ABSTRACT
Theropod dinosaurs likely radiated in the wake of the End-Triassic Extinction, but the early history of their diversification remains obscure. The Elliot Formation (EF) and its lateral equivalents in southern Africa preserves abundant continental Late Triassic and Early Jurassic vertebrate fossils, representing an opportunity to study the early phases of theropod evolution. However, Elliot Formation theropod remains are scarce relative to other dinosaurian groups, and most of the South African record pertains to a single taxon, Megapnosaurus rhodesiensis. We present morphological and osteohistological data on two theropod distal tibiae from South Africa’s upper EF. The two new tibiae are larger than any known specimens of M. rhodesiensis, and they show a fast-growing woven-parallel complex (WPC). One of the specimens bears a line of arrested growth, but neither shows outer circumferential lamellae. These observations suggest that both specimens are immature and distinguishable from M. rhodesiensis based on osteohistology. Comparative anatomical observations, including body mass comparisons, further support this distinction. We cannot rule out an identification for one of our tibiae as Dracovenator regenti, the only other valid EF theropod taxon known from body fossils. Our observations indicate that there is likely at least one additional, relatively large-bodied upper EF theropod species that remains unknown from adequate skeletal material.
INTRODUCTION
Theropod dinosaur fossils are first known from the Late Triassic. They were relatively rare members of Late Triassic ecosystems, with low taxonomic and morphological diversity (Brusatte et al., Citation2008). However, after the End-Triassic Extinction (ETE) they diversified rapidly to become the dominant small- to large-bodied predators of Jurassic continental ecosystems (Brusatte et al., Citation2008). Late Triassic and Early Jurassic theropod body fossils are relatively rare, but their ichnofossils are extremely well-represented assemblages from this time period, particularly in the Newark Supergroup of North America (e.g. Olsen et al., Citation2002) and in the Elliot Formation of southern Africa (e.g. Sciscio et al., Citation2016; Bordy et al. Citation2020).
The Elliot Formation (Main Karoo Basin: Stormberg Group) of South Africa is one of the few fossiliferous continental deposits spanning the ETE (e.g. Olsen & Galton, Citation1984; Bordy et al, Citation2020). The lithostratigraphic boundary between its upper and lower portions is generally considered an approximate marker of the mass extinction based on the biostratigraphy, magnetostratigraphy, and chronostratigraphy (Haughton, Citation1924; Haughton & Brink, Citation1954; Olsen & Galton, Citation1984; Kitching & Raath, Citation1984; Knoll, Citation2004, Citation2005; Sciscio et al., Citation2016; Viglietti et al., Citation2020a, Citation2020b; McPhee et al., Citation2017; Bordy et al., Citation2020). The formation contains a wealth of dinosaurian body fossils, dominated by sauropodomorphs (∼65%) and to a lesser extent, ornithischian dinosaurs (∼23%) (Viglietti et al., Citation2020a, Citation2020b). Theropod dinosaurs are rare in this unit (<2% of occurrences; Bordy et al., Citation2020), and any discovery of their fossils has the potential to help understand the early radiation of Theropoda. Currently, there are two valid theropod taxa known from body fossils in South Africa’s Jurassic upper Elliot Formation: the small-bodied Megapnosaurus rhodesiensis Raath, Citation1969 (estimated at 13 kg, Benson et al., Citation2014), and the larger Dracovenator regenti Yates, Citation2005. Yates (Citation2005) estimated that the skull of Dracovenator was about 500 mm in total length, similar to the phylogenetically close Dilophosaurus wetherilli, and this observation suggests Dracovenator weighed about 350 kg (Benson et al., Citation2014).
Our continued work in the collections of the Evolutionary Studies Institute discovered two previously undescribed distal theropod tibiae from the upper Elliot Formation (uEF) of South Africa, representing taxa considerably larger than known specimens of Megapnosaurus rhodesiensis. In this study, we describe and compare their anatomy and osteohistology to assess theropod taxonomic diversity of the earliest Jurassic in southern Africa.
Institutional Abbreviations—BP, Bernard Price Institute for Palaeontological Research (University of the Witwatersrand), Johannesburg, South Africa; IVIC—P, Colección Paleontológica del Centro de Ecología, Instituto Venezolano de Investigaciones Científicas, Caracas, Venezuela; HMN MB R., Museum für Naturkunde der Humboldt Universität, Berlin, Germany; PULR, Paleontología, Universidad Nacional de La Rioja, La Rioja, Argentina; PVL, Instituto Miguel Lillo, San Miguel de Tucumán, Argentina; QG, Natural History Museum of Zimbabwe, Bulawayo, Zimbabwe; TMM, Texas Memorial Museum, Austin, Texas, U.S.A.; UCMP, University of California Museum of Paleontology, Berkeley, California, U.S.A.
MATERIALS AND METHODS
The study material consists of two right distal theropod tibiae, BP/1/4903 and BP/1/6215, both from the upper Elliot Formation of South Africa. Specimen BP/1/4903 was recovered on the farm Mequatling 459 in the Clocolan area of the Setsoto Local Municipality, Free State, South Africa (incorrectly identified in Smith and Kitching [Citation1997] as cadastral unit Mequatling 278). It is part of a multi-taxon bonebed containing the mixed postcranial remains of typical upper Elliot taxa, including Massospondylus carinatus, Heterodontosaurus tucki, and possibly Lesothosaurus diagnosticus. The specimen collection records indicate that the bonebed containing BP/1/4903 was collected from the ‘Tritylodon Acme Zone’ (Smith & Kitching, Citation1997). Recent biostratigraphic work (Bordy et al., Citation2020; Viglietti et al., Citation2020b) shows that Tritylodon occurs in two distinct stratigraphic levels, both positioned close to the middle of the upper Elliot Formation, in horizons dated as Early Jurassic (Hettangian–Sinemurian). The second theropod tibia, BP/1/6215, was found on the farm Damplaats 55 (Butler et al., Citation2007) in the Ladybrand area of the Mantsopa Local Municipality, Free State, South Africa. The specimen collection records indicate that it was found ±50 m above the contact between the upper and lower Elliot formations, also indicative of an Early Jurassic age, although potentially older than BP/1/4903.
