ABSTRACT
Remote Devonian exposures in central Australia have produced significant but highly fragmentary remains of fish-grade tetrapodomorphs. We describe a new tetrapodomorph from the Middle–Late Devonian (Givetian–Frasnian) Harajica Sandstone Member of the Amadeus Basin, Northern Territory, which is represented by several nearly complete skulls along with much of the body and postcranial skeleton. The new form has a posteriorly broad postparietal shield, broad, triangular extratemporal bones, and a lanceolate parasphenoid. The spiracular openings are particularly large, a character also recorded in elpistostegalians and Gogonasus, demonstrating that these structures, suggestive of spiracular surface air-breathing, appeared independently in widely differing nodes of the stem-tetrapod radiation. A phylogenetic analysis resolves the new form within a cluster of osteolepidid-grade taxa, either as part of a polytomy or as the most basally branching representative of a clade containing ‘osteolepidids,’ canowindrids, and megalichthyids.
INTRODUCTION
Clade Tetrapodomorpha comprises the limbed tetrapods and their closest fish relatives, whose earliest record is from the Pragian of China (Lu et al., Citation2012). The group diversified greatly in both marine and freshwater habitats during the Middle-to-Late Devonian while giving rise to several distinct lineages, including the earliest limbed tetrapods (Ahlberg, Citation2018). Whereas the tetrapods flourished after the Devonian, limbless fish-grade tetrapodomorphs underwent a marked reduction in diversity during the Carboniferous, with only a handful of representatives persisting into the early Permian before vanishing from the fossil record (Romano et al., Citation2014).
Australia is renowned for its excellent fossil record of Devonian tetrapodomorph fishes, with most discoveries in non-marine Givetian–Famennian sites located in the southeast of the continent (Ahlberg & Johanson, Citation1997; Johanson & Ahlberg, Citation1997, Citation1998; Long, Citation1985a, Citation1985b, Citation1987; Thomson, Citation1973; Young et al., Citation2013, Citation2019). The oldest Australian tetrapodomorph occurrence is in the Emsian–?Eifelian Hatchery Creek Group of Burrinjuck, New South Wales, the material initially provisionally referred to the European genus Gyroptychius by Young and Gorter (Citation1981), but more recently recognized to contain several taxa including a form close to Kenichthys from the Emsian of China (Hunt & Young, Citation2012; Young & Lu, Citation2020). Outside of this region, the spectacular Devonian reef deposits of the Kimberley region of north-western Western Australia have produced the exceptionally preserved marine vertebrate assemblage of the Gogo Formation (Long & Trinajstic, Citation2010), which includes Gogonasus Long Citation1985a, one of the best preserved of all tetrapodomorph fishes (Holland & Long, Citation2009; Long et al., Citation1997, Citation2006).
In contrast to these exceptional records, fossil collections from the extensive Devonian exposures of central Australia remain poorly sampled yet have already provided significant insights into the early evolution and distribution of tetrapodomorphs in Eastern Gondwana. The fossil record of this region includes the potentially oldest remains referrable to the Rhizodontida, based on fragmentary material from the Early–Middle Devonian Wuttagoonaspis fossil fish assemblage of the Georgina Basin of the Northern Territory and western Queensland (Young & Goujet, Citation2003). Elsewhere in East Gondwana, the rhizodontid Aztecia occurs in the Middle–Late Devonian of the Transantarctic Mountains (Johanson & Ahlberg, Citation2001; Young et al., Citation1992), and the most basally branching rhizodontid known from reasonably complete remains is Gooloogongia loomesi from the late Frasnian of Canowindra, New South Wales (Johanson & Ahlberg, Citation1998).
Other central Australian discoveries include several forms placed within the ‘Osteolepididae,’ a group now generally considered to represent a paraphyletic assemblage of basally-branching tetrapodomorphs (Ahlberg & Johanson, Citation1998). Muranjilepis winterensis is based on parietal and post-parietal shields from Mount Winter, in the Northern Territory (Young & Lu, Citation2020; Young & Schultze, Citation2005). Isolated cosmoid scales with posterior serrations from an unidentified ‘osteolepidid’ occur in the Cravens Peak Beds of the Toomba Range, western Queensland (Young & Schultze, Citation2005). These sites are dated as Emsian–Eifelian, and thus are also of comparable age to some of the earliest known tetrapodomorph fossils from Yunnan, China, namely Kenichthys campbelli (Chang & Zhu, Citation1993; Zhu & Ahlberg, Citation2004) from the Emsian Chuandong Formation, and the oldest and basally branching representative of the clade, Tungsenia paradoxa from the Pragian Posongchong Formation (Lu et al., Citation2012).
