge, and this emargination does not extend very far dorsally from the ventral margin of the jaw, so the effective reflected lamina is relatively small (). It should be noted that is based on a surface scan in which matrix in the angular cleft was fully prepared out, so the angular cleft may be underestimated in this figure. Still, the space is distinctly smaller than that seen in therapsids. The anterodorsal edge of the cleft forms a straight line extending anteroventrally to the ventral margin of the jaw. The posterior edge of the reflected lamina curves posterodorsally where it meets the angular body, forming a slight dorsal notch ().
FIGURE 3. Surface scan of the angular region in the sphenacodontid Dimetrodon limbatus (FMNH UC 1001) in left lateral view. Angular cleft overlain onto model. Thick dotted line indicates anterior edge of the ventrally hanging reflected lamina. Box on silhouette illustrates location of images. Abbreviations: ac, angular cleft; ak, angular keel; rl, reflected lamina. Scale bar equals 1 cm.
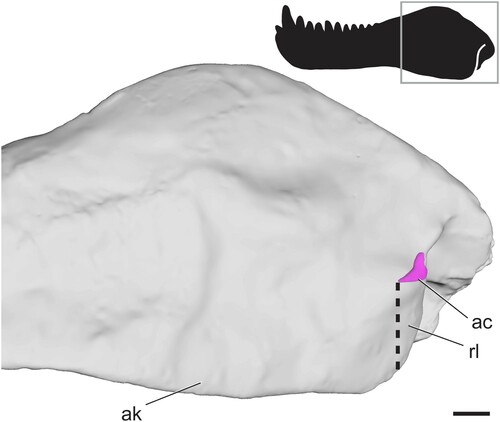
Biarmosuchia—The mandible is poorly known in biarmosuchians because specimens of these therapsids are relatively rare (Rubidge and Sidor, Citation2001; Sidor, Citation2015; Whitney and Sidor, Citation2016). Smith et al. (Citation2006) noted a similar overall pattern of radiating ridges and fossae in four biarmosuchian genera (Hipposaurus, Lemurosaurus, Lobalopex, and Paraburnetia), but in-depth comparisons of angular surface features have not yet been made for this group. Here, we recognize four fossae that are present in nearly identical positions and orientations in every biarmosuchian specimen with a well-preserved angular (). In lateral view, a horizontal ridge extending from the contact between the angular and dentary meets a near-vertical ridge near the middle of the angular. Together, these ridges meet at an approximately right angle to bound a triangular fossa facing anterodorsally from the center of the angular, here termed the anterodorsal fossa. The horizontal ridge also meets a posteroventrally oriented ridge at an oblique angle in the center of the angular, forming a wide, triangular fossa facing anteroventrally and spanning much of the ventral portion of the angular. This posteroventrally oriented ridge bounds a third fossa that faces posteriorly to posteroventrally. The shape of this third fossa is roughly triangular but varies in width among the species examined. In Bullacephalus, Paraburnetia, and Lemurosaurus, the posteroventral fossa is bordered dorsally by a wide, laterally convex area, dorsal to which is a small fourth fossa facing posterodorsally. This posterodorsal fossa has been figured in Biarmosuchus (; Sigogneau-Russell and Tchudinov, Citation1972), Proburnetia (Sigogneau-Russell, Citation1989; Rubidge and Sidor, Citation2002), Herpetoskylax (Sidor and Rubidge, Citation2006), and is possibly present in Lobalopex (Sidor et al., Citation2004). It is difficult to determine whether this fossa is present in Hipposaurus, as this area seems to be deformed in CGP/1/66, and the posterodorsal fossa is a subtle feature.
FIGURE 4. Surface and CT scans of the angular region in biarmosuchians. A, Hipposaurus sp. (CGP/1/66) in left lateral view; B, Paraburnetia sneeubergensis (SAM-PK-K10037) in left lateral view; C, Hipposaurus sp. (CGP/1/66) with angular cleft overlay; D, Paraburnetia sneeubergensis (SAM-PK-K10037) in ventral view showing anterior extent of the angular cleft. Box on silhouette illustrates location of images. Abbreviations: ac, angular cleft; adf, anterodorsal fossa; ak, angular keel; avf, anteroventral fossa; dn, dorsal notch; ml, medial lamina; pdf, posterodorsal fossa; pvf, posteroventral fossa; rl, reflected lamina; vcba, ventral connection to body of the angular. Scale bars equal 1 cm.
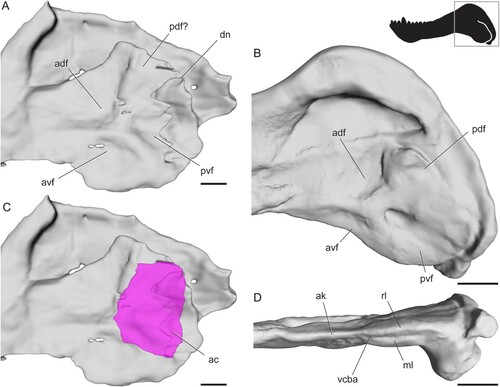
The extent of the angular cleft, and therefore the overlying reflected lamina, can be seen in . It is expanded beyond the condition seen in sphenacodontids, but it is still restricted to the posterior portion of the angular and does not underlie all of the fossae seen on the lateral surface of the angular. The dorsal notch is farther anterior than what is seen in sphenacodontids. The anterior extent of the angular cleft appears related to the position of the vertical ridge. In Hipposaurus, the cleft is deeply incised anteriorly into the angular, with the ventral contact between the reflected lamina and angular body located far posteriorly (). In contrast, Paraburnetia shows a ventral connection farther anterior, at about the level of the posteroventral ridge, meaning that the angular cleft opens ventrally as well as posteriorly in this specimen (). The discrepancy between the two specimens examined here likely indicates variation in the shape of the angular cleft among biarmosuchians, perhaps between basal members of the clade and its derived burnetiamorph subclade, although it should be noted that this morphology is somewhat unclear in the CT scan of CGP/1/66 and should be interpreted with caution.
FIGURE 5. Surface scans of the angular region in tapinocephalian dinocephalians. A, Moschops capensis (AMNH FR5550) in left lateral view; B, ‘Delphinognathus’ conocephalus (SAM-PK-713) in left lateral view; C, Moschops capensis (AMNH FR5550) in ventral view showing anterior extent of the angular cleft; D, ‘Delphinognathus’ conocephalus (SAM-PK-713) in ventral view showing anterior extent of the angular cleft. Box on silhouette illustrates location of images. Abbreviations: ak, angular keel; dn, dorsal notch; pdf, posterodorsal fossa; rl, reflected lamina; vcba, ventral connection to body of the angular. Scale bars equal 1 cm.
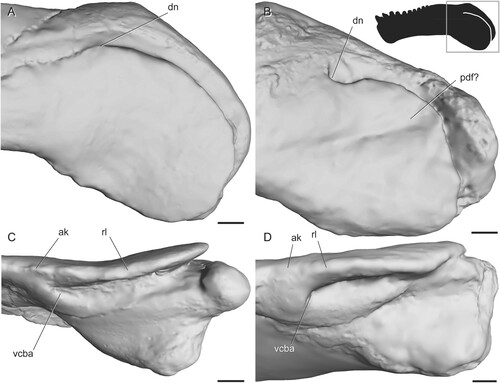
The CT data indicate that the ridges and fossae on the reflected lamina are made up of corrugations in the thin bone rather than differences in thickness across the structure, which agrees with observations of serial sections in other therapsids (Maier and van den Heever, Citation2002). However, ridges on the angular body are formed by thickening of the bone.
Dinocephalia—The reflected lamina is thicker relative to its surface area in dinocephalians than in most other therapsids, although it is often only a few millimeters thick. Most dinocephalians lack ridges and fossae on the reflected lamina itself, although some anteosaurs have a thick bony boss on the angular just anterior to the reflected lamina. The size and shape of this boss is highly variable between species (Kammerer, Citation2011). The only dinocephalian with evidence for surface features on the reflected lamina itself is the holotype of ‘Delphinognathus’ (SAM-PK-713), which has a posteriorly to posterodorsally facing groove. The surface of this specimen does not appear to be broken, worn, or warped, so this is likely a real feature.
Though we did not use CT data to determine the exact shape of the angular cleft, the extent of the cleft can be discerned from the dorsal and ventral connections between the reflected lamina and the angular body. In dinocephalians, both of these connection points are located far anteriorly, resulting in a large reflected lamina relative to the angular keel (). The ventral connection is at a level slightly anterior to that of the dorsal connection.
Anomodontia—Anomodonts evolved jaw morphologies that were highly disparate from those seen in other early therapsids or in mammals (Sidor, Citation2003) and, unsurprisingly, this diverse and geologically long-lived group had the highest variability in angular anatomy. Overall, the reflected lamina is relatively large in anomodonts, extending farther below the ventral margin of the jaw than in other therapsids. The dorsal notch is located anteriorly near the dentary, and a vertical ridge extends dorsally from this notch, sometimes reaching the surangular (Angielczyk et al., Citation2021b). The Triassic kannemeyeriids have often been described as having a uniquely large reflected lamina that nearly reaches the articular posteriorly (Janensch, Citation1952; Keyser, Citation1974; Keyser and Cruickshank, Citation1979), although we also observed this condition in some specimens of earlier-diverging dicynodonts such as Diictodon (BP/1/356).