Morphological descriptions were done with personal inspection of specimens and reference to the published literature. Linear measurements were taken with a Mitutoyo digimatic caliper (model: CD-6”C, Aurora, [IL], US) and angular measurements were taken using ImageJ (Schneider et al., Citation2012) on high-resolution digital photographs in orthogonal viewing planes. We employ non-standard Romerian terminology for our anatomical observations (e.g., anterior/posterior rather than rostral/caudal). Where possible, we use the descriptive terms “long/short”, “tall/low”, and “broad/narrow” to describe variation in the anteroposterior, dorsoventral, and mediolateral directions, respectively, and when we deviate from this convention we provide appropriate directional specifications.
The two tibiae were cast, photographed, drawn, and measured prior to thin sectioning to ensure that all relevant morphological data were recorded. Thin sectioning methods follow that of Botha-Brink et al. (Citation2018). All thin sectioning was conducted at the National Museum, Bloemfontein, South Africa. A total of 8 slides were produced for BP/1/4903 and 11 slides were produced for BP/1/6215. Completed sections are archived at the Evolutionary Studies Institute, University of the Witwatersrand, Johannesburg, South Africa catalogued under the specimen numbers BP/1/4903 1-8 and BP/1/6215 1-11. The renders are available online under the Morphosource project: 000460203. This study was conducted under South African Heritage Resources agency destructive sampling permit ID: 11484.
The bone microstructure revealed on thin sections was viewed and imaged under normal and cross-polarized light. Observations and photographs were made using the software NIS Elements 4.5 (Nikon Corp., Tokyo, Japan) connected to a DS-Fi3 camera mounted on a Nikon Eclipse Ci-POL microscope. Comparative osteohistological terminology follows that of Prondvai et al. (Citation2014) and de Buffrénil et al. (Citation2021a). Comparisons were made with an ontogenetic series of the closely related contemporaneous theropod Megapnosaurus rhodesiensis as well as other theropods from the relevant literature (see for full list and references).
TABLE 1. Distal mediolateral width measurements of various theropods.
RESULTS
Morphological Description
BP/1/6215 is the distal end of a right tibia (). The shaft is broken a short distance proximal to the epiphyseal expansion. The posterolateral process is missing the distal half of the lateral side, but the remainder of the distal end is complete. The anterolateral process is complete, but the dorsal margin of its cortical surface is slightly eroded, and this erosion continues onto the anterior portion of the shaft. The posterior surface is complete and well preserved, except for the distal tip of the posterior ridge.
FIGURE 1. Three distal theropod tibiae. BP/1/6215 in A, anterior view, B, interpretive drawing of anterior view, C, posterior view, and D, distal view. BP/1/4903 in E, anterior view, F, interpretive drawing of anterior view, G, posterior view and H, distal view. Megapnosaurus rhodesiensis juvenile distal tibia BP/1/4944 in I, anterior view J, interpretive drawing of anterior view, K, posterior view, and L, distal view. Dashed lines show positions of thin sections. Abbreviations: alp, anterolateral process; faf, fibula articular facet; plp, posterior lateral process; pr, posterior ridge; t, tuberosity. All scale bars equal 10 mm.
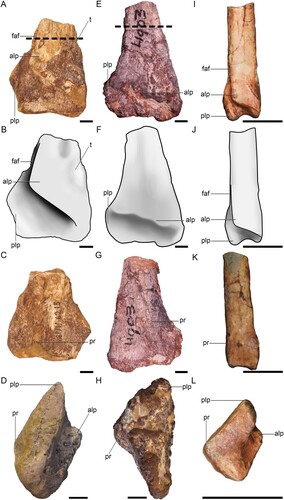
The short, preserved portion of the shaft has an ovoid cross-section, with the major axis oriented mediolaterally. The lateral and medial margins of the shaft are subparallel, contrasting with the morphology of BP/1/4903. The distal margin of the anterolateral process is sharply demarcated from the anterior surface of the posterolateral process by a lightly rugose lip of bone. Ventral to this lip, the anterolateral process is concave before grading into the flat anterior surface of the posterolateral process. This concavity bears numerous faint, anteroposteriorly oriented striations and a series of small pits that open distally and slightly laterally. The distal margin of the anterolateral process extends proximolaterally at approximately a 38° angle from the line of the distal end of the tibia, standing in marked contrast to the lower angle of BP/1/4903. The lateral side of the distal margin ends abruptly as a rounded eminence well medial to the level of the lateral margin of the posterolateral surface. This corner then extends a short way distally before grading into the anterior tibial shaft. The medial side of the distal margin grades smoothly into the mediodistal corner of the tibia, forming a U-shaped junction with the distal surface. Although preservation of the cortex of the anterior surface of the anterolateral process is poor, the medial side appears to bear a low tuberosity that extends onto the medial side of the distal tibia. The distal side of this tuberosity is shallowly concave as it grades into the anterior surface of the mediodistal corner of the anterolateral process. The posterolateral process expands laterally from the tibial shaft, forming a 45° slope with respect to the proximodistal line of its lateral margin. Although the laterodistal corner is broken, the available morphology suggests that the lateral expansion is subquadrangular in anterior view. The proximal portion of the posterolateral process that is lateral to the anterolateral process is shallowly concave, and this concavity extends a short way proximally along the anterolateral side of the tibial shaft before being obscured by erosion and poor preservation. The anterior surface of the remainder of the posterolateral process is flat to slightly convex and it bears faint, proximodistally oriented striations. The most salient feature of the posterior surface of the distal tibia is a raised, proximodistally extending ridge of bone that is slightly medial to the midline of the tibia shaft. This ridge forms a mediolaterally broad triangle in cross section, and is most prominently developed only at the level of the lateral expansion of the posterolateral process. Proximal to this level, the ridge grades smoothly and rapidly into the posterior tibial shaft. The distal surface of the tibia is subtly saddle-shaped, with a low, convex medial side that projects slightly farther distally than the flattened lateral side. A shallowly mediolaterally concave and anteroposteriorly flat region separates these two portions.
