ABSTRACT
Although known from more than 180 sites in North America, sites with records of multiple Megalonyx elements and individuals are rare. The Tarkio site in southwestern Iowa is unique with three contemporaneous individuals at different stages of development. Among these is one of the largest (ca. 1286 kg) and most complete adult Megalonyx jeffersonii skeletons (ca. 50%, NISP, 123) known. An intermingled sub-adult (ca. 25%, NISP, 63) represents a comparatively complete skeleton for this ontogenetic stage. A third individual, an infant known only from both scapulae (NISP 2), is commingled with sub-adult remains. Scapula sizes indicate that the two juveniles were 50 and 30% of the adult’s size. Differential bone preservation and pattern of scatter shows that the adult decomposed lying on its left side and the remains were partially scavenged. Multiple taphonomic proxies demonstrate that the skeletons were subaerially exposed for a limited period, but fluvial transport was not a factor. These individuals, preserved in the same low-energy microstratigraphic unit, apparently died concurrently and probably represent a Megalonyx social unit. Using mammal body size to estimate life history, the estimated average lifespan for M. jeffersonii was around 19 years; gestation time was about 14 months; the interbirth interval was approximately 3 years; and sexual maturation occurred at around 6.5 years. OSL analysis indicates that the sloths were deposited about 106,000 years ago (MIS substage 5ec). Paleoenvironmental proxies suggest an interglacial environment of open woodland along a stream with marshes, like the environment in southwest Iowa today.
INTRODUCTION
The primary goal of this report is to document the discovery of an ontogenetic series of three individuals of Megalonyx jeffersonii, place them in well-defined geological and taphonomic context, and argue that these individuals died contemporaneously and represent a social unit. Megalonyx represents one of four genera of Late Pleistocene North American temperate ground sloths. Megalonyx jeffersonii was the size of a modern ox, Bos taurus. The species first appeared (McDonald, Citation1977) in the late Irvingtonian (250–300 ka) and is the only species of the genus recorded during the Rancholabrean. Megalonyx jeffersonii was widely distributed, from Subarctic Canada to central Mexico and from the east to the west coasts of the U.S.A. (). It was common in mid-latitudes, even during cold glacial advances, and survived numerous glacial stades and interstades. This suggests a greater thermal tolerance than modern sloths. While common throughout the latest glacial advance including the last ones (11–35 ka) its demise (McDonald et al., Citation2015) coincided with the North American megafaunal extinction (∼11 ka).
FIGURE 1. Megalonyx jeffersonii localities in North America and Iowa. Iowa map includes county borders. Star, Tarkio Locality. Circles, Wisconsinan localities. Triangles, Sangamon localities. Black, localities recorded in Hoganson and McDonald (Citation2007). Red, localities recorded since 2007. Blue, previously undifferentiated Sangamon localities (Hoganson and McDonald, Citation2007). Numbers, localities of previously recovered Iowa Megalonyx and Paramylodon specimens are detailed in Table S1. The proximity with either the Mississippi or Missouri River drainage systems is apparent.
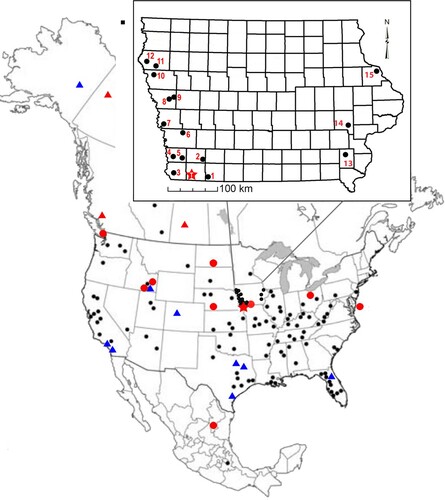
Megalonyx jeffersonii was widely distributed and its paleoecological associations were variable, but it is frequently associated with a non-analog, spruce-dominated, mixed conifer-hardwood forest present across the northern U.S.A. (Schubert et al., Citation2004; Hoganson and McDonald, Citation2007). With a hypselodont dentition composed entirely of dentine, it was a browser (McDonald, Citation2021). Megalonyx is unique among all the North American ground sloths for having plantigrade hind feet, a primitive characteristic for sloths. It is frequently reconstructed in a bipedal stance, which it probably assumed while feeding. The genus increased in body size through time with M. jeffersonii being the youngest and the largest species in the genus (McDonald, Citation2005). Possible sexual dimorphism has been proposed based on the presence of two size morphs (McDonald, Citation1977). Given time averaging, chronological size increase and wide geographic distribution of the species, the size differences within the sample also may be chronoclinal, topoclinal, or some combination. The monospecific Tarkio Site, with three contemporaneous individuals in different stages of ontogenetic development and associated paleoecological indicators, provides a unique opportunity to understand social structure and reproductive strategies of Jefferson’s ground sloth as well as its ecological parameters that have not been possible at other sites.
Although Megalonyx jeffersonii is a widespread North American taxon and known from 180 localities () including 15 from Iowa (Supplemental Data, Table S1), multi-element sites are rare. Furthermore, only two sites in North America—Big Bone Cave, Kentucky (Mercer, Citation1897) and ABc-3 Cave, Alabama (Holte, Citation2012)—have produced more than one individual. Only four other multi-element sites—Orem, Utah (Gillette et al., Citation1999); Point-of-the-Mountain, Utah (McDonald et al., Citation2001); American Falls, Idaho (Hopkins et al., Citation1969) and Pigeon Creek, Kentucky (Leidy, Citation1855)—are in stratigraphic context. All other partial skeletons are known from either cave or bog deposits.
The first comprehensive description of Megalonyx anatomy was based primarily on a partial skeleton from Pigeon Creek, Henderson County, Kentucky. The description also included individual bones from West Virginia, Tennessee, Mississippi, and Alabama (Leidy, Citation1855) and later, the sesamoids (Leidy, Citation1860). However, the paucity of relatively complete specimens with supporting paleoecological data has limited intraspecific comparisons and examinations of broader ontogenetic, sexual, chronological, ecological, and geographic trends within the taxon. Tarkio has yielded approximately one half of an adult skeleton () with 123 skeletal elements (not including 16 loose teeth) of a possible 248 skeletal elements (Supplemental Data, Table S2). It is one of the few relatively complete Megalonyx adults known and it is the second largest individual documented to date. Tarkio is the only site where an adult is intermingled in primary context with remains of two juveniles (). In addition, other Megalonyx sites have not been associated with extensive paleoecological proxies to the extent of those at Tarkio.
FIGURE 2. Element recovery schematic for the adult Tarkio Valley Megalonyx. Illustration by Meghan Mahoney, after Hay (Citation1914:fig. 1).
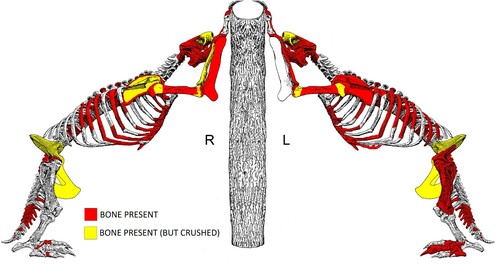
FIGURE 3. Right scapula relative sizes for the Tarkio Valley infant, sub-adult and adult. Scale is 10 cm.
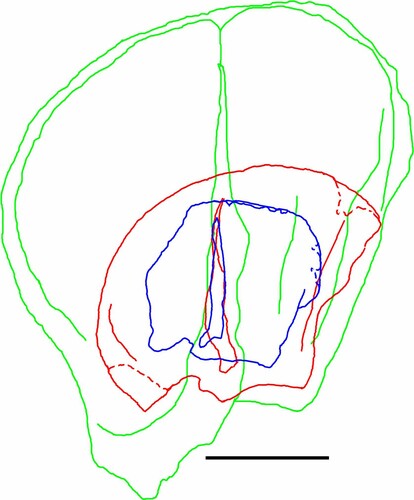
At ACb-3 Cave, the original excavators reported as many as 16 individuals (Shaver et al., Citation2006). Subsequent analysis (Holte et al., Citation2010; Holte, Citation2011) confirmed the remains of seven individuals (one complete skeleton plus six partial skeletons). Holte (Citation2012) later suggested that the cave served as a thermal buffer during winter and/or a maternity den for M. jeffersonii. The specimens probably became entombed between 170,000 and 115,000 BP based on U-series dates recovered from associated travertine deposits (Lively et al., Citation1992; Holte, Citation2012:36), roughly contemporaneous with the Tarkio group. Hence, the ABc-3 cave individuals probably represent a time transgressive accumulation. The assemblage at Tarkio appears to represent a single event. Big Bone Cave, Van Buren County, Tennessee includes three individuals of M. jeffersonii, each recovered from a separate passage and exhibiting differential preservation. One is the type specimen for Harlan’s (Citation1831) Megalonyx laqueatus, now considered a junior synonym of M. jeffersonii (McDonald, Citation1977). Safford (Citation1892) described another partial skeleton collected in a separate location within the cave, while Mercer (Citation1897) published on a third individual from yet another passage. Hence, none of the Big Bone Cave individuals are associated.
Partial skeletons of juvenile Megalonyx jeffersonii are currently known from four localities: Crankshaft Cave, Missouri (Parmalee et al., Citation1969) (ISM 499310, 15 MNI); Devil’s Den, Florida (Martin and Webb, Citation1974) (UF 9300, 10 MNI); Warm Mineral Springs, Florida (McDonald, Citation1977) (UF 170700, ∼30 MNI); and ACb-3 Cave, Alabama. At ACb-3 Cave there are three juveniles of differing ontogenetic stages: an infant (RMM 6353, cranium and 23 postcranial elements), a juvenile (RMM 5430, 14 cranial bones and the left dentary), and a sub-adult (RMM 6389, cranial elements and eight postcranial elements) (Holte, Citation2012). The presence of three different ontogenetic stages preserved in a single time horizon at the Tarkio site is a substantial addition to the adolescent M. jeffersonii record and provides insight into the reproductive strategy of the species.
MATERIALS AND METHODS
Fossil Assemblage
Location—The Tarkio site () is in the valley of the West Tarkio Creek, Page County, Iowa, 9.7 km southwest of Northboro. The West Tarkio joins the East and Central branches of the Tarkio basin to form the Tarkio River, a tributary of the Missouri River. The precise location of the site is recorded in the Paleontological Repository, Department of Earth and Environmental Sciences, the University of Iowa. A fifth metacarpal of Paramylodon harlani, reported by McDonald (Citation2012), discovered on a gravel bar 20 m downstream from the Tarkio locality, cannot be referred to the Tarkio M. jeffersonii locality.
Discovery—Exposure of the sloth locality began in 1917 when Page County began straightening the West Tarkio Creek (Supplemental Data, Fig. S1) to eliminate meanders from farm fields (Woida, Citation2006). Down cutting began immediately, and the creek bed dropped 7 meters, reaching the current grade in 1975 when lateral migration resulted in a cut bank to the southeast (Athen property). When water from the century flood of 1993 receded, the northwest bank (Tiemann property) became the cut bank, resulting in the sloth’s exposure.
In 2001, Bob Athen noticed an unusual round object in the creek bed and excavated a femur. He brought it, along with several smaller bones to the University of Iowa for identification. Co-author Semken recognized these as M. jeffersonii, noted the co-occurrence of large and small elements, and believed that the remains represented a primary deposit. A site visit revealed several trunk elements embedded in a blue-gray clay, and permission was granted by both landowners to collect the remaining parts of the skeleton. Excavation was difficult because the bone scatter was either in the creek bed or under 2–3-meter-thick slump blocks, which undoubtedly assisted preservation, or beneath the 7-meter-high northwest bank of Tarkio Creek evident in the aerial photo (Supplemental Data, Fig. S1).
Excavation—Excavation protocol was dictated by the most efficient way to relocate the creek and always occurred behind a levee, usually constructed of excavation matrix over a previously excavated trench-like block. Excavation block boundaries were not as sharp as those depicted in , but care was taken to ensure that each overlapped. Excavation block numbers are designated by the prefix MM to correspond to entries (Semken and Brenzel, Citation2010) in the excavation journal, “Megalonyx Matters” (Supplemental Data, Appendix S1) and are sequential for each enlargement of the quarry. The site was excavated in approximately 3-meter-wide blocks with elements mapped by triangulation from iron benchmarks positioned at the front of the southwest and northeast corners of MM-7. Water-screening of 10 grab samples (0.5 m3 each) from the first three excavations did not yield any microvertebrates and screening was discontinued in favor of selective flotation. Horgen et al. (Citation2007) provide a brief history of the discovery and a synopsis of excavation sequence can be found in Mahoney et al. (Citation2009).
