ABSTRACT
Pliocene South American mammals prior to the Great American Biotic Interchange comprise the evolutionary pinnacle of a long-lasting process of continental isolation, in which large-scale orogeny and climate change were instrumental. These processes resulted in unique assemblages of extinct groups with peculiar anatomies. However, interpretation of the functional roles that these organisms would have played in the ecosystem is challenging because well-preserved fossils of this time interval in South America are scarce. Here, we describe a new Early Pliocene assemblage of native herbivorous megamammals documented by skeletons in anatomical connection from the Peruvian Andean plateau (city of Yauri in Espinar, Cusco Department). Fossil-bearing deposits pertain to Member C of the El Descanso Formation dated at 4.75 ± 0.5 Ma, when the plateau attained near-modern elevations and a Puna-type plant ecosystem. The mammal assemblage is dominated by xenarthrans, including three ground sloths (cf. Megatheriops rectidens, Simomylodon uccasamamensis, and Proscelidodon sp.) and Andinoglyptodon mollohuancai gen. et sp. nov., the first Pliocene glyptodontine known by a well-preserved cranium and dermal armor. Notoungulates are represented by the toxodontid Posnanskytherium viscachanense. Both selective and bulk feeders are identified, suggesting the existence of diverse plant resources in the Pliocene Puna ecosystem. These native mammals constituted the typical Pliocene herbivorous guild of the central Andean plateau and provide an opportunity to investigate the structure of mammalian communities just before the arrival of Holarctic immigrants.
RESUMEN
Los mamíferos sudamericanos del Plioceno previos al Gran Intercambio Biótico Americano constituyen el pináculo evolutivo de un proceso prolongado de aislamiento continental, en el que la orogenia a gran escala y el cambio climático fueron fundamentales. Estos procesos resultaron en ensambles únicos de grupos extintos con anatomías inusuales. Sin embargo, la interpretación de los roles funcionales que estos organismos habrían jugado en el ecosistema es un reto porque fósiles bien preservados de este intervalo temporal en Sudamérica son escasos. Aquí, describimos un nuevo ensamble de megamamíferos herbívoros nativos del Plioceno temprano documentado por esqueletos en conexión anatómica del altiplano Andino peruano (ciudad de Yauri en Espinar, departamento de Cusco). Los depósitos con fósiles pertenecen al Miembro C de la Formación El Descanso datados en 4.75 ± 0.5 Ma, cuando el altiplano alcanzó elevaciones casi iguales a las modernas y un ecosistema de plantas de tipo Puna. El ensamble de mamíferos está dominado por xenartros, incluídos tres perezosos terrestres (cf. Megatheriops rectidens, Simomylodon uccasamamensis y Proscelidodon sp.) y Andinoglyptodon mollohuancai gen. et sp. nov., el primer gliptodonte del Plioceno conocido por elementos craneales y la armadura dérmica bien conservados. Los notungulados están representados por el toxodóntido Posnanskytherium viscachanense. Se identificaron tanto alimentadores selectivos como masivos, lo que sugiere la existencia de diversos recursos vegetales en el ecosistema de la Puna del Plioceno. Estos mamíferos nativos constituyeron el gremio de herbívoros típico del Plioceno del altiplano andino central y proveen una oportunidad para investigar la estructura de las comunidades de mamíferos justo antes de la llegada de los inmigrantes holárticos.
INTRODUCTION
The modern Central Andean Plateau is characterized by physical traits such as extreme daily thermal oscillations, low relative humidity, high elevation, and reduced partial pressure of oxygen, which are believed to be responsible for the relatively low plant diversity in this ecosystem (Baied & Wheeler, Citation1993). Today, this altiplanic ecoregion (also known as Puna) supports an equally low diversity of herbivorous mammals whose biggest inhabitant, the camelid vicugna (Vicugna vicugna), does not exceed 50 kg (Acebes et al., Citation2018). Albeit some differences resulting from slightly wetter conditions (inferred by a higher number of fern taxa), these physical and floral traits typical of the modern Andean Plateau, were already in place by the Early Pliocene (Kar et al., Citation2016, Martínez et al., Citation2020). Herbivorous mammalian communities supported by this plant ecosystem in the Pliocene, however, differed drastically, not only in terms of taxonomic composition and diversity, but particularly in terms of body mass. Indeed, fossiliferous localities in the Pliocene, right before the main pulse of faunal arrival from North America during the Great American Biotic Interchange (GABI), document a rich mammalian diversity in the Andean Plateau with at least eight megamammal (i.e., > 40 kg) species (see Anaya & MacFadden, Citation1995).
Fossils from Pliocene localities in the Andean Plateau are also known from Bolivia (e.g., Pomata-Ayte: Marshall et al., Citation1983; Pujos et al., Citation2016; Inchasi: Anaya & MacFadden, Citation1995; Ayo Ayo-Viscachani: Boscaini, Fernández-Monescillo et al., Citation2021). Other Andean localities with Late Miocene–Pliocene pre-GABI herbivorous mammals are known from Argentina (e.g., Maimará of Jujuy: Reguero & Candela, Citation2008; Huayquerías of Mendoza: Garrido et al., Citation2017; Santa María of Tucumán and Catamarca: Nasif et al., Citation2019).
In 2005, the first fossil mammal from the Peruvian Andean Plateau was discovered in the locality of Espinar, while excavations were being carried out for the construction of a soccer stadium in the city of Yauri (Tejada, Baez et al., Citation2009; Tejada, Gamarra et al., Citation2009). Since then, several well-preserved specimens have been recovered in the city documenting a rich pre-GABI fossil mammalian community. The paleontological record also includes finely preserved fishes, ostracods, diatoms, leaves, and fruits (Martínez et al., Citation2020; Tejada, Baez et al., Citation2009; Vélez et al., Citation2018). Here, we provide the first formal description of the Early Pliocene mammalian assemblage from Espinar, including one new glyptodontid species that reveals ecological differences between Pliocene and Pleistocene forms. Documenting the Espinar mammalian community during the Early Pliocene, shortly after the landscape would have reached near-modern elevations, acquired modern environmental conditions, and before the arrival of immigrant herbivores during the GABI is important for several reasons. First, from an anatomical perspective, the information preserved in the Espinar fossil mammals allows assessment of character evolution associated with changes of feeding ecology in the Andes as well as a critical evaluation of the taxonomic significance of some morphological characters. Second, the Espinar fauna allows the evaluation of the regional impact of the rise of the Andes among coeval mammalian communities across the continent. Third, it represents crucial evidence toward understanding mammal community structures just before the arrival of Holarctic immigrants.
GEOGRAPHIC AND GEOLOGIC SETTING
The Andes are home to numerous intermontane basins containing thick continental successions, with the Descanso-Yauri basin in southern Peru being one of the best-known in the southern Peruvian region. It contains a 1.8 km-thick Mio–Pliocene sequence associated with the Descanso Formation. These exposures consist of three lithostratigraphic units, in ascending order: Member A, Member B, and Member C (Carlotto, Citation2013; Cerpa et al., Citation2002). Fossil remains discussed in this paper were collected from the latter unit. Member C is composed of finely laminated fossiliferous diatomaceous silt- and sandstones, interbedded with massive to cross-bedded sandstone and conglomerate beds, as well as local syndepositional ash beds. It represents a N-NE flowing fluvial system that gradually gave rise to lacustrine and palustrine environments (Cerpa et al., Citation2002), preserving abundant plant (pollen, leaves, wood), invertebrate (ostracods), and vertebrate (fish, mammal) remains. The age of Member C was recently assessed as latest Miocene to Early Pliocene (5.4–3.9 Ma; Kar et al., Citation2016; Martínez et al., Citation2020). In this study, a 3 m-thick ash outcropping on the NW margin of the Yauri city, near the area of the first paleontological discoveries, was further analyzed for U/Pb radiometric dating (sample E19-14; 14°784′S, 71°418′W; , S1–S3). U/Pb analyses of zircons from this ash yielded an age of 4.75 ± 0.5 Ma (see Supplementary Data 1, Table S1). Mammal remains described herein are correlated with sedimentary levels from the Versalles area (Martínez et al., Citation2020; 3907 m a.s.l.), but are considered at least 1 My older than those outcropping at Cerro Púcara (Garbage Pit locality, 3937 m a.s.l.; Vélez et al., Citation2018).
FIGURE 1. A, location of Yauri city (Espinar) in relation to other landmarks in southern Peru. B, map of Yauri (Espinar) indicating sampled tuff (star, E19-14), existing radiometric dates (squares), and known fossiliferous localities (dots) as follows: 1, Santísima Cruz [MUESP 21]; 2, Alto Huarca; 3, Huacracanto [MUESP 10]; 4, Huisaccollana [MUESP 11/ESP 9-1]; 5, Villa Versalles [MUESP 4, MUESP8, MUESP 9/ESP6]; 6, AAHH A A Cáceres [MUESP 20]; 7, Estadio [MUESP 3]; 8, NW-Estadio [E19-13]; 9, Los Angeles; 10, Versalles tuff (Martínez et al., Citation2020); 11, Cerro Pucará (Kar et al., Citation2016). C, chronostratigraphic position of fossiliferous localities (numbers as in inset B) and existing radiometrically dated tuffs from Member C (†Kar et al., Citation2016; ‡Martínez et al., Citation2020). Maps sources: 30 arc-second DEM of South America (Data Basin Dataset), U.S. Geological Survey's Center for Earth Resources Observation and Science (EROS).
![FIGURE 1. A, location of Yauri city (Espinar) in relation to other landmarks in southern Peru. B, map of Yauri (Espinar) indicating sampled tuff (star, E19-14), existing radiometric dates (squares), and known fossiliferous localities (dots) as follows: 1, Santísima Cruz [MUESP 21]; 2, Alto Huarca; 3, Huacracanto [MUESP 10]; 4, Huisaccollana [MUESP 11/ESP 9-1]; 5, Villa Versalles [MUESP 4, MUESP8, MUESP 9/ESP6]; 6, AAHH A A Cáceres [MUESP 20]; 7, Estadio [MUESP 3]; 8, NW-Estadio [E19-13]; 9, Los Angeles; 10, Versalles tuff (Martínez et al., Citation2020); 11, Cerro Pucará (Kar et al., Citation2016). C, chronostratigraphic position of fossiliferous localities (numbers as in inset B) and existing radiometrically dated tuffs from Member C (†Kar et al., Citation2016; ‡Martínez et al., Citation2020). Maps sources: 30 arc-second DEM of South America (Data Basin Dataset), U.S. Geological Survey's Center for Earth Resources Observation and Science (EROS).](/cms/asset/11e91000-13fe-4760-b8b4-9e86d9737cd6/ujvp_a_2237079_f0001_oc.jpg)
MATERIAL AND METHODS
Fossil mammals are housed in the Dirección Desconcentrada de Cultura de Cusco (Cusco, Peru) and the Museo Municipal de Espinar (MUESP; Espinar, Peru). Remains of the new glyptodontine (MUESP 4) are described and compared with other members of the clade. Preliminary taxonomic identifications and anatomical data are provided for other mammals recorded in Member C. These identifications are mainly based on cranial material. Most postcranial and some cranial elements are not yet prepared and therefore not available for analyses. Comparisons were limited to most important and diagnostic characters. Number designation for molariforms in Cingulata and Pilosa is merely topological and does not imply homology. Anatomical terminology for cingulates follows Gillette and Ray (Citation1981). For the cingulate exoskeleton, the descriptions are restricted to the osseous dermal armor composed of bony elements called ‘osteoderms.’ Dietary categories follow the morphofunctional criteria of Bargo et al. (Citation2006). Body mass estimations were calculated for the sloths and the toxodontid following Toledo et al. (Citation2014) and Damuth (Citation1990), but not for the new glyptodontine because estimations for these animals require postcranial data that are not available (see Fariña, Citation1995; Vizcaíno et al., Citation2011a). Relative muzzle width (i.e., width at lingual Mf4/width at labial Mf1; RMW) was calculated for the glyptodontine to evaluate putative feeding habits after Vizcaíno et al. (Citation2011b). Description of all anatomical measurements, and calculations of body mass and RMW are available in the Supplementary Data 1 (Appendices 2–4; Figs. S4, S5). In this study, we do not use the mammalian biostratigraphic units proposed for South America (i.e., “South American Land Mammal Ages” or SALMAs) due to their uncertain definition and pertinence for the late Cenozoic interval outside the Pampean region (see Cione & Tonni, Citation1996; Tomassini et al., Citation2013).