In all anomodonts except the early-diverging Biseridens, there is an oval or triangular external mandibular fenestra between the dentary and the angular. Posterior and ventral to this fenestra are two ridges, one vertical and one horizontal, that meet at a right angle, enclosing an anterodorsal fossa at the posterior and ventral edges of the external mandibular fenestra (, ). Liu et al. (Citation2010) reported two fossae facing posteriorly on the reflected lamina of Biseridens, and published figures of Patranomodon also seem to show this arrangement (Rubidge and Hopson, Citation1996). No non-dicynodont anomodonts were examined in person for this study, but photographs of Ulemica clearly show at least two fossae in addition to the anterodorsal one: a broad, triangular fossa facing anteroventrally and a thinner fossa facing posteriorly (). The area around the dorsal notch appears to be broken in this specimen, but in Suminia (PIN 2212/62) there is a strong vertical ridge near the dorsal notch bordering a small fossa facing posterodorsally (). Other surface features cannot be discerned in PIN 2212/62, as the rest of the reflected lamina seems to be heavily deformed.
FIGURE 6. Surface scans and photos of the angular region in anomodonts showing surface features in left lateral view. A, photo of Ulemica invisa (PIN 157/1112); B, photo of Suminia getmanovi (PIN 2212/62); C, Eodicynodon oosthuizeni (BPI/1/6230); D, Pristerodon mackayi (BP/1/6685); E, Emydops arctatus (BP/1/1307); F, Kembawacela kitchingi (NHCC LB18); G, Cistecephalus microrhinus (BP/1/253); H, Tropidostoma microtrema (SAM-PK-8633); I, Lystrosaurus curvatus (BP/1/5086). Box on silhouette illustrates location of images. Areas filled with hashed lines indicate external mandibular fenestra. Solid, curved lines in C and G mark the posterior edge of the preserved reflected lamina. Photo in B courtesy of Brandon Peecook. Abbreviations: adf, anterodorsal fossa; af, anterior fossa; avf, anteroventral fossa; dn, dorsal notch; pdf, posterodorsal fossa; pvf, posteroventral fossa; vf, ventral fossa. Scale bars equal 1 cm.
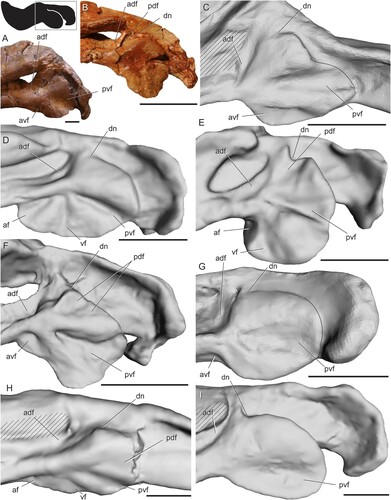
FIGURE 7. Surface and CT scans of the angular region in dicynodonts. A, Niassodon mfumukasi (ML1620); B, Endothiodon bathystoma (SAM-PK-K11031, right side mirrored) in lateral view; C, Niassodon mfumukasi (ML1620) with angular cleft overlay; D, Endothiodon bathystoma (SAM-PK-K11034) in ventral view showing anterior extent of the angular cleft; E, Stahleckeria potens (AMNH FR3857) in left lateral view; F, Stahleckeria potens (AMNH FR3857) with angular cleft overlay. Box on silhouette illustrates location of images. Thin dotted line indicates approximated minimum extent of missing reflected lamina in C and approximated minimum extent of unprepared angular cleft in F. Thick dotted line indicates anterior edge of the ventrally hanging reflected lamina. Abbreviations: ac, angular cleft; adf, anterodorsal fossa; ak, angular keel; avf, anteroventral fossa; dn, dorsal notch; pvf, posteroventral fossa; rl, reflected lamina; vcba, ventral connection to body of the angular. Scale bars equal 1 cm.
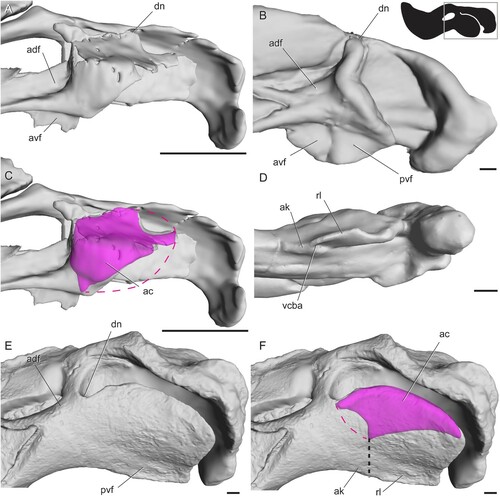
There is extensive variation in the surface features of the angular among species of basal dicynodonts, ranging from three to five fossae separated by radiating ridges. Despite this variation, the fossae and ridges tend to have similar positions among taxa. The ridges radiate from a point just anterior to the center of the angular in lateral view. In addition to the anterodorsal fossa described above, all dicynodonts have a fossa facing posteriorly to posteroventrally, which is bounded dorsally by a ridge extending roughly posteriorly (, ; Angielczyk and Rubidge, Citation2013; Angielczyk et al., Citation2016). In many dicynodonts, there is also a wide fossa facing anteroventrally just ventral to the horizontal ridge described above (, ). An additional ridge extending ventrally sometimes subdivides this fossa into two parts, a thin anterior component here termed the anterior fossa and a wider posterior component here termed the ventral fossa (). The anterior, ventral, and posteroventral fossae, along with their associated bounding ridges, have previously been described in dicynodonts (King, Citation1988; Sullivan and Reisz, Citation2005; Angielczyk and Rubidge, Citation2010). Occasionally, a small posterodorsal fossa is also present (; Angielczyk et al., Citation2016). Angielczyk et al. (Citation2021a) reported an additional ridge splitting the posterodorsal fossa into two in Kembawacela, and this subtle feature is also visible in our scan (). Niassodon has a unique morphology in which the reflected lamina balloons out laterally to enclose a mediolaterally expanded angular cleft (). In the closely related Endothiodon bathystoma, the reflected lamina is thickened into a dorsoventrally elongate boss at its anteroventral end (). This thickening is absent in Endothiodon tolani (Cox and Angielczyk, Citation2015).
In general, surface features on the angular are simplified in derived dicynodonts. For example, in cryptodonts, the angular has fewer ridges and fossae than in more basal forms, though Tropidostoma does have a complex topography (). Geikia and the large-bodied Aulacephalodon have only the anterodorsal fossa and the posteroventral fossa, and this arrangement is also found in all dicynodontoids, making it the most common topographic pattern found in dicynodonts (, ; Janensch, Citation1952; Rowe, Citation1980; Liu, Citation2020). In these forms, the posteroventral fossa is bounded dorsally by a wide, convex area spanning the dorsal half of the reflected lamina.
The extent of the angular cleft is somewhat variable within Dicynodontia, but the dorsal notch is consistently placed far anteriorly. The early-diverging Niassodon has an angular cleft that extends anteriorly to just beneath the vertical ridge bordering the anterodorsal fossa (). The anterior edge of the angular cleft follows a straight line ventrally from this area. The ventral edge of the angular keel and reflected lamina is missing in this specimen, so it is uncertain whether the location of the cleft coincides with any surface features in this region. In Pristerodon (SAM-PK-K10153), Endothiodon (SAM-PK-K11034), and Tropidostoma (SAM-PK-8633) the ventral connection between the reflected lamina and the angular body occurs at the level of the ridge between the anteroventral and posteroventral fossae (). This is also true in more derived dicynodonts, although in some of these taxa the posteroventral fossa is located farther posteriorly relative to the dorsal notch, resulting in an angular cleft whose anterior margin is angled posteroventrally (). As in CGP/1/66, the surface features overlying the angular cleft are formed by corrugations in the bone, while ridges on the angular body are formed by thickening of the bone.
Gorgonopsia—Gorgonopsians have a stereotyped pattern of four fossae on their reflected lamina facing anterodorsally, posterodorsally, posteroventrally, and anteroventrally (; Kemp, Citation1969; Sigogneau-Russell, Citation1989; Kammerer, Citation2016). The ridges between the fossae form a cross-like shape with a strong vertical ridge and a weaker horizontal one. The vertical ridge tends to run in a slightly posteroventral direction. The anterior end of the horizontal ridge is at about the level of the ventral margin of the dentary, but its posterior end is near the dorsal notch. The horizontal ridge is often broader, less pronounced, and greatly attenuated in length compared with the vertical ridge (). As a result, the horizontal ridge is occasionally masked by specimen deformation, leading some authors to interpret the angular as lacking it (e.g., Barghusen, Citation1968). The horizontal ridge was reported to be absent in Nochnitsa, which has been proposed to be the basalmost gorgonopsian (Kammerer and Masyutin, Citation2018). While it is possible that this specimen has been deformed in a way that erased the subtle horizontal ridge, the absence of the ridge in this specimen may be a genuine feature indicating the plesiomorphic condition for Gorgonopsia. The horizontal ridge ranges from being straight to having dorsal concavities which produce small, blind-ended pockets, near where it intersects the vertical ridge (). Its anterior and posterior ends generally flare out into broad convex surfaces.
FIGURE 8. CT scan of the angular region in the gorgonopsian Cynariops robustus (MB.R.999). A, model showing surface features; B, model from A with angular cleft overlay. Box on silhouette illustrates location of images. Thick dotted line indicates anterior edge of the ventrally hanging reflected lamina. Abbreviations: ac, angular cleft; adf, anterodorsal fossa; ak, angular keel; avf, anteroventral fossa; dn, dorsal notch; for, foramen; pdf, posterodorsal fossa; pvf, posteroventral fossa; rl, reflected lamina. Scale bar equals 1 cm.