FIGURE 4. Bone distribution map for the Tarkio Valley Megalonyx locality. Elements without icons: Adult: A, atlas; CL, clavicle; F, femur; H, humerus; M, mandible; P, palate (half); PE, pelvis; R, radius; S, sternebra; SK, skull; SR, sternal rib; T, tibia; U, ulna. Juvenile: cl, clavicle; h, humerus; pe, pelvis. Other: r, rodent humerus; t, turtle (pond); ts, turtle (snapping). MM refers to both the excavation block and the online excavation journal Megalonyx Matters (Supplemental Data, Appendix S1). Missing MM numbers on the map relate to journal entries for heavy equipment days or planning session notes. Elements are sided on the paleontological repository inventory (Supplemental Data, Table S1).
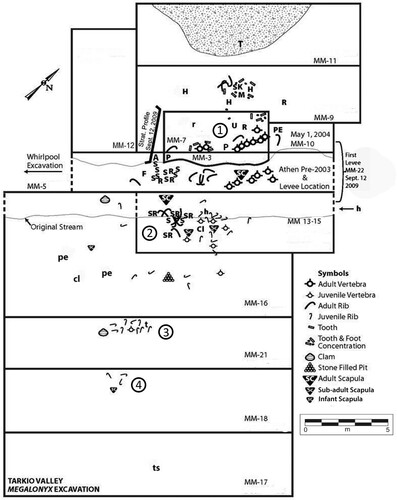
Curation—The three Tarkio individuals are curated in the Paleontological Repository, Department of Earth and Environmental Sciences, University of Iowa, Iowa City, Iowa, U.S.A., and each has a unique catalog number, Adult, SUI 101037; Sub-adult, SUI 102849; and Infant, SUI 130544. Individual elements for each are identified by an alphabetical suffix (Supplemental Data, Table S2) and photographs are freely available at Iowa Research Online (see instructions in Supplemental Data, Appendix S3). Descriptions and links to these datasets are in Supplemental Data, Appendix S3. Some sub-adult and infant remains, other than the scapulae, are indistinguishable and cataloged with the sub-adult. Hard copies of Megalonyx Matters, the excavation journal (Supplemental Data, Appendix S1) and all project resources, including excavation photographs, are cataloged in the repository. Most are available on the University of Iowa Paleontological Repository network drive (Supplemental Data, Appendix S3). The term “juvenile” was used for the Sub-adult during excavation and primary Tarkio Valley research. Juvenile was changed to Sub-adult (SUI 102849) at a late stage of the project. Likewise, the Infant (SUI 130544) was initially referred to as “baby.” These synonyms are common in repository records.
Institutional Abbreviations—ISM, Illinois State Museum, Springfield, IL, U.S.A.; RMM, Red Mountain Museum, Birmingham, AL, U.S.A.; SM, Sanford Museum, Cherokee, IA, U.S.A.; SUI, University of Iowa Paleontological Repository, Department of Earth and Environmental Sciences, Iowa City, IA, U.S.A.; UF, Florida Museum of Natural History, University of Florida, Gainesville, FL, U.S.A.; UIC, University Illinois, Chicago, IL, U.S.A.; UIMNH, University Iowa Museum of Natural History, Iowa City, IA, U.S.A.; USDM, University of South Dakota Museum, Vermillion, SD, U.S.A.; USNM, United States National Museum of Natural History, Washington, D.C., U.S.A.
Geological, aDNA Analysis, and Paleontological Methods
aDNA—Five bone and tooth fragments were submitted to the Ancient DNA Centre at McMaster University to sequence, following established aDNA extraction protocols (Rohland and Hofreiter, Citation2007) and PCR amplification using xenarthran/sloth specific primers. None produced adequate samples for analytical results. To potentially enhance recovery, the adult astragalus (SUI 101037 BH), a large, dense bone recovered away from the modern stream, was cored for large scale aDNA extraction followed by a concentration step on Microcon filters to reduce 15,000–20,000 μl of extract down to 200 μl. No sloth amplicons were produced. The right scapula of the infant Tarkio sloth (SUI 130544A) is stored in the original field jacket and refrigerated to assist future aDNA analysis. Analytical details are in Supplemental Data, Appendix S4.
Geological Cores—Two cores were taken with a Giddings Model HDST trailer mounted drilling rig. In order to tie the sloth-bearing unit into the local stratigraphy, the cores were taken on both sides of West Tarkio Creek (Supplemental Data, Fig. S1). Core SL2B (; Supplemental Data, Appendix S5), was initiated on floodplain deposits above the northwest bank (MM-13). The second core, SL-1 (; Supplemental Data, Appendix S5), was located on a loess-mantled upland terrace remnant about 45 m east on the opposite (southeast) bank. The cores were described while still moist, indoors under full spectrum light and are based on a 3-inch diameter core from 0–660 cm, and a 2-inch diameter core from 660 cm to base (Supplemental Data, Appendix S5). Grain size, organic carbon, and stable carbon isotope samples were collected from the lower few meters of each core (). Grain-size analyses were performed on sedimentary units in the cores at the University of Iowa’s Quaternary Materials Laboratory using a modified version of the pipette method (Soil Survey Staff, Citation1999). Total organic carbon and carbon isotope analyses were performed on finely ground and decalcified samples at the University of Kansas’ Keck Paleoenvironmental and Environmental Stable Isotope Laboratory using a Costech 4010 elemental analyzer (EA) to combust and measure sample carbon content, and a linked Thermo Finnigan MAT 253 IRMS for isotope analysis. The results, especially the stable carbon isotopes (C3/C4 ratios) of blue-gray clay equivalents, are discussed with the paleobotanical analysis.
FIGURE 5. Generalized stratigraphy of cores drilled on either side of West Tarkio Creek, Tarkio Valley Megalonyx locality. Core SL-1 was drilled on the eastern loess-mantled terrace and did not penetrate to the level of the sloth-associated deposits. Core SL-2B was drilled on the West Tarkio floodplain and penetrated deposits likely correlative with the sloth-bearing alluvium in the creek bed excavation. Dashed line connecting stratigraphy in the two cores is inferred correlation of buried soil horizon. Radiocarbon and OSL ages are discussed in the text.
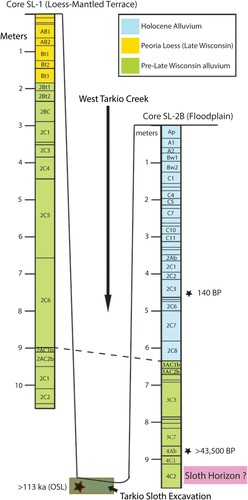
FIGURE 6. Grain-size, total organic carbon and carbon isotope profiles for the lower 6 meters of core SL-1 and SL-2B. A, core SL-1 (Southeast Bank); B, core SL-2B (Northwest Bank). Lower zone of core SL-2B inferred to correlate with sloth-bearing deposits in the creek bed is highlighted in red. The buried soil (2AC1b, 2AC2b) located at 9 meters depth is inferred to correlate with the buried soil (3AC1b, 3AC2b) between 6- and 7-meters depth in core SL-2b.
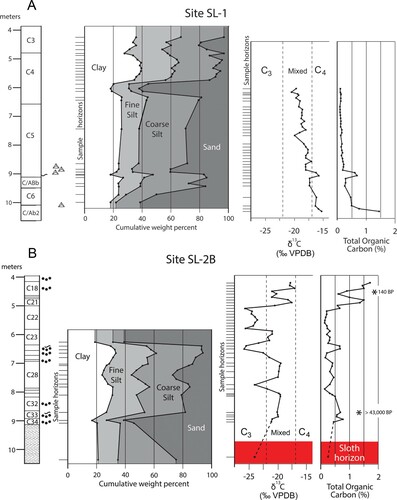
Optically Stimulated Luminescence (OSL)—OSL ages were performed at the S.L. Forman, Luminescence Dating research laboratory, Department of Earth and Environmental Sciences, University of Illinois-Chicago on quartz grains collected immediately below the bone bed (Supplemental Data, Table S3). Josephs (Citation2009) published descriptions of two samples of the blue-gray, sloth-bearing matrix, one adhering to a vertebra and the other to the sub-adult clavicle, using the technique devised by Josephs and Bettis (Citation2003).
Taphonomy—Various methods have been devised to quantitatively compare osteological preservation in faunal assemblages (e.g., Lyman, Citation1994a). Percent preservation was calculated by dividing the number of bones preserved in a skeletal group (e.g., forelimbs) by the total number of bones expected in a complete skeleton and then multiplying by 100. Data for the adult are finite, but because it is possible that a few of the ribs assigned to the sub-adult may belong to the infant, these numbers may be slightly biased against the infant.
To assess the potential of fluvial transport, the three bone group classifications (I, II, and III) of Voorhies (Citation1969) were employed. For bone modifications, each specimen was examined without magnification and bone modification surveys are ongoing. Weathering stages were categorized after Behrensmeyer (Citation1978). Bone surface modifications were classified after studies by Hill (Citation1989) and Haynes (Citation1980, Citation1983). Bone fracture types were adapted from Marshall (Citation1989).
Rare earth element (REE) geochemistry has been used for several decades to determine relative age, provenance, degree of reworking, and depositional environment in paleontological deposits (Elderfield and Pagett, Citation1986; Trueman and Benton, Citation1977; Truman et al., Citation2006; MacFadden et al., Citation2007; Suarez et al., Citation2010). A series of REE samples were collected from a rib of the adult Megalonyx jeffersonii (SUI 101037 CC). The rib was recovered medial side up and progressively weathered from the proximal to distal end. It was divided into seven segments with each assigned a weathering stage based on Behrensmeyer’s (Citation1978) six-stage scale. Zero represents the least weathered bone and five indicates the most weathered, highly fragmented bone. After assigning weathering stages (segments A, B and C: 0.5; segment D: 3, segments E and F: 4, segment G: 5), powdered samples were taken from both the medial and lateral sides of the rib and analyzed by inductively coupled plasma mass spectrometry (ICP-MS).
Ontogenetic Ages—Three different ontogenetic stages of the Tarkio Megalonyx were identified based on size differences of the scapula. Holte (Citation2012) used the terms infant, juvenile, sub-adult, and adult to describe four stages of ontogenetic development of specimens from ACb-3 Cave. We have applied infant, sub-adult, and adult to the Tarkio specimens and refer to the younger specimens collectively as juveniles. We do not infer comparable developmental stages for these terms between ACb-3 and Tarkio.
Paleobotany—Of five pollen samples examined from the site, only the sample associated with the adult pelvis produced palynomorphs. Each was collected because of direct association of another proxy. This sample yielded eight identifiable taxa. Each of three plant macrofossil samples yielded identifiable taxa. The most diverse macrofossil sample, which was collected beneath the adult pelvic complex, included 16 taxa. A second plant macrofossil sample taken directly from SU-1, the historic channel (), produced 10 taxa, none of which were associated with the pelvis sample in SU-3. Another sample, collected in SU-3 with the mollusk concentration (), only produced two taxa and documents that plant macrofossils, as the mollusks and pollen, are not uniformly distributed in the sloth-bearing deposit.
Plant macrofossils (Supplemental Data, Table S4) were collected using a slightly modified Dausman Flote-Tech A1 flotation system. Identification of these was based on comparative collections in the Paleontology Repository, University of Iowa. Mollusk identifications were completed with comparative specimens in the Mississippi Valley Archaeology Center, University of Wisconsin-La Crosse, Wisconsin.
Stable Isotopes—Four different dentine layers of an adult lower molariform (SUI 101037 X) were examined by SEM and optical/X-ray at the Pennsylvania State University. Eight equally spaced samples from the occlusal end were drilled from each of the four layers of the longitudinally sectioned tooth (SUI 101037 X) for isotopic analyses. These were augmented by 15 samples from dentine layer one (labial) and nine samples from dentine layer four (lingual). Samples were taken along two transects within observable (presumed) growth increments. The 56 samples were processed for carbonate carbon and oxygen isotope ratios. Multiple samples were necessary to capture the internal variability between the four dentine layers.
STRATIGRAPHY AND SEDIMENTOLOGY
Regional Stratigraphy
Core SL2B transgressed Late Holocene alluvium to a depth of 4.64 m. Wood from the base (4.45–4.55 m and within the Late Holocene alluvium) was dated to 140 ± 40 RCYBP (Beta-297998), consistent with the arrival of Euro-American settlers and widespread plowing in SW Iowa. The alluvium is underlain by a weakly expressed soil formed in an earlier Holocene alluvium that extended to 6.45 m. Pre-late Wisconsin alluvium continues to the base of the core at 9.47 m. Deposits lithologically like the silty, blue-green, sloth-bearing matrix (hereafter the blue-gray clay) appear at 9.12 m and extend to the core bottom. A radiocarbon date on wood collected within the pre-late Wisconsin organic-rich clay loam (8.66–8.92 m) and blue-gray equivalent exceed 43,500 RCYBP (Beta-297999). Deposits at the base of core SL-2B (floodplain location) appear to correlate with the fine-grained sloth-bearing, blue-gray alluvial sediments of stratigraphic Sub-Unit 3 described below. These are overlain by an upward-fining sequence that has a buried AC soil profile formed in its upper part (3AC1b and 3AC2b). The base of the overlying Holocene alluvial sequence is marked by a sharp increase in sand.