Institutional Abbreviations––CAL, Colección Paleontológica, Centro de Museos, Universidad de Caldas, Manizales, Caldas, Colombia; MACN-Pv, Museo Argentino de Ciencias Naturales “Bernardino Rivadavia,” Buenos Aires, Argentina; MLP, Museo de La Plata, La Plata, Argentina; MMP, Museo Municipal de Ciencias Naturales “Lorenzo Scaglia,” Mar del Plata, Argentina; MNHN, Museum National d’Histoire Naturelle, Paris, France; MNHN-Bol, Museo Nacional de Historia Natural, La Paz, Bolivia; MUESP, Museo Municipal de Espinar, Cusco, Peru; MUSM, Museo de Historia Natural de la Universidad Nacional Mayor de San Marcos, Lima, Peru.
Anatomical Abbreviations––BZYW, bizygomatic width; Cf and cf, upper and lower caniniform teeth, respectively; Mf and mf, upper and lower molariform teeth, respectively.
Taxonomic Considerations––The Glyptodontinae clade encompasses the genera Boreostemma Carlini et al., Citation2008 (Middle Miocene), Glyptodontidium Cabrera, Citation1944 (Late Miocene), Glyptodon Owen, Citation1838 (Pleistocene), and Glyptotherium Osborn, Citation1903 (Pleistocene), the latter from northernmost South America and North America (Carlini et al., Citation2008; Gillette & Ray, Citation1981). The validity of the genus Paraglyptodon Rovereto, Citation1914 (Late Pliocene) has been questioned due to the absence of distinct features in the two Paraglyptodon species (P. chapalmalensis Ameghino in Rovereto, Citation1914 and P. uquiensis Castellanos, Citation1953) relative to species of Glyptodon (Cruz et al., Citation2016). Here, Paraglyptodon is referred for comparisons and the validity of the genus is discussed. The Late Miocene megatheriine Megatheriops Ameghino and Kraglievich, Citation1921 is represented by a single species, Megatheriops rectidens Rovereto, Citation1914 (De Iuliis, Citation1996; Pujos & Salas, Citation2004). This taxon is only known by the type specimen (MACN Pv 2818: partial skull, mandibles, both humeri, right ulna) recovered from the Huayquerías Formation of Mendoza, Argentina (De Iuliis, Citation1996). Similarities have been recognized between Megatheriops and Pyramiodontherium Rovereto, Citation1914 (Pujos, Citation2006), the latter from the Late Miocene–Pliocene of Catamarca, Tucumán, and La Rioja, Argentina and composed by Py. bergi (Moreno & Mercerat, Citation1891), Py. brevirostrum Carlini et al., Citation2002, and Py. scillatoyanei De Iuliis et al., Citation2004. The mylodontine Simomylodon Saint-André et al., Citation2010 was erected based on latest Miocene–Pliocene (∼5.4–2.8 Ma) skeletal material from the Bolivian Plateau and only comprises its type species, S. uccasamamensis (Saint-Andre et al., Citation2010). Pleurolestodon dalenzae is a junior synonym of the mylodontine S. uccasamamensis (Boscaini et al., Citation2018; Boscaini, Toledo et al., Citation2021). The scelidotheriine Proscelidodon Bordas, Citation1935 comes from Late Miocene–Pliocene sediments of Argentina (Miño-Boilini et al., Citation2011, Citation2019; Pujos et al., Citation2012; Taglioretti et al., Citation2014). Its presence in Bolivia (Anaya & MacFadden, Citation1995) has been challenged (Pujos et al., Citation2012). This genus is represented by four species (Pr. patrius, Pr. almagroi, Pr. rothi, and Pr. gracillimus), of which Pr. patrius exhibits a high degree of morphological variation (Pujos et al., Citation2012). Finally, the toxodontid Posnanskytherium Liendo Lazarte, Citation1943 is only known from the Pliocene of the Bolivian Plateau (Anaya & MacFadden, Citation1995; Liendo Lazarte, Citation1943; Saint-André, Citation1999) and comprises four species (Po. desaguaderoi, Po. inchasense, Po. pacis, and Po. viscachanense).
SYSTEMATIC PALEONTOLOGY
XENARTHRA Cope, Citation1889
CINGULATA Illiger, Citation1811
GLYPTODONTIDAE Gray, Citation1869
GLYPTODONTINAE Gray, Citation1869
ANDINOGLYPTODON MOLLOHUANCAI, gen. et sp. nov.
(, , , )
FIGURE 2. Andinoglyptodon mollohuancai gen. et sp. nov. (holotype, MUESP 4). Skull in A, anterior view; B, left lateral view; C, dorsal view; D, occlusal view; E, posterior view. Left mandible in F, occlusal view; G, medial view; H, lateral view. Abbreviations: ac, articular condyle; bo, basioccipital; bs, basisphenoid; cp, coronoid process; dmx, descending process of the maxillae; f, foramina; fr, frontal; iof, infraorbital foramen; lf, lacrimal foramen; Mf1, Mf2, Mf7, Mf8, upper molariform tooth positions; mf1, mf6, mf8, lower molariform tooth positions; mfo, mental foramina; mpsq, mastoid process of the squamosal; nc, nuchal crest; no, narial opening; oc, occipital condyle; or, orbit; pa, parietal; pe, petromastoid; pgf, postglenoid fossa; pop, paraoccipital process; prm, palatal ridge of the maxillae; s, mandibular symphysis; so, supraoccipital; sq, squamosal; ss, symphyseal spout; v, foramen ovale; zysq, zygomatic process of the squamosal. Scale bar equals 5 cm.
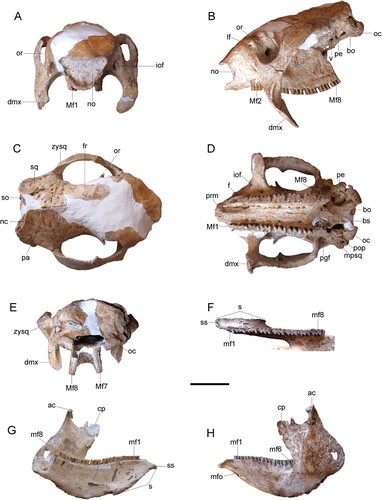
FIGURE 3. Andinoglyptodon mollohuancai gen. et sp. nov. (holotype, MUESP 4). A, dorsal carapace; B, anterior osteoderms; C, lateral osteoderms; D, dorsal osteoderms; E, portion of accessory caudal ring; F, portion of caudal ring 2 or 3; G, H, caudal rings 4, 5, or 6; I, right femur in dorsal view. Abbreviations: dro, distal row of osteoderms; fh, femoral head; gt, greater trochanter; pro, proximal row of osteoderms; pt, patellar trochlea; tt, third trochanter. Scale bars equal 5 cm.
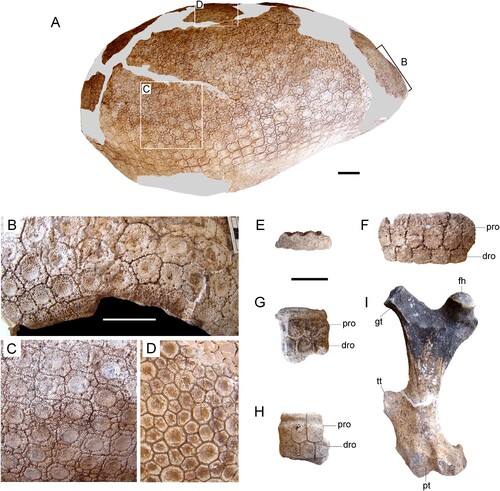
TABLE 1. Cranial and mandibular measurements (mm) of Andinoglyptodon mollohuancai (holotype, MUESP 4). Abbreviation: e, estimated. Measurements modified from Von den Driesch (Citation1976).
Holotype––MUESP 4, partial skull and left mandible of a morphologically adult individual (obliterated skull sutures); reconstructed dorsal carapace including anterior, lateral, and posterodorsal osteoderm portions; left and right femurs; caudal armor: partial accessory ring, three partial caudal rings, and isolated mobile and fixed caudal osteoderms. Original material at MUESP but casts of upper dentition and selected portions of the dorsal carapace are housed at MUSM.
Geographic and Stratigraphic Provenance––Holotype collected in Villa Versalles, city of Yauri, Espinar Province, Cusco Department; Locality ESP6: 14°47.087′S, 71°24.963′W (locality 5, ); discovered on July 2, 2005. Lower levels of Member C of El Descanso Formation, Early Pliocene (4.75 ± 0.5 Ma).
Diagnosis––Glyptodontine similar in size to Boreostemma acostae with relatively long and slender snout (shorter in Paraglyptodon uquiensis, Glyptodon, and Glyptotherium; wider in Glyptodon and Glyptotherium); nasal aperture trapezoid and wider-than-tall (trapezoid and taller-than-wide in other glyptodontines); ventral margin of the orbit closer to the alveolar plane than to the dorsal skull profile; semicircular spout-like predental symphysis; the height of the horizontal ramus increases posteriorly (more homogeneous height along the horizontal ramus in Glyptodon); long anteroposterior base of ascending ramus of the mandible (closer in shape to G. muñizi than to G. clavipes which is anteroposteriorly shorter); relatively slender femur; greater trochanter at the same level of the femoral head (similar to G. reticulatus); third femur trochanter positioned just distal to the midshaft; low level of the articular condyle of the mandibles relative to the tooth row (higher in Glyptodon and Glyptotherium).
Etymology––Andinoglyptodon in reference to the holotype being found in the high Andes; mollohuancai after Oscar Mollohuanca, former mayor of Espinar who fought against economic and political power to protect the environment in Espinar and was killed in unclear circumstances.
Comparative Description––The skull is relatively long and high at the frontoparietal region. From the posterior limit of the orbits, the rostrum descends anteriorly, thus the snout is low relative to other glyptodontines, such as MPP 4676 (referred to cf. P. chapalmalensis by Oliva et al., Citation2010), P. uquiensis (MACN-Pv 5377), Glyptodon and Glyptotherium, in which the snout is much higher. The attenuation of the rostrum is reinforced by the distinct sigmoidal shape of the alveolar plane in lateral view, which is elevated anterior to the zygomatic arch (). In palatal view, right and left upper dental series (Mf1–Mf8) are roughly parallel to each other, only diverging slightly posteriorly (). Molariforms are fully trilobate, except for Mf1 which is only weakly lobated on the labial margin. As in Glyptodon, there is an axial palatal ridge in the maxillae at the level of Mf1 (). The palate bears several large foramina, particularly at the level of Mf2 and Mf3. The RMW (width at lingual Mf4/width at labial Mf1) in Andinoglyptodon is 0.742, comparable to that of Propalaehoplophorus australis (0.745) and slightly higher than those of Glyptodon species (0.631–0.714; Vizcaíno et al., Citation2011b).