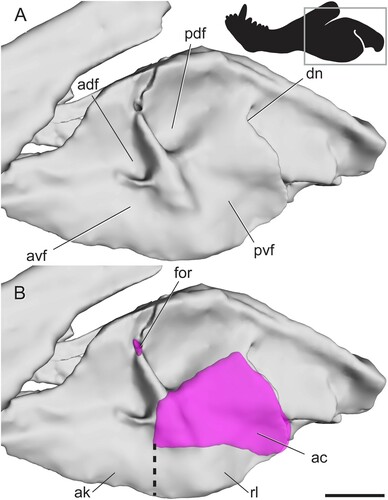
The posterior margin of the reflected lamina barely extends beyond the level of the dorsal notch, exposing a wider portion of the angular body laterally in the angular gap than in other therapsids (). The horizontal ridge marks the dorsal boundary of the angular cleft. The anterior boundary of the cleft is slightly anterior to the level of the vertical ridge, a feature also visible in a specimen of Cyonosaurus (BP/1/2598) and previously reported by Kemp (Citation1969). The dorsal half of the vertical ridge and the anterior half of the horizontal ridge are formed by thickening of the bone, while the parts of these ridges that overlie the angular cleft are formed by corrugations in the bone.
We observed a foramen at the dorsal tip of the vertical ridge in well-preserved specimens of Aelurognathus, Arctops, Cynariops, Lycaenops, and Scylacocephalus (). Kemp (Citation1969) figured the foramen, as well as three others along the dentary-angular contact, in an acid-prepared specimen of Arctognathus, but did not comment on their significance. Segmentation of the dorsal foramen in the scan of Cynariops reveals that it penetrates ventromedially from its appearance on the lateral surface of the angular and emerges on the medial side of the angular. The vertical ridge is otherwise formed by solid bone in Cynariops and all the other therapsids for which we had CT data. A similar foramen is unknown in other therapsids and its function in gorgonopsians remains obscure.
Therocephalia— The angular of therocephalians has a well-developed ridge at the anterior edge of the dorsal notch that runs posterodorsally, sometimes reaching or even slightly overlapping the surangular (). This ridge has been termed the angular crest (Barghusen, Citation1968), and its position is consistent with that of the dorsal ridge seen in other therapsids, especially the dorsally far-reaching ridge seen in many anomodonts (Angielczyk et al., Citation2021b). Some authors have mistakenly considered the bone surface between the angular crest and the convex surface posteroventral to it as part of the reflected lamina (Barghusen, Citation1968), but it is actually the angular body visible in a large dorsal notch (). This surface can appear to be part of the reflected lamina in specimens where the lamina is tightly pressed against the angular body. In eutherocephalians, an external mandibular fenestra is present between the angular and dentary, as in most anomodonts (; Huttenlocker, Citation2009).
FIGURE 9. Surface and CT scans and photos of the angular region in therocephalians. A, Hofmeyria atavus (TM 254) in left lateral view; B, photo of cf. Ictidostoma sp. (BP/1/3155, right side mirrored) in lateral view; C, pristerognathid therocephalian CGP RMS 1185 in left lateral view; D, interpretive drawing of cf. Ictidostoma sp. (BP/1/3155, right side mirrored); E, Lycosuchus vanderrieti (MB.R.995) in left lateral view; F, Pristerognathus polyodon (SAM-PK-11942) in medial view, G, Lycosuchus vanderrieti (MB.R.995) with angular cleft overlay. Box on silhouette illustrates location of images. Areas filled with hashed lines indicate external mandibular fenestra. Abbreviations: ac, angular cleft; acr, angular crest; adf, anterodorsal fossa; af, anterior fossa; dn, dorsal notch; pdf, posterodorsal fossa; pvf, posteroventral fossa; vcba, ventral connection to body of the angular; vf, ventral fossa. Scale bars equal 1 cm.
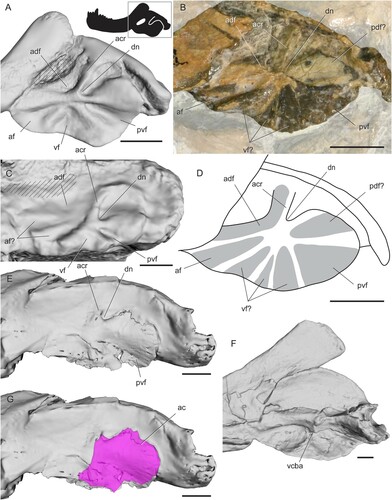
Therocephalians have a stereotyped pattern of four fossae radiating from a point just anterior to the center of the angular in lateral view (; Huttenlocker, Citation2009). Barghusen (Citation1968) described and numbered three of these fossae and the ridges bounding them, though these names were never broadly adopted in the therapsid literature. Interestingly, the arrangement of the fossae in therocephalians is nearly identical to that seen in some basal dicynodonts (). The angular crest meets a horizontal ridge on the body of the angular at a slightly oblique angle. Together, these ridges border the anterodorsal fossa. When present, the external mandibular fenestra is surrounded by this fossa on its ventral and posterior edges, as in dicynodonts. Ventral to the horizontal ridge is the thin anterior fossa (C1 of Barghusen, Citation1968), which is angled slightly ventrally. A gentle ridge bounds this fossa posteriorly, separating it from the wide ventral fossa (C2 of Barghusen, Citation1968). Further posterior is another gentle ridge and a posteroventral fossa (C3 of Barghusen, Citation1968), which is bordered dorsally by a wide, convex area spanning the dorsal half of the reflected lamina. The ridges and convexities described above radiate outwardly from roughly the same point just anterior to the middle of the angular, as in some dicynodonts (, ).
This stereotyped topographic pattern is present across nearly all therocephalians, with differences confirmed in only a few specimens. BP/1/3155, which is likely referrable to the hofmeyriid Ictidostoma, exhibits three additional ridges splitting the typical therocephalian fossae (). Based on their position, two of the extra ridges seem to split the ventral fossa into three smaller fossae, and the third ridge extends along the free dorsal edge of the reflected lamina, bounding a posterodorsal fossa. An additional ridge is also seen in the pristerognathid therocephalian specimen CGP RMS 1185 from the Tapinocephalus Assemblage Zone of South Africa, in which the anterior fossa seems to be split in two (). Liu and Abdala (Citation2017) reported only two fossae in the holotype of Dalongkoua fuae, the ventral and posteroventral fossae, though it is possible that other fossae were either overlooked or not well preserved in that specimen.
The dorsal notch is placed far anteriorly in therocephalians, resulting in an extensive free dorsal edge of the reflected lamina (Huttenlocker, Citation2009). The anterior edge of the angular cleft follows a fairly straight path ventrally near the point where the ridges meet. At its ventral end, the angular cleft curves anteriorly (). Much of the ventral portion of the angular is missing in the specimen of Lycosuchus examined, so this may be an incomplete picture of the extent of the angular cleft. However, the specimen of Pristerognathus (SAM-PK-11942) has the medial face of the reflected lamina partially prepared and seems to show that the ventral connection with the angular body occurs along the edge of the posteroventral fossa near the point from which the surface ridges radiate, which seems consistent with CT scan data for Lycosuchus and descriptions in the literature (; Barghusen, Citation1968).
Cynodontia— The reflected lamina is reduced in cynodonts so that the lateral surface of the angular body is broadly exposed. The topography of the angular is simplified in basal cynodonts compared with most other non-mammalian therapsids. At least two fossae are generally present, one facing posteriorly to posteroventrally and another ventral to that which faces antero- to posteroventrally (). These two fossae are present in Thrinaxodon; descriptions of a simple, splint-like reflected lamina in this genus (summarized by Parrington, Citation1979) were based on specimens missing much of the dorsal portion of the reflected lamina. Figures of the basal cynodont Charassognathus seem to indicate that the anteroventral fossa is divided into anterior and ventral components by a ridge, as in therocephalians and some dicynodonts (Botha et al., Citation2007). A posterodorsal fossa is also present in Galesaurus () and possibly Charassognathus (Botha et al., Citation2007). Eucynodonts completely lack ridges and fossae on the angular, where the bone is substantially reduced into a hook-shaped structure in lateral view (Allin and Hopson, Citation1992).
FIGURE 10. Surface and CT scans and photos of the angular region in non-eucynodont cynodonts. A, Procynosuchus delaharpeae (BP/1/226) in left lateral view; B, Galesaurus planiceps (BP/1/5064) in left lateral view; C, Thrinaxodon liorhinus (BP/1/2513) in left lateral view; D, Nshimbodon muchingaensis (NHCC LB277, right side mirrored) in lateral view; E, Thrinaxodon liorhinus (TM81, right side mirrored) in medial view; F, Nshimbodon muchingaensis (NHCC LB277, right side mirrored) with angular cleft overlay. Box on silhouette illustrates location of images. Thin dotted line indicates approximated minimum extent of missing reflected lamina. Thick dotted line indicates anterior edge of the ventrally hanging reflected lamina. Curved, solid lines indicate posterior edge of preserved dentary and posterior edge of the reflected lamina. Abbreviations: ab, angular body; ac, angular cleft; ak, angular keel; avf, anteroventral fossa; d, dentary; dn, dorsal notch; pdf, posterodorsal fossa; pvf, posteroventral fossa; rl, reflected lamina; vcba, ventral connection to body of the angular. Scale bars equal 1 cm.
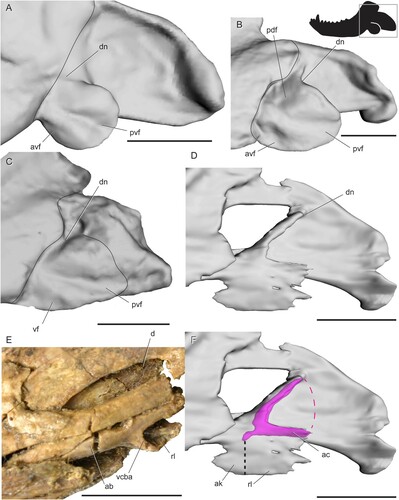
There is no substantial angular keel in cynodonts, so the whole lateral portion of the angular is a free-hanging reflected lamina with an angular cleft separating it from the angular body (, ). In non-eucynodont cynodonts, the sole remnant of an angular keel is a small finger of bone curving along the anteroventral edge of the medial face of the reflected lamina (). Eucynodonts show no evidence of an angular keel; their reflected lamina becomes a thin, simple splint of bone (Hopson and Kitching, Citation2001). The reflected lamina dips below the ventral margin of the jaw in Trirachodon berryi (), but it overlaps the body of the angular in Probainognathus ().