The relatively large anterior exposure of the posterolateral process and its subquadrangular outline in anterior view in BP/1/6215 are similar to Liliensternus liliensterni (MB.R 2175.7.8.1) and to Zupaysaurus rougieri (Ezcurra & Novas, Citation2007; Ezcurra & Brusatte, Citation2011; Ezcurra, Citation2017) and differ from the smaller, more lobate posterolateral processes of Gojirasaurus quayi (Carpenter, Citation1997), Tachiraptor admiribilis (Langer et al., Citation2014), Megapnosaurus, Lepidus, Cryolophosaurus and Dilophosaurus wetherilli (Ezcurra, Citation2017). In BP/1/6215, Liliensternus, Tachiraptor admiribilis (Langer et al., Citation2014), and likely in Dilophosaurus wetherilli (reconstruction obscures these features slightly; Marsh & Rowe, Citation2020), the angle of the distal end of the anterolateral process relative to the distal tibia margin exceeds 33°, whereas this angle is less than 25° in Coelophysis bauri, Zupaysaurus rougieri, and Gojirasaurus quayi (Carpenter, Citation1997). There is no indication of a well-developed ‘anterior diagonal tuberosity’ on the anteromedial side of the anterolateral process, which is similarly lacking in Powellvenator, Liliensternus, Gojirasaurus and Zupaysaurus and is unlike the prominent tuberosity present in some other early branching neotheropods including Camposaurus arizonensis, Megapnosaurus rhodesiensis, and Coelophysis bauri (Ezcurra, Citation2017). Finally, as in Liliensternus, the proximodistally extending ridge on the posterior tibial surface of BP/1/6215 is mesially positioned, whereas other early branching theropods either appear to lack this feature (e.g., Camposaurus [Ezcurra & Brussate, Citation2011]), or rather bear a ridge that is positioned closer to the medial margin of the posterior surface (e.g., Powellvenator [Ezcurra, Citation2017], Zupaysaurus [Ezcurra, Citation2017], Lepidus praecisio [Nesbitt & Ezcurra, Citation2015], BP/1/4903). BP/1/6215 has a distal width similar to that of Liliensternus lilensterni (MB R. 2175) and Tachiraptor admiribilis (IVIC-P-2867) ().
BP/1/4903 is the distal end of a right tibia (). It preserves slightly more of the shaft than BP/1/6215. The cortical surfaces of the shaft, the anterior surface of the anterolateral process, and the posterior surface of the posterolateral process are intact, but are replete with cracks that are infilled with adhering, maroon matrix likely containing oxidized iron. The distal margin of the anterolateral process and the anterior surface of the posterolateral process are only poorly preserved and are covered with more of this maroon matrix. The distal surface of the tibia is lightly eroded, obscuring its precise morphology, but likely not obliterating much of its length.
The preserved portion of the shaft has a flat anterior surface and convex posterior, medial, and lateral surfaces. In anterior or posterior view, the lateral margin scribes a shallowly concave arc and the medial margin is slightly convex. The epiphyseal expansion is more pronounced on the lateral side, but it is much more gradual than the abrupt expansion of BP/1/6215, grading smoothly into the shaft.
The anterolateral process is well demarcated from the posterolateral process by a proximolaterally extending ridge, but this ridge appears to be less undercut than in BP/1/6215. It also differs markedly from BP/1/6215 in being set at approximately a 20° angle relative to the distal surface (as opposed to a 38° angle). Although the distal surface is eroded, it is nearly complete, and we view it as extremely unlikely that erosion could result in this large of an angular difference between the two specimens. On its medial side, the anterolateral process extends to the medial margin of the epiphysis, where its junction is obscured by poor preservation and adhering matrix. On its lateral side, the distal margin of the anterolateral process appears to extend to the lateral margin of the distal tibia, contrasting with the morphology of BP/1/6215 where it ends well medial to the lateral margin. Even if erosion has removed some of the lateral margin, the lateral extent of the distal margin of the anterolateral process is more laterally positioned than BP/1/6215.
The lateral side of the posterolateral process is moderately expanded from the distal end. The morphology of the preserved portion suggests that it was semicircular in anterior or posterior views. Unlike BP/1/6215, the lateral side lacks a groove or recessed area lateral to the anterolateral process for the articulation of the fibula, suggesting that the fibular articulation would have been slightly offset from the tibia.
The posterior surface bears a prominent, proximodistally oriented ridge that extends along the lateral side, being inset only a short way from the lateral margin. This ridge extends as far distally as the preserved portion allows us to observe, separating the posterior surface into a narrower posterolaterally and a broader posteromedially facing portion, and gives the tibial shaft a broad, triangular cross section. This condition is markedly different from BP/1/6215 in that the ridge is much more extensive proximally and posteriorly, and that it is positioned on the lateral side of the bone.
The poor preservation of BP/1/4903, and its origin as an element within a multi-taxon bonebed, necessitate differentiation from other co-occurring dinosaurs. A distal tibia of a small (juvenile or small adult; taxon unknown) sauropodomorph from the same bonebed differs from BP/1/4903 in being mediolaterally proportionally narrower, anteroposteriorly proportionally much thicker, and with a quadrangular rather than triangular cross-sectional geometry. Furthermore, that specimen bears the more typical sauropodomorph arrangement of the anterolateral and posterolateral processes being separated by a deep lateral notch. A distal tibia of an ornithischian from the same bone bed (BP/1/8702, Lesothosaurus diagnosticus) differs in that the anterolateral process only occupies the lateral half of the anterior surface of the distal end, in that the distal surface bears a deep midline notch that clearly separates the distal surface into medial and lateral portions, and the posterior ridge is much less pronounced. These comparisons readily indicate that other co-occurring dinosaurian taxa can be differentiated from the theropod material.