Core SL-1 transected 1.84 m of late-Wisconsin Peoria Loess to a truncated soil formed in pre-late Wisconsin alluvium that extends to the base of the core at 10.65 m, topographically above the level of the sloth-bearing matrix. The analyzed interval of core SL-1 (upland location) is entirely within what we interpret as pre-Wisconsin alluvium and did not reach sufficient depth to encounter sloth-bearing, blue-gray clay correlatives but did traverse a buried AC soil profile (2AC1b, 2AC2b) that apparently is correlative with the buried soil formed in pre-Wisconsin alluvium in core SL-2B (). The carbon isotopic profiles generally indicate mixed vegetation in the pre-Wisconsin alluvium with slightly more contributions from C4 vegetation in core SL-1. This is typical of floodplain settings today where riparian forests line the active channel belt in a landscape with significant grasslands on uplands and broad terraces.
Intra-site Stratigraphy
Excavations in November 2009 created a stratigraphic profile within the blue-gray clay. The profile (), located just west of the sloth bone concentration (), exposed three stratigraphic sub-units (SUs) within the unit, described here in ascending stratigraphic order.
FIGURE 7. A, intrasite profile showing relationship between Sub-Units 2 and 3 (SU2 and SU3). (Scale bars are 1 m (horizontal) and 0.5 m (vertical) long. B, detail of discontinuous, reddish-brown sand laminations in lower part of profile (scale bar in 1 cm increments). C, detail of profile in A, showing redoximorphic bands that trace trough-cross-bedding in SU2 (alternating red and black portions of scale are 10 cm long).
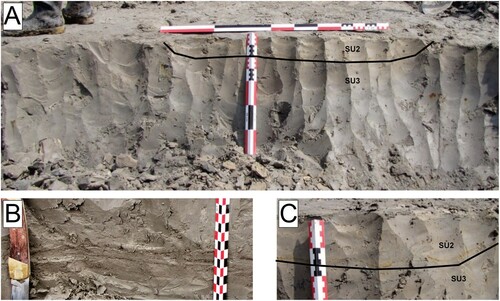
Sub-Unit 3—This SU is a bluish gray silt loam. The sediment matrix has sufficient very fine sand that it will not ribbon. Also present are laminations and lenses of fine to medium sand with rare pebbles. No sloth bones were observed in the profile, but SU-3 is interpreted as the primary sloth-bearing deposit. SU-3 is also inferred, based on lithology, to be correlated with the basal 4C horizon in Core SL2B, floodplain location (), described as bluish gray (5B 5/1) silt loam (Supplemental Data, Appendix S4).
Sub-Unit 2—SU-2 is a laminated grayish-brown silt loam that appears to fill a deep trough (1.5 m wide × 0.3 m deep) inset unconformably into SU-3 (). Redoximorphic concentrations occur as thin, wavy bands, perhaps representing bedding planes. SU-2 was observed in the northwest part of the excavations (, MM-9, 11, and 12), filling shallow troughs (3 cm deep × 3–5 cm wide) eroded into SU-3. The troughs appear to have converged into an area of coarse pebbly sand in the northwestern-most excavation (, MM-11). The distal tibia of the adult sloth and a bone fragment sufficiently large to be from the sloth were embedded in this pebbly sand and suggest that there was minor reworking of the remains shortly after burial.
Sub-Unit 1—SU-1 is a laminated blue-gray loam to a very fine, sandy loam. The unit was poorly exposed at a location north of the profile, as it is overlain by excavation overburden.
It yielded small, reworked, relatively unweathered, modern-looking bone fragments, bits of wood, and a whiteware ceramic fragment of Euro-American origin.
Sub-Unit 3 is interpreted as overbank alluvium deposited by relatively slow moving or still water, an inference supported by soil micromorphology (Josephs, Citation2009 and see below). SU-2 is interpreted as a relatively short-lived flood event that eroded into SU-3 and, in MM-11, resulted in the localized reworking of a few sloth bones from SU-3. SU-1 is interpreted as historic alluvium deposited by the West Tarkio Creek in its deeply entrenched channel. It may represent the reworking of SU-3 and SU-2 sediments by water flowing over the sloth-bearing deposit.
Micromorphology of the Blue-Gray Clay
Josephs (Citation2009) published descriptions of two samples of the blue-gray, sloth-bearing matrix (SU-3), one adhering to an adult vertebra and the other to the sub-adult clavicle, using the technique devised by Josephs and Bettis (Citation2003). The thin-section analysis of the composition, texture, and fabric revealed the blue-gray clay was a dense, loess-based, silty loam with rare (<5%) clasts of very fine to coarse clasts with a granitic composition. The angular sand particles exhibited a moderate horizontal orientation consistent with microstratigraphic fluvial evidence exhibited by the deposit. Elongated bone microchips also exhibited this orientation. Sand-sized particles are composed of till-derived granitic minerals. The micromorphological evidence suggests that the Tarkio Megalonyx remains were originally buried by fluvially redeposited loess under low-energy flow.
Radiometric Age of the Tarkio Megalonyx-bearing Deposits
The sloth locality was originally regarded as late Wisconsin (MIS 2–3, 14–57 ka, MIS 4, 71 ka inclusive), an age based on dates recorded for other regional Late Pleistocene mammals and the locality’s apparent association with overlying Holocene deposits (Ray et al., Citation2013). Two Optically Stimulated Luminescence (OSL) ages (Brown and Forman, Citation2012) obtained on quartz grains in the sand immediately under the sloth-bearing blue-gray silty clay (SU-3), produced dates of 113 ka (UIC 3109) and 100 ka (UIC 3110) and average at 106 ka (Supplemental Data, Table S3) indicating that the sediments enclosing the sloth remains are slightly more than 100,000 years old (∼MIS 5c) and are Sangamon Interglacial in age (MIS 5, 57–130 ka) and part of the early Rancholabrean North American Land Mammal Age.
SYSTEMATIC PALEONTOLOGY
Order XENARTHRA Cope, Citation1889
Family MEGALONYCHIDAE Gervais, 1855
Genus MEGALONYX Harlan, Citation1825
MEGALONYX JEFFERSONII Desmarest, Citation1822
Note—Labeled images of all specimens of M. jeffersonii recovered as part of this study are available as stable datasets through the Iowa Digital Library. Digital Object Identifiers, links to image datasets, and file descriptions are available in Supplemental Data, Appendix S2.
Description—The cranium (SUI 10137 DK) is fragmented but includes the lingual alveolar margins of the palate and the posterior portion of the braincase. Portions of the right (SUI 10137 AX) and left (10137 AW) mandibles, including the mandibular condyles, and mandibular symphysis (SUI 10137 AY) were also recovered. The upper (SUI 10137 AF and BV) and lower (10137 BL and U) caniniforms both have diagnostic Megalonyx morphology (Leidy, Citation1855; Stock, Citation1925; McDonald, Citation1977). The caniniforms of Megalonyx differ from other megalonychids by having an ovate cross section with a lingual column clearly separated by a mesial and distal sulcus and a flat, crushing occlusal surface worn perpendicular to the axis of the tooth. Other megalonychids generally have a caniniform with a triangular cross section and the occlusal surfaces worn either parallel or oblique to the axis of the tooth.
In the postcranial skeleton, the adult exhibits the distinctive calcaneum and astragalus characteristic of the plantigrade feet of the genus Megalonyx. There is only one postcranial element that distinguishes M. jeffersonii from other species, a co-ossified proximal and second phalanx of digit 3 of the pes. The left of this element (SUI 101037 BG) was recovered at Tarkio. The 123 recovered elements can all be referred to one individual (). The presence of a single adult is substantiated because many of the bones of the left pes and the rib cage, especially the sternebrae and ossified sternal ribs, readily articulate, and because none of the adult elements are repeated.
TABLE 1. Tarkio Megalonyx Skeleton Inventory and preservation percentage for the adult and sub-adult skeletons. The infant is only known from the left and right scapulae. Does not include ear ossicles (6), hyoids (7), manus sesamoids (16), lunale and cyamelle sesamoids (4), pes sesamoids (12). The numbers in parentheses represent the number of identified elements (NISP). Some infant elements may be present.
The mature status of the adult is supported by the presence of parallel-sided teeth, complete obliteration of the epiphyseal lines in all limb epiphyses and the anterior portion of the skull, and well-developed limb muscle scars. However, the parietal/occipital suture is still open and indicates that the posterior portion of the cranium matures late in M. jeffersonii. Grass (Citation2019), in a morphometric study of ground sloth scapulae, documents that the Tarkio juveniles are those of Megalonyx.
In an attempt to support species identification, six samples, including the concentration from the astragalus, were analyzed for aDNA. None of the samples produced unique sloth amplicons with available primers.
THANATOCOENOSIS
A single carapace fragment each for a softshell turtle (Apalone), snapping turtle (Chelydra), and an unidentified pond turtle are the only other vertebrates recovered from the thanatocoenosis. Thus, Tarkio is essentially a monospecific, multi-individual Megalonyx jeffersonii association within a single depositional unit (SU-3, blue-gray clay). Three distinct Megalonyx individuals of different ontogenetic stages (adult, sub-adult, and infant) are preserved. Remains of the three sloths were distributed in four disarticulated, overlapping, or juxtaposed clusters () along a northeast-southwest strike. Cluster 1, primarily the remains of the adult, is in the northeast portion of the site (). The adult’s anterior skeletal elements are toward the north. The remains, except for two distended thoracic vertebral strings, are jumbled and appear to be randomly distributed. This is especially evident in the orientation of the adult ribs, which can be up to 180° out of phase with each other (two rib pairs in MM-9, five in MM-13, two in MM-16, three in MM-21, and one in MM-18; ). Except for the left femur, five caudal vertebrae, three right foot bones, and a scattered but complete left foot, post synsacral elements are missing. Differential preservation favoring left elements () suggests that the adult decayed while lying on its left side. The sub-adult remains, scattered primarily in Cluster 2, were immediately adjacent to and partially overlapping those of the adult in a more widely distributed scatter to the southwest (, MM-13). The left infant scapula (SUI 130554 B) is in this cluster. Cluster 3 is about 3 meters southwest of Cluster 2 and consists of nine juvenile ribs, a sub-adult vertebra and tibia. Cluster 4 is composed of three juvenile ribs and the infant right scapula (SUI 13054 A). This suggests that some ribs cataloged with the sub-adult inventory may belong to the infant. The two infant scapulae (SUI 130554 A and B) are separated by about 9 meters.
When the sub-adult trunk elements are reassembled (), a single size class of juvenile elements is apparent suggesting that most, if not all juvenile ribs, belong to the sub-adult. It is common for the remains of infants to be poorly represented in the fossil record because infant bones are typically incompletely ossified. Trampling, predation, scavenging, fluvial removal, etc. also factor into their destruction. Stiner et al. (Citation2012) observed that mountain lions (Felis concolor), and presumably other large carnivores, frequently devour all the bones of young individuals.
FIGURE 8. Composite sub-adult trunk assembly suggests that most of the ribs belonged to the subadult. The scale bar for the rib cage is 20 cm. The infant scapula is to the upper right, the sub-adult to the lower left. The enlargement of the remodeled puncture wound in rib SUI 102849L documents past trauma. The punctured rib is noted by a bracket. The scale bar on the latter is 10 mm.
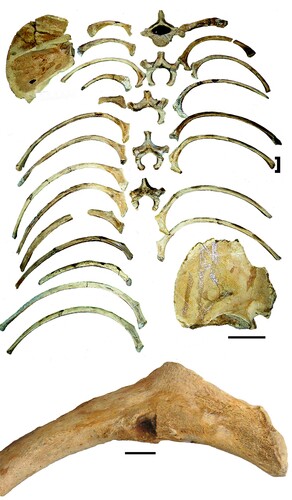
TAPHONOMY
Fluvial Sorting and Re-deposition
Voorhies (Citation1969) Group I, composed of lighter, flatter bones, is the first assortment of skeletal elements normally removed hydrodynamically. For the adult, the Group I percent preservation () is ribs (58%) with sternal ribs at 63%, vertebrae (cervical = 14%, thoracic = 39%, lumbar = 33%, sacrum = 100%, caudal = 33%), sternebrae (89%), patella (0%), scapulae (100%), manus (21%), and pes (ca. 30%). Group II is composed primarily of limb bones. Tarkio yields: forelimbs (83%), hind limbs (17%), and pelvis (100%). Group III, lag elements, are represented by the cranium and mandibles and are present at Tarkio. All three Voorhies Groups are well represented and document that fluvial transport, except for post-depositional channeling (Sub-Unit 2), only had minor, if any, impact on the adult. The sub-adult distribution, based on the proposed reconstruction of the sub-adult skeleton (), is largely represented by Group I trunk elements with 61% rib preservation. Twenty-seven percent of the thoracic vertebrae were preserved, both scapulae and only one each of the clavicles and ilia (50%). All other heavier elements are missing. It is notable that fluvial action was sufficient to remove the rib epiphyses but not the body. Only two disarticulated vertebral epiphyses (SUI 102849 N and W) were recovered.