FIGURE 11. Surface and CT scans of the angular region in non-mammalian eucynodonts in left lateral view. A, Trirachodon berryi (BP/1/4658); B, Probainognathus jenseni (PVSJ 410) with angular cleft overlay. Box on silhouette illustrates location of images. Curved, solid line in B indicates posterior edge of preserved dentary. Abbreviations: ac, angular cleft; dn, dorsal notch. Scale bars equal 1 cm.
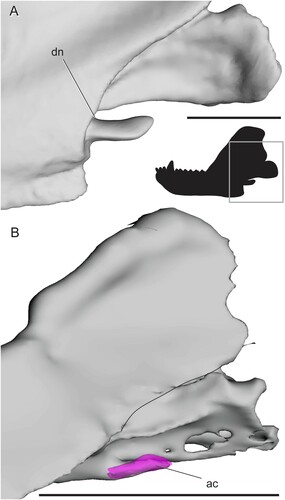
DISCUSSION
Comparative Anatomy of the Angular Complex
Revised Definition of the Reflected Lamina—The reflected lamina has been traditionally defined as the part of the angular that is mediolaterally separated from the angular body (Allin, Citation1975). Under this definition, the reflected lamina is restricted to the portion of the angular that overlies the angular cleft, but some authors have also included the ventrally hanging angular keel as part of the reflected lamina (King, Citation1981). It is also common in the literature to describe all surface features on the lateral surface of the angular as occurring on the reflected lamina, but our results indicate that much of this topography is more precisely understood to be located on the body of the angular, not its reflected lamina. Given the varied use of this term and the data presented here, we propose an updated definition of the term ‘reflected lamina’: the part of the angular bounding the angular cleft laterally, and the ventral continuation of this sheet of bone below the body of the angular. The more anterior part of the angular that hangs ventral to the jaw margin but is not separated mediolaterally from the body of the angular is the angular keel. Our revised definition has the benefit of relying on specific landmarks (i.e., the angular cleft and the ventral jaw margin) that allow for a precise delineation of each part of the angular. It is also useful because the reflected lamina and the part of the angular body with surface features would undoubtedly have different biomechanical properties (e.g., vibration quality or resistance to muscle strain), suggesting they should be considered separate functional units. The main downside to our proposed definition of the reflected lamina is that it is defined based on an internal structure (the angular cleft) that cannot be easily visualized in every fossil. Fortunately, there is a degree of correspondence between the external morphology and the extent of the underlying angular cleft: in every therapsid with angular ridges and fossae that we examined, the anterior extent of the angular cleft roughly coincides with the ridge bounding the posteroventral fossa anteriorly. This fossa is present in nearly all therapsids, so delineating the extent of the reflected lamina with only an exterior view of a given fossil should be feasible. The correspondence between the angular cleft and the overlying topography across therapsid clades also implies some degree of homology between reflected laminae under our definition, which is another reason this definition is biologically meaningful.
Systematic Review—Stereotyped patterns of ridges and fossae on the angular have long been recognized in gorgonopsians and therocephalians (Barghusen, Citation1968; Sigogneau, Citation1970; Sigogneau-Russell, Citation1989; Huttenlocker, Citation2009). Gorgonopsians have a clear vertical and a subdued horizontal ridge, whereas therocephalians have four radiating ridges and a long, pronounced angular crest. Distinct, consistent patterns have been more difficult to appreciate in other therapsid clades because of the rarity of mandibular material and/or a high degree of diversity in form within clades. Here we outline broad scale patterns of angular topography that are common within biarmosuchians, anomodonts, and cynodonts.
Biarmosuchians have a pattern of four radiating ridges, three of which are sharply defined while the posterior ridge is a broader, convex area (). These ridges bound four fossae, three of which are large and triangular whereas the posterodorsal fossa is smaller and more U-shaped. Anomodonts are diverse and particularly abundant in museum collections and thus highly variable, but general patterns can be seen in each subclade. We were unable to examine non-dicynodont anomodont material in person for this study, but photographs and published reports seem to agree that these taxa generally have an anterodorsal fossa shaped like a right triangle, a wide anteroventral fossa, a posterior to posteroventral fossa, and a small posterodorsal fossa (; Rubidge and Hopson, Citation1996; Rybczynski, Citation2000; Liu et al., Citation2010). Non-bidentalian dicynodonts exhibit remarkable variability in topography, but they often have four or five radiating fossae, sometimes missing the posterodorsal fossa and/or the ventral ridge subdividing the anteroventral fossa (). Most bidentalian dicynodonts only have the anterodorsal and posteroventral fossae, although some cryptodonts, particularly Tropidostoma, retain more complex angular topography. Non-eucynodont cynodonts are also variable, but they typically have at least the anteroventral and posteroventral fossae.
FIGURE 12. The distribution of angular traits within non-mammalian synapsids. A, cladogram showing relationships of the major synapsid subclades, with schematic left angulars depicting the condition for each group; B, expanded cladogram illustrating the diversity of angular topography in Dicynodontia. The following synapomorphies are noted: (1) dorsal notch located more anteriorly, thus creating a long dorsal free margin of the reflected lamina; (2) angular cleft enlarged; (3) reflected lamina has quadripartite ridge system with attendant fossae; (4) loss of ridges and fossae on reflected lamina; (5) pronounced vertical ridge on reflected lamina; (6) dorsal notch positioned more posteriorly, reducing free edge of the reflected lamina; (7) presence of one of more foramina at dorsal end of vertical ridge; (8) angular crest present; (9) external topography of angular reduced to two fossae; (10) reflected lamina fully separated, with angular cleft open dorsally and ventrally; (11) reflected lamina reduced to small, curved element; (12) external ridges and fossae of angular absent; (13) external angular ridges and fossae reduced. See for voucher specimens for each taxon. Higher level cladistic topology based on Sidor and Hopson (Citation1998), with dicynodont topology based on Olroyd et al. (Citation2018) and Kammerer and Ordoñez (Citation2021).
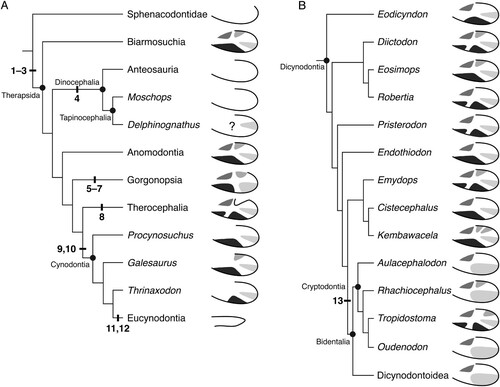
Recognition of these within-clade patterns will hopefully provide a baseline for future descriptive work, giving researchers a better idea of what to expect when examining a new specimen. This is especially important because the ridges and fossae on the angular are often subtle and easily masked by deformation, resulting in specimen descriptions that can inadvertently oversimplify the morphology present. For example, descriptions of non-bidentalian dicynodonts often miss subtle surface features on the angular that were recognized here (e.g., Crompton and Hotton, Citation1967; Sullivan and Reisz, Citation2005; Angielczyk and Rubidge, Citation2010), which is unsurprising given the sometimes overwhelming variability in this group and the lack of thorough comparisons of this morphology across taxa. We also encourage the use of the fossa names introduced here, as a consistent naming scheme will facilitate comparisons between taxa.
Evolution and Homologies of the Angular Complex
depicts the phylogenetic distribution of anatomical modifications to the angular region suggested by the systematic review discussed above. The cladistic relationships used are primarily those discussed by Rubidge and Sidor (Citation2001), with the dicynodont part of the tree based on Olroyd et al. (Citation2018) and Kammerer and Ordoñez (Citation2021). However, it should be noted that several studies have suggested alternative arrangements, and most agree that character support for the higher-level clades is weak (Hopson and Barghusen, Citation1986; Rowe, Citation1988; Sidor and Rubidge, Citation2006; Kemp, Citation2009).
A substantial morphological gap exists between sphenacodontids and the earliest therapsids, with Sidor and Hopson (Citation1998) noting that at least 36 character changes separate the two. Here, we recognize three angular features as first evolving in therapsids: the dorsal notch being located more anteriorly (leading to a longer free dorsal margin of the reflected lamina), enlargement of the angular cleft, and the development of a quadripartite ridge system with attendant fossae. The consistency of the positions and orientations of the individual fossae and ridges on the angular across highly disparate therapsid clades strongly suggests that at least some of these structures are homologous. The posteroventral fossa is nearly ubiquitous, absent only in dinocephalians (), and is thus highly likely to be homologous between therapsids. Almost every therapsid with angular surface features also has an anterodorsal fossa, with only cynodonts lacking this feature, presumably as a result of the enlarged dentary overlapping the angular in this area. The anteroventral fossa and its presumed derivatives (the anterior and ventral fossae of therocephalians and some dicynodonts) are found in all therapsids with ridges and fossae on the reflected lamina except most of the bidentalian dicynodonts. The presence of an anteroventral fossa in basal anomodonts () suggests that this feature is homologous among major therapsid groups and was lost in some dicynodonts. The presence of an additional ridge subdividing the anteroventral fossa in therocephalians and basal dicynodonts is interesting but unlikely to represent a direct homology, as this feature is sporadically present in dicynodonts, absent in Eodicynodon, and unreported for any non-dicynodont anomodont. The posterodorsal fossa is also variably present in early-diverging dicynodonts, but it is present in many other therapsids. The distinctive shape of the dorsal notch in therocephalians may even be a derivative of this posterodorsal fossa, as the tissue between the ridges normally bounding this fossa in other taxa may not have ossified in therocephalians. This hypothesis is supported by the fact that the shape and relative position is consistent between the therocephalian dorsal notch and the posterodorsal fossa of other therapsids (). Barghusen (Citation1968) recognized the similarity between these two structures in therocephalians and gorgonopsians and proposed a functional link between them. A fossa in this position is also seen in several non-dicynodont anomodonts (; Rubidge and Hopson, Citation1996; Rybczynski, Citation2000; Liu et al., Citation2010), which supports the possibility that it is homologous across therapsids and was lost in some cynodonts and dicynodonts. The homology of the specific fossae and ridges among therapsids is also supported by their consistent spatial relationship to the angular cleft. The anterior extent of the cleft seems to roughly coincide with the ridge that bounds the posteroventral fossa anteriorly, as does the ventral connection with the angular body. This suggests that the ridge between the posteroventral and anteroventral/ventral fossae is homologous across therapsids.