The low angle of the distal margin of the anterolateral process relative to the distal tibial margin is similar to early neotheropods like Coelophysis bauri, Gojirasaurus quayi, Powellvenator podocitus, and Zupaysaurus rougieri and differs markedly from the higher angles of BP/1/6215, Dilophosaurus wetherilli (Marsh & Rowe, Citation2020), and Tachiraptor admiribilis (Langer et al., Citation2014. Although its preservation is poor, BP/1/4903 appears to lack the ‘anterior diagonal tuberosity’ of Camposaurus arizonensis, Megapnosaurus rhodesiensis, and Coelophysis bauri (Ezcurra & Brusatte, Citation2011; Ezcurra, Citation2017). In anterior view, the posterolateral process is lobate, rather than quadrangular, resembling the condition present in Megapnosaurus rhodesiensis (), Gojirasaurus, Tachiraptor and differing from the subquadrangular posterolateral processes of BP/1/6215, Zupaysaurus, Liliensternus, and Coelophysis bauri (Ezcurra, Citation2017). The concave lateral margin of the tibial shaft as it meets the distal end of the tibia is similar to Dilophosaurus wetherilli (Marsh & Rowe, Citation2020), Liliensternus (MB.R.2175.7.8.1), Tachiraptor admiribilis (Langer et al., Citation2014), and differs from the straighter lateral margins of Powellvenator, Lepidus, Gojirasaurus, Camposaurus, Cryolophosaurus (Smith et al., Citation2007), and Berberosaurus (Allain et al., Citation2007). The lateral position of the ridge on the posterior surface of the tibia of BP/1/4903 is similar to those of Camposaurus arizonensis, and possibly Gojirasaurus, and differs to BP/1/6215 and Liliensternus, where this ridge is more medially positioned, and to Berberosaurus and Cryolophosaurus, where this ridge is absent. BP/1/4903 has a slightly smaller distal width compared to BP/1/6215 ().
Osteohistology
BP/1/6215 () has a large, open medullary cavity. There are large resorption cavities on the farthest lateral posterior edge of the specimen. These cavities are seen throughout the sections, but become smaller in the more distal sections. The specimen has numerous, haphazardly arranged, round osteocyte lacunae in compacted coarse cancellous bone (CCCB) surrounding the medullary cavity. CCCB is prominent in the distal metaphyseal region of the bone. This process compacts the trabeculae (Enlow, Citation1963). This is seen throughout the sections, including the most distal and proximal sections. The inner cortex has numerous scattered secondary osteons.
FIGURE 2. Osteohistology of theropod tibiae BP/1/6215 and BP/1/4903 from the Early Jurassic of South Africa. A, the primary cortex of BP/1/6215 consists of a highly vascularized WPC. B, BP/1/4903 showing a WPC. It has a LAG (white arrowhead) within an annulus and there is an increase in radial and circular vascular canals after the LAG. C, the cortex of BP/1/4903 indicating the LAG (white arrowhead) and two possible annuli (black arrowheads).
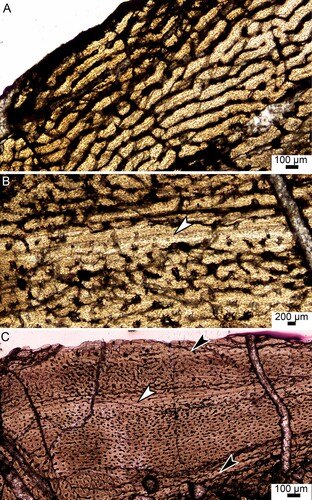
A thin region of endosteal bone is visible towards the distal epiphysis. The only primary bone tissue that is preserved is the outer cortex, which consists of a woven-parallel complex (WPC) (). The amount of woven-parallel bone tissue decreases towards the epiphysis. The bone tissue contains a mixture of longitudinally oriented primary osteons and simple canals with short anastomoses. There is no decrease in the number or size of vascular canals towards the periphery.
There are no growth marks visible. This may be due to the young ontogenetic status of the individual, which might have died during its first year. However, the sections were taken from the distal metaphysis of the bone, which means that some of the growth marks may be missing due to secondary remodeling. The features of the bone tissues nevertheless indicate that this individual was a juvenile. Furthermore, outer circumferential lamellae (OCL) was also not preserved.
BP/1/4903 () has a large, open medullary cavity with numerous resorption cavities in the perimedullary region. The bone tissue consists of round, haphazardly arranged osteocyte lacunae within a woven-fibered bone matrix. The vascular canals comprise numerous longitudinally-oriented primary osteons. These features indicate the presence of a WPC (woven-fibered bone with parallel-fibered bone comprising the primary osteons as defined by Prondvai et al., Citation2014 and de Buffrénil et al., Citation2021a). A thin region of endosteal bone is visible throughout the specimen except for the most distal and proximal sections.
BP/1/4903 has one clear line of arrested growth (LAG) within an annulus of lamellar bone (). LAGs indicate a temporary cessation in growth whereas annuli indicate a temporary decrease in growth. These cyclical growth marks are usually seasonal (de Buffrénil et al., Citation2021b). There are possibly two more growth marks, one in the inner cortex and one at the bone periphery (). This cannot be confirmed due to poor preservation of the inner cortex and insufficient amount of peripheral bone in the outermost cortex. The number of longitudinal vascular canals decreases slightly after the mid-cortical LAG. There are more circular and radial vascular canals outside the LAG than before it. Vascular canals are present, but sparse within the annulus. Outer circumferential lamellae (OCL), which indicates that the individual has ceased growing, is absent. An OCL is a sparsely vascularized area and is composed of parallel-fibered or lamellar bone (Cormack, Citation1987) and usually contain many closely spaced LAGs (Horner et al., Citation1999). In contrast, BP/1/4903 was still actively growing at the time of death as shown by the highly vascularized WPC at the sub-periosteal surface.
The highly vascularized WPC observed in these specimens is typical of theropod bone tissues (e.g., Woodward et al., Citation2011; Griffin & Nesbitt, 2019; Shen et al., Citation2019) including the penecontemporaneous coelophysoids Megapnosaurus rhodesiensis (), Segisaurus halli Carrano, Hutchinson, and Sampson, Citation2005, and Coelophysis bauri (Cope, Citation1887) (Barta & Norell, Citation2019). shows BP/1/6215 and BP/1/4903 in scale compared to an adult M. rhodesiensis (BPI001).