In Andinoglyptodon the snout is not truncated, as opposed to that of Glyptodon, Glyptotherium, and possibly Paraglyptodon (Cuadrelli et al., Citation2018; Gillette & Ray, Citation1981; Oliva et al., Citation2010; Soibelzon et al., Citation2010). The narial opening bears a shape of an inverted trapezoid as in other glyptodontines (, ) except for Boreostemma acostae (Zurita et al., Citation2013). In Andinoglyptodon the narial opening is wider-than-tall (, ), in contrast to the typical taller-than-wide opening of most glyptodontines. The dorsal margin of the narial opening is only slightly concave, differing from the deeply concave margin of Glyptodon. The internasal septum is either not ossified or retreated to the narial passage, and so it is not visible. The lacrimal foramen is surrounded by a rugous surface within the lacrimal bone, which is not particularly inflated as in Glyptodon. The zygomatic arch is massive and the descending process of the maxilla projects posteroventrally (). The postorbital bar is incomplete. In contrast to the high position of the orbits of Paraglyptodon, Glyptodon, and Glyptotherium, in Andinoglyptodon the orbits are located relatively closer to the level of the toothrow. The orbital notch is narrow and U-shaped as in Boreostemma acostae (Zurita et al., Citation2013), whereas this notch bears more equivalent proportions (i.e., circular) in Paraglyptodon uquiensis (MACN-PV 5377), P. chapalmalensis (MPP 4676; ), Glyptodon (), and Glyptotherium. The parietal and occipital regions are similar to those of Glyptodon, with the occipital plane tilted anteriorly. The occipital condyles are lateromedially expanded, a feature considered a synapomorphy of the Glyptodontinae (Fernicola, Citation2008; ).
FIGURE 4. Comparative schematics of glyptodontine skulls. Andinoglyptodon mollohuancai (holotype, MUESP 4): A, anterior view; B, left lateral views. C, Paraglyptodon uquiensis (MACN-Pv 5377) in anterior view. D, cf. Paraglyptodon chapalmalensis (MPP 4676) in lateral view (reversed). E, F, Glyptodon muñizi (MACN 8706) in E, anterior view; F, left lateral view. Scale bar equals 5 cm.
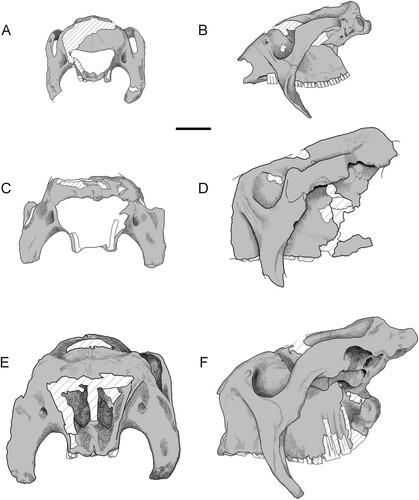
The general shape of the mandible resembles that of Glyptodon and Glyptotherium, with a long tooth row (mf1–mf8) that occupies most of the horizontal ramus and a high anterodorsal ascending ramus, the latter in correspondence with the posterodorsal orientation of jugal-squamosal zygomatic portion. Although the articular condyle is markedly elevated relative to the tooth row (), its position is proportionally lower than in Glyptodon and Glyptotherium. The posterior-most extremity of the ascending ramus is located slightly above the level of the tooth row, closer to the condition of Glyptodon than that of Glyptotherium (Gillette & Ray, Citation1981). It seems that the mandibular rami were not fully fused at the symphysis. The symphysis ends at the level of the anterior lobe of the mf4 and its distal tip turns downward, as in Glyptodon. The tip of the symphysis of Andinoglyptodon bears a slender semicircular spout () whereas this spout is wider and more quadrangular in Glyptodon and Glyptotherium (Gillette & Ray, Citation1981; Soibelzon et al., Citation2010). Two mental foramina are located at the level of mf1. As are the upper teeth, the lower teeth are trilobate, with an incipient lobation in the lingual margin of mf1–mf3. The maximum depth of the horizontal ramus occurs at the level of the m6 (). Although the depth of the horizontal ramus is variable in Glyptodon and Glyptotherium, the maximum depth in these taxa generally occurs anterior to m6 within a ramus of nearly parallel ventral and alveolar margins (except in Glyptotherium arizonae). The mandible is unknown for Paraglyptodon.
The dorsal carapace is preserved but its general shape was distorted during preparation and reconstruction (). The total length of the dorsal carapace is about 831 mm, slightly larger than that of Boreostemma acostae (CAL-896: 770 mm; Zurita et al., Citation2013). In general, osteoderms of the dorsal carapace in Andinoglyptodon are thinner and smaller than those of Paraglyptodon (MACN 6162, MACN-PV 5344a), Glyptodon, and Glyptotherium, but virtually undistinguishable in other features. In Andinoglyptodon, the external surface of the osteoderms is punctate and bears a large central figure separated from a row of peripheral figures by a circular sulcus (). Additional radial sulci limit 6–10 peripheral figures within this single row. The sulci are U-shaped in transversal section. Foramina (hair follicles) are set at most intersections of radial and circular sulci. Within this rosette pattern, the shape of the osteoderms varies from quadrangular in anterior and lateral regions () to hexagonal in dorsal and posterior areas () of the carapace. A faint delimitation between central and peripheral figures characterizes the anterior and lateral osteoderms whereas deep sulci clearly define the central and peripheral figures of the posterodorsal osteoderms. Sutural margins separate osteoderms in the anterior and lateral parts of the carapace, whereas posterodorsal osteoderms are fused and peripheral figures are shared among neighboring osteoderms (). In posterodorsal osteoderms, each figure bears a concavity (central depression), particularly noticeable in the central figure. A faint concavity in the central figure is also observed in dorsal osteoderms close to the anterior aperture (). A similar concavity in the central figure was recognized in some specimens assigned to Paraglyptodon, Glyptodon muñizi, and Glyptodon clavipes from Argentina (Cruz et al., Citation2016), and an adult Pleistocene specimen from Cusco, here referred to G. clavipes (INC-CUS 1). In contrast, Glyptodontidium tuberifer (MLP 39-IV-25-10) bears posterior osteoderms with a convex central figure. Nuchal osteoderms are quadrilateral and flat. The osteoderms of the lateral and posterior borders (caudal notch) are not well preserved, but those preserved bear a flat to slightly convex surface with an enlarged central figure. Thus, the weak or prominent conical bosses of the lateral and posterior borders of the carapace of Glyptodontidium, Paraglyptodon, Glyptodon, and some species of Glyptotherium were probably absent in Andinoglyptodon (). Osteoderms of the distal row of the caudal rings show no evidence of conical bosses as well. In Glyptodon and Glyptotherium, these ring osteoderms usually exhibit a similar geometry to the marginal osteoderms of the caudal notch.
The femur is relatively slender (, ). The greater trochanter reaches the height of the femoral head. The third trochanter is located just distal to the midshaft, resembling Parapropalaehoplophorus (Croft et al., Citation2007). This trochanter is closer to the distal end in Glyptodon. The femur medial length of Propalaehoplophorus australis (198 mm; from Scott, Citation1903) represents 78% of that measurement in Andinoglyptodon (holotype: 255 mm). Because the femur of Andinoglyptodon is not much larger than that Propalaehoplophorus australis, the body mass of the Peruvian form is estimated as similar to or slightly higher than that of Propalaehoplophorus (81.1–93.48 kg: Vizcaíno et al., Citation2011b).
PILOSA Flower, Citation1883
TABLE 2. Measurements (mm) of the femur of Andinoglyptodon mollohuancai (holotype, MUESP 4). Modified from Von den Driesch (Citation1976).
MEGATHERIIDAE Owen, Citation1843
MEGATHERIOPS Ameghino and Kraglievich, Citation1921
Type Species––Megatheriops rectidens Rovereto, Citation1914.
Temporal Range––Late Miocene to Early Pliocene.
Geographic Distribution––Argentina (Mendoza Province) and Peru (Cusco Department).
cf. MEGATHERIOPS RECTIDENS Rovereto, Citation1914
(, )
FIGURE 5. Ground sloths and Toxodontidae from Espinar. A, cf. Megatheriops rectidens, skull (MUESP 10) in left lateral view. Simomylodon uccasamamensis skulls in left lateral view: B, juvenile skull (MUESP 11); C, adult skull (MUESP 16). Proscelidodon sp. (MUESP 20): D, skeleton in anatomical connection; E, skull and mandible in lateral view. F, Posnanskytherium viscachanense, skull (MUESP 3) in left lateral view. The skulls are oriented with the occipital condyles horizontal. Abbreviations: cav, caudal vertebrae; Cf, upper caniniform; crv, cervical vertebra; hu, right humerus; I2, upper incisive tooth 2; j, jugal; lbv, lumbar vertebrae; lf, lacrimal foramen; M1, M3, upper molar tooth 1 and 3; Mf1–Mf3, upper molariform tooth positions; mn, mandible; n, nasal; no, narial opening; oc, occipital condyle; or, orbit; P1, P4, upper premolar tooth 1 and 4; pl, pelvis; pmx, premaxilla; ra, right radius; sa, sacrum; sc, left scapula; sk, skull; trv, thoracic vertebrae; ul, right ulna; zmx, zygomatic process of the maxilla; zysq, zygomatic process of the squamosal. Scale bars equal 5 cm.
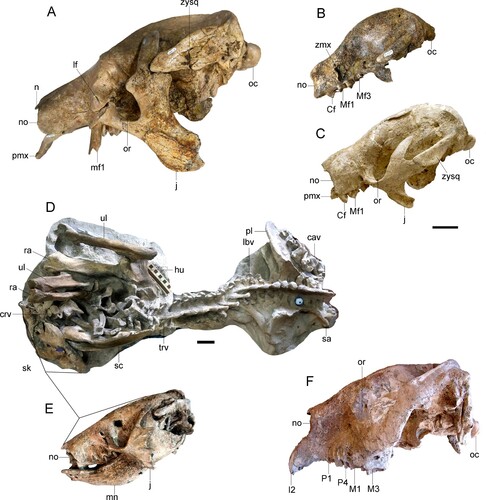
FIGURE 6. Snout and dentition of mammals from Espinar in ventral view. A, Andinoglyptodon mollohuancai; B, cf. Megatheriops rectidens; C, Simomylodon uccasamamensis; D, Posnanskytherium viscachanense. Measurements for the relative muzzle width (1, width at labial Mf1; 4, width at lingual Mf4) are shown for Andinoglyptodon. Scale bar equals 5 cm.
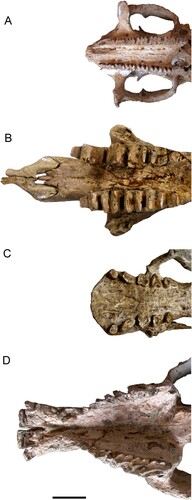
Referred Specimens––MUESP 10, Partial skeleton of a subadult individual, including skull and jaw.