Under the relationships shown in , the loss of an external ridge system would be a reversal for dinocephalians and, indeed, its lack has previously been considered a dinocephalian synapomorphy under some phylogenetic topologies (Sidor, Citation2000). This could be related to the agonistic headbutting behavior that has been inferred for dinocephalians based on pachyostosis in the skull (Barghusen, Citation1975; Benoit et al., Citation2016). A thin reflected lamina may be vulnerable to damage during fights, so this structure may have thickened in dinocephalians, masking the fine surface details seen in other therapsids (J. A. Hopson, pers. comm.). The mandible has not been reconstructed as directly participating in dinocephalian headbutting, so this hypothesis would depend on how exactly stresses in the skull were distributed and what other antagonistic behaviors occurred. However, it should be noted that the phylogenetic position of dinocephalians is debatable. Sidor and Rubidge (Citation2006) placed a monophyletic Dinocephalia (Anteosauria + Tapinocephalia) as the earliest diverging group of therapsids in their majority rule tree, in which case the lack of ridges would be interpreted as a plesiomorphy. Alternatively, Watson and Romer (Citation1956) suggested that dinocephalians and anomodonts were close relatives, which was emphasized by King (Citation1988), and more recently by Liu et al. (Citation2010), which would likely only reinforce the character optimization outlined in . The apparent presence of a fossa in ‘Delphinognathus’ is puzzling. It is important to note that ‘Delphinognathus’ is likely a nomen dubium, possibly representing a young individual of a known genus (Atayman-Güven et al., Citation2009). Though this fossa could be an ontogenetically variable feature in dinocephalians, small individuals of the anteosaurian dinocephalian Sinophoneus lack surface features (Liu, Citation2013), so ontogeny alone cannot account for the morphology seen in ‘Delphinognathus.’ Further research on dinocephalian jaws with well-preserved surface features may help clarify whether the reflected lamina is truly devoid of surface features in this clade. Ultimately, the phylogenetic arrangement of non-theriodont therapsids lacks stability and must be readdressed with improved character data.
The ancestral condition for anomodonts is unclear because we were unable to directly examine any non-dicynodont anomodonts where the angular was sufficiently well preserved for this study. However, the condition described for Biseridens (Liu et al., Citation2010) and evident in photographs of Ulemica and Suminia () is very similar to what we show for Eosimops, and so this reflects our best understanding (). As noted above, dicynodonts are taxonomically diverse and show a corresponding diversity in their angular morphology. Bidentalian dicynodonts reduced complexity of the ridge system, but better sampling is needed to explain the re-occurrence of these ridges in Tropidostoma.
The morphology of the angular in gorgonopsians has long been recognized as diagnostic for the group (Kemp, Citation1969, Citation1982; Sigogneau, Citation1970; Sigogneau-Russell, Citation1989) and we identify three features as likely synapomorphies of this clade. The first feature is the pronounced vertical ridge on the external surface of the angular. This feature is very well known, but our analysis suggests that it is best understood as a highly modified version of therapsid quadripartite morphology, with the horizontal rami reduced substantially, and not an independent evolution of angular topography. Second, the dorsal notch is positioned relatively posteroventrally, reducing the free edge of the reflected lamina to only its more posterior portion. Third, the presence of one or more foramina at the dorsal end of the vertical ridge is a feature that we have seen only in gorgonopsians among taxa with a reflected lamina. A systematic appraisal of the latter feature might be useful for within-clade relationships.
Gorgonopsians and biarmosuchians were found to be sister taxa by Sidor (Citation2000), a result discussed by Sidor and Rubidge (Citation2006) and more recently recovered by Liu et al. (Citation2010). The angular in biarmosuchians is similar to that of gorgonopsians in that the free dorsal margin of the reflected lamina is relatively shorter than in other therapsids, but it does not approach the unique condition seen in gorgonopsians. Relatedly, our review suggests that biarmosuchians have a relatively anteroposteriorly short reflected lamina region, and this likely contributes to the appearance of a posterior placement for the dorsal notch. Additional data will need to be gathered to test our suggestion, but for the time being we do not recognize any derived features of the angular shared between gorgonopsians and biarmosuchians that would support a particularly close relationship between the two groups.
It should be noted that the earliest-diverging gorgonopsian Nochnitsa apparently lacks a horizontal ridge separating the two dorsal fossae from their ventral counterparts (Kammerer and Masyutin, Citation2018). This has been interpreted as the ancestral condition for Gorgonopsia. The horizontal ridge is subtle and often obscured by deformation in gorgonopsian specimens, so additional specimens of early gorgonopsians will aid in our understanding of the ancestral condition in this clade. Given the distribution and similarities of the fossae across therapsids, we propose that a topographic pattern of four fossae separated by four ridges was present in the common ancestor of all known therapsids and was inherited by most of its descendants.
Therocephalian relationships have received renewed scrutiny over the past decade, including revisiting Kemp’s (Citation1972) hypothesis that this group is better understood as paraphyletic relative to cynodonts (Abdala et al., Citation2008). Recent work has advocated for the traditional hypothesis of therocephalian monophyly (Hopson and Barghusen, Citation1986; Huttenlocker Citation2009), which agrees with the elongated angular crest being unique to therocephalians and best considered a synapomorphy of the group.
The fossil record of non-mammalian cynodonts is well known to record a pronounced reduction in the size of the reflected lamina of the angular, and this transformation is often captured in cladistic data sets (Sidor and Hopson, Citation1998). Our review suggests that a reduction in the surface features of the angular can be considered a cynodont synapomorphy, even though the overall size of the reflected lamina was only slightly reduced compared with the condition in other theriodonts. In addition, the ventral connection between the reflected and medial laminae shifted anteriorly in cynodonts, resulting in a fully separated reflected lamina with an angular cleft that was open both dorsally and ventrally. In eucynodonts, the reflected lamina was reduced to a small, curved element and the angular lacked all surface features.
Function of the Angular Complex
Our systematic review reveals a few clues that help to narrow down the potential functions of the angular complex in non-mammalian therapsids. Many authors have considered the angular cleft as an area for the insertion of the posterior pterygoideus muscle (Romer and Price, Citation1940; Watson, Citation1953; Barghusen, Citation1968), although King (Citation1981) favored the attachment of throat muscles here and others have suggested that the other jaw adductors might partially insert in the dorsal region of the cleft (Westoll, Citation1943; Kemp, Citation1982). Under this scenario, the cleft would be filled with muscle attached to the medial lamina and/or the medial face of the reflected lamina. Keyser (Citation1974) attributed increased robusticity of the reflected lamina in kannemeyeriid dicynodonts to a change in diet and roughly correlated it with the reduction or loss of the quadrate foramen. King et al. (Citation1989) noted striations on the medial lamina and medial face of the reflected lamina in dicynodonts that they interpreted as muscle scars. This hypothesis is also consistent with our findings that a trend towards the simplification of angular topography is seen in both dicynodonts and cynodonts (), two clades in which the pterygoideus musculature was likely reduced over time (Kemp, Citation1982; King et al., Citation1989).
However, the shape and orientation of the angular cleft make it unlikely that the cleft was solely a site for muscle attachment in all non-eucynodont therapsids. The cleft is so deeply incised and bounded by bone at its ventral edge in some taxa (e.g., Hipposaurus and some dicynodonts; , ) that any muscle originating from the quadrate ramus of the pterygoid must take an indirect path across the thin edges of the medial lamina to access the deeper parts of the cleft. If this structure was only involved in muscular attachment, one would expect the angular cleft to exist only where the posterior pterygoideus could easily reach it. While there could be structural or developmental reasons that this additional space existed, it seems likely that there was another function for the angular cleft, even if this function was secondary to a function in muscle attachment. The posterior pterygoideus is also expected to insert near the articular (Barghusen, Citation1973), so the anterior extension of the angular cleft in therapsids is inconsistent with a purely muscular interpretation of the structure. It is also important to consider that the angular cleft is quite narrow in many therapsids (e.g., 2–10 mm), even in large dinocephalians and dicynodonts, which makes it unlikely that a large jaw adductor would be able to contract in this space (Camp, Citation1948; Allin, Citation1975; Barghusen, Citation1976), although a tendinous insertion is another possibility. Corrugations on the medial surface of the reflected lamina would make this an uneven surface, which would be difficult for a muscle to insert onto from a ventral direction.
Several authors have also proposed that the fossae and ridges on the lateral face of the angular served as muscle attachment sites, and that the reflected lamina was largely useful in providing additional area for the attachment of multiple muscles in this region of the jaw. The fossae on the ventral half of the angular have been reconstructed as sites for the attachment of throat constrictor, tongue, and hyoid muscles, usually in therocephalians (Janensch, Citation1952; Barghusen, Citation1968; Kemp, Citation1982). Various branches of the external jaw adductors are often considered to insert on the more dorsal portion of the reflected lamina, specifically in the posterodorsal fossa in gorgonopsians and the dorsal notch in therocephalians (Barghusen, Citation1968; Kemp, Citation1969, Citation1982; King, Citation1981). Kemp (Citation1982) proposed that a muscle similar in function to the mammalian digastric attached to the reflected lamina and pulled posteroventrally, interpreting thin striations on the surface of the reflected lamina in dinocephalians and dicynodonts as muscle scars associated with his suggested jaw opening function.