FIGURE 3. Osteohistology of three Megapnosaurus rhodesiensis tibiae from the Lower Jurassic of South Africa. A, BPI008, showing compacted coarse cancellous bone before a LAG (black arrow head) and a WPC after to the subperiosteal surface. A thin layer of endosteal bone (white arrow) is present close to the medullary cavity. No OCL is present indicating the individual had not reached maximum size; B, BP/1/6614, showing a WPC with longitudinally oriented primary osteons and circular and radial vascular canals. One LAG is present in the outer cortex (white arrowhead); C, BPI001, showing a WPC with longitudinally oriented primary osteons. An OCL (black brackets) at the sub-periosteal surface indicates that the individual had reached maximum size. The white arrowhead indicates a mid-cortical LAG. Abbreviation: CCCB, compacted coarse cancellous bone; MC, medullary cavity.
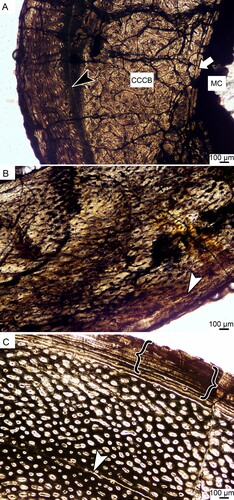
Chinsamy (Citation1990) described the femoral osteohistology of Megapnosaurus rhodesiensis (at that time assigned to the genus Syntarsus), a theropod that is contemporaneous with the specimens in this study. Juvenile BPI008 () has a large open medullary cavity with no visible trabeculae. The cortex is largely comprised of CCCB, but a thin layer of WPC was observed in the outer cortex. A LAG separates the two regions of bone. Specimen BP/1/6614 () has a large medullary cavity. A very thin region of endosteal bone is present. The cortex has numerous, round, haphazardly arranged osteocyte lacunae. A small section of CCCB is present near the medullary cavity in the more distal sections. The majority of the cortex is comprised of a highly vascularized WPC. There is a LAG in the outer cortex of the specimen. The majority of the primary osteons are longitudinally oriented. There are numerous primary osteons and a few simple radial vascular canals.
Adult Megapnosaurus specimens, for example BPI001 (), have a large medullary cavity, with no visible trabeculae. A thin region of endosteal bone is present in some of the individuals. The cortex is comprised of numerous, round, haphazardly arranged osteocyte lacunae. These are found in a highly vascularized WPC that is present throughout the specimens of all the individuals (Chinsamy, Citation1990). The primary osteons are almost all longitudinally oriented, with a few circumferential vascular canals being present. Certain specimens have scattered secondary osteons near the medullary cavity. At least one LAG is observed in the mid-cortex of specimens which have an OCL. This demonstrates that M. rhodesiensis could have reached maturity within two years. The LAGs of these specimens are associated with poorly vascularized annuli.
Carrano et al. (Citation2005) studied the coelophysid Segisaurus halli, which contains bone tissues comprised of longitudinally oriented vascular canals in a WPC with at least five LAGs and no OCL. The specimen (UCMP 32101) was classified as a subadult (Carrano et al., Citation2005). These findings are similar to what we observed in BP/1/4903. However, BP/1/4903 has one LAG and possibly two more annuli and BP/1/6215 has no LAG nor OCL indicating that both of our specimens are ontogenetically younger than S. halli. As with S. halli and M. rhodesiensis, BP/1/4903 experienced periods of slower growth and at least one temporary cessation in growth. Barta and Norell (Citation2019) sampled a large number of C. bauri tibia and fibulae. Their analysis also showed a WPC. The vascular canals are longitudinal and reticular. However, Barta and Norell (Citation2019) found that none of their individuals had an OCL, even those with four LAGs.
DISCUSSION
The two new specimens presented here, BP/1/6215 and BP/1/4903, most likely represent juvenile or early subadult individuals of different theropod taxa. This assertion is supported by consilient evidence from both comparative anatomy and osteohistology. Both BP/1/6215 and BP/1/4903 bear strong similarity to the distal tibiae of early branching neotheropods in bearing an anterolateral process that originates close to the medial side of the distal tibia with a distal margin oblique to the distal margin of the tibia. Moreover, they can readily be distinguished from other known Elliot Formation non-theropod dinosaurs in their gross morphology, and from known Megapnosaurus specimens by their much larger size ().
FIGURE 4. Osteohistology of two theropod tibiae from the Early Jurassic of South Africa compared with a fully grown Megapnosaurus rhodesiensis tibia. A, BP/1/6125 tibia with no visible growth marks is the largest of the set. B, BP/1/4903 tibia has one clear LAG and possibly two other growth marks. C, adult M. rhodesiensis (BPI001). The study specimens are both juveniles/early subadults and larger than the largest known M. rhodesiensis specimen. Scale bar equals 2 mm. Abbreviation: MC, medullary cavity.
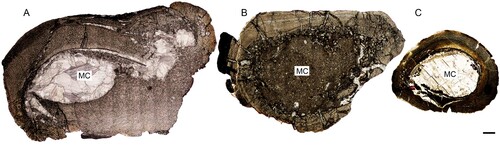
The most salient morphological difference between BP/1/6215 and BP/1/4903 is the angle of the distal margin of the anterolateral process, which differs by nearly 20° relative to the distal margin of the tibia. Although phenotypic variability in early branching theropods is known to be relatively high, to our knowledge the extent of the variation in the distal tibia described here has never been reported for an ontogenetic series of dinosaur. Comparisons to other neotheropods with preserved tibiae also indicate that this angle is variable and supports distinctions among genera (Ezcurra & Brussatte, Citation2011:fig. 2; Nesbitt & Ezcurra, Citation2015:fig. 2). Another major difference is in the position and shape of the proximodistal ridge on the posterior surface, which is weakly expressed in BP/1/6215 and developed closer to the posterior midline and is strongly expressed in BP/1/4903 and is placed on the lateral side. Other, smaller differences include the concavity of the lateral margin of the tibial shaft and the extent of the lateral side of the distal border of the anterolateral process. These features could plausibly differ due to preservation, although we note that in BP/1/6215 the available evidence suggests that the distal fibula was much more closely appressed to the anterior surface of the distal tibia than in BP/1/4903 and both of these differences reflect that distinction.