The dominance of Group I elements and only two Group II sub-adult elements and no group I elements suggest that the missing sub-adult remains are not a reflection of fluvial action. The infant, known only from its two scapulae, may have been subject to fluvial removal. However, the presence of adult and sub-adult elements cannot be attributed to fluvial activity because Group I elements are largely present (). This interpretation is enhanced by random orientation of the elements (). This interpretation is supported by site microstratigraphy and soil micromorphology, both of which reflect still- or slow-water movement.
Incremental internment also is supported by fine sand and silt laminations within the blue-gray clay (SU-3), progressive adult rib deterioration (REE), finger clams that specialize in environments that periodically dry, and orientated microparticles indicative of slow-moving water. All suggest burial in slowly aggrading deposits characteristic of floodplains. All transport groups are present, but there was some post-depositional fluvial entrenchment (, MM-11). This is documented by the presence of the distal portion of the adult tibia (SUI 101037 DI) and the only sub-adult limb bone recovered (distal humerus, SUI 102849 K; Supplemental Data, Appendix S2) in a shoe-string sand (SU-2) cut into the sloth-bearing matrix (SU-3).
However, while the orientation of the individual elements was random, the individuals tended to cluster () with the adult dominant in cluster 1, the subadult in cluster 3, and the infant in cluster 4. Adult and subadult remains overlap in cluster 2. The adult cranium and mandible (Voorhies Group III) were located toward the north; with the appendicular elements (Voorhies Group II) generally distributed immediately south in Cluster 1 (). Voorhies Group I elements (ribs, vertebrae) were well represented but more widely distributed with three adult ribs located north of the cranium (, MM 9). While clustered but not imbricated, the bones clearly were re-arranged postmortem. As previously noted, this is especially evident in the orientation of the adult ribs which can be 180° out of phase with each other. Although orientation data were not quantified, absence of preferred alignment (Voorhies, Citation1969) tends to negate hydrological sorting (). Portions of the juvenile remains possibly drifted on skin rafts because the right scapula was deposited lateral side up and the left lateral side down for both the sub-adult and infant.
Weathering and Fractures
Intermingled adult dental and foot elements, rodent gnawing (Supplemental Data, Fig. S2), palate fragments separated by 2 meters (6.6 ft), dry fracture and each of Behrensmeyer’s (Citation1978) weathering stages demonstrate that the remains were variously exposed subaerially. Small and flat adult bones and some limb bones, e.g., right ulna SUI 101037 BP and left radius SUI 10137 BY, are well-preserved (Supplemental Data, Appendix S2). Larger elements (e.g., humeri, SUI 101037 DQ and DR), with rare exception (femur, SUI 101037 BR), exhibit extensive dry-bone transverse fractures (e.g., Marshall, Citation1989). Proximal and distal portions of the fused long bone epiphyseal regions are fractured and slightly offset from their shaft. The largest element, the synsacrum (SUI 101037 BS), was totally decomposed (Behrensmeyer, Stage 5) except for the acetabular regions and encapsulated in a 10 cm (3.9 in) vertical unit, which suggests trampling.
The cranium (SUI 101037DK) and mandibles (SUI 101037 AW and AX) suffered substantial weathering with rounded-bone rubble zones separating fractures, which is indicative of trampling rather than sediment compaction. Although the adult is not bone density modified (Lyman, Citation1994b:235–258), the two juveniles are with the appendicular elements almost wholly missing. This distribution is typical of scavenging. Also, the randomly distributed juvenile remains, which only exhibit sporadic dry fractures, are characteristic of trampling (Fiorillo, Citation1989). The preservation of clusters indicates that subaerial exposure was not extensive, at least for most of the adult and sub-adult skeletons.
Bone Surface Modifications
Punctures, Grooves, Abrasion, Striations, and Polish—The only observed modification is a healed puncture wound () on the interior surface of the sub-adult rib that is likely from a pre-mortem encounter with a carnivore. Lack of modification on all other Tarkio bones may seem enigmatic for scavenged carcasses. However, Faith (Citation2007) found that the amount of bone modification and destruction by scavengers in hyena dens is a function of the availability of meat. If meat is abundant, then scavengers may ignore bones. Similarly, Mech and Peterson (Citation2003), as well as Peterson and Ciucci (Citation2003), found the same relationship between wolves and white-tailed deer remains. If availability is limited, then scavengers gnaw for further nourishment. With three juxtaposed dead sloths, food was likely abundant. In a study of landscape taphonomy of Bison carcasses in western South Dakota, Milideo (Citation2015) found that as many as 50% of the scavenged bison carcasses did not exhibit any bone surface modification.
Preferential Bone Preservation and Scavenging—Most terrestrial carnivores process carcasses in a systematic pattern (Haynes, Citation1982; Blumenshine, Citation1986). They first open the visceral cavity and consume the internal organs, a rich source of protein with minimal energy expended by the scavengers. They next begin eating the carcass from the posterior towards the anterior end. Again, this is a function of the distribution of meat with the hindquarters usually the more muscular portion of the carcass. This pattern may explain the differential preservation favoring anterior as opposed to posterior skeletal elements at Tarkio (; ).
Limb elements are frequently removed from the rest of the carcass so that the scavengers can eat without competition or for transportation to den sites (Binford, Citation1981). and document the loss of posterior skeletal elements as characteristic of scavenging. The lack of most limb bones of both juveniles favors removal by scavengers (Haynes, Citation1982), especially since these bones are much lighter than those of the adult but are too heavy for removal by fluvial activity, as noted previously.
For completely scavenged carcasses, skeletal elements that contain less meat or nutrient value (e.g., ribs, pelvis, vertebrae, trunk, and cranial elements) are left at the site. The primary preservation of trunk and cranial elements for all Tarkio individuals is consistent with scavenging (Blumenshine, Citation1986). The only partially articulated elements excavated consisted of six thoracic vertebrae in near life position (, MM 7) and six additional thoracic vertebrae from a disarticulated but linear series in the creek bed collected by the Athens (). Thus, the vertebral column, with its elastic, sheath-like connective tissue, was the last portion of the skeleton to become dismembered, as is typical of a scavenged carcass. The survival of the anterior limb elements without bone surface modifications may be indicative of incomplete scavenging resulting from an overabundance of meat.
Rodent Gnawing—Only two bones from the Tarkio adult exhibit rodent gnawing, a right rib (SUI 101037BT) and the acetabular region of the synsacrum (SUI 101037 BS; Supplemental Data, Fig. S2). Both are evidence that rodents were present and that some elements of the adult skeleton were sub-aerially exposed for a time. The absence of gnawing on the sub-adult remains could be a result of more rapid burial of smaller skeletal elements. Incremental burial is supported by site microstratigraphy, and progressively weathered ribs and REE uptake (below).
Rodent gnawing may also serve as a paleoenvironmental proxy. Guthrie (Citation1990:216–217) observed that in more arid environments, e.g., grasslands, where plants are mineral-rich, rodents do not exploit bones. In more mesic conditions, forests, and perhaps tall-grass prairie as well as mesic tundra, plants are mineral-depleted, and rodents gnaw skeletal elements to make up for dietary mineral deficiencies (Guthrie, Citation1990:217). Tappen (Citation1994) similarly found that in the tropical Ituri rain forest, the frequency of rodent gnawing is greater than in the savannas of Parc National de Virungas. Even though Brain (Citation1981) suggested that rodent gnawing was common in savannas, Tappen (Citation1994:671) rejected this, except perhaps for porcupine lairs, because savanna frequency did not approach that of the Ituri rain forest. Even though rodent gnawing at Tarkio is sparse, its presence is consistent with an open woodland and marsh biota as suggested by carbon isotopes, plant macrofossils, stable isotopes, and pollen (see below).
Paleopathology
The adult skeleton exhibits two pathologically ankylosed caudal vertebrae (SUI 10137 CN; ). The complete caudal series was not recovered so it is not possible to precisely determine the location of these vertebrae within the series of about 18 caudal vertebrae. Based on their size, general morphology, and development of the transverse processes, they are probably from the proximal third of the sequence. A CT scan of the specimen () indicates that the infection is confined to the external surface and does not extend internally. The primary locus of the pathology is on the ventral margin of the centra of the two adjacent vertebrae including the hemal arch. There is a pseudarthrosis between the transverse processes of the vertebrae, which is not normally present.
FIGURE 9. Diseased and fused caudal vertebrae (SUI 101037CN) of the Tarkio adult, proximal to the top. A, dorsal; B, ventral; C, computerized tomography (CT) dorsal; D, CT ventral.
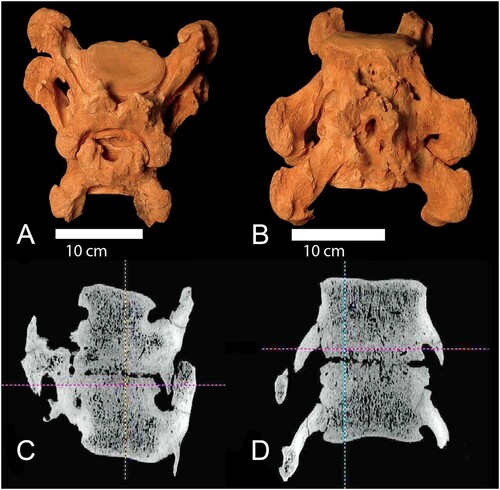
The pathology is restricted to these two vertebrae and the articulating anterior and posterior caudals to these were not affected. This pattern of anomalous bone growth is suggestive of infectious spondylitis with the resulting ankylosing of the two vertebrae. The immediate cause of the pathology is unknown, but the pathological bone growth is probably the result of a secondary infection. In humans, bacterial infections such as brucellosis and salmonellosis have been associated with spondylitis (Govender and Schweitzler, Citation2003; Pappas et al., Citation2005).
Deterioration (REE)
Rare Earth Elements (REEs) occur in extremely low concentrations in living tissues but are rapidly absorbed into the bone after death (Trueman and Tuross, Citation2002). Previous studies have found depth-related variations in bone REE patterns (Trueman and Tuross, Citation2002) as well as heterogeneous REE signatures due to partial fossilization (Suarez et al., Citation2010). There is little research regarding the impact of weathering on REE signatures. To this end, a differentially weathered Tarkio Megalonyx rib (SUI 101037 CC) was selected for analysis. The rib shows a systematic increase in weathering from the more deeply buried distal end to the topographically higher, and thus longer exposed, proximal end along with a corresponding increase in normalized REE concentrations on the medial side of the rib (). The most weathered segment on the medial side (segment G, weathering stage 5) is approximately 4–6 times more enriched in REEs than the least weathered segment (segment A, stage 0.5), depending on the rare earth measured. This is likely due to increased collagen degradation and mechanical breakage on the longest exposed portion, creating greater void space in the bone. Thus, weathering creates a greater surface area interaction with REE-bearing pore waters. The less exposed lateral side does not show this relationship as clearly. It was impossible to sample the most weathered segments F and G (weathering stages 4 and 5, respectively) from the lateral side as the bone was adhered to its protective jacket.
FIGURE 10. Rare earth element patterns exhibited by medial and lateral views of the Megalonyx rib (SUI 101037CC), normalized to Post-Archean Australian Shale (REEN). Sample locations and assigned weathering stages (Behrensmeyer, Citation1978) are depicted in the insets and show samples A–G for the medial side (n = 8) and A–E for the lateral side (n = 6). Segment B was sampled twice on each side and rib segment A is proximal. Solid lines indicate minimally weathered rib segments and dotted lines indicate more weathered segments.
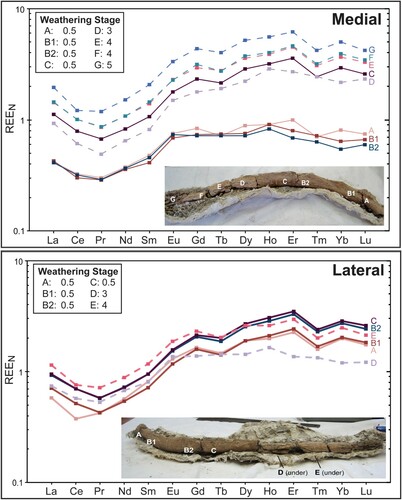
As the REEs are incorporated into the bone, it appears that the weathering stage has a less severe effect on the ratios between each of the elements compared with the individual elemental concentrations. However, section D presents an anomalous REE signature from both the medial and lateral sides. When this rib was excavated, a second rib was overlying it near section D. This overlying rib may have interrupted the flow of REE-carrying pore waters, insulating the underlying rib. These REE variations in response to weathering have significant implications for interpreting REE signatures in fossil bones. Historically, REE signatures in bone are interpreted as a function of depositional environment and time, but uptake also seems to be a function of weathering. Thus, taphonomic processes must be considered before drawing conclusions from REE data (Grimm, Citation2011; Grimm and Graham, Citation2011). The weathering pattern exhibited by the rib further substantiates incremental burial of the sloth remains.