The hypothesis that each fossa was a site for the attachment of a specific muscle offers a satisfying explanation for the mostly conserved orientation of the fossae across Therapsida. That said, the existing propositions for the identity of those muscles all suffer from the same shortcoming: a lack of comparison of topographic patterns between therapsid clades. Oftentimes the same fossa is proposed to have different muscular associations in different taxa. For example, Barghusen (Citation1968) placed the insertion for his zygomaticomandibularis muscle in the posterodorsal fossa in gorgonopsians and the possibly homologous U-shaped dorsal notch in therocephalians. He claimed that a reflected lamina with an extensive free dorsal margin could not withstand the tension exerted by this muscle, yet many anomodonts have both a posterodorsal fossa and a free dorsal margin. It is unlikely that these fossae would have retained their orientation if they served as attachments for different muscles between therapsid clades, so any muscles proposed to attach to the angular ridges and fossae should be consistent in the majority of therapsids. The overall similarity between basal anomodonts and other therapsids is difficult to explain if the ridges and fossae relate to musculature, as anomodonts are the only non-eucynodont therapsids in which mastication has been inferred, and their jaw musculature was accordingly unique (King, Citation1988). The repeated loss of fossae is also surprising if each fossa housed a muscle attachment, especially given the high degree of variation in the presence and absence of fossae among some closely related taxa (). Some of this variation may be attributable to differences in preservation, but it is unlikely to explain all of the observed variation. Another issue with this hypothesis is that some of the fossae have shapes or orientations that would likely not serve as useful attachments for any known muscle. The anterodorsal fossa is seen in most non-eucynodont therapsids, but the only muscle that would attach to the mandible from an anterodorsal direction would be the specialized jaw adductor of dicynodonts (analogous with the mammalian masseter; King et al., Citation1989). This fossa is absent in cynodonts, so even the masseter itself could not have inserted here. Similarly, the anteroventral fossa(e) would only provide a small area of attachment for the usually wide intermandibularis muscles that have been proposed to reside here (Janensch, Citation1952; Barghusen, Citation1968). Our systematic survey across Therapsida complicates the hypothesis that the external angular fossae served as sites for muscle attachment.
There are conflicting views on whether the thin reflected lamina could withstand the forces that would be applied to it by muscle action. Some authors assert that this structure was too thin and poorly supported in many therapsids to serve as a muscle attachment site (Maier and Ruf, Citation2016), although others consider it suitable for smaller throat muscles (Barghusen, Citation1968). However, some authors have suggested that the corrugations strengthen the reflected lamina against strain from attached muscles (Parrington, Citation1955), while others favor a more general strengthening function that is not necessarily related to muscle attachments (Allin, Citation1975; Maier and van den Heever, Citation2002). However, it is striking that the basic pattern of four fossae and ridges in roughly the same orientations is found across clades with such broadly differing ecologies. The directionality of the fossae and ridges is probably important for their function if they are so highly conserved, which makes it unlikely that their only purpose was to provide general structural support to the reflected lamina. Importantly, a chief finding of this study is that much of this topography is not located on the reflected lamina itself; it is on the angular body or angular keel, which is often thicker than the reflected lamina and probably did not require the same amount of structural support. The ridges and fossae on the angular probably did not exist solely to strengthen the reflected lamina.
Adding to the confusion surrounding the angular complex is the foramen at the dorsal tip of the vertical ridge in gorgonopsians (). This foramen is visible in well-preserved specimens of both early- and late-diverging genera, so it is likely a common feature of Gorgonopsia as a whole. To our knowledge, the function of this foramen has never been addressed in the literature. Its absence in other therapsids suggests that its role in the function of the angular complex was unique to gorgonopsians and, possibly, that its appearance is related to the posterior shift in the position of the dorsal notch characteristic of the group. Scan data is not sufficient to determine if the impression of a blood vessel is present adjacent to the foramen medially, and so it is unclear if the foramen housed a nerve, blood vessel, or both. Future research on the contents of this foramen would likely further our understanding of the function of the angular complex in gorgonopsians.
Overall, muscle attachment seems incapable of explaining all of the features seen in the morphology of the angular in non-mammalian therapsids. There are areas of the angular cleft in some taxa that would be inaccessible to any known muscle. The four fossae and ridges that were probably plesiomorphic for Therapsida (although possibly excluding dinocephalians; see above) do not have known muscles that could have fit into each of them in every taxon. There is also variation in the presence or absence of the posterodorsal fossa and a subdivision of the anteroventral fossa, even between closely related taxa. Structural support alone also cannot explain the consistent directionality of the fossae among therapsids. Even a combination of these two does not explain the consistent presence of the anterodorsal fossa on the body of the angular. There was probably an additional function for these structures.
The idea that the angular complex was involved in sound reception before the appearance of the mammalian middle ear is widely favored (Allin, Citation1975; Crompton and Parker, Citation1978; Allin and Hopson, Citation1992; Laurin, Citation1998; Laaß, Citation2016). This hypothesis is supported by several lines of circumstantial evidence, such as the often loose connection between the dentary and postdentary bones in therapsids and the orientation of the stapes towards the postdentary bones in a few taxa (Laaß, Citation2016). Some features of the angular complex itself are also consistent with this hypothesis. The deeply incised angular cleft could be explained by an auditory function, as this space may have been useful as a resonating chamber for sounds collected by the overlying tissues (Westoll, Citation1943). The trend towards expanding the angular cleft and freeing the reflected lamina from the angular body in multiple therapsid clades would support this hypothesis, as these changes would likely allow the reflected lamina to vibrate more effectively. However, King (Citation1981) questioned whether the ridges and fossae on the angular would provide any function for a sound receiver, and an auditory function for these surface features has never been proposed, to our knowledge. One potential auditory function for the corrugations could be in altering the vibrational qualities of the angular. The shape of an object affects both the way that it vibrates and the way that sound waves reflect off it. The folds of the mammalian pinna serve to alter the sound waves entering the external ear, amplifying biologically relevant frequencies and providing information about the direction of sound sources (Kahana and Nelson, Citation2006). A similar function could be conceived for the topography of the reflected lamina if it was indeed involved in sound reception, especially if the orientation of the fossae could somehow aid in the perception of directionality. Even the ridges and fossae on the angular body could potentially be useful in this way, depending on how exactly the structure and the tissue around it was vibrating. Similarly, if the resonator hypothesis of Westoll (Citation1943) were true, the corrugations could alter the sounds produced by the angular cleft, although one might expect more interspecific variability if this structure was used in vocal communication with conspecifics. An auditory function for the ridges and fossae of the angular is highly speculative and would require confirmation from biomechanical modeling, but it is the only hypothesis proposed to our knowledge that could potentially explain all aspects of the angular complex. Therefore, it warrants further investigation. Biomechanical modeling of the angular complex could reveal any acoustical consequences for different patterns of surface topology, expansion of the angular cleft, and changes in the relative size of the reflected lamina across Synapsida.
CONCLUSIONS
The ancestral condition for the lateral surface of the therapsid angular was likely a pattern of four fossae bounded by ridges radiating from a central point, with the position of each fossa remaining fairly consistent across the major therapsid subclades. Individual fossae and ridges on the angular may be useful phylogenetic characters for assessing the relationships between the major therapsid clades. Alterations to the ancestral topographic pattern generally track phylogeny, with most therapsid subclades having a stereotyped variant of this topographic pattern. The recognition of similarities within and between therapsid clades will allow more informative descriptions of angular topography in new specimens. These similarities also imply functional similarity across non-eucynodont therapsids, and our findings pose several problems for reconstructed muscle attachments on the angular complex. A multi-functional role in hearing and muscular attachment may explain the anatomy of these features.
Supplemental Material
Download MS Word (47.9 KB)ACKNOWLEDGMENTS
This project was funded by the AAUW American Fellowship, the University of Washington WRF-Hall Fellowship and Snyder Award, the Society of Vertebrate Paleontology Cohen Prize, the Paleontological Society Feldmann Grant (all to SLO), as well as NSF ANT-1341304 and EAR 1337569 (to CAS). We thank R. Araújo, E.-M. Bendel, J. Benoit, A. Duhamel, J. Maisano, L. Pusch, DigiMorph.org, the Evolutionary Studies Institute in Johannesburg, and the Museum für Naturkunde in Berlin for providing access to the CT data used in this study. Museum collections access and assistance was generously provided by K. Angielczyk, E. Butler, C. Byrd, H. Fourie, M. Goodwin, P. Holroyd, S. Jirah, N. Mchunu, W. Simpson, Z. Skosan, A. Stroup, and B. Zipfel. We thank M. Rivin for training on the white light scanner and R. Eng for access to photography equipment. The photo for was provided by B. Peecook. C. Kammerer and A. Huttenlocker aided with taxonomic identification for some specimens. J. Hopson, P. Kramer, S. Santana, G. Wilson Mantilla, and reviewers C. Abbott and F. Abdala provided feedback that greatly improved the project. SLO would also like to thank R. M. S. Smith for hosting her during her visit to the Iziko South African Museum and V. Radermacher for providing transport to museums that looked much closer on the map.
LITERATURE CITED
- Abdala, F., B. S. Rubidge, and J. van den Heever. 2008. The oldest therocephalians (Therapsida, Eutheriodontia) and the early diversification of Therapsida. Palaeontology 51:1011–1024.
- Adams, L. A. 1919. A memoir on the phylogeny of the jaw muscles in recent and fossil vertebrates. Annals of the New York Academy of Sciences 28:51–166.
- Allin, E. F. 1975. Evolution of the mammalian middle ear. Journal of Morphology 147:403–437.