Geographic and Stratigraphic Provenance––Locality Huacracanto, Calle Apurimac, block 2, Huacracanto, city of Yauri, Espinar, Cusco; 14°47.808′S, 71°24.645′W (locality 3 in ). Collected in 2008 by Gregorio “Goyo” Agramonte and JG. Lower levels of the Member C of El Descanso Formation, Early Pliocene (4.75 ± 0.5 Ma).
Comments––MUESP 10 corresponds to a subadult individual slightly smaller than, but with general skull and jaw morphology similar to, the holotype of M. rectidens (MACN 2818), from the Late Miocene of Huayquerías, Mendoza Province, Argentina. As in M. rectidens, MUESP 10 is characterized by having a relatively long and slender cranium with a high, dome-like (convex) skull roof, straight tubular rostrum, upper molariforms oriented anteroventrally, jugal rooted in the maxillae at the level of Mf2, and lower margin of the orbit leveled to the maxillary alveolar plane (, ). The palate between the molariforms is narrow, but probably not as narrow as that of the type of M. rectidens. Molariforms are quadrangular, exclusive of Mf1 which is trapezoid in shape (). Some of the features recognized in the skull of MUESP 10 and Megatheriops rectidens are also observed in species of Pyramiodontherium (Cabrera, Citation1928; Carlini et al., Citation2002), but the latter shows differences in the shape of the rostrum. In Pyramiodontherium species the predental portion of the maxillae bears a lateral expansion toward its anterior end (Carlini et al., Citation2002), whereas in MUESP 10 and M. rectidens this is virtually parallel-sided. Maxillary tooth row is laterally bowed in Pyramiodontherium species, with the alveoli of Mf1 and Mf2 less quadrangular than in MUESP 10 and M. rectidens. The main discrepancy between the Peruvian Megatheriops and the holotype of M. rectidens is the orientation of the occipital condyle, being more downwardly inclined in the latter than in the former. This feature is related with the head posture and its variation might have ecological implications (Coutier et al., Citation2017).
TABLE 3. Selected cranial measurements (mm) of cf. Megatheriops rectidens (MUESP 10), Simomylodon uccasamamensis (MUESP 16), Proscelidodon sp. (MUESP 20), and Posnanskytherium viscachanense (MUESP 3). Abbreviation: e, estimated. Measurements modified from Von den Driesch (Citation1976).
MUESP 10 preserved both premaxillae. They are Y-shaped, anteroventrally inclined, and not fused to each other nor to the maxillae (, ). The lateral ramus of the premaxillae is stout and contacts the maxillae within a deep U-shaped notch. The medial ramus is reduced to a nubbin but bears a sutural contact with an anterior odd process of the vomer(?). Contact between both premaxillae occurs along the anterior elongate processes. Elongate processes are absent in Eremotherium, but characterizes Megatherium species (De Iulis, Citation1994; Pujos, Citation2006). Premaxillae are poorly known in pre-Pleistocene South American megatheriines (see Saint-André & De Iuliis, Citation2001). This is the largest mammal from Espinar, with an estimated body mass of 1837.5 kg.
MYLODONTIDAE Gill, Citation1872
MYLODONTINAE Gill, Citation1872
SIMOMYLODON Saint André, Pujos, Cartelle, De Iuliis, Gaudin, McDonald, and Mamani Quispe, Citation2010
Temporal Range––Late Miocene and Pliocene.
Geographic Distribution––Bolivia (Oruro, Potosí, La Paz Provinces), Argentina (Jujuy), and Peru (Cusco Department).
SIMOMYLODON UCCASAMAMENSIS Saint André, Pujos, Cartelle, De Iuliis, Gaudin, McDonald, and Mamani Quispe, Citation2010
(, )
Referred Specimens––MUESP 16, Partial skull, left mandible, and ulna; MUESP 11, juvenile individual, partial skull, fragmentary jaw, cervical vertebrae.
Geographic and Stratigraphic Provenance––MUESP 16, city of Yauri (no detailed data); MUESP 11, Barrio San Lucas, Comunidad Huisaccollana, city of Yauri, Espinar; Locality ESP9-1: 14°47.925′S, 71°24.50′W (locality 4 in ). Collected in April 2009. Lower levels of Member C of El Descanso Formation, Early Pliocene (4.75 ± 0.5 Ma).
Comments––Robust cranium with a high and convex skull roof and a lower, short, massive rostrum (). In front of the zygomatic process of the maxillae, the rostrum expands anteriorly. Consequently, the rostrum bears a wide V-shaped palate with straight, divergent tooth rows, as in other mylodontines. The upper and lower dental series show no diastema between the two most anterior teeth (Cf1/cf1 and Mf1/mf1). Upper tooth rows are straight in Espinar specimens, and not bowed medially as in Glossotherium chapadmalense (MMP 245-M; Boscaini et al., Citation2022). As is typical in Simomylodon, the premaxillae are short, either forming a rounded (; MUESP 16) or wide V-shaped (MUESP 11) anterior margin. The horizontal ramus of the mandible is trapezoid along the tooth row (cf1, mf1–mf3), with the lowest height at cf1 and deepening posteriorly. The symphyseal spout raises and expands anteriorly. The lower tooth rows are oblique to the horizontal ramus in occlusal view.
The abundant cranial material of Simomylodon from Bolivia has shown enormous intraspecific variation, particularly in the relative size, robustness, and snout morphology, attributed in part to sexual dimorphism (Boscaini et al., Citation2018, Citation2019). The adult individual MUESP 16 from Espinar would correspond to the robust (i.e., male) morphotype, characterized by having large size and a wide snout with a rounded distal margin. The morphology of MUESP 11, a juvenile individual, lacks part of the premaxillae, precluding any confident morphotype determination. As identified, the gracile (i.e., female) and robust (i.e., male) morphotypes are not only based in relative robustness, but also in a distinct morphology of the premaxillae, a condition not strictly linked to body size or adultness. If skeletal differences between genders result from sexual selection in early stages of ontogeny as occur in some slow-growing mammals (Leigh, Citation1995), then an early ontogenetic differentiation within Simomylodon would reinforce disparities in niche occupation between morphotypes (Boscaini et al., Citation2019). Based on several bones of a specimen from Bolivia (MNHN-Bol-V-003375), the mean body mass of Simomylodon is estimated at 348.5 kg.
SCELIDOTHERIINAE Ameghino, Citation1904
PROSCELIDODON Bordas, Citation1935
Type Species––Proscelidodon patrius (Ameghino, Citation1888)
Temporal Range––Late Miocene to Late Pliocene?
Geographic Distribution––Argentina (Provinces of Mendoza, Catamarca, Buenos Aires, La Rioja, and Jujuy), Bolivia (Inchasi, Chuquisaca Department), and Peru (Espinar, Cusco Department).
PROSCELIDODON sp.
()
Referred Specimens––MUESP 20, sub-complete skeleton, including skull and jaws in anatomical connection.
Geographic and Stratigraphic Provenance––Calle Yauri, Lote 06 Mz C2 of the PP JJ Andrés A. Cáceres, Yauri, Espinar; 14°47.000′S, 71°24.881′W (locality 6 in ); discovered on March 8, 2017, by local citizen, Oblitas Choqie Ccaña. Lower levels of the Member C of El Descanso Formation, Early Pliocene (4.75 ± 0.5 Ma).
Comments––Bones of this skeleton are in anatomical connection, including the skull and jaws (). This identification must be taken with caution because key anatomical structures (dentition, palatal surface, basicranium, dorsal shape of the skull, etc.) are still inaccessible (). MUESP 20 () is an adult individual of roughly same size of Proscelidodon patrius (holotype: MACN 8075). Proportions of the skull in the Espinar scelidotheriine closely correspond to those of the holotype of Pr. patrius. Comparisons with Pr. gracillimus are limited because the holotype (MACN 8470) is badly damaged. MNHN-Bol-V-003353 from the Pliocene of Inchasi (Bolivia) referred to Pr. patrius (Anaya & MacFadden, Citation1995) is more gracile than MUESP 20, and could correspond to a distinct genus (FP, pers. observ.). The convex skull roof in MUESP 20 in lateral view is a remarkable feature, since most specimens referred to Proscelidodon show a flat to sinuous dorsal profile in this region. The body mass is estimated at 316.0 kg.
NOTOUNGULATA Roth, Citation1903
TOXODONTIDAE Owen, Citation1845
POSNANSKYTHERIUM Liendo Lazarte, Citation1943
Temporal Range––Pliocene.
Geographic Distribution––Bolivia (Inchasi, Chuquisaca Department) and Peru (Espinar, Cusco Department).
POSNANSKYTHERIUM VISCACHANENSE Saint-André, Citation1999
(, )
Referred Specimens––MUESP 3, adult individual, partial skeleton with skull and jaws; MUESP 8, adult individual, partial maxilla including (M1–M3) and left ear region, left partial mandible with m1–m3, hyoid bones; MUESP 9, young individual, partial skull damaged and distorted; MUESP 15, adult individual, rostrum and palatal region with most cheek teeth, but broken at the alveolar series level; MUESP 17, young individual, partial jaws.
Geographic and Stratigraphic Provenance––MUESP 3, soccer stadium of Yauri, city of Yauri, Espinar; ESP4: 14°46.844′S, 71°24.841′W (locality 5 in ); discovered by Adolfo Champú on March 7, 2005. MUESP 8 and MUESP 9, Villa Versalles, city of Yauri, Espinar; ESP6: 14°47.087′S, 71°24.963′W. Lower levels of Member C of El Descanso Formation, Early Pliocene (4.75 ± 0.5 Ma). MUESP 15 and MUESP 17, no precise data, city of Yauri, Espinar.
Comments––MUESP 3 is a relatively small individual almost indistinguishable from the holotype of Posnanskytherium viscachanense (MNHN VIZ 38), the latter collected at the Pliocene locality of Viscachani, south to La Paz. As typical in Posnanskytherium, in lateral view the skull roof is tall and flat (), whereas the rostrum proportions vary among species. Posnanskytherium desaguaderoi (MHNH-Bol-V GB-004) from Bolivia bears a tall and relatively long rostrum. In Po. inchasense, the rostrum is comparatively short and robust. In MUESP 3 and MNHN VIZ 38, the rostrum is short as well, but not as massive and clearly lower than in the aforementioned species. The snout is particularly low at the narial opening because the dorsal profile of the rostrum uniformly descends anteriorly from the level of the postorbital processes. In dorsal view, the zygomatic arches are widely rounded in MUESP 3, not as the more paraxial sides of the zygomatics of Po. desaguaderoi. In ventral view, the proportions of the premaxillae and maxillae seem to be similar in all recognized species, but the dental formulae of MUESP 3 differ by the presence of a small upper canine, thus the dentition of this specimen is I2/3, C1/1, P4/3, M3/3 (, ). Instead, MUESP 15 bears no upper canine. Upper molars are very slender and have a distinctly elongated paracone. Lower molars of Espinar specimens (MUESP 3 and MUESP 8) bear a single lingual (meta-entoconid) fold as Po. viscachanense from Ayo Ayo (MNHN-AYO 191) and MNHN-Bol-V 003360 from Inchasi, the latter referred to Po. desaguaderoi (Anaya & MacFadden, Citation1995). This fold is virtually imperceptible in Po. inchasense (holotype, MNHN-Bol-V 350). Espinar specimens also differ from Po. inchasense in having a shallower horizontal ramus. The body mass is estimated at 157.5 kg.