PALEOECOLOGY
Paleobotany
The only paleobotanical samples (Supplemental Data, Table S4) to yield both plant macrofossils and pollen were collected beneath the pelvis. The pelvic sample (SU-3), containing 16 taxa, includes Leersia oryzoides (cut grass), Scirpus validus (soft-stem bulrush), Typha (cattail), Lycopus americanus (American water-horehound) and Glyceria (mannagrass), consistent with a marshy environment (Native Plant Database Staff, Citation2021). Other species, including Urtica dioica (stinging nettle), Amaranthus (amaranth), Thlaspi arvense (field pennycress), and Chenopodium (goosefoot), are consistent with open, somewhat disturbed ground, and Verbena hastata (common vervain) and Lobelia spicata (pale-spiked lobelia) are indicative of stream banks in prairies.
Verbena urticifolia (white vervain) and Aralia racemosa (spikenard) are found in open deciduous woodlands. The single Picea (spruce) needle fragment was probably derived from reworked glacial sediments. Pollen analysis included Quercus (oak), Cedrus (cedar), Pinus (pine), Carya (hickory), Poaceae (grass), Ambrosia (ragweed), Dryopteris (wood fern), and Lycopodium (club moss). This combination is typical of modern gallery forests along stream courses in Iowa today (Native Plant Database Staff, Citation2021).
Plants with C4 photosynthetic pathways (warm season grasses) have more isotopically heavy carbon in their tissues than plants with C3 pathways (cool season grasses, most wetland and woody vegetation). The Tarkio carbon isotopic profiles taken from the two cores () generally indicate mixed C3/C4 composition in the pre-Wisconsin alluvium with slightly more contributions from C4 vegetation in core SL-1 than in SL2B.
Pollen, plant macrofossils and carbon isotopes coincide to document a marshy site typical of floodplain settings today where riparian forests line the active channel belt in a landscape with significant grasslands on uplands and broad terraces (e.g., Baker et al., Citation1992, Citation1996) and support a moderate climate in an interglacial environment. In contrast, glacial and interstadial sites from the Midwest all have predominantly conifer pollen and macrofossils (Williams et al., Citation2001). Other Midwest Sangamon interglacial sites show similar environments (Gruger, Citation1972; Zhu and Baker, Citation1995; Curry and Baker, Citation2000). The paleobotany of Tarkio supports an interglacial paleoenvironment for the ∼106 ka year-old Sangamon (MIS substage 5ec) sloth-bearing deposit.
Mollusks
Three molluscan taxa – a large unionid and the spheroids Pisidium (pill or pea clams) and Sphaerium (fingernail clams) – were primarily recovered from a small (0.5 m2) area near a juvenile rib concentration (, MM21). A single fragmentary unionid specimen was in MM-16 (). Because each genus contains several species, their taxonomy is difficult and their paleoecological value is limited and specific identification was not attempted. All species of both spheroid genera may occur in almost any type of water body, but pea clams can withstand both cold and drying. Fingernail clams primarily reside in brackish water. Both genera can cohabit waters that other mollusks cannot tolerate. The low molluscan diversity suggests brackish water and suggests that periodic drying occurred during deposition of sloth-bearing sediments.
Stable Isotopes
Examination of the molariform (SUI 101037 X) revealed the presence of the four dentine layers, each of which exhibits the distinct hardness, porosity, and thickness characteristic of the Xenarthra (Bryk et al., Citation2010a, Citation2010b). Dentine band width and hardness dictates, in part, occlusal morphology that develops with wear during mastication (Naples, Citation1990). Carbonate δ13C varied greatly between the four dentine layers ( A–C) but is relatively consistent within each layer (). The mean δ13C values, in order of depletion, were: Layer 1: −10.1‰; Layer 2: −11.2‰; Layer 3: −8.5‰; Layer 4: −8.4‰; Layer 4: Transect −8.8‰; and Layer 1: Transect −10.3‰ (Bryk et al., Citation2010a, Citation2010b). Vickers Hardness (VH) tests were also conducted for each of the dentine layers (; Smith and Sandly, Citation1922). Of the four layers, Layer 2 exhibits the most depleted δ13C signature and the greatest VH value. Layers 3 and 4 display the most enriched carbon isotope values but do not exhibit the lowest VH number.
FIGURE 11. Stable isotope evaluation, transverse cross section, Tarkio Valley adult Megalonyx molariform (SUI-101037X). A, the four distinct dentine layers are numbered to avoid ambiguity in nomenclature; B, growth increments longitudinal image; C, sampling square locations 1–8 beginning at the occlusal end of the tooth; D, carbonate δ13C (‰, VPDB) for all samples by layer number; E, Vickers hardness index for each dentine layer using both 50 g and 100 g of force.
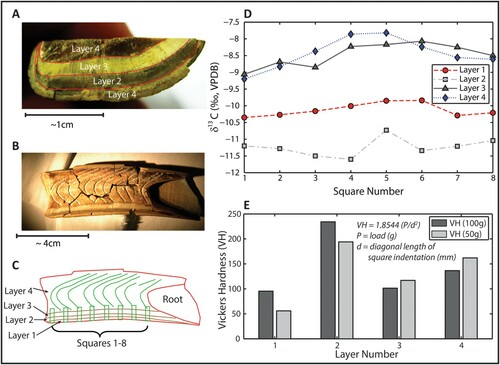
The wide variation in carbon isotope values (nearly 4‰) between dentine layers is interpreted to be diagenetic and the alteration appears to affect each dentine layer differentially, as observed in Eremotherium (Larmon et al., Citation2019). Diagenesis is of special concern for Xenarthrans because they lack enamel, the most favorable (and typically least altered) source for stable isotope analysis. Dentine typically contains less of the inorganic mineral hydroxyapatite than tooth enamel, rendering it more susceptible to diagenesis. There is presently no direct method to assess the alteration of primary isotopic signatures in biologically derived hydroxyapatite. Therefore, despite measuring 56 samples from a single tooth, it was not possible to determine unambiguously which of the four dentine layers exhibited minimal digenetic alteration.
The lack of a temporal signal along presumed growth layers supports the assertion that diagenesis is the mechanism for isotopic variation between dentine layers at Tarkio. The mean δ18O value across all samples was low (5.2‰), further suggesting that the tooth has been substantially altered (Kohn et al., Citation2005). Although we interpret the spread in carbon isotopes as a diagenetic signal, Vickers Hardness as a diagenetic indicator is insufficient to explain the isotopic variability between layers, and we cannot rule out the possibility of differential but primary fractionation processes within each layer occurring as the tooth grew. The spread in isotope data suggests that in general, a single measurement is insufficient to place an organism ecologically. For Megalonyx jeffersonii in particular, a full histological analysis of the tooth and/or an independent method for assessing diagenesis is required to understand the isotopic differences among the four dentine layers. While the evidence for diagenesis is clear, all δ13C values still lie between −8‰ and −11‰. Two fall firmly within the woodland herbivore portion of the spectrum and two are on the boundary between the woodland herbivore and the grassland herbivore spectra of Kohn et al. (Citation2005:fig. 1). This suggests that the Tarkio sloths probably inhabited moderately open woodland, an environment that is generally interpreted for M. jeffersonii (Schubert et al., Citation2004). This is consistent with the pollen, carbon isotope, and plant macrofossil data from Tarkio.
TARKIO MEGALONYX PALEOBIOLOGY
Ontogenetic Development and Body Size
Because of the many unique aspects of sloth dental anatomy (including the lack of deciduous teeth, a life-long, ever-growing dental battery, distinctive morphological changes with wear and the lack of comparative homology with the teeth of other placentals), it is impossible to quantify the relative ontogenetic ages of fossil sloths based on dentition. Instead, it is necessary to use stages of epiphyseal fusion. Adulthood for the large Tarkio specimen was established by the presence of obliterated sutures in all post-cranial elements. Complete fusion is also present along all facial sutures. While the face was fully mature, the lambdoidal suture was still open, the only cranium of 27 crania examined by co-author McDonald with an open lambdoidal suture, indicating that the braincase was continuing to grow. In view of the robust post-cranial sample at Tarkio, it appears that the last suture to fuse in M. jeffersonii was the lambdoidal suture.
While we cannot directly determine the lifespan, gestation time and age at sexual maturation, these values can be estimated using formulae derived by Blueweiss et al. (Citation1978) in their study of the relationship between mammalian body size, lifespan, birth interval, and gestation period (). Using their formula for calculating the average lifespan for a species, the estimated average lifespan for M. jeffersonii is 19 years, gestation time is 14 months; and maturation occurs at 6.5 years. In the living two-toed sloth, Choloepus didactylus, the gestation time is 10.5 months (Partridge, Citation1991). Most living megaherbivores that produce one offspring at a time have a long gestation period, up to 22 months in the African elephant (∼3600 kg), Loxodonta (Lang, Citation1967) and 18–22 months in the Asian elephant (∼3000 kg), Elephas (Deraniyagala, Citation1955). The white rhinoceros, Ceratotherium (∼1900 kg), has a gestation period of 16–19 months (Rawlins, Citation1979).
TABLE 2. Life history parameters for Megalonyx jeffersonii based on formulae from Blueweiss et al. (Citation1978) using the estimated weight of 1286 kg for the adult Tarkio Valley specimen. Abbreviations: W = weight in grams, y = days.
Based on an estimated weight (McDonald, Citation2005:table 2) of 1286 kg for the adult and, assuming a minimum of a 2-year interval between successive births (), a female M. jeffersonii optimally could have produced about five offspring during her lifetime, or four offspring with a 3-year interbirth interval. Of course, if the calculation underestimates the average lifespan of M. jeffersonii, then the number of potential offspring would have been higher. For example, Sacher’s (Citation1959) equation for estimating the lifespan of a mammal tlife = 28.3Mb0.19 results in a lifespan of 49 years for M. jeffersonii based on a body mass of 1286 kg. Large body size suggests that Megalonyx was a K-selected species with a low birth rate and a high investment in long-term parental care.
Sexual Dimorphism
Sexual dimorphism has been described for: the megathere, Eremotherium (Cartelle and Bohórques, Citation1982); the North American mylodont, Paramylodon (McDonald, Citation2006); and South American scelidothere, Scelidotherium (Miño-Boilino and Zurita, Citation2015). In these taxa, all skull sizes are comparable in length, but gracile versus robust morphologies in the three genera are attributed to sexual dimorphism. Depending upon the measurement, the Tarkio adult is one of the three largest individuals known for Megalonyx (). While sexual dimorphism based on cranial morphology in Megalonyx is not evident, bimodality appears in bivariate plots of available mandibular and humeral measurements (McDonald, Citation1977:fig. 9; Supplemental Data, Table S5: see Supplemental Table S6 for other selected post-cranial measurements). However, there are no independent criteria to assign a sex to either mode. Because females are larger than males in some mammals (Ralls, Citation1977), an a priori assumption that the smaller individuals are females is not reasonable.
FIGURE 12. Bivariate plots comparing the adult Megalonyx jeffersonii from Tarkio Valley (open diamond) with other specimens of the species from Iowa (open square) and outside of Iowa (solid squares). A, upper caniniform; B, radius; C, astragalus; D, femur. Element measurements are tabulated in Supplemental Data, Table S6.
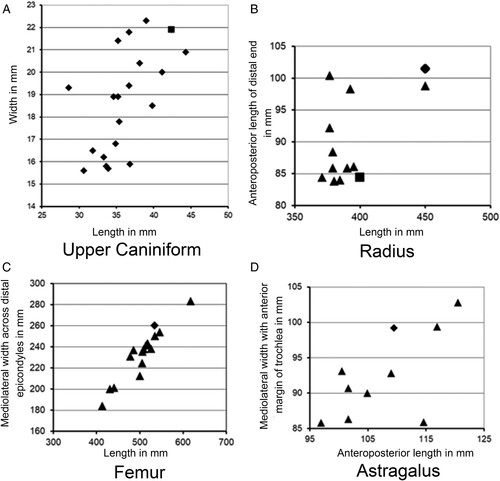
In many mammals the development of the sagittal crest, when present, is sexually dimorphic and better developed in males. While a sagittal crest is not present in robust crania of Paramylodon, it is evident in all robust specimens, inferred to be males, of Eremotherium and Scelidotherium and absent in gracile crania. A sagittal crest is present in Megalonyx, but its development is highly variable. The most pronounced Megalonyx jeffersonii sagittal crest is in the type of M. milleri, now considered a junior synonym of M. jeffersonii (McDonald, Citation1977), but this specimen also has a sloping occiput, a proposed female characteristic in Paramylodon (McDonald, Citation2006). While the total length of the Tarkio Valley cranium (SUI 101037 DK) could not be measured, the braincase, which has a vertical occiput, is sufficiently complete to document a poorly developed sagittal crest (5 mm maximum height) despite the relatively large size of the individual. As in other mammals, the presence of a diminutive crest supports the possibility that the Tarkio adult is a female but, because of documented variability, this cannot be proven. The direct association of infant, sub-adult, and adult individuals does suggest that the mother, if not the adult preserved at the site, was nearby. A mother/offspring relationship, or a parent/offspring association, is the most likely scenario for the Tarkio trio.