- Allin, E. F., and J. A. Hopson. 1992. Evolution of the auditory system in Synapsida (“mammal like reptiles” and primitive mammals) as seen in the fossil record; pp. 587–614 in The evolutionary biology of hearing. Springer, New York, NY.
- Angielczyk, K. D., and C. F. Kammerer. 2018. Non-mammalian synapsids: the deep roots of the mammalian family tree; pp. 117–198 in F. E. Zachos and R. J. Asher (eds.), Handbook of Zoology: Mammalia: Mammalian Evolution, Diversity and Systematics. De Grutyer, Berlin.
- Angielczyk, K. D., and B. S. Rubidge. 2010. A new pylaecephalid dicynodont (Therapsida, Anomodontia) from the Tapinocephalus Assemblage Zone, Karoo Basin, Middle Permian of South Africa. Journal of Vertebrate Paleontology 30:1396–1409.
- Angielczyk, K. D., and B. S. Rubidge. 2013. Skeletal morphology, phylogenetic relationships and stratigraphic range of Eosimops newtoni Broom, 1921, a pylaecephalid dicynodont (Therapsida, Anomodontia) from the middle Permian of South Africa. Journal of Systematic Palaeontology 11:191–231.
- Angielczyk, K. D., J. Benoit, and B. S. Rubidge. 2021a. A new tusked cistecephalid dicynodont (Therapsida, Anomodontia) from the upper Permian upper Madumabisa Mudstone formation, Luangwa Basin, Zambia. Papers in Palaeontology 7:405–446.
- Angielczyk, K. D., J. Liu, and W. Yang. 2021b. A redescription of Kunpania scopulusa, a bidentalian dicynodont (Therapsida, Anomodontia) from the ? Guadalupian of northwestern China. Journal of Vertebrate Paleontology 41: e1922428.
- Angielczyk, K. D., B. S. Rubidge, M. O. Day, and F. Lin. 2016. A reevaluation of Brachyprosopus broomi and Chelydontops altidentalis, dicynodonts (Therapsida, Anomodontia) from the middle Permian Tapinocephalus Assemblage Zone of the Karoo Basin, South Africa. Journal of Vertebrate Paleontology e1078342.
- Atayman-Güven, S., B. S. Rubidge, and F. Abdala. 2009. Taxonomic re-evaluation of tapinocephalid dinocephalians. Palaeontologia Africana 44:83–87.
- Barghusen, H. 1968. The lower jaw of cynodonts (Reptilia, Therapsida) and the evolutionary origin of mammal-like adductor jaw musculature. Postilla 116:1–49.
- Barghusen, H. 1973. The adductor jaw musculature of Dimetrodon (Reptilia, Pelycosauria). Journal of Paleontology, 1:823–834.
- Barghusen, H. 1975. A review of fighting adaptations in dinocephalians (Reptilia, Therapsida). Paleobiology, 1:295–311.
- Barghusen, H. 1976. Notes on the adductor jaw musculature of Venjukovia, a primitive anomodont therapsid from the Permian of the USSR. Annals of the South African Museum 69:249–260.
- Bendel, E.-M., C. F. Kammerer, N. Kardjilov, V. Fernandez, and J. Fröbisch. 2018a. Cranial anatomy of the gorgonopsian Cynariops robustus based on CT-reconstruction. PLOS One 13:e0207367.
- Bendel, E.-M., C. F. Kammerer, N. Kardjilov, V. Fernandez, and J. Fröbisch. 2018b. Cranial Anatomy of the Gorgonopsian Cynariops robustus Based on CT-Reconstruction. Data Publisher: Museum für Naturkunde Berlin (MfN) - Leibniz Institute for Evolution and Biodiversity Science.
- Benoit, J., P. Manger, V. Fernandez, and B. S. Rubidge. 2016. Cranial bosses of Choerosaurus dejageri (Therapsida, Therocephalia): Earliest evidence of cranial display structures in eutheriodonts. PLOS One 11(8):e0161457.
- Benoit, J., P. Manger, V. Fernandez, and B. S. Rubidge. 2017. The bony labyrinth of late Permian Biarmosuchia: palaeobiology and diversity in non-mammalian Therapsida. Palaeontologia Africana 52:58–77.
- Botha, J., F. Abdala, and R. M. H. Smith. 2007. The oldest cynodont: new clues on the origin and early diversification of the Cynodontia. Zoological Journal of the Linnean Society 149:77–492.
- Bramble, D. M. 1978. Origin of the mammalian feeding complex: models and mechanisms. Paleobiology 4:271–301.
- Broom, R. 1932. The Mammal-like Reptiles of South Africa and the Origin of Mammals. Witherby, London, 376 pp.
- Camp, C. L. 1948. The dicynodont ear. Special Publications of the Royal Society of South Africa, Robert Broom Commemmorative Volume 109–111.
- Castanhinha, R., R. Araújo, L. C. Junior, K. D. Angielczyk, G. Martins, R. M. S. Martins, C. Chaouiya, F. Beckman, and F. Wilde. 2013. Bringing dicynodonts back to life: Paleobiology and anatomy of a new emydopoid genus from the upper Permian of Mozambique. PLOS One 8:e80974.
- Cox, C. B., and K. D. Angielczyk. 2015. A new endothiodont dicynodont (Therapsida, Anomodontia) from the Permian Ruhuhu Formation (Songea Group) of Tanzania and its feeding system. Journal of Vertebrate Paleontology 35:e935388.
- Crompton, A. W. 1963. The evolution of the mammalian jaw. Evolution 17:431–439.
- Crompton, A. W. 1972. The evolution of the jaw articulation of cynodonts; pp. 231–251 in K. A. Joysey and T. S. Kemp (eds.), Studies in Vertebrate Evolution. Oliver and Boyd, Edinburgh.
- Crompton, A. W., and N. Hotton. 1967. Functional morphology of the masticatory apparatus of two dicynodonts (Reptilia, Therapsida). Postilla 108:1–51.
- Crompton, A. W., and P. Parker. 1978. Evolution of the mammalian masticatory apparatus. American Scientist 66:192–201.
- DeMar, R. E., and H. Barghusen. 1972. Mechanics and the evolution of the synapsid jaw. Evolution 26:622–637.
- Farmer, C. G. 2000. Parental care: the key to understanding endothermy and other convergent features in birds and mammals. The American Naturalist 155:326–334.
- Hopson, J. A. 2012. The role of foraging mode in the origin of therapsids: Implications for the origin of mammalian endothermy. Fieldiana Life and Earth Sciences 5:126–148.
- Hopson, J. A., and H. Barghusen. 1986. An analysis of therapsid relationships; pp. 83–106 in N. Hotton, P. D. MacLean, J. J. Roth, and E. C. Roth (eds.), The Ecology and Biology of the Mammal-like Reptiles. Smithsonian Institution Press, Washington.
- Hopson, J. A., and J. W. Kitching. 2001. A probainognathian cynodont from South Africa and the phylogeny of nonmammalian cynodonts. Bulletin of the Museum of Comparative Zoology 156(1):5–35.
- Huttenlocker, A. K. 2009. An investigation into the cladistic relationships and monophyly of therocephalian therapsids (Amniota: Synapsida). Zoological Journal of the Linnean Society 157:865–891.
- Huttenlocker, A. K., and C. A. Sidor. 2020. A basal nonmammaliaform cynodont from the Permian of Zambia and the origins of mammalian endocranial and postcranial anatomy. Journal of Vertebrate Paleontology 40:e1827413.
- Huttenlocker, A. K., S. A. Singh, A. C. Henrici, and S. S. Sumida. 2021. A Carboniferous synapsid with caniniform teeth and a reappraisal of mandibular size-shape heterodonty in the origin of mammals. Royal Society Open Science 8:211237.
- Janensch, V. W. 1952. Über den Unterkiefer der Therapsiden. Paläontologische Zeitschrift 26:229–247.
- Kahana, Y., and P. A. Nelson. 2006. Numerical modelling of the spatial acoustic response of the human pinna. Journal of Sound and Vibration 292:148–172.
- Kammerer, C. F. 2011. Systematics of the Anteosauria (Therapsida: Dinocephalia). Journal of Systematic Palaeontology 9:261–304.
- Kammerer, C. F. 2016. Systematics of the Rubidgeinae (Therapsida: Gorgonopsia). PeerJ 4: e1608.
- Kammerer, C. F., and V. Masyutin. 2018. Gorgonopsian therapsids (Nochnitsa gen. nov. and Viatkogorgon) from the Permian Kotelnich locality of Russia. PeerJ 6:e4954.
- Kammerer, C. F., and M. D. Ordoñez. 2021. Dicynodonts (Therapsida: Anomodontia) of South America. Journal of South American Earth Sciences 108:10317.
- Kemp, T. S. 1969. On the functional morphology of the gorgonopsid skull. Philosophical Transactions of the Royal Society of London B 256:1–83.
- Kemp, T. S. 1972. Whaitsiid Therocephalia and the origin of cynodonts. Philosophical Transactions of the Royal Society of London B 264:1–54.
- Kemp, T. S. 1982. Mammal-like Reptiles and the Origin of Mammals. Academic Press, Inc., London, 363 pp.
- Kemp, T. S. 2007. The origin of higher taxa: macroevolutionary processes, and the case of the mammals. Acta Zoologica 88:3–22.
- Kemp, T. S. 2009. Phylogenetic interrelationships and pattern of evolution of the therapsids: testing for polytomy. Palaeontologia Africana 44:1–12.
- Kermack, K. A., F. Mussett, and H. W. Rigney. 1973. The lower jaw of Morganucodon. Zoological Journal of the Linnean Society 53:87–175.
- Keyser, A. W. 1974. Evolutionary trends in Triassic Dicynodontia. Palaeontologia Africana 17:57–68.
- Keyser, A. W., and A. R. I. Cruickshank. 1979. The origin and classification of Triassic dicynodonts. Transactions of the Geological Society of South Africa 82:81–108.