DISCUSSION AND CONCLUSIONS
Mammals from Espinar: A Glimpse of the Pliocene Andean Faunas
The central Andean plateau fauna from Espinar is remarkable by the amazing preservation of their fossil mammals, largely consisting of skeletons in anatomical connection and with no geometric deformation. As fossiliferous deposits are located within the urban area of the city of Yauri, searching and collecting procedures are limited. To date, we have identified five taxa of herbivorous mammals within two conspicuous native South American clades, Xenarthra and Notoungulata. Xenarthrans constitute the dominant component of the fauna, including a new glyptodontine, Andinoglyptodon mollohuancai gen. et sp. nov., and three taxa of ground sloths, the Megatheriidae cf. Megatheriops rectidens (Megatheriinae) and the Mylodontidae Simomylodon uccasamamensis (Mylodontinae) and Proscelidodon sp. (Scelidotheriinae). Notoungulates are represented by the Toxodontidae Posnanskytherium viscachanense. Small mammals and predators are currently unknown from the Pliocene of Espinar. Albeit rare, these faunal components are reported from coeval Andean localities that have been more extensively sampled (Anaya & MacFadden, Citation1995; Marshall & Sempere, Citation1991).
The vertebrate-bearing deposits of Espinar are dated at ∼4.8 Ma (Early Pliocene; Kar et al., Citation2016 and this contribution) and document taxa previously recovered from Late Miocene and Pliocene Andean localities in Bolivia and Argentina (). Among them, Simomylodon and Posnanskytherium were previously known only from the Bolivian-northernmost Argentina plateau and discovered in almost every locality encompassing the Pliocene interval (e.g., Pomata-Ayte, earliest Pliocene: Marshall et al., Citation1983; Inchasi late Early Pliocene [4.0–3.3 Ma]: Anaya & MacFadden, Citation1995; and Ayo Ayo-Viscachani, Late Pliocene [∼2.9 Ma]: Marshall and Sempere, Citation1991; Casira, Late Miocene–Pliocene: Quiñones et al., Citation2019). The distribution of these animals is now northward into Peru, but still within the same Andean plateau domain. Proscelidodon attained a larger geographic distribution, including both Andean and non-Andean areas, but the species within the genus are poorly diagnosed (Anaya & MacFadden, Citation1995; Miño-Boilini et al., Citation2011). This is a crucial taxon because it might have been the sole scelidotheriine from the Late Miocene–Pliocene time frame (McDonald, Citation1987; Miño-Boilini et al., Citation2011; Pujos et al., Citation2012). The finely preserved skeleton from Espinar will be key to improve the characterization of Proscelidodon species and to provide a better understanding of the evolution of later relatives (i.e., Catonyx, Valgipes, and Scelidotherium).
FIGURE 7. Map showing selected Upper Miocene–Pliocene localities (stars) in Peru, Bolivia, and Argentina, where the mammalian genera discovered in Espinar (silhouettes) have been documented. Peru: 1, Espinar. Bolivia: 2, La Paz; 3, Ayo-Ayo; 4, Choquecota; 5, Pomata-Ayte; 6, Inchasi; 7, Casira. Argentina: 8, Casira; 9, Maimará; 10, Uquia; 11, Huayquerías; 12, Mar del Plata. Map source: U.S. Geological Survey's Center for Earth Resources Observation and Science (EROS).
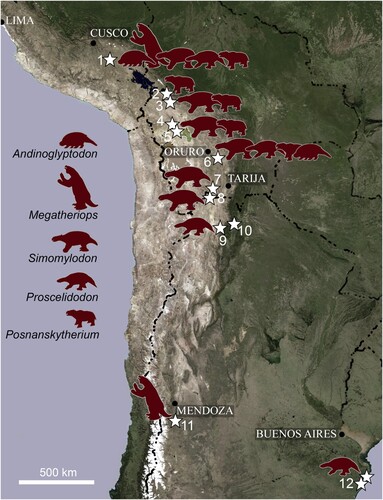
The Peruvian fossil referred here as a putative subadult individual of Megatheriops rectidens is the only record besides the holotype (MACN 2818) from the Huayquerías of Mendoza in Argentina, a rich Andean vertebrate locality regarded as Late Miocene (∼5.8 Ma: Garrido et al., Citation2017; ). Differences between the Peruvian and the Argentinian specimens are subtle. The Peruvian specimen might possess a wider palate between molariforms if the holotype of M. rectidens was not compressed by taphonomic processes. The preserved premaxillae reveal that the general configuration of those bones in Megatherium was already present in Megatheriops, including an elongate anterior process, a strong lateral process, and a nubbin-like medial process. Indeed, the premaxillae of the so-called Andean species of Megatherium (see De Iuliis et al., Citation2009), such as Megatherium tarijense and Megatherium celendinense, are essentially robust versions of those observed in the Peruvian Megatheriops. Since the premaxillary shape is suggested to be intimately linked to feeding habits (see below), changes in the morphology of the premaxillae within Andean megatheriines might reflect a shift in ecological niches for these animals in the high Andes from the Pliocene to the Pleistocene, yet a phylogenetic context is necessary to trace this evolution.
Andinoglyptodon and the Pliocene Glyptodontine Conundrum
The recognition of Andinoglyptodon as a glyptodontine stems basically on characters of the dorsal carapace, such as the presence of osteoderms with a single row of peripheral figures and 12 or less peripheral figures per osteoderm (Fernicola & Porpino, Citation2012; Zurita et al., Citation2013). Andinoglyptodon also bears a U-shaped osteoderm sulci as in Boreostemma, Glyptodontidium, Paraglyptodon, Glyptodon, and Glyptotherium. This feature might diagnose the glyptodontines (Carlini et al., Citation2008) but its distribution among tropical glyptodonts is poorly known (see Tejada-Lara et al., Citation2015). The rosette pattern of the osteoderms is characteristic of glyptodontines but is considered plesiomorphic by its presence in the archaic Propalaehoplophorinae (Fernicola & Porpino, Citation2012). We identified two cranio-dental characters that might diagnose glyptodontines and are observed in Andinoglyptodon: trilobate upper molariforms (incipient in Mf1; also present in Panochthus) and occipital condyles lateromedially expanded (see Carlini et al., Citation2008; Fernicola, Citation2008).
Within glyptodontines, Andinoglyptodon possesses significant differences in the skull and jaws relative to Pliocene and Pleistocene forms known so far by cranial material. Pleistocene taxa, such as Glyptodon and Glyptotherium, are considerably bigger than Andinoglyptodon and exhibit a deep, massive, and more quadrangular rostrum (). This feature is emphasized in these genera by the presence of a truncated snout with a high narial opening. In contrast, in Andinoglyptodon the narial opening is wider-than-tall and the rostrum is lower, relatively long, and slender at the anterior end. The symphyseal spout of the mandible is semicircular and narrow. An elongated rostrum is also recognized in Boreostemma acostae from the Middle Miocene of the Villavieja Formation, Colombia (Zurita et al., Citation2013).
The Pliocene genus Paraglyptodon is problematic because its species (P. chapalmalensis and P. uquiensis after Oliva et al., Citation2010) were diagnosed on scattered remains of the dermal armor (Castellanos, Citation1953; Rovereto, Citation1914). The type series of Paraglyptodon uquiensis also includes a portion of a skull (MACN-Pv 5377) that preserves the rostrum, zygomatics, and partial dentition (). Regarding P. chapalmalensis, well-preserved cranial material (MMP 4676) recovered from the type locality was recently conferred to this species (Oliva et al., Citation2010; ). In a review of Paraglyptodon, Cruz and colleagues (2016) concluded that MACN-Pv 5377 cannot confidently be assigned to P. uquiensis because it was not found associated with the type osteoderms and shows no differences with Pleistocene glyptodontines. In the same work, the osteoderm type materials of P. chapalmalensis (MAVN-Pv 6162) and P. uquiensis were attributed to juvenile individuals of Pleistocene Glyptodon that might have rolled from upper levels, an asseveration supported by other authors only for P. chapalmalensis (Zurita et al., Citation2016). The present study revealed that both skulls (P. chapalmalensis: MACN-Pv 5377 and P. uquiensis: MMP 4676) are of comparable size and much larger (MACN-PV 5377, BZYW = ∼229 mm) than the skull of Andinoglyptodon (BZYW = ∼155 mm; ). Most cranial features of Glyptodon species (which are absent in the Peruvian taxon) are recognized in these Argentinian fossils, such as the typical square, truncated, and high snout. If these skulls (i.e., MACN-Pv 5377 and MMP 4676) are from the Late Pliocene, then Glyptodon species existed in that time frame. In contrast, lateral and anterior osteoderms of Andinoglyptodon are almost identical to those of Paraglyptodon and Glyptodon. It is true that osteoderm material of P. chapalmalensis bears features observed in juvenile stages of Glyptodon (see Zurita et al., Citation2016), but these features are also present in certain osteoderms of Andinoglyptodon (), suggesting large intraspecific variation among glyptodontine taxa.
Comparisons between Andinoglyptodon and the Late Miocene species Glyptodontidium tuberifer show noticeable differences in the osteoderms of the posterodorsal region of the carapace. Posterodorsal osteoderms of Glyptodontidium bear a rosette pattern in which peripheral figures are comparatively larger than those of Andinoglyptodon. The central figure is convex in Glyptodontidium whereas it is concave in Andinoglyptodon. If present, conical bosses of the caudal notch osteoderms were poorly developed in Andinoglyptodon, in contrast to the prominent conical bosses of Glyptodontidium.
Among the rich Bolivian and Argentinian record of Neogene terrestrial mammals, we consider that only one is attributable to Andinoglyptodon. The material consists of a portion of a dorsal carapace and caudal elements from the locality of Inchasi and originally referred to Paraglyptodon (MNHN-Bol-V 003366; Anaya & MacFadden, Citation1995:fig. 4). Dorsal carapace osteoderms in this specimen are almost undistinguishable from the anterolateral osteoderms of Andinoglyptodon regarding the rosette pattern, surface texture, shape, size, and relative thickness (). Because these features are of only limited value for glyptodontine taxonomy, Andinoglyptodon-like osteoderms from Inchasi associated to cranial remains would be necessary for a conclusive identification.
FIGURE 8. Anterolateral carapace osteoderms: A, Andinoglyptodon mollohuancai holotype MUESP 4; B, cf. Andinoglyptodon (MNHN-Bol-V 006369) from Inchasi. Scale bar equals 5 cm.
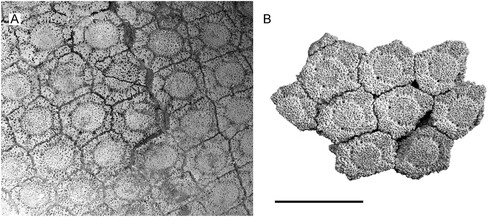
Andinoglyptodon clearly differs on cranial grounds from Pliocene and Pleistocene relatives, but these are almost undistinguishable on the basis of the dermal armor, indicating that osteoderm evidence alone is insufficient to draw definitive taxonomic conclusions. Osteoderm differences between Pliocene and Pleistocene glyptodontines are subtle and generally range within the wide ontogenetic and individual variation shown by putative species.