The two distal tibiae described here bear suites of features common in early branching neotheropods, including the genera Berberosaurus, Camposaurus, Coelophysis, Cryolophosaurus, Dilophosaurus, Lepidus, Liliensternus, Megapnosaurus, Tachiraptor, and Zupaysaurus. While these characteristics in general support phylogenetic positions close to the origins of Neotheropoda, neither the suite of features present in BP/1/4903 nor BP/1/6215 overlap completely with comparable neotheropod species. This indicates that the distal tibial morphology of early neotheropods is relatively plastic and does not warrant ascribing either of the Elliot specimens to known neotheropod genera with preserved tibiae.
The combined osteohistological evidence of the presence of WPC, lack of an OCL, and minimal endosteal remodeling clearly shows that neither BP/1/4903 nor BP/1/6215 had reached skeletal maturity at the time of death. These osteohistological features are typical in theropods (Padian & Woodward, Citation2021), particularly in individuals in the exponential phase of their growth curves (Erickson et al., Citation2001), and suggests that BP/1/4903 and BP/1/6215 were in the early part of their most rapid growth period. This indicates that both specimens had the potential to grow substantially larger during their ontogeny. For example, in this portion of its growth curve, Megapnosaurus increases about 400% in body mass, and larger-bodied theropods have proportionally larger increases (Erickson et al., Citation2001). Should BP/1/4903 and BP/1/6215 followed similar growth trajectories, their adult size would have been among the largest of the known early Jurassic theropods, such as Zupaysaurus and Dilophosaurus. These findings highlight the importance of knowing the ontogenetic age of specimens when performing body size studies (Qin et al., Citation2019).
Body size increase of theropods across the ETE has been a key focus of several studies (e.g. Olsen et al., Citation2002; Griffin, Citation2019; Griffin & Nesbitt, Citation2020), and serves as an example of evolutionary replacement following a mass extinction event (e.g. Olsen et al., Citation2002; Wignall & Atkinson, Citation2020). However, most known dinosaur specimens from the Triassic–Jurassic boundary were skeletally immature individuals (e.g. Chinsamy, Citation1990; Griffin, Citation2019; Chapelle et al., Citation2021, Citation2022; Botha et al., Citation2022). Our findings add more immature specimens to this fossil record, underscoring the need for caution when measuring specimens for assessing macroevolutionary hypotheses regarding the effects of mass extinction, such as the ‘Lilliput effect’.
Currently, there are only two described species of theropod dinosaur from the upper Elliot Formation of South Africa: the coelophysoid M. rhodesiensis and the dilophosaurid Dracovenator regenti. The maximum body size of BP/1/4903 and BP/1/6215 is likely much greater than that reached by known specimens of Megapnosaurus () and potentially as large as Dracovenator, which was about 350 kg (Yates, Citation2005; Benson et al., Citation2014). The difference in growth marks and the comparative anatomy indicates that BP/1/4903 and BP/1/6215 do not represent an ontogenetic series. Although growth and anatomical plasticity has been documented in early branching members of dinosaur lineages (e.g. Griffin, Citation2018; Chapelle et al., Citation2021; Barta et al., Citation2022) such extreme differences in the morphology of the distal tibia along an ontogenetic series are to our knowledge unknown.
Dracovenator regenti is the only described theropod from the upper Elliot Formation that likely reached a size comparable to the tibiae presented here. However, it is known from a single specimen that lacks appendicular elements, precluding comparison between it and BP/1/4903 or BP/1/6215. It is therefore possible that one of the specimens represents D. regenti, while the other represents a distinct taxon. The stratigraphic position of Dracovenator in the upper Elliot Formation is similar to that of BP/1/6215, but lower than that of reported Tritylodon specimens (Bordy et al., Citation2020), suggesting that BP/1/4903 is younger in age. Because upper Elliot Formation taxa can have relatively broad stratigraphic ranges and D. regenti is a singleton representative of its species, we cannot use stratigraphic arguments to rule out D. regenti affinities for either. Regardless, the two tibiae represent medium-large bodied theropods that likely would have been among the top predators in the Early Jurassic upper Elliot ecosystems.
The present study presents two distal theropod tibiae from the upper Elliot Formation of South Africa. Osteohistology, comparative anatomy, and size comparisons show that neither specimen can be ascribed to Megapnosaurus rhodesiensis, the most common upper Elliot theropod taxon, and that at most one specimen could pertain to Dracovenator regenti. Their differences in morphology and microstructure additionally show that they represent separate taxa. Neither specimen presents an OCL, indicating that both were skeletally immature at the time of death. These findings show that there is at least one additional medium-sized theropod dinosaur taxon present in the upper Elliot Formation that remains unknown from more diagnostic material.
ACKNOWLEDGMENTS
We thank S. Gubuza for her aid in thin sectioning the material, B. Zipfel and S. Jirah for assisting in the collections. This research was funded by the National Research Foundation for BMW’s honors degree, JB (GUN No. 117704 and 136513) and JNC (GUN No. 118794 and 136516), JB was funded by the Palaeontological Scientific Trust (PAST), and JB and JNC were funded by the GENUS (DSI-NRF Centre of Excellence in Palaeosciences). The South African Heritage Resources Agency is thanked for their role in granting destructive sampling permits.
LITERATURE CITED
- Allain, R., Tykoski, R., Aquesbi, N., Jalil, N. E., Monbaron, M., Russell, D., & Taquet, P. (2007). An abelisauroid (Dinosauria: Theropoda) from the Early Jurassic of the High Atlas Mountains, Morocco, and the radiation of Ceratosaurs. Journal of Vertebrate Paleontology, 27(3), 610–624.
- Barta, D., & Norell, M. (2019). Bone histology reveals high individual variation in growth among a population of early dinosaurs. 11th North American Paleontological Conference, 68.
- Barta, D. E., Griffin, C. T., & Norell, M. A. (2022). Osteohistology of a Triassic dinosaur population reveals highly variable growth trajectories typified early dinosaur ontogeny. Scientific Reports 12, 17321.