- King, G. M. 1981. The functional anatomy of a Permian dicynodont. Philosophical Transactions of the Royal Society of London B 291:243–322.
- King, G. M. 1988. Anomodontia; pp. 1–84 in O. Kuhn (ed.), Handbuch der Paläoherpetologie, 17th ed. Fischer-Verlag, Stuttgart.
- King, G. M., B. W. Oelofsen, and B. S. Rubidge. 1989. The evolution of the dicynodont feeding system. Zoological Journal of the Linnean Society 96:185–211.
- Laaß, M. 2016. Virtual reconstruction and description of the cranial endocast of Pristerodon mackayi (Therapsida, Anomodontia). Journal of Morphology 276:1089–1099.
- Laurin, M. 1998. New data on the cranial anatomy of Lycaenops (Synapsida, Gorgonopsidae), and reflections on the possible presence of streptostyly in gorgonopsians. Journal of Vertebrate Paleontology 18:765–776.
- Lautenschlager, S., P. Gill, Z. X. Luo, M. J. Fagan, and E. J. Rayfield. 2017. Morphological evolution of the mammalian jaw adductor complex. Biological Reviews of the Cambridge Philosophical Society 92:1910–1940.
- Liu, J. 2013. Osteology, ontogeny, and phylogenetic position of Sinophoneus yumenensis (Therapsida, Dinocephalia) from the Middle Permian Dashankou Fauna of China. Journal of Vertebrate Paleontology 33:1394–1407.
- Liu, J. 2020. Taoheodon baizhijuni, gen. et sp. nov. (Anomodontia, Dicynodontoidea), from the Upper Permian Sunjiagou Formation of China and its implications. Journal of Vertebrate Paleontology 40:e1762088.
- Liu, J., and F. Abdala. 2017. Therocephalian (Therapsida) and chroniosuchian (Reptiliomorpha) from the Permo-Triassic transitional Guodikeng Formation of the Dalongkou Section, Jimsar, Xinjiang, China. Vertebrata PalAsiatica 55:24–40.
- Liu, J., B. S. Rubidge, and J. Li. 2010. A new specimen of Biseridens qilianicus indicates its phylogenetic position as the most basal anomodont. Proceedings of the Royal Society B 277:rspb20090883.
- Maier, W., and J. A. van den Heever. 2002. Middle ear structures in the Permian Glanosuchus sp. (Therocephalia, Therapsida), based on thin sections. Fossil Record 5:309–318.
- Maier, W., and I. Ruf. 2016. Evolution of the mammalian middle ear: a historical review. Journal of Anatomy 228:270–283.
- Meng, J., S. Bi, X.-T. Zheng, and X.-L. Wang. 2018. Ear ossicle morphology of the Jurassic euharamiyidan Arboroharamiya and evolution of mammalian middle ear. Journal of Morphology 279:441–457.
- Olroyd, S. L., C. A. Sidor, and K. D. Angielczyk. 2018. New materials of the enigmatic dicynodont Abajudon kaayai (Therapsida, Anomodontia) from the lower Madumabisa Mudstone Formation, middle Permian of Zambia. Journal of Vertebrate Paleontology 37:e1403442.
- Palmer, R. W. 1913. Note on the lower jaw and ear ossicles of a foetal Perameles. Anatomischer Anzeiger 43:510–515.
- Parrington, F. R. 1955. On the cranial anatomy of some gorgonopsids and the synapsid middle ear. Proceedings of the Zoological Society of London 25:1–40.
- Parrington, F. R. 1979. The evolution of the mammalian middle and outer ears: A personal review. Biological Reviews of the Cambridge Philosophical Society 54:369–387.
- Pusch, L., J. Ponstein, C. F. Kammerer, and J. Fröbisch. 2019a. Novel endocranial data on the early therocephalian Lycosuchus vanderrieti underpin high character variability in early theriodont evolution. Frontiers in Ecology and Evolution 7:464.
- Pusch, L., J. Ponstein, C. F. Kammerer, and J. Fröbisch. 2019b. Cranial anatomy of Lycosuchus vanderrieti. Data Publisher: Museum für Naturkunde Berlin (MfN) - Leibniz Institute for Evolution and Biodiversity Science.
- Reisz, R. R. 1986. Pelycosauria; pp. 1–102 in P. Wellnhofer (ed.), Encyclopedia of Paleoherpetology, Part 17A. Gustav Fischer, Stuttgart.
- Reisz, R. R., D. S. Berman, and D. Scott. 1992. The cranial anatomy and relationships of Secodontosaurus, an unusual mammal-like reptile (Synapsida: Sphenacodontidae) from the early Permian of Texas. Zoological Journal of the Linnean Society 104:127–184.
- Romer, A. S., and L. I. Price. 1940. Review of the Pelycosauria. Special Papers of the Geological Society of America 28.
- Rowe, T. B. 1980. The morphology, affinities, and age of the dicynodont Geikia elginensis; pp. 269–294 in L. L. Jacobs (ed.), Aspects of Vertebrate History: Essays in Honor of Edwin Harris Colbert. Museum of Northern Arizona Press.
- Rowe, T. B. 1988. Definition, diagnosis, and the origin of Mammalia. Journal of Vertebrate Paleontology 8:241–164.
- Rubidge, B. S., and J. A. Hopson. 1996. A primitive anomodont therapsid from the base of the Beaufort Group (upper Permian) of South Africa. Zoological Journal of the Linnean Society 117:115–139.
- Rubidge, B. S., and C. A. Sidor. 2001. Evolutionary patterns among Permo-Triassic therapsids. Annual Review of Ecology and Systematics 32:449–480.
- Rubidge, B. S., and C. A. Sidor. 2002. On the cranial morphology of the basal therapsids Burnetia and Proburnetia (Therapsida: Burnetiidae). Journal of Vertebrate Paleontology 22:257–267.
- Rybczynski, N. 2000. Cranial anatomy and phylogenetic position of Suminia getmanovi, a basal anomodont (Amniota: Therapsida) from the late Permian of Eastern Europe. Zoological Journal of the Linnean Society 130:329–373.
- Sidor, C. A. 2000. Evolutionary trends and relationships within the Synapsida. University of Chicago, Chicago, 370 pp.
- Sidor, C. A. 2003. Evolutionary trends and the origin of the mammalian lower jaw. Paleobiology 29:605–640.
- Sidor, C. A. 2015. The first biarmosuchian from the upper Madumabisa Mudstone Formation (Luangwa Basin) of Zambia. Palaeontologia Africana 49:1–7.
- Sidor, C. A., and J. A. Hopson. 1998. Ghost lineages and “mammalness”: Assessing the temporal pattern of character acquisition in the Synapsida. Paleobiology 24:254–273.
- Sidor, C. A., and B. S. Rubidge. 2006. Herpetoskylax hopsoni, a new biarmosuchian (Therapsida: Biarmosuchia) from the Beaufort; pp. 76–113 in Amniote Paleobiology: Perspectives on the Evolution of Mammals, Birds, and Reptiles. The University of Chicago Press, Chicago.
- Sidor, C. A., J. A. Hopson, and A. W. Keyser. 2004. A new burnetiamorph therapsid from the Teekloof Formation, Permian, of South Africa. Journal of Vertebrate Paleontology 24:938–950.
- Sigogneau, D. 1970. Révision systématique des gorgonopsiens Sud-Africains. Cahiers de Paléontologie. Éditions du Centre National de la Recherche Scientifique, Paris, 417 pp.
- Sigogneau-Russell, D. 1989. Theriodontia I; pp. 1–127 in P. Wellnhofer (ed.), Encyclopedia of Paleoherpetology, Part 17B. vol. 17C. Gustav Fischer, Stuttgart.
- Sigogneau-Russell, D., and P. K. Tchudinov. 1972. Reflections on some Russian eotheriodonts (Reptilia, Synapsida, Therapsida). Palaeovertebrata 5:79–109.
- Smith, R. M. H., B. S. Rubidge, and C. A. Sidor. 2006. A new burnetiid (Therapsida: Biarmosuchia) from the upper Permian of South Africa and its biogeographic implications. Journal of Vertebrate Paleontology 26:331–343.
- Spindler, F. 2016. Morphological description and taxonomic status of Palaeohatteria and Pantelosaurus (Synapsida: Sphenacodontia). Freiberger Forschungshefte (C: Paläontologie, Stratigraphie, Fazies) 550:1–57.
- Sullivan, C., and R. R. Reisz. 2005. Cranial anatomy of the late Permian dicynodont Diictodon. Annals of Carnegie Museum 74:45–75.
- Watson, D. M. S. 1912. On some reptilian lower jaws. Annals and Magazine of Natural History 10:573–587.
- Watson, D. M. S. 1953. The evolution of the mammalian ear. Evolution 7:159–177.
- Watson, D. M. S., and A. S. Romer. 1956. A classification of therapsid reptiles. Bulletin of the Museum of Comparative Zoology 114:37–89.
- Westoll, T. S. 1943. The hyomandibular of Eusthenopteron and the tetrapod middle ear. Proceedings of the Royal Society B 131:393–414.
- Whitney, M. R., and C. A. Sidor. 2016. A new therapsid from the Permian Madumabisa Mudstone Formation (Mid-Zambezi Basin) of southern Zambia. Journal of Vertebrate Paleontology 36:e1150767.
- Wiley, D. F., N. Amenta, D. A. Alcantara, D. Ghosh, Y. J. Kil, E. Delson, W. Harcourt-Smith, F. J. Rohlf, K. St. John, and B. Hamann. 2005. Evolutionary Morphing; pp. 431–438 in C. T. Silva, E. Gröller and H. E. Rushmeier (eds.), Proceedings of the IEEE Visualization 2005 (VIS ‘05). Minneapolis. Institute of Electrical and Electronics Engineers, Piscataway.