Pliocene Environmental Conditions in the Central Andean Plateau: A Mammalian Perspective
At first glance, the Espinar mammal assemblage could be associated with grassland environments because it is composed by herbivorous forms with ever-growing (hypselodont) dentition (Damuth & Janis, Citation2011; Janis et al., Citation2000; Jardine et al., Citation2012). However, a closer look to the premaxillae and maxillae anatomy reveals a variety of morphologies that can provide a more complex environmental interpretation (, ; ). The shape of the premaxillae and maxillae reflects the shape of the muzzle. In herbivorous mammals, particularly in ungulates, the muzzle width has been linked to feeding strategies and diet, and by extension to plant resources (Janis & Ehrhardt, Citation1988; Janis et al., Citation2000). The predominance of xenarthrans in the Espinar fauna might be seen as a drawback for environmental inferences because their peculiar feeding anatomy lacks ecological analogs in modern ecosystems (Anaya & MacFadden, Citation1995; Bargo et al., Citation2006). For example, nitrogen isotopic analyses of amino acids on the Pleistocene ground sloth Mylodon darwini revealed that this species fed on items of animal origin in addition to plants (Tejada et al., Citation2021), a feeding behavior that would have been hard, if not impossible to infer, solely from morphology. In lack of geochemical data, however, muzzle reconstructions from craniodental morphology provide a widely used proxy to infer dietary preferences within the herbivorous guild of xenarthrans (Bargo et al., Citation2006).
Within the Espinar fauna, relatively wide muzzles are inferred for the toxodontid Posnanskytherium and the mylodontine Simomylodon based on their square-shaped and wide premaxillae (Boscaini et al., Citation2019). Posnanskytherium and Simomylodon would have exploited a wide range of resources close to the ground (e.g., grasses and small plants) because mammals with wide muzzles are generally reconstructed as grazers and bulk feeders (e.g., Toxodon [MacFadden & Shockey, Citation1997] and Glossotherium [Bargo et al., Citation2006]). Additionally, isotopic analyses indicate that Posnanskytherium from Inchasi fed on C3 plants in its diet, including woody plants and montane grasses (MacFadden et al., Citation1994). Robust and gracile morphs of Simomylodon might have exploited a wide range of resources even beyond the grazer guild (Boscaini et al., Citation2019).
Proscelidodon with a slender and long snout, and Megatheriops bearing slender premaxillae, were narrow-muzzled ground sloths with prehensile lips after the reconstruction criteria of Bargo and colleagues (2006) for Scelidotherium and Megatherium. This kind of anatomy is associated with selective feeders and browsers. Browsers make use of their prehensile lips to actively select parts of trees and shrubs, such as leaves and young shoots. Andinoglyptodon with its narrow and rounded premaxillae might have been a mixed or selective feeder. The RMW value obtained for Andinoglyptodon (0.742) places this taxon within the group proposed for putative selective feeders, which includes Early Miocene taxa and Glyptodon species (i.e., values above 0.6: Vizcaíno et al., Citation2011b). Thus, the Espinar assemblage depicts the existence of both selective and bulk feeders, as should be expected in a mixed, wooded grassland ecosystem (). We expect a comparable signal from Pliocene faunas described for the Bolivian Andes (Anaya & MacFadden, Citation1995; Marshall & Sempere, Citation1991).
FIGURE 9. Life reconstruction of the Early Pliocene mammalian fauna from the Andean plateau of Espinar. From left to right: Andinoglyptodon mollohuancai, Simomylodon uccasamamensis, Posnanskytherium viscachanense, Proscelidodon sp., and cf. Megatheriops rectidens. Art: Jorge A. González.
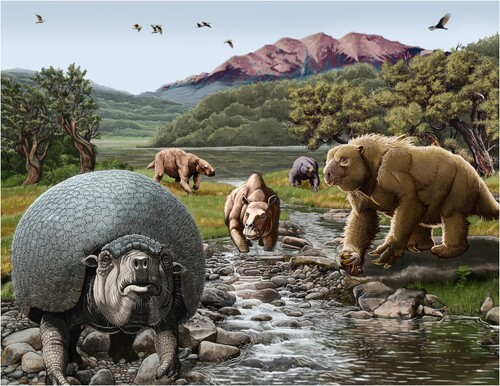
Our preliminary body mass assessment indicates that most of the Pliocene mammals from Espinar largely surpassed 100 kg, with ground sloths ranging between about 316 kg (Proscelidodon sp.) and 1838kg (sub-adult cf. Megatheriops). Studies based on fossil leaves and pollen imply that these large herbivores inhabited the oldest known Puna ecosystem within the Central Andean plateau around 4.8 Ma, when paleoelevations might have reached near-modern values (∼3800 m a.s.l.; Kar et al., Citation2016; Martínez et al., Citation2020). Vegetation in this ecosystem was dominated by Poaceae (grasses) and Cyatheaceae (scaly tree ferns) and included taxa of shrubs and trees (such as Polylepis, Quercus, Myrsine, cf. Schinus, Podocarpus, Berberis, and Alnus) living either in the modern Puna or close to the treeline (Martínez et al., Citation2020).
The main difference between the Puna ecosystem in the Pliocene and in the present relates to a higher diversity and abundance of ferns and shrubs in the former, which is linked to slightly wetter conditions in the Pliocene (Martínez et al., Citation2020). Would the selective feeders documented in Espinar have fed upon ferns? Ferns are not the favorite food of herbivorous mammals in the present but there are reports of selective feeders (i.e., deer) that include ferns in their diet (Haeussler et al., Citation1990). Polylepis was also identified within the Pliocene Puna ecosystem and would have represented an important resource for selective feeders, such as Megatheriops and Proscelidodon since this tree constitutes relatively dense forests in the high Andes (Hansen et al., Citation1994). A recent discovery of a pristine Andean landscape in Cusco dominated by Polylepis suggests that prior to human occupation, tree-dominated forest-grassland mosaics prevailed (Sylvester et al., Citation2017).
An overall similar Puna ecosystem to the current one supported a community of at least five megamammals of different ecomorphotypes in the Early Pliocene of the Andean Plateau. Giant mammals, natives and immigrants, ruled and persisted throughout the Pleistocene in this ecoregion (Marshall et al., Citation1984; Lucas, Citation2008), suggesting that the low extant diversity of mammalian herbivores in the Andean plateau might be a consequence of the Pleistocene–Holocene extinction, rather than attributable solely to the modern-type of floral composition.
The Espinar mammal assemblage is the first known so far from the Pliocene of the Peruvian Andes. This initial study only includes the fauna living at about modern elevations from deposits of Member C, within the city of Yauri. However, fossils of vertebrates and plants with exquisite anatomical details were reported from earlier deposits in the region (Member B; Martínez et al., Citation2020; Tejada, Baez et al., Citation2009; Tejada, Gamarra et al., Citation2009). This pre-Pliocene record documents life during the Middle Miocene–Late Miocene interval, when the elevation of the plateau was only ∼1700m a.s.l. (Martínez et al., Citation2020). Future studies focusing on these older localities in Espinar will contribute to understanding the long-term evolution of South American native mammals and Andean ecosystems.
AUTHOR CONTRIBUTIONS
RS-G and JVT: conceptualization, data collection and curation, anatomical description, writing—original draft, formal analysis, funding acquisition, writing—review and editing; DO: data collection, formal analysis, writing—review and editing; JG: data collection and curation, writing—review and editing; DAF and FP: formal analysis, writing—review and editing. All authors edited, reviewed, and gave final approval for publication.
LIST OF SUPPLEMENTARY FILES
Supplementary Data 1: Supplementary figures, tables, and appendices.
Supplemental Material
Download MS Word (13.8 MB)ACKNOWLEDGMENTS
We dedicate this research to the inhabitants of the Espinar province and the K’ana nation for their great commitment to the recovery and protection of the paleontological legacy of the area. Special thanks to our friends G. Agramonte, J. Apaza, W. Inca, G. Umasi, H. Huaranca, V. Chacra Sayo from Cusco and Espinar, for help, support, and camaraderie during expeditions. We are indebted to M. Reguero (MLP), A. Kramarz (MACN), B. Mamani (MNHN-Bol), G. Billet, and C. de Muizon (MNHN) for kindly providing access to the collections under their care. We also thank N. Valencia, G. Jara-Almonte, D. Baez, G. Vilchez, C. Jaramillo, C. Martínez, K. Quispe, L. Cerpa, E. Díaz, W. Aguirre, E. Rojas, C. Cumpa, T. Mercado, the IFEA staff, and the Departamento Físico-Químico de la Dirección Desconcentrada de Cultura de Cusco for fossil preparation, field, and/or administrative support. Special thanks to P. A. Carrasco for assistance in processing images and data. Our appreciation to the former mayor of Espinar, O. Mollohuanca, who supported our field and lab work in 2011. We also thank both anonymous reviewers and editors J. Meachen and B. Anemone, whose comments and suggestions greatly improved our manuscript. We acknowledge support from CONCYTEC, Peru: grant no. 034-2019-02-FONDECYT-BM-INC.INV and Basic Science grant no. 104-2018-FONDECYT to RS-G. JVT acknowledges support by the Institut Français d’Études Andines (Beca Andina), and to G. Lomné in particular, for financial and logistic support during early stages of this study. Start-funds provided by Caltech to JVT financially helped some aspects of this contribution.
LITERATURE CITED
- Acebes, P., Wheeler, J., Baldo, J., Tuppia, P., Lichtenstein, G., Hoces, D., & Franklin, W. L. (2018). Vicugna vicugna. The IUCN red list of threatened species 2018: e.T22956A18540534.
- Ameghino C., & Kraglievich, L. (1921). Descripción del “Megatherium gallardoi” C. Amegh. descubierto en el Pampeano inferior de la ciudad de Buenos Aires. Anales del Museo Nacional de Historia Natural de Buenos Aires, 31, 134–156.
- Ameghino, F. (1888). Rápidas diagnosis de algunos mamíferos fósiles nuevos de la República Argentina. Coni P.E., Buenos Aires, 17 p.
- Ameghino, F. (1904). Nuevas especies de mamíferos cretáceos y terciarios de la República Argentina. Anales de la Sociedad Científica Argentina, 58, 225–291.
- Anaya, F., & MacFadden, B. J. (1995). Pliocene Mammals from Inchasi, Bolivia: The Endemic Fauna Just Before The Great American Interchange. Bulletin of the Florida Museum of Natural History, 39(3), 87–140.
- Baied, C. A., & Wheeler J. C. (1993). Evolution of High Andean Puna Ecosystems: Environment, Climate, and Culture Change over the Last 12,000 Years in the Central Andes. Mountain Research and Development, 13(2), 145.
- Bargo, M. S., Toledo, N., & Vizcaíno, S. F. (2006). Muzzle of South American Pleistocene Ground Sloths (Xenarthra, Tardigrada). Journal of Morphology, 267(2), 248–263.
- Bordas, A. F. (1935). Observaciones sobre los géneros “Scelidodon” Ameghino y “Proscelidodon” n. g. Physis, 11, 484–491.
- Boscaini, A., Fernández-Monescillo, M., Pérez, M. E., Mamani Quispe, B., Andrade Flores, R., Gaudin, T. J., Marivaux, L., Antonie, P. O., Münch, P., & Pujos, F. (2021). El yacimiento de Ayo Ayo-Viscachani (Plioceno-Pleistoceno, Departamento de La Paz, Bolivia): 50 años desde su descubrimiento. XII Congreso de La Asociación Paleontológica Argentina.