The size of the adult, as documented () by the upper caniniform (SUI 101037 AF and U), radius (SUI 101037 DH), femur (SUI 101037 BR), and astragalus (SUI 101037 BH; Supplemental Data, Table S5), exceeds that of most other individuals of the species. An estimate of the body mass based on the adult Tarkio femoral length is 1286 kg, about 15% larger than the average body mass of 1090 kg calculated for M. jeffersonii by McDonald (Citation2005:table 1). Measurements of the scapulae of the three sloths indicate the sub-adult was about 50% (643 kg) of the adult’s body size and the infant would have been about 30% (386 kg) of the size of the adult (Grass, Citation2014).
Sexual dimorphism is also well documented in the body size of mammals and, when present, the male usually, but not always, is the larger of the two. If the Tarkio adult Megalonyx is female, it may indicate that Megalonyx females tended to be larger than the males and sexual dimorphism accounts for the bimodal size distribution recorded by McDonald (Citation1977:110–118). There are numerous examples in mammals (Ralls, Citation1977:table 1), primarily in smaller species but also in marine taxa, primates and larger carnivores, where the female is larger. This relationship is rare in large terrestrial herbivores, but it does occur (Ralls, Citation1977). The female is larger in Tapirus terrestris (South American tapir; Luxenberg, Citation2014) and T. indicus (Malaysian tapir; Gearty, Citation2012), and in Okapia johnsoni (okapi; Hart, Citation2013). Lara-Ruiz and Chiaello (Citation2005) documented that in the maned sloth, Bradypus torquatus, females are larger than males. However, Boscaini et al. (Citation2019) report that, while females tend to be larger in all tree sloths, the differences are not statistically significant.
Although Megalonyx shared denning sites (Holte, Citation2012), those sites are interpreted as time transgressive and do not provide evidence that M. jeffersonii formed social groups, like those hypothesized for some species of South American ground sloths (Lindsey et al., Citation2020). Even in herds, mothers take prime responsibility for their young. Larger body size in females may be an important adaptation for protection (Ralls, Citation1976) and critical for young Megalonyx survival, especially in the absence of male parental involvement. This would be especially true within dispersed family units.
Paleopathology
An infection indicated by the ankylosed caudal vertebrae (SUI 10137CN; ) possibly contributed to the death of the adult. If the infection spread throughout the body, it may have had a detrimental effect on the general health of the animal that ultimately factored in its demise. The death of the adult undoubtedly would severely impact the survival potential of the two younger and dependent sloths.
IMPLICATIONS FOR THE ASSOCIATION OF THREE TARKIO INDIVIDUALS
As indicated above, the remains of the three sloths were recovered in four disarticulated clusters, with Clusters 1 and 2 partially overlapping, over a 360 m2 surface (). Each cluster generally represented a different individual (adult, subadult, and infant). The adult, with approximately 50% recovery (123 skeletal elements plus 16 teeth) including most of the rib cage, is the most complete of the three individuals. The sub-adult () is one of the two most complete juvenile M. jeffersonii skeletons known. Based on associations in Cluster 2 (), the sub-adult elements include 19 ribs; both scapulae (SUI 102849 C and G); a clavicle (SUI 102849 S); a partial humerus (SUI 102849 K); an ilium (SUI 102849 AJ) and ischium (SUI 102849 AW), two non-fused vertebral centra (SUI 102849 N and W); one vertebral epiphysis (SUI 102849 B) and six non-fused thoracic neural arches (SUI 102849 R, X, AH, AR, AS, and BJ) as well as SUI 102849 P, which was fused to its centrum along with both epiphyses. The infant is only positively represented by both scapulae (SUI 130544 A and B), but three ribs in Cluster 4 were juxtaposed () in MM18. Most of the ribs and vertebral elements probably belong to the sub-adult based on proportions () and cluster association (, MM13 and 16). Other than the fully fused cervical (SUI 102849P), it is impossible to assign any other element to either the sub-adult or infant given the lack of comparative immature specimens. However, the other centra conform in size to the fused specimen, suggesting that Cluster 2 is largely composed of sub-adult elements.
While it is possible that the three individuals died at different times and accumulated in the same area, this scenario seems highly unlikely given the low probability of three different individual sloths of separate ontogenetic ages accumulating at different times in the same place without stratigraphic separation. Their burial in finely laminated deposits confirms contemporaneous death, at least within a season. Furthermore, at sites where multiple individuals accumulate over time, there is usually an attraction (mineral lick, saline spring, cave, etc.) that lures a variety of species of animals (e.g., Brain Citation1981; Saunders Citation1988; Behrensmeyer et al., Citation1992; Rogers et al. Citation2007). There is no evidence for such a feature at Tarkio. As indicated above, the only other vertebrate taxa associated with the three sloth individuals are turtle carapace fragments. Thus, it appears that the Tarkio site was not an attractant because other taxa would be expected in a time-averaged sample.
Juxtaposition and integration of the bone scatters of the three individuals demonstrates that the carcasses decayed almost contemporaneously in the same microstratigraphic unit and were partially scattered prior to burial. The association of large adult and smaller juvenile elements, including ribs, in a fine-grained matrix virtually precludes secondary mixing via fluvial transport. However, epiphyses for the relatively small sub-adult centra, with one exception, and all ribs are missing, presumably removed by passive fluvial action indicated by sediment micromorphology. Transverse fractures and element re-arrangement are characteristic of trampling before burial.
Only two scapulae can be definitively assigned to the infant and, while some infant ribs may be present, most are missing. The scapulae, as well as smaller sub-adult ribs, are well preserved and suggest that poor ossification is not involved in bone deterioration. Heavier infant elements should be present. Thus, removal by carnivorans is a likely cause of their absence.
Most of the sternebrae and sternal ribs for the adult sloth were recovered near each (), demonstrating that the heavier adult rib cage elements were less impacted by post-mortem processes than the smaller, lighter bones of the juveniles. Because of: overlapping skeletal elements of different densities; primary occurrence within the same stratigraphic horizon; shared taphonomic histories; absence of other taxa and burial in a low energy, pond-like environment, we interpret the co-occurrence of the three sloths as the result of primary internment at the death site. While the cause of death cannot be determined, a catastrophic event, e.g., drought, lightning, or disease, is possible. The death of the adult, for whatever reason, may have secondarily contributed to the death of the juveniles.
The co-occurrence of an adult with two juveniles of different sizes and different ontogenetic ages may provide the first direct evidence of a Megalonyx family group or, at least, provide insight into supervised juvenile care in an extinct sloth. The most parsimonious scenario for the Tarkio trio relationship is that of a mother and two offspring. Even in herding placentals where there is collective parenting, the mother is the primary provider. In the living two-toed (Choloepus didactylus) and three-toed (Bradypus torquatus) sloths, there is only a single newborn (Partridge, Citation1991; Lara-Ruiz and Chiarello, Citation2005) and like modern sloths, M. jeffersonii most likely gave birth to a single offspring within a 2–3-year period. In the modern sloths all parental care is provided by the mother as male and female sloths do not form permanent pair-bonds (Garcés-Restrepo et al., Citation2017).
Based on the size of the infant scapula () and its stage of ossification, it appears that it reflects the most recent birth. Metrics place this individual at about 30% the size of the adult (Grass, Citation2014). While the exact age of the sub-adult cannot be determined, its scapula dimensions are significantly larger, about 50% of the size of the adult (Grass, Citation2014). This individual represents a significant growth interval prior to the birth of the infant. The adult was apparently parenting both younger sloths. It is noteworthy that the sub-adult was still receiving parental investment at the time of the birth of the infant. This scenario suggests that like most large mammals, ground sloths provided extended parental care. It is also reasonable to infer that the sub-adult was weaned by the time of the birth of the infant.
The two living genera of sloths, Choloepus and Bradypus, have convergent evolutionary histories with a Megalonyx/Choloepus lineage distinct from that of Bradypus (Nyakatura, Citation2011). They also have similar reproductive strategies (Taube et al., Citation2001). Both care for their young after weaning, but offspring depart before the next infant arrives. Therefore, the reproductive strategy we have proposed for the Tarkio Megalonyx may not be applicable to other extinct giant ground sloth genera (e.g., Paramylodon, Eremotherium, etc.). Sexual maturity based on the body size of the adult can be estimated at about 6.5 years using the relationships calculated by Blueweiss et al. (Citation1978) (). In living megaherbivores, birth intervals typically vary from 2–4 years (Owen-Smith, Citation1998). We, therefore, suggest that the interbirth period in M. jeffersonii could have been 3 years and that the larger sub-adult would have been about 3 years older than the infant but not yet sexually mature. Having achieved 50% of adult size in about 3–4 years, and assuming a constant rate of growth, the sub-adult would have achieved adult size and sexual maturity in another 3–4 years at which time it would leave the mother, coincident with the birth of the next offspring.
SUMMARY
The remains of three individual Megalonyx jeffersonii were recovered from a single bedding plane, in West Tarkio Creek, southwestern Iowa. An interglacial age (MIS Substage 5c) is documented by the regional stratigraphic context and an OSL date of ∼106,000 years ago from the bone bearing Sub-Unit 3. The associated pollen, plant macrofossils, mollusks, rodent-gnawed bone, sedimentary carbon isotopes, and stable isotopes from a Tarkio Megalonyx tooth indicate that the sloths lived along a stream in an open-hardwood forest with marshy areas. This environment is like temperate southwestern Iowa today.
The Megalonyx individuals from Tarkio represent three different ontogenetic stages: adult, sub-adult, and infant. The adult and sub-adult are among the most complete known skeletons for their respective developmental stages. Body mass equations suggest that the adult weighed around 1286 kg and that, based on differential scapulae sizes, the younger sloths were 50% and 30% of that size with weights around 643 kg for the sub-adult and about 386 kg for the infant. The adult probably is a female based on its poorly developed sagittal crest. Its large body size suggests that M. jeffersonii females may have been larger than the males. Using formulae for mammal body size to project lifestyles, we calculate that the estimated average lifespan for M. jeffersonii was ∼19 years, gestation time was ∼14 months; the interbirth interval was ∼3 years, and sexual maturation occurred ∼6.5 years.
Mollusks, sediment micromorphology, a range of bone weathering stages and finely laminated sediment indicate episodic deposition during burial in a backwater situation. The preferential preservation of skeletal elements documents that the adult was probably lying on its left side during decomposition. The carcasses and bones were subaerially exposed for a limited amount of time as indicated by weathering stages, rodent gnawing, and bone dispersal. MNI counts will only accommodate one individual for each ontogenetic stage. Fluvial action was incapable of transporting even the smallest adult skeletal elements, e.g., sesamoids, juvenile ribs, and vertebrae. However, low-level fluvial activity (e.g., floating) might best explain the absence of small, light juvenile epiphysial elements for the ribs and vertebrae. All three of Voorhies (Citation1969) transport stages are well represented by the adult skeleton, which additionally refutes fluvial transport as a major taphonomic process at Tarkio. Other taphonomic processes such as scavenging and trampling probably contributed to the dismemberment and dissemination of the remains.
The most parsimonious interpretation of the three sloths is that they represent a social unit and died contemporaneously. First, all three sloths are preserved in the same low-energy microstratigraphic unit without any evidence of fluvial transport. Second, they occur in intermixed bone clusters with both the adult and sub-adult skeletons relatively complete. All indicating relatively rapid burial with limited subaerial exposure. The lack of associated vertebrate fauna, other than three turtle fragments, indicates that Tarkio is a mono-taxon site and not a time-averaged “bonebed.” Furthermore, it is highly unlikely that three individuals of the same species of differing ontogenetic stages would die in the same location unless they died and were buried contemporaneously. There is no specific evidence for the cause of death of the three sloths other than it was nearly contemporaneous.