- Benson, R. B. J., Campione, N. E., Carrano, M. T., Mannion, P. D., Sullivan, C., Upchurch, P., & Evans, D. C. (2014). Rates of dinosaur body mass evolution indicate 170 million years of sustained ecological innovation on the avian stem lineage. PLoS Biology, 12(5), e1001853.
- Bordy, E. M., Abrahams, M., Sharman, G. R., Viglietti, P. A., Benson, R. B. J., McPhee, B. W., Barrett, P. M., Sciscio, L., Condon, D., Mundil, R., Rademan, Z., Jinnah, Z., Clark, J. M., Suarez, C. A., Chapelle, K. E. J., & Choiniere, J. N. (2020). A chronostratigraphic framework for the upper Stormberg Group: Implications for the Triassic-Jurassic boundary in southern Africa. Earth-Science Reviews, 203, 103120.
- Botha-Brink, J., Bento Soares, M., & Martinelli, A. G. (2018). Osteohistology of Late Triassic prozostrodontian cynodonts from Brazil. PeerJ, 6(6), e5029.
- Botha, J., Choiniere, J. N., & Barrett, P. M. (2022). Osteohistology and taphonomy support social aggregation in the early ornithischian dinosaur Lesothosaurus diagnosticus. Palaeontology, 65(4), 1–21.
- Brusatte, S. L., Benton, M. J., Ruta, M., & Lloyd, G. T. (2008). Superiority, competition, and opportunism in the evolutionary radiation of dinosaurs. Science, 321(5895), 1485–1488.
- de Buffrénil, V., de Ricqlès, A., Zylberberg, L., & Padian, K. (Eds.). (2021a). Vertebrate Skeletal Histology and Paleohistology. CRC.
- de Buffrénil, V., Quilhac, A., & Castanet, J. (2021b). Cyclical growth and skeletochronology. In V. de Buffrénil, A. de Ricqlès, L. Zylberberg, & K. Padian (Eds.), Vertabrate Skeletal Histology and Paleohistology (pp. 626–644). CRC.
- Butler, R. J., Smith, R. M. H., & Norman, D. B. (2007). A primitive ornithischian dinosaur from the Late Triassic of South Africa, and the early evolution and diversification of Ornithischia. Proceedings of the Royal Society B: Biological Sciences, 274(1621), 2041–2046.
- Carpenter, K. (1997). A giant coelophysoid (Ceratosauria) theropod from the upper Triassic of New Mexico, USA. Neues Jahrbuch Fur Geologie Und Palaontologie - Abhandlungen, 205(2), 189–208.
- Carrano, M. T., Hutchinson, J. R., & Sampson, S. D. (2005). New information on Segisaurus halli, a small theropod dinosaur from the Early Jurassic of Arizona. Journal of Vertebrate Paleontology, 25(4), 835–849.
- Chapelle, K. E. J., Botha, J., & Choiniere, J. N. (2021). Extreme growth plasticity in the early branching sauropodomorph Massospondylus carinatus. Biology Letters, 17(5), 20200843.
- Chapelle, K. E., Barrett, P. M., Choiniere, J. N., & Botha, J. (2022). Interelemental osteohistological variation in Massospondylus carinatus and its implications for locomotion. PeerJ, 10, e13918.
- Chinsamy, A. (1990). Physiological implications of the bone history of Syntarsus rhodesiensis (Saurischia: Theropoda). Palaeontologia Africana, 27, 77–82.
- Cope, E. D. (1887). The dinosaurian genus Coelurus. The American Naturalist 21:367–369.
- Cormack, D. H. (1987). Ham’s histology, 9th edition. Lippincott Williams and Wilkins, Philadelphia.
- Enlow, D. H. (1963). Principles of bone remodeling: An account of post-natal growth and remodeling processes in long bones and the mandible (F. G. Evans (ed.)). Charles C. Thomas, Springfield.
- Erickson, G. M., Rogers, K. C., & Yerby, S. A. (2001). Dinosaurian growth patterns and rapid avian growth rates. Nature, 20, 429–433.
- Ezcurra, M. D., & Novas, F. E. (2007). Phylogenetic relationships of the Triassic theropod Zupaysaurus rougieri from NW Argentina. Historical Biology, 19(1), 35–72.
- Ezcurra, M. D., & Brusatte, S. L. (2011). Taxonomic and phylogenetic reassessment of the early neotheropod dinosaur Camposaurus arizonensis from the Late Triassic of North America. Palaeontology, 54(4), 763–772.
- Ezcurra, M. D. (2017). A new early coelophysoid neotheropod from the Late Triassic of northwestern Argentina. Ameghiniana, 54(5), 506–538.
- Griffin, C. T. (2018). Developmental patterns and variation among early theropods. Journal of Anatomy, 232(4), 604–640.
- Griffin, C. T. (2019). Large neotheropods from the Upper Triassic of North America and the early evolution of large theropod body sizes. Journal of Paleontology, 93(5), 1010–1030.
- Griffin, C. T., & Nesbitt, S. J. (2020). Does the maximum body size of theropods increase across the Triassic–Jurassic boundary? Integrating Ontogeny, Phylogeny, and Body Size. Anatomical Record, 303(4), 1158–1169.
- Haughton, S. H. (1924). The fauna and stratigraphy of the Stormberg Series. Annals of the South African Museum, 12, 393–497.
- Haughton, S. H., & Brink, A. S. (1954). A bibliographic list of reptillia from the Karroo beds of Africa. Palaeontologia Africana, 2, 1–171.
- Horner, J. R., De Ricqlès, A., & Padian, K. (1999). Variation in dinosaur skeletochronology indicators: Implications for age assessment and physiology. Paleobiology, 25(3), 295–304.
- Kitching, J. W., & Raath, M. A. (1984). Fossils from the Elliot and Clarens Formations (Karoo Sequence) of the northeastern Cape, Orange Free State and Lesotho, and a suggested biozonation based on tetrapods. Palaeontologia Africana, 25, 111–125.
- Knoll, F. (2004). Review of the tetrapod fauna of the “Lower Stormberg Group” of the main Karoo Basin (southern Africa): implication for the age of the Lower Elliot Formation. Bulletin de La Société Géologique de France, 175(1), 73–83.