- Boscaini, A., Gaudin, T. J., Mamani Quispe, B., Münch, P., Antoine, P. O., & Pujos, F. (2018). New well-preserved craniodental remains of Simomylodon uccasamamensis (Xenarthra: Mylodontidae) from the Pliocene of the Bolivian Altiplano: Phylogenetic, chronostratigraphic and palaeobiogeographical implications. Zoological Journal of the Linnean Society, 185(2), 459–486.
- Boscaini, A., Gaudin, T. J., Toledo, N., Mamani Quispe, B., Antoine, P. O., & Pujos, F. (2019). The earliest well-documented occurrence of sexual dimorphism in extinct sloths: evolutionary and palaeoecological insights. Zoological Journal of the Linnean Society, 187(1), 229–239.
- Boscaini, A., Toledo, N., Mamani Quispe, B., Andrade Flores, R., Fernández-Monescillo, M., Marivaux, L., Antoine, P. O., Münch, P., Gaudin, T. J., & Pujos, F. (2021). Postcranial anatomy of the extinct terrestrial sloth Simomylodon uccasamamensis (Xenarthra, Mylodontidae) from the Pliocene of the Bolivian Altiplano, and its evolutionary implications. Papers in Palaeontology, 7(3), 1557–1583.
- Boscaini, A., Toledo, N., Pérez, L. M., Taglioretti, M. L., & McAfee, R. K. (2022). New well-preserved materials of Glossotherium chapadmalense (Xenarthra, Mylodontidae) from the Pliocene of Argentina shed light on the origin and evolution of the genus. Journal of Vertebrate Paleontology, 42(1), 1–16.
- Cabrera, Á. (1928). Sobre algunos megaterios pliocenos. Revista del Museo de La Plata, 31, 339–352.
- Cabrera, Á. (1944). Los Gliptodontoideos del Araucaniano de Catamarca. Revista del Museo de La Plata, 3, 5–76.
- Carlini, A. A., Brandoni D., Scillato-Yané G. J., & Pujos F. (2002). Una nueva especie de megaterio del Mio-Plioceno de Catamarca, Argentina. Ameghiniana, 39, 367–377.
- Carlini, A. A., Zurita, A. E., Scillato-Yané, G. J., Sánchez, R., & Aguilera, O. A. (2008). New Glyptodont from the Codore Formation (Pliocene), Falcón State, Venezuela, its relationship with the Asterostemma problem, and the paleobiogeography of the Glyptodontinae. Paläontologische Zeitschrift, 82(2), 139–152.
- Carlotto, V. (2013). Paleogeography and tectonic controls on the evolution of Cenozoic basins in the Altiplano and Western Cordillera of southern Peru. Tectonophysics, 589, 195–219.
- Castellanos, A. (1953). Descripción de restos de Paraglyptodon uquiensis n. sp. de Uquía (Senador Pérez). Jujuy. Memorias del Museo de Entre Ríos 32:1e32.
- Cerpa, L., Carlotto, V., Sempere, T., & Meza, P. (2002). Geodynamic Evolution of the Miocene Descanso-Yauri basin, Cusco Region, Peru. International Symposium on Andean Geodynamics, 5, 133–136.
- Cione, A. & Tonni, E. P. (1996). Reassesment of the Pliocene-Pleistocene continental time scale of Southern South America. Correlation of the type Chapadmalalan with Bolivian sections. Journal of Sourh American Earth Sciences, 9, 221-236.
- Cope, E. D. (1889). The Edentata of North America. American Naturalist, 23, 657–664.
- Coutier, F., Hautier, L., Cornette, R., Amson, E., & Billet, G. (2017). Orientation of the lateral semicircular canal in Xenarthra and its links with head posture and phylogeny. Journal of Morphology, 278(5), 704–717.
- Croft, D. A., Flynn, J. J., & Wyss, A. R. (2007). A new basal glyptodontid and other xenarthra of the early Miocene Chucal Fauna, northern Chile. Journal of Vertebrate Paleontology, 27(4), 781–797.
- Cruz, L. E., Fernicola, J. C., Taglioretti, M., & Toledo, N. (2016). A reassessment of the taxonomic status of Paraglyptodon Castellanos, 1932 (Mammalia, Cingulata, Glyptodontia). Journal of South American Earth Sciences, 66, 32–40.
- Cuadrelli, F., Zurita, A. E., Toriño, P., Miño-Boilini, A. R., Rodríguez-Bualó, S., Perea, D. & Acuña Suarez, G. E. (2018). Late Pleistocene Glyptodontinae (Mammalia, Xenarthra, Glyptodontidae) from southern South America: a comprehensive review. Journal of Vertebrate Paleontology, 38(5), DOI: 10.1080/02724634.2018.1525390
- Damuth, J. (1990). Problems in estimating body masses of archaic ungulates using dental measurements. In J. Damuth & B. J. MacFadden (Eds.), Body Size in Mammalian Paleobiology: Estimation and Biological Implications (pp. 229-253). Cambridge University Press, Cambridge, United Kingdom.
- Damuth, J., & Janis, C. M. (2011). On the relationship between hypsodonty and feeding ecology in ungulate mammals, and its utility in palaeoecology. Biological Reviews, 86(3), 733–758.
- De Iuliis, G. (1994). Relationships of the Megatheriinae, Nothrotheriinae, and Planopsinae: some skeletal characteristics and their importance for phylogeny. Journal of Vertebrate Paleontology, 14, 577–591.
- De Iuliis, G. (1996). A systematic review of the Megatheriinae (Mammalia: Xenarthra: Megatheriidae). [Ph.D. dissertation]. University of Toronto, Canada, 719 pp.
- De Iuliis, G., Pujos, F., & Tito, G. (2009). Systematic and Taxonomic Revision of the Pleistocene Ground Sloth Megatherium (Pseudomegatherium) tarijense (Xenarthra: Megatheriidae). Journal of Vertebrate Paleontology, 29(4), 1244–1251.
- De Iuliis, G., Ré, G. H., & Vizcaíno, S. F. (2004). The Toro Negro megatheriine (Mammalia, Xenarthra): A new species of Pyramiodontherium and a review of Plesiomegatherium. Journal of Vertebrate Paleontology, 24(1), 214–227.
- Fariña, R. (1995). Limb bone stength and habits in large glyptodonts. Lethaia, 28, 189–196.
- Fernicola, J. C. (2008). Nuevos aportes para la sistemática de los Glyptodontia Ameghino 1889 (Mammalia, Xenarthra, Cingulata). Ameghiniana, 45, 553–574.
- Fernicola, J. C., & Porpino, K. O. (2012). Exoskeleton and Systematics: A Historical Problem in the Classification of Glyptodonts. Journal of Mammalian Evolution, 19(3), 171–183.
- Flower, W. H. (1883). On the arrangement of the orders and families of existing Mammalia. Proceedings of the Zoological Society of London, 178–186.
- Garrido, A. C., Bonini, R. A., & Barbeau, D. L. (2017). Paleoambiente, edad y vertebrados de la Formación Huayquerías (Mioceno tardío), provincia de Mendoza, República Argentina. XX Congreso Geológico Argentino, 1, 50–55.
- Gill, T. (1872). Arrangement of the families of mammals with analytical tables. Smithsonian Miscellaneous Collections, 11:vi+1–98.
- Gillette, D. D., & Ray, C. E. (1981). Glyptodonts of North America. Smithsonian Contributions to Paleobiology, 40, 1–255.
- Gray, J. E. (1869). Catalogue of carnivorous, pachydermatous, and edentate Mammalia in the British Museum. British Museum (Natural History) Publications, London, 398 pp.
- Haeussler, S., Coates, D., & Mather, J. (1990). Autecology of common plants in British Columbia: a literature review. FRDA Research Program, Research Branch, BC Ministry of Forests and Lands. British Columbia, Canada.
- Hansen, B. C. S., Seltzera, G. O., & Wright Jr., H. E. (1994). Late Quaternary vegetational change in the central Peruvian Andes. Palaeogeography, Palaeoclimatology, Palaeoecology, 109(2–4), 263–285.
- Illiger, K. (1811). Prodromus Systematis Mammalium et Avium Additis Terminis Zoographicis Utriusque Classis. C. Salfeld, Berlin, xviii, 301 pp.
- Janis, C. M., Damuth, J., & Theodor, J. M. (2000). Miocene ungulates and terrestrial primary productivity: Where have all the browsers gone? Proceedings of the National Academy of Sciences of the United States of America, 97(14), 7899–7904.
- Janis, C. M., & Ehrhardt D. (1988). Correlation of the muzzle width and relative incisor width with dietary preference in ungulates. Zoological Journal of the Linnean Society, 92, 267–284.
- Jardine, P. E., Janis, C. M., Sahney, S., & Benton, M. J. (2012). Grit not grass: Concordant patterns of early origin of hypsodonty in Great Plains ungulates and Glires. Palaeogeography, Palaeoclimatology, Palaeoecology, 365–366, 1–10.
- Kar, N., Garzione, C. N., Jaramillo, C., Shanahan, T., Carlotto, V., Pullen, A., Moreno, F., Anderson, V., Moreno, E., & Eiler, J. (2016). Rapid regional surface uplift of the northern Altiplano plateau revealed by multiproxy paleoclimate reconstruction. Earth and Planetary Science Letters, 447(1), 33–47.
- Leigh, S. R. (1995). Socioecology and the Ontogeny of Sexual Size Dimorphism in Anthropoid Primates. American Journal of Physical Anthropology, 97(4), 339–356.
- Liendo-Lazarte, M. (1943). Nota Preliminar Sobre un Pequeño Toxodonte del Altiplano: Posnanskytherium desaguaderoi: gen. nov. sp. nov. Museo Nacional de Tiahuanacu, Sección de Paleontología, La Paz, Bolivia, 13 p.
- Lucas, S. (2008). Cuvieronius (Mammalia, Proboscidea) from the Pleistocene of Ulloma, Bolivia. In S. G. Lucas, G. S. Morgan, J. A. Spielmann & D. R. Prothero (Eds.), Neogene Mammals (pp. 399–402). Nuevo Mexico Museum of Natural History.
- MacFadden, B. J., & Shockey, B. J. (1997). Ancient feeding ecology and niche differentiation of Pleistocene mammalian herbivores from Tarija, Bolivia: Morphological and isotopic evidence. Paleobiology, 23(1), 77–100.
- MacFadden, B. J., Wang, Y., Cerling, T. E., & Anaya, F. (1994). South American fossil mammals and carbon isotopes: a 25 million-year sequence from the Bolivian Andes. Palaeogeography, Palaeoclimatology, Palaeoecology, 107(3–4), 257–268.
- Marshall, L. G., Berta, A, Hoffstetter, R., Pascual, R., Reig, O. A., Bombin, M., & Mones, A. (1984). Mammals and stratigraphy: Geochronology of the continental mammal-bearing Quaternary of South America. Paleovertebrata, Mémoire Extraordinaire, 1–76.
- Marshall, L. G., Hoffstetter, R., & Pascual, R. (1983). Mammals and stratigraphy: Geochronology of the continental mammal-bearing Tertiary of South America. Paleovertebrata, Mémoire Extraordinaire, 1–93.