The association of the trio indicates that Megalonyx, like most megaherbivores and living sloths, gave birth to one offspring at a time. Although rare in modern sloths, which are several orders of magnitude smaller than Megalonyx, parental care in Megalonyx apparently extended beyond weaning of an older sibling. Because both living sloth genera have habitat preferences quite different from Megalonyx, their reproductive behavior cannot be applied to extinct sloths. Tarkio provides a unique glimpse into the paleobiology of an enigmatic, extinct animal that coexisted with a diverse megafauna during the Pleistocene.
Supplemental Material
Download PDF (709 KB)ACKNOWLEDGMENTS
This Megalonyx association would never have been discovered if it were not for B. Athen’s sharp eye in discerning an unusual shape in the West Tarkio creek bed, excavating it, realizing its significance, and getting professional identification. The recovery of the remains, which transgressed two properties, was enhanced by the generosity of B. and S. Athen, Northboro, IA as well as D. and L. Tiemann, originally from Westboro, MO, who provided heavy equipment access to the excavation across their fields and donated the specimens to the University of Iowa. Mather and Sons Excavation realized the need for levee construction and immediately responded to our request below cost. W. Mott, who volunteered to operate rented light equipment, attended all digs. R. McAfee, Ohio Northern University and K. McCarville, Upper Iowa University, provided much-needed expertise at the site. University of Iowa laboratory analyses were provided by E. Hoffman, Radiology (CT), College of Medicine; J. Thomas, Electron Microprobe Laboratory, Earth, and Environmental Science; S. Struckman, Prototyping Center, College of Engineering; L. Hunter (nee Evans), Department of Anthropology, prepared the juvenile rib display; J. Dorale, Stable Isotope Laboratory, Earth and Environmental Sciences prepared thin-and polished molariform sections. R. Feranec, NY State Museum, provided stable isotope assistance; R. Richards, Indiana State Museum, for archival data on the Henderson Megalonyx; S. Holte-Weaver, The Mammoth Site, for ACb-3 juvenile counts, and M. Pardi, Illinois State Museum for data on the Crankshaft juvenile. All specimen preparation, cataloging, and photography was performed by volunteers in the UI Museum of Natural History. K. Tefft and M. Dietz photographed the individual skeletal elements (Supplemental Data, Appendix S2). Sponsoring organizations were the Iowa Archeological Society, Take A Kid Outdoors, Boy Scouts of America, Central Iowa Gem and Mineral Society, Greater Shenandoah Historical Museum, and Iowa Office of the State Archaeologist. Numerous individuals, listed by event in Megalonyx Matters (Supplemental Data, Appendix S1) came from Iowa, Illinois, Missouri, Wisconsin, and Nebraska. The Corps of Engineers, Rock Island District, approved a Dewatering Permit to temporarily relocate the West Tarkio; J. Mead, The Mammoth Site of Hot Springs; F. Pujos, Instituto Argentino de Nivologfa, Glaciologia y Ciencias Ambientales; B. Schubert, East Tennessee State University; E. Lundelius, Jr., University of Texas, J. Harris, Natural History Museum in Los Angeles; M. Borths, Curator of Fossil Primates at the Duke University Lemur Center, and an unnamed reviewer offered valuable comments. S. Semken, Ice Cube Press; A. Swanson, student, Brown University; and P. Thorson-Work, Maine State Museum, assisted with figure preparation. This project was supported by NSF grants SEGR 0618036, 0829393, and EAGAR 1037817.
LITERATURE CITED
- Baker, R. G., E. Bettis, and D. Schwert. 1996. Holocene paleoenvironments of northeast Iowa. Ecological Monographs 66:203–224.
- Baker, R. G., L. J. Maher, C. A. Chumbley, and K. L. Van Zant. 1992. Patterns of Holocene Environmental Change in the Midwest. Quaternary Research 37:379–389.
- Behrensmeyer, A. K. 1978. Taphonomic and ecologic information from bone weathering. Paleobiology 4:150–162.
- Behrensmeyer, A. K., J. D. Damuth. W. A. DiMichele, R. Potts, H. D. Sues, and S. L. Wing 1992. Terrestrial Ecosystems Through Time: Evolutionary Paleoecology of Terrestrial Plants and Animals. University of Chicago Press, Chicago.
- Binford, L. R. 1981. Bones: ancient men and modern myths. New York: Academic Press.
- Blueweiss, H. F., V. Kudzma, D. Nakashima, R. Peters, and S. Sams. 1978. Relationships between body size and some life history parameters. Oecologia 37:257–272.
- Blumenschine, R. J. 1986. Carcass consumption sequences and the archaeological distinction between scavenging and hunting. Journal of Human Evolution 15:639–659.
- Boscaini, A., T. J. Gaudin, N. Toledo, B. M. Quispe, P. Antoine, and F. Pujos. 2019. The earliest well-documented occurrence of sexual dimorphism in extinct sloths: evolutionary and palaeoecological insights. Zoological Journal of the Linnean Society 187:229–239, https://doi.org/10.1093/zoolinnean/zlz011.
- Brain, C. K. 1981. The Hunters or the Hunted? – An Introduction to African Cave Taphonomy. University of Chicago Press, Chicago.
- Brown, N. D., and S. L. Forman. 2012. Evaluating a SAR TT-OSL protocol for dating fine-grained quartz within Late Pleistocene loess deposits in the Missouri and Mississippi river valleys, United States. Quaternary Geochronology 12:87–97.
- Bryk, A., R. Feranec, and H. A. Semken. 2010a. Carbon Isotope Analysis of Adult Molariform Dentin Layers from the Tarkio Valley Ground Sloth, Megalonyx jeffersonii, from Southwestern Iowa. Program and Abstracts, American Quaternary Association 21:80.
- Bryk, A., R. Feranec, H. A. Semken and L. Eccles. 2010b. Stable Isotope Analysis of an Extinct Pleistocene Ground Sloth, Megalonyx jeffersonii, from the Tarkio Valley of Southwestern Iowa: Implications for Bioapatite Diagenesis. Journal of Vertebrate Paleontology 30 (3, Supplement):66A.
- Cartelle, C., and G. A. Bohórques. 1982. Eremotherium laurillardi Lund, 1842. Parte I. Determinaço especifica e dimorfismo sexual. Iheringia, Série Geológica, Porto Alegre, 7:45–63.
- Cope, E. D. 1889 The Edentata of North America. American Naturalist 23:657–664.
- Curry, B.B., and R. G. Baker. 2000. Paleohydrology, vegetation, and climate during the last interglaciation (Sangamon Episode) in south-central Illinois. Palaeogeography, Paleaeoclimatology, and Palaeoecology 155:59–81.
- Deraniyagala, P.E.P. 1955. Some extinct elephants, their relatives and the two-living species. Ceylon National Museum, Colombo, Ski Lanka, 161 pp.
- Desmarest, A. G. 1822. Mammalogie ou dèscription des espéces de mammifères. 4 Agasse, Paris, France.
- Elderfield, H., and R. Pagett. 1986. Rare earth elements in Ichthyoliths: Variations with redox conditions and depositional environment. The Science of the Total Environment 49:175–197.
- Faith, J.T. 2007. Sources of variation in carnivore tooth-mark frequencies in a modern spotted hyena (Crocuta crocuta) den assemblage, Amboseli Park, Kenya. Journal of Archaeological Science 34:1601–1609.
- Fiorillo, A.R. 1989. An experimental study of trampling: implications for the fossil record; pp. 61–72 in R. Bonnichsen and M. H. Sorg (Eds.), Bone Modification, Center for the Study of the First Americans, Orono, ME.
- Garcés-Restrepo, M.F., M. Z. Peery, B. Reid, and J. N. Pauli. 2017. Individual reproductive strategies shape the mating system of tree sloths. Journal of Mammalogy 98:1417–1425.
- Gearty, W. 2012. “Tapirus indicus” (On-line), Animal Diversity Web. Available at https://animaldiversity.org/accounts/Tapirus_indicus/. Accessed October 19, 2019.
- Gervais P. 1855. Recherches sur les mammifères fossiles de l'Amérique méridionale. Comptes Rendus de l'Académie des Sciences 40: 1112–1114.
- Gillette, D. D., H. G. McDonald, and M. C. Hayden. 1999. The first record of Jefferson’s ground sloth, Megalonyx jeffersonii, in Utah (Pleistocene, Rancholabrean Land Mammal Age). Utah Geological Survey Miscellaneous Publication 99: 509–521.
- Govender, S., and G. Schweitzler. 2003. Salmonella spondyitis in inflammatory disorders of the spine; pp. 1–7 in S. Govender (ed.), Inflammatory diseases of the spine. TTG Asia Media Pty Ltd, Singapore.
- Grass, A. D. 2014. Inferring Lifestyle and Locomotor Habits of Extinct Sloths Trough Scapula Morphology and Implications for Convergent Evolution in Extant Sloths. Ph. D. dissertation, University of Iowa, Iowa City, Iowa, 114 pp.
- Grass, A. D. 2019. Inferring differential behavior between giant ground sloth adults and juveniles through scapula morphology. Journal of Vertebrate Paleontology. DOI: 10.1080/02724634.2019.1569018
- Grimm, B. L. 2011. The effects of weathering on rare earth element (REE) uptake: A study from the Pleistocene Tarkio Valley and Eocene Bones Galore fossil sites. B.S. thesis, Pennsylvania State University, State College, Pennsylvania, 32 pp.
- Grimm, B. L, and R. W. Graham. 2011. The effects of weathering on rare earth element (REE) uptake: a study from the Pleistocene Tarkio Valley and Eocene Bones Galore fossil sites. Journal of Vertebrate Paleontology 31(Supplement, 3):120A.
- Gruger, E. 1972. Pollen and Seed Studies of Wisconsinan Vegetation in Illinois, USA. Geological Society of America Bulletin 83:2715–2734.
- Guthrie, R. D. 1990. Frozen Fauna of the mammoth steppe: The story of Blue Babe. University of Chicago Press, Chicago, 338 pp.
- Harlan R. 1825. Fauna Americana: being a description of the mammiferous animals inhabiting North America. Anthony Finley. Philadelphia, Pennsylvania.
- Harlan, R. 1831. Description of the fossil bones of the Megalonyx discovered in “White Cave” Kentucky. Journal of the Academy of Natural Science, Philadelphia ser. 1,6:269–288.
- Hart, J. A. 2013. Okapia johnstoni. pp. 110–115 in: J.S. Kingdon and M. Hoffmann (eds), The Mammals of Africa. Volume VI: Pigs, Hippopotamuses, Chevrotain, Giraffes, Deer and Bovids. Bloomsbury Publishing, London.
- Hay, O. P. 1914. The Pleistocene Mammals of Iowa. Iowa Geological Survey, Annual Report 23 for 1912, 662 pp.
- Haynes, G. 1980. Evidence of carnivore gnawing on Pleistocene and Recent mammalian bones. Paleobiology 63:341–351.
- Haynes, G. 1982. Utilization and skeleton disturbances of North American prey carcasses. Arctic 35:266–281.
- Haynes, G. 1983. A guide for differentiating mammalian carnivore taxa responsible for gnaw damage to herbivore limb bones. Paleobiology 9 (2): 164–172.
- Hill, A. 1989. Bone modification by spotted hyenas; pp. 169–178 in R. Bonnichsen and M. H. Sorg (Eds.), Bone Modification, Center for the Study of the First Americans, Orono, ME.
- Hoganson, J. W., and H. G. McDonald. 2007. The first report of the occurrence of Jefferson’s ground sloth (Megalonyx jeffersonii) in North Dakota and its paleobiogeographical and paleoecological significance. Journal of Mammalogy 88:73–80.
- Holte, S. E. 2011. The osteological ontogeny of the Megalonyx jeffersonii material from ACb-3 Cave, Colbert County, Alabama. Journal of Vertebrate Paleontology 31(3 Supplement):127A.
- Holte, S. E. 2012. Description of Jefferson’s Ground Sloth (Megalonyx jeffersonii) from Acb-3 Cave, Colbert County, Alabama, with Comments on Ontogeny, Taphonomy, Pathology, and Paleoecology. M.Sc. thesis, East Tennessee State University, Johnson City, Tennessee, 180 pp.
- Holte, S. E., B. W. Schubert, and S. C. Wallace. 2010. Analysis of the Megalonyx jeffersonii material from ACB-3 Cave, Colbert County, Alabama and an examination of measuring techniques for ground sloths. Journal of Vertebrate Paleontology 30(3, Supplement):107A.
- Hopkins, M. L, R. Bonnichsen, and D. Fortsch. 1969. The stratigraphic position and faunal associates of Bison (Gigantobison) latifrons in southeastern Idaho, a progress report. Tebiwa12:1–7.
- Horgen, S., D. Brenzel, and H. A. Semken. 2007. The Tarkio Valley ground sloth excavations: an adult Jefferson’s ground sloth (Megalonyx jeffersonii) in association with two juveniles from the late Pleistocene of southwestern Iowa. Journal of Vertebrate Paleontology 27(3, Supplement):92A.