- Knoll, F. (2005). The tetrapod fauna of the Upper Elliot and Clarens formations in the main Karoo Basin (South Africa and Lesotho). Bulletin de La Société Géologique de France, 176(1), 81–91.
- Langer, M. C., Rincón, A. D., Ramezani, J., Solórzano, A., & Rauhut, O. W. M. (2014). New dinosaur (Theropoda, stem-Averostra) from the earliest Jurassic of the La Quinta formation, Venezuelan Andes. Royal Society Open Science, 1(2).
- Marsh, A. D., & Rowe, T. B. (2020). A comprehensive anatomical and phylogenetic evaluation of Dilophosaurus wetherilli (Dinosauria, Theropoda) with descriptions of new specimens from the Kayenta Formation of northern Arizona. Journal of Paleontology, 94(S78), 1–103.
- McPhee, B. W., Bordy, E. M., Sciscio, L., & Choiniere, J. N. (2017). The sauropodomorph biostratigraphy of the Elliot Formation of southern Africa: Tracking the evolution of Sauropodomorpha across the Triassic-Jurassic boundary. Acta Palaeontologica Polonica, 62(3), 441–465.
- Nesbitt, S. J., & Ezcurra, M. D. (2015). The early fossil record of dinosaurs in North America: A new neotheropod from the base of the upper Triassic Dockum group of Texas. Acta Palaeontologica Polonica, 60(3), 513–526.
- Olsen, P. E., & Galton, P. M. (1984). A review of the reptile and amphibian assemblages from the Stormberg of southern Africa, with special emphasis on the footprints and the age of the Stormberg. Palaeontologia Africana, 25, 87–110.
- Olsen, P. E., Kent, D. V., Sues, H.-D., Koeberl, C., Huber, H., Montanari, A., Rainforth, E. C., Fowell, S. J., Szajna, M. J., & Hartline, B. W. (2002). Ascent of dinosaurs linked to an iridium anomaly at the Triassic-Jurassic boundary. Science, 296(5571), 1305–1307.
- Padian, K., & Woodward, H. N. (2021). Archosauromorpha: Avemetatarsalia – Dinosaurs and their relatives. In V. de Buffrénil, A. de Ricqlès, L. Zylberberg, & K. Padian (Eds.), Vertabrate Skeletal Histology and Paleohistology (pp. 511–549). CRC.
- Prondvai, E., Stein, K. H. W., de Ricqlès, A., & Cubo, J. (2014). Development-based revision of bone tissue classification: The importance of semantics for science. Biological Journal of the Linnean Society, 112(4), 799–816.
- Qin, Z., Clark, J., Choiniere, J., & Xu, X. (2019). A new alvarezsaurian theropod from the Upper Jurassic Shishugou Formation of western China. Scientific Reports, 9(1), 1–15.
- Raath, M. A. (1969). A new coelurosaurian dinosaur from the Forest Sandstone of Rhodesia. Arnoldia, 4(28), 1–25.
- Rauhut, O. W. M. (2003). The Interrelationships and Evolution of Basal Theropod Dinosaurs. Special Papers in Palaeontology, 69, 1–213.
- Schneider, C. A., Rasband, W. S., & Eliceiri, K. W. (2012). NIH Image to ImageJ: 25 years of image analysis. Nature Methods, 9(7), 671–675.
- Sciscio, L., Bordy, E. M., Reid, M., & Abrahams, M. (2016). Sedimentology and ichnology of the Mafube dinosaur track site (Lower Jurassic, eastern Free State, South Africa): A report on footprint preservation and palaeoenvironment. PeerJ, 2016(8).
- Shen, C., Lü, J., Gao, C., Hoshino, M., Uesugi, K., & Kundrát, M. (2019). Forearm bone histology of the small theropod Daliansaurus liaoningensis (Paraves: Troodontidae) from the Yixian Formation, Liaoning, China. Historical Biology, 31(2), 253–261.
- Smith, R., & Kitching, J. (1997). Sedimentology and vertebrate taphonomy of the Tritylodon Acme Zone: A reworked palaeosol in the Lower Jurassic Elliot Formation, Karoo supergroup, South Africa. Palaeogeography, Palaeoclimatology, Palaeoecology, 131(1–2), 29–50.
- Smith, N. D., Makovicky, P. J., Hammer, W. R., & Currie, P. J. (2007). Osteology of Cryolophosaurus ellioti (Dinosauria: Theropoda) from the Early Jurassic of Antarctica and implications for early theropod evolution. Zoological Journal of the Linnean Society, 151(2), 377–421.
- Tykoski, R. S. 2005. Anatomy, ontogeny, and phylogeny of coelophysoid theropods. [Doctoral thesis, The University of Texas].
- Viglietti, P. A., McPhee, B. W., Bordy, E. M., Sciscio, L., Barrett, P. M., Benson, R. B. J., Wills, S., Tolchard, F., & Choiniere, J. N. (2020a). Biostratigraphy of the Scalenodontoides Assemblage Zone (Stormberg Group, Karoo Supergroup), South Africa. South African Journal of Geology, 123(2), 239–248.
- Viglietti, P. A., McPhee, B. W., Bordy, E. M., Sciscio, L., Barrett, P. M., Benson, R. B. J., Wills, S., Chapelle, K. E. J., Dollman, K. N., Mdekazi, C., & Choiniere, J. N. (2020b). Biostratigraphy of the Massospondylus Assemblage Zone (Stormberg Group, Karoo Supergroup), South Africa. South African Journal of Geology, 123(2), 249–262.
- Wignall, P. B., & Atkinson, J. W. (2020). A two-phase end-Triassic mass extinction. Earth-Science Reviews, 208(July), 103282.
- Woodward, H. N., Rich, T. H., Chinsamy, A., & Vickers-Rich, P. (2011). Growth dynamics of Australia’s polar dinosaurs. PLoS ONE, 6(8), 4–8.
- Yates, A. M. (2005). A new theropod dinosaur from the Early Jurassic of South Africa and its implications for the early evolution of theropods. Palaeontologica Africana, 41, 105–122.