- Marshall, L. G., & Sempere, T. (1991). The Eocene to Pleistocene Vertebrates of Bolivia and Their Stratigraphic Context: A Review. In R. Suarez-Soruco (Ed.), Fósiles y Facies de Bolivia - Vol. I Vertebrados, 12(3–4), 631–652.
- Martínez, C., Jaramillo, C., Correa-Metrío, A., Crepet, W., Moreno, J. E., Aliaga, A., Moreno, F., Ibañez-Mejia, M., & Bush, M. B. (2020). Neogene precipitation, vegetation, and elevation history of the Central Andean Plateau. Science Advances, 6(35), 1–9.
- McDonald, H. G. (1987). A systematic review of the Plio-Pleistocene Scelidothere ground sloths (Mammalia: Xenarthra: Mylodontidae). [Unpublished Ph.D. dissertation]. University of Toronto, Toronto, 478 pp.
- Miño-Boilini, Á. R., De Los Reyes, M., Zurita, A. E., Arrouy, M. J., & Poiré, D. G. (2019). Pliocene Scelidotheriinae (Xenarthra, Tardigrada) from the Pampean region of Argentina: Morphology, chronology, and comments on the diversity of the subfamily. Comptes Rendus Palevol, 18(3), 325–334.
- Miño-Boilini, A. R., Tomassini, R. Oliva, C., & Manera de Bianco, T. (2011). Adiciones al conocimiento de Proscelidodon Bordas, 1935 (Mammalia, Xenarthra, Scelidotheriinae). Revista Brasileira de Paleontología, 14, 269–278.
- Moreno, F. P., & Mercerat A. (1891). Exploración arqueológica de la provincia de Catamarca. Paleontología. Revista del Museo de La Plata, 1, 222–236.
- Nasif, N. L., Esteban, G., Georgieff, S. M., Ibañez, L., & Spagnuolo, C. M. (2019). Biocronoestratigrafía y paleoambientes del Mioceno tardío de Catamarca, Tucumán y Santiago del Estero, Argentina. Ópera Lilloana 52: Mioceno Al Pleistoceno Del Centro y Norte de Argentina, 229–250.
- Oliva, C., Zurita, A. E., Dondas, A., & Scillato-Yané, G. J. (2010). Los Glyptodontinae (Xenarthra, Glyptodontidae) del Piso/Edad Chapadmalalense (Plioceno tardío): revisión y aportes a su conocimiento. Revista Mexicana de Ciencias Geológicas, 27(1), 112–120.
- Osborn, F. H. (1903). Glyptotherium texanum, a new glyptodont from the lower Pleistocene of Texas. Bulletin of the American Museum of Natural History, 19,491–494.
- Owen, R. (1838). Note of the Glyptodon; pp. 178b–178e in W. Parish (ed), Buenos Ayres and the Province of the Rio de la Plata, their present state, trade, and debt; with some account from original documents of the progress of geographical discovery in those parts of South America during the last sixty years J. Murray, London.
- Owen, R. (1843). Zoologycal summary of the extinct and living animals of the order Edentata. Edinburgh New Philosophical Journal of Sciences and Arts, 35, 353-360.
- Owen, R. (1845). Descriptive and illustrated catalogue of the fossil organic remains of Mammalia and Aves. Museum of the Royal College of Surgeons of London, London, 391 pp.
- Pujos, F. (2006). Megatherium celendinense sp. nov. from the Pleistocene of the Peruvian Andes and the Phylogenetic Relationships of Megatheriines. Paleontology, 49(2), 285–306.
- Pujos, F., Candela, A., Galli, C. I., Coira, B. L., Reguero, M. A., De Los Reyes, M., & Abello, M. A. (2012). The Scelidotheriine Proscelidodon (Xenarthra: Mylodontidae) from the Late Miocene of Maimará (Northwestern Argentina, Jujuy Province). Ameghiniana, 49(4), 668–674.
- Pujos, F., De Iuliis, G., Mamani Quispe, B., Adnet, S., Andrade Flores, R., Billet, G., Fernández-Monescillo, M., Marivaux, L., Münch, P., Prámparo, M. B., & Antoine, P. O. (2016). A new nothrotheriid xenarthran from the early Pliocene of Pomata-Ayte (Bolivia): new insights into the caniniform–molariform transition in sloths. Zoological Journal of the Linnean Society, 178(3), 679–712.
- Pujos, F., & Salas, R. (2004). A new species of Megatherium (Mammalia: Xenartha: Megatheriidae) from the Pleistocene of Sacaco and Tres Ventanas, Peru. Palaeontology, 47(3), 579–604.
- Quiñones, S. I., Miño-Boilini, Á. R., Zurita, A. E., Contreras, S. A., Luna, C. A., Candela, A. M., Camacho, M., Ercoli, M. D., Solís, N., & Brandoni, D. (2019). New records of Neogene Xenarthra (Mammalia) from eastern Puna (Argentina): Diversity and biochronology. Journal of Paleontology, 93(6), 1258–1275.
- Reguero, M. A. & Candela, A. M. (2008). Bioestratigrafía de las secuencias neógenas tardías de la Quebrada de Humahuaca, Provincia de Jujuy. Implicancias paleoambientales y paleobiogeográficas. 17° Congreso Geológico Argentino (Jujuy), Relatorio, 286–296.
- Roth, S. (1903). Noticias preliminares sobre nuevos mamíferos fosiles del Cretáceo superior y Terciario inferior de la Patagonia. Revista del Museo de La Plata, 11, 133–158.
- Rovereto, C. (1914). Los estratos araucanos y sus fósiles. Anales del Museo Nacional de Historia Natural, Buenos Aires, 25, 1–247.
- Saint-André, P.-A. (1999). Les Toxodontidés du Néogène de l’Altiplano bolivien. Paleontographica Abteilung A, 251(5–6), 79–148.
- Saint-André, P.-A., & De Iuliis, G. (2001). The smallest and most ancient representative of the genus Megatherium Cuvier, 1796 (Xenarthra, Tardigrada, Megatheriidae), from the Pliocene of the Bolivian Altiplano. Geodiversitas, 23(4), 625–645.
- Saint-André, P.-A., Pujos, F., Cartelle, C., De Iuliis, G., Gaudin, T. J., McDonald, H. G., & Mamani Quispe, B. (2010). Nouveaux paresseux terrestres (Mammalia, Xenarthra, Mylodontidae) du Néogène de l’Altiplano bolivien. Geodiversitas, 32(2), 255–306.
- Scott, W. B. (1903). Mammalia of the Santa Cruz Beds. Volumen V, Paleontology. Part I, Edentata. 2. Glyptodontia and Gravigrada; pp. 107-227 in W. B. Scott (ed.), Reports of the Princeton University Expeditions to Patagonia, 1896-1899. Princeton University, E. Schweizerbart'sche Verlagshandlung (E. Nägele), Stuttgart.
- Soibelzon E., Miño-Boilini A. R., Zurita A. E., & Krmpotic C. M. (2010). Los Xenarthra del Ensenadense (Pleistoceno Inferior a Medio) de la Región Pampeana (Argentina). Revista Mexicana de Ciencias Geológicas, 27, 449–469.
- Sylvester, S. P., Heitkamp, F., Sylvester, M. D. P. V., Jungkunst, H. F., Sipman, H. J. M., Toivonen, J. M., Gonzales Inca, C. A., Ospina, J. C., & Kessler, M. (2017). Relict high-Andean ecosystems challenge our concepts of naturalness and human impact. Scientific Reports, 7(3334), 1–13.
- Taglioretti, M., Miño-Boilini, Á. R., Scaglia, F., & Dondas, A. (2014). Presencia de Proscelidodon patrius (Xenartha, Scelidotheriinae) en la Formación Chapadmalal (Plioceno Superior), Mar Del Plata, Buenos Aires, Argentina: Implicancias Bioestratigráficas. Ameghiniana, 51(5), 420–427.
- Tejada, J., Báez, D., Gamarra, J., & Salas-Gismondi, R. (2009). Los mamíferos del Mioceno y Plioceno de Espinar (Cusco): expedición y resultados preliminares del proyecto. Bulletin de l’Institut Français d’Études Andines, 38(2), 359–364.
- Tejada J. V., Flynn J. J., MacPhee R., O’Connell T. C., Cerling T. E., Bermudez L., Capuñay C., Wallsgrove N., & Popp B. N. (2021). Isotope data from amino acids indicate Darwin’s ground sloth was not an herbivore. Scientific Reports, 11, 18944.
- Tejada, J., Gamarra, J., & Salas-Gismondi, R. (2009). Los mamíferos del Mioceno y Plioceno de Espinar (Cusco): antecedentes y proyecto. Bulletin de l’Institut Français d’Études Andines, 38(1), 131–135.
- Tejada-Lara, J. V, Salas-Gismondi, R., Pujos, F., Baby, P., Benammi, M., Brusset, S., de Franceschi, D., Espurt, N., Urbina, M., & Antonie, P.-O. (2015). Life in Proto-Amazonia: Middle Miocene mammals from the Fitzcarrald arch (Peruvian Amazonia). Palaeontology, 58(2), 1–38.
- Toledo, N., Cassini, G. H., Vizcaíno, S. F., & Bargo, M. S. (2014) Mass estimation of Santacrucian sloths from the Early Miocene Santa Cruz Formation of Patagonia, Argentina. Acta Palaeontologica Polonica, 59(2), 267–280.
- Tomassini, R. L., Montalvo, C. I., Deschamps, C. M., & Manera, T. (2013). Biostratigraphy and biochronology of the Monte Hermoso Formation (early Pliocene) at its type locality, Buenos Aires Province, Argentina. Journal of South American Earth Sciences, 48, 31–42.
- Vélez, M. I., Jaramillo, C., Salazar-Ríos, A., Benito, X., Fritz, S., Tapia, P., Lubiniecki, D., Kar, N., & Escobar, J. (2018). Aquatic ecosystems in a newly formed ecospace: Early Pliocene lakes in the Central Andean Altiplano. Palaeogeography, Palaeoclimatology, Palaeoecology, 490, 218–226.
- Vizcaíno, S. F., Blanco, R. E., Bender, J. B., & Milne, N. (2011a). Proportions and function of the limbs of glyptodonts. Lethaia, 44(1), 93–101.
- Vizcaíno, S. F., Cassini, G. H., Fernicola, J. C., & Bargo, M. S. (2011b). Evaluating habitats and feeding habits through ecomorphological features in glyptodonts (Mammalia, Xenarthra). Ameghiniana, 48(3): 305–319.
- Von den Driesch, A. (1976). A Guide to the Measurement of Animal Bones from Archaeological Sites. (1st ed.). Peabody Museum of Archaeology and Ethnology, Harvard University, 136 pp.
- Zurita, A. E., González Ruiz, L. R., Gómez-Cruz, A. J., & Arenas-Mosquera, J. E. (2013). The most complete known Neogene Glyptodontidae (Mammalia, Xenarthra, Cingulata) from northern South America: Taxonomic, paleobiogeographic, and phylogenetic implications. Journal of Vertebrate Paleontology, 33(3), 696–708.
- Zurita, A. E., Taglioretti, M., De los Reyes, M., Cuadrelli, F., & Poire, D. (2016). Regarding the real diversity of Glyptodontidae (Mammalia, Xenarthra) in the late Pliocene (Chapadmalalan Age/Stage) of Argentina. Anais Da Academia Brasileira de Ciências, 88(2), 809–827.