- Josephs, R. L. 2009. Micromorphological Investigations at the Tarkio Valley Sloth Site, Page County, Iowa. Current Research in the Pleistocene. 26:198–201.
- Josephs, R. L., and E. A. Bettis III. 2003. A practical alternative to Kubiena Boxes for the collection of samples for micromorphological analysis. Geoarchaeology 18:567–70.
- Kohn, M. J., M. McKay, and J. L. Knight. 2005. Dining in the Pleistocene-Who’s on the menu. Geology 33:649–652.
- Lang, E. M. 1967. The birth of an African elephant, Loxodona africana at Basle Zoo. International Zoo Yearbook 7:154–157.
- Lara-Ruiz, P., and A. Garcia Chiarello. 2005. Life-history traits and sexual dimorphism of the Atlantic forest maned sloth Bradypus torquatus (Xenarthra: Bradypodidae). Journal of Zoology, London 267:63–67.
- Larmon, J. T., H. G. McDonald, S. Ambrose, L. R. G. DeSantis, and L. J. Lucero. 2019. A year in the life of a Giant Ground Sloth during the Last Glacial Maximum in Belize. Science Advances 2019; 5: eaau1200, 9 pp.
- Leidy, J. 1855. A memoir on the extinct sloth tribe of North America. Smithsonian Contributions to Knowledge 7:68 pp.
- Leidy, J. 1860. Remarks on the structure of the feet in Megalonyx. Transactions of the American Philosophical Society, n.s. 11:107.
- Lindsey, E. L., E. X. L. Reyes, G. E. Matzke, K. A. Rice, and H. G. McDonald. 2020. Monodominant late-Pleistocene megafauna locality from Santa Elena, Ecuador: Insight on the biology and behavior of giant ground sloths. Paleogeography, Paleoclimatology, Paleoecology 544:1–9, https://doi.org/10.1016/j.palaeo.2020.109599.
- Lively, R. S., G. L. Bell, and J. P. Lamb. 1992. Uranium series dates from travertines associated with a late Pleistocene megafauna in ACb-3 Cave, Alabama. Southeastern Geology 33:1–8.
- Luxenberg, S. 2014. “Tapirus terrestris” (On-line), Animal Diversity Web. Available at https://animaldiversity.org/accounts/Tapirus_terrestris/. Accessed October 19, 2019.
- Lyman, R. L. 1994a. Quantitative units and terminology in zooarchaeology. American Antiquity, 59:36–71.
- Lyman, R. L. 1994b. Vertebrate Taphonomy. Cambridge University Press, New York. 524p.
- MacFadden, B. J., J. Labs-Hochstein, R. C. Hulbert, Jr., and J. A. Baskin. 2007. Revised age for the late Neogene terror bird (Titanis) in North America during the Great American Interchange. Geology 35:123–126.
- Mahoney, M. C., H. A. Semken, D. J. Brenzel, and S. E. Horgen. 2009. Sloth on! The diary of an ice-age giant ground sloth excavation. Newsletter of the Iowa Archeological Society 59:3–6.
- Marshall, L. G. 1989. Bone modification and “The Laws of Burial.” pp. 7–24 in R. Bonnichsen and M. Sorg (eds.), Bone Modification. Center for the Study of First Americans, Orono, ME.
- Martin, R. A., and Webb, S. D. 1974. Late Pleistocene mammals from the Devil’s Den fauna, Levy County. In S. D. Webb (ed.), Pleistocene Mammals of Florida. University Florida Press. Pp. 114–145. Gainesville, Florida.
- McDonald, H. G. 1977. Description of the osteology of the extinct gravigrade edentate with observations on its ontogeny, phylogeny, and functional anatomy. M. Sc. thesis, University of Florida, Gainesville, Florida, 328 pp.
- McDonald, H. G. 2005. Paleoecology of extinct Xenarthrans and the Great American Biotic Interchange. Bulletin of the Florida Museum of Natural History 45:313–333.
- McDonald, H. G. 2006. Sexual dimorphism in the skull of Harlan's ground sloth. Natural History Museum of Los Angeles County Contributions to Science No. 510:1–9.
- McDonald, H. G. 2012. Harlan’s Ground sloth (Paramylodon harlani) (Xenarthra: Mylodontidae) from the Late Pleistocene (Rancholabrean) of Iowa. Journal of the Iowa Academy of Science: 119:16–21.
- McDonald, H. G. 2021. Yukon to the Yucatan: Habitat partitioning in North American Late Pleistocene Ground Sloths (Xenarthra, Pilosa). Journal of Palaeosciences 70: 237–251.
- McDonald, H. G., W. E. Miller, and T. H. Morris. 2001. Taphonomy and significance of Jefferson's Ground Sloth (Xenarthra: Megalonychidae) from Utah. Western North American Naturalist 61:64–77.
- McDonald, H, G. T. W. Stafford, and D. M. Gnidovec. 2015. Youngest radiocarbon date for Jefferson’s ground sloth (Megalonyx jeffersonii) (Xenartha, Megalonychidae). Quartenary Research83:355–359.
- Mech, L. D., and R. O. Peterson. 2003. Wolf-prey relations; pp. 131–160 in L.D. Mech and L. Boitani, (eds.); Wolves: behavior, ecology and conservation. Chicago: University of Chicago Press.
- Mercer, H. C. 1897. The finding of the remains of the fossil sloth at Big Bone Cave, Tennessee in 1896. Proceedings of the American Philosophical Society 36:70.
- Milideo, L. E. 2015. Actualistic and Statistical Approaches to Taphonomic Interpretations in Quaternary Environments. Unpublished PhD Dissertation. The Pennsylvania State University, State College, PA.
- Miño-Boilino, Á. R., and A. E. Zurita. 2015. Dimorphism in Quaternary Scelidotheriinae (Mammalia, Xenarthra, Phyllophaga). Palaeontologia Electronica 18.1.12A: 1–16. https://palaeo-electronica.org/content/2015/1102-dimorphism-in-scelidotheriinae
- Naples, V. L. 1990. Morphological changes in the facial region and a model of dental growth and wear pattern development in Nothrotheriops shastensis. Journal of Vertebrate Paleontology 10:372–389.
- Native Plant Database Staff. 2021. Native Plant Database, Natural Resources Conservation Service, United States Department of Agriculture. https://plants.usda.gov/home/plantProfile?symbol=CEOC2
- Nyakatura, J. A. 2011. The Convergent Evolution of Suspensory Posture and Locomotion in Tree Sloths. Journal Mammal Evolution 19:225–234. https://doi.org/10.1007/s10914-011-9174-x
- Owen-Smith, R. N. 1998. Megaherbivores. Cambridge: Cambridge University Press.
- Pappas, G, N. Akritidis, M. Bosilkovski, and E. Tsianos. 2005. Brucellosis. New England Journal of Medicine 352:2325–2336.
- Parmalee, P. W., R. D. Oesch, and J. E. Guilday. 1969. Pleistocene and Recent vertebrate faunas from Crankshaft Cave, Missouri. Illinois State Museum, Report of Investigations No. 14, 37 pp.
- Partridge, J. 1991. The two-toed sloth. International Zoo News 38:23–28.
- Peterson, R. O., and P. Ciucci. 2003. The wolf as a carnivore; pp. 104–130 in L.D. Mech and L. Boitani, (eds.); Wolves: behavior, ecology and conservation. Chicago: University of Chicago Press.
- Ralls, K. 1976. Mammals in which females are larger than males. Quarterly Review of Biology 51:245–276.
- Ralls, K. 1977. Sexual dimorphism in mammals: Avian models and unanswered questions. The American Naturalist 111:917–938.
- Rawlins, C. G. C. 1979. The breeding of white rhinos in captivity: a comparative study. Der Zoologische Garten 49:1–7.
- Ray, H. A., A. E. Haj, J. D. Loch, E. A. Bettis III, H. A. Semken, K. M. Rocheford, and S. L. Forman. 2013. The stratigraphy and paleoecology of Pleistocene deposits associated with giant ground sloths (Megalonyx jeffersonii), Page County, Iowa. Geological Society of America, Abstracts with Programs 45:119.
- Rogers, R. R., D. A. Eberth, and A. R. Fiorillo. 2007. Bone Beds – Genesis, Analysis and Paleobiological Significance. University of Chicago Press, Chicago.
- Rohland, N., and M. Hofreiter. 2007. Ancient DNA extraction from bones and teeth. Nature Protocols, 2(7), 1756–1762. https://doi.org/10.1038/nprot.2007.247
- Sacher, G. A. 1959. Relation of lifespan to brain weight and body weight in mammals; pp. 115–141 in G.E.W. Wolstenholme (ed), Ciba Foundation Colloquium on Aging, Vol. 1.
- Safford, J. M. 1892. The pelvis of Megalonyx and other bones from Big Bone cave, Tennessee. Bulletin of the Geological Society of America 3:121–123.
- Saunders, J. J. 1988. Fossiliferous spring sites in Southwestern Missouri; pp. 127–149 in S. Laub, N. G. Miller, and D. W. Steadman (eds), Late Pleistocene and Early Holocene paleoecology and archaeology of the eastern Great Lakes region. Bulletin of the Buffalo Society of Natural History 33.
- Schubert, B. W., R. W. Graham, H, G. McDonald, E. C. Grimm, and T. W. Stafford. 2004. Latest Pleistocene paleoecology of Jefferson’s ground sloth (Megalonyx jeffersonii) and elk-moose (Cervalces scotti) in northern Illinois. Quaternary Research 61:231–240.
- Semken, H. A., and D. Brenzel. 2010. Megalonyx Matters. University of Iowa, Museum of Natural History. Available at https://mnh.uiowa.edu/megalonyx-matters. Accessed February 3, 2019.
- Shaver, W. L, Schubert B. W., and S. C. Wallace. 2006. The Cave of Sloths: A Description of Megalonyx jeffersonii remains from ACb-3 Cave, Colbert County, Alabama. Journal of Vertebrate Paleontology 26 (Supplement 3):124A.
- Smith, R. L., and G. E. Sandly. 1922. An accurate method of determining the hardness of metals, with particular reference to those of a high degree of hardness. Proceedings of the Institution of Mechanical Engineers 102:623–641.
- Soil Survey Staff, 1999. Soil Taxonomy, A Basic System of Soil Classification for Making and Interpreting Soil Surveys. Agricultural Handbook No. 436, 2nd edition, United States Department of Agriculture, Natural Resources Conservation Service. U. S. Government Printing Office, Washington, D. C., 886 pp.
- Stiner, M. C., N. D. Munro, and M. Sanz. 2012. Carcass damage and digested bone from mountain lions (Felis concolor): implications for carcass persistence on landscapes as a function of prey age. Journal of Archaeological Science 39:896–907.
- Stock, C. 1925. Cenozoic gravigrade edentates of western North America. Carnegie Institute of Washington Publication 331:1–206.
- Suarez, C.A., D. L. Macpherson, L. A. Gonzalez, and D. E. Grandstaff. 2010. Heterogeneous rare earth element patterns and concentrations in fossil bone: Implications for the use of REE in vertebrate taphonomy and fossilization history. Geochimica et. Cosmochimica Acta 74:2970–2988.
- Tappen, M. 1994. Bone weathering in the tropical environment. Journal of Archaeological Science 21:667–673.
- Taube, E., J. Keravec, J. Vie, and J. Duplantier. 2001. Reproductive biology and postnatal development in sloths, Bradypus and Cholepus: review with original data from the field (French Guiana) and from captivity. Mammal Review 31:173–188.
- Trueman, C. N., and M. J. Benton. 1977. A geochemical method to trace the taphonomic history of reworked bones in sedimentary settings. Geology 25:263–266.
- Trueman, C. N., and N. Tuross. 2002. Trace elements in recent and fossil bone apatite. Reviews in Mineralogy and Geochemistry 48:489–521.
- Trueman, C. N., A. K., Behrensmeyer, R. Potts, and N. Tuross. 2006. High-resolution records of location and stratigraphic provenance from the rare earth element composition of fossil bones. Geochimica et Cosmochimica Acta 70:4343–4355.
- Voorhies, M. R. 1969. Taphonomy and population dynamics of an Early Pliocene vertebrate fauna, Knox County, Nebraska. University of Wyoming, Contributions to Geology, Special Paper 1:1–69.
- Williams, J. W., B. N. Shuman, and T. Webb III. 2001. Dissimilarity analyses of late-Quaternary vegetation and climate in eastern North America. Ecology 82:3346–3362.
- Woida, K. 2006. West Tarkio Creek Watershed Plan and Environmental Impact Statement, Appendix D-Investigation and Analysis Report, USDA Natural Resources Conservation Service, Des Moines, Iowa, 104 pp.
- Zhu, H., and R. G. Baker 1995. Vegetation and climate of the last glacial-interglacial cycle in Southern Illinois, USA. Journal of Paleolimnology 14: 337–354, https://doi.org/10.1007/BF00682432