Another highly fragmentary record was a tetrapodomorph first mentioned by Young (Citation1985) as a “rhipidistian crossopterygian,” based on fragmentary material collected in 1973 from a fossil fish assemblage at “locality 6” () in the Harajica Sandstone Member of the Parke Siltstone, part of the non-marine Pertnjara Group of the Amadeus Basin in the Northern Territory (Jones, Citation1972, Citation1991). The material comprised a partial maxillary or dentary, an isolated mandibular element, and a denticulate plate presumed to come from the mouth cavity. The Harajica fossil fish assemblage is dominated by the widely distributed antiarch Bothriolepis sp. (Young, Citation1985), associated with the phyllolepid placoderm Placolepis harajica (Young, Citation1988, Citation2005), two types of acanthodian fin-spines (Young, Citation1985), and the dipnoan Harajicadipterus youngi (Clement, Citation2009).
FIGURE 1. Locality maps for the fossil material described in this work. A, the Amadeus Basin of central Australia. All fossils were collected from locality 6 (redrawn from figure 1 in Young, Citation1985). B, geological map of western MacDonnell Ranges, NT, showing locality 6 about 2 km southwest of Stokes Pass (redrawn from figure 6 in Young, Citation1985). Abbreviations: Pzk, Paleozoic.
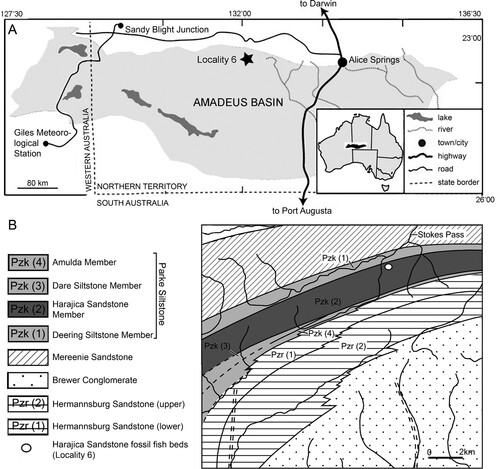
Additional specimens of the Harajica tetrapodomorph were recovered in collecting expeditions in 1991 and 2016 (), and this paper describes and analyses this new taxon. The new form is the only tetrapodomorph fish known from central Australia that is represented by articulated fossil material.
FIGURE 2. Harajicadectes zhumini from the Harajica Sandstone Member (Givetian–Frasnian), Northern Territory, Australia, Holotype NTM P6410. A, photographed as a natural mold in situ as it was discovered in 2016; B, as a whitened latex peel; and C, interpretative drawing.
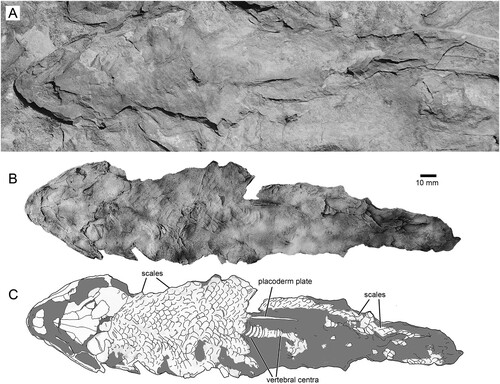
FIGURE 3. Harajicadectes zhumini, skulls in dorsal view, all whitened latex peels of the original fossil molds. A, photographs and B, drawings of NTM P6410 (holotype); C–D, CPC 39948 (paratype); E–F, NMVP 228722; G–H, AMF 99704.
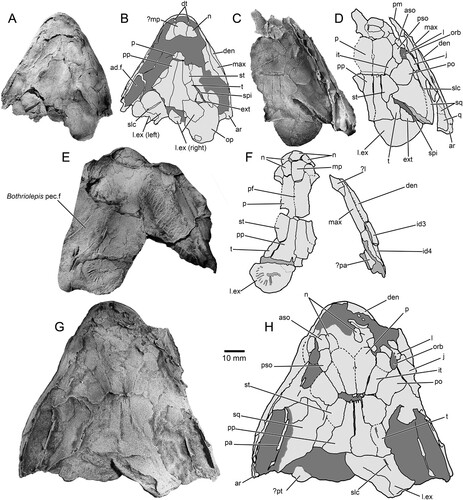
FIGURE 4. Harajicadectes zhumini, snout, jaws, and palate. A, photograph and B, drawing of a fragment of the snout in left dorsolateral view, CPC 39949B. C, photograph and D, drawing of isolated mandible, NMVP 253964. E, left maxilla in mesial view, NMV P253965B. F, partial maxilla and lingual surface of the mandible, NMV P253965A. G, photograph, H, drawing, and I, attempted reconstruction of the palate, CPC 33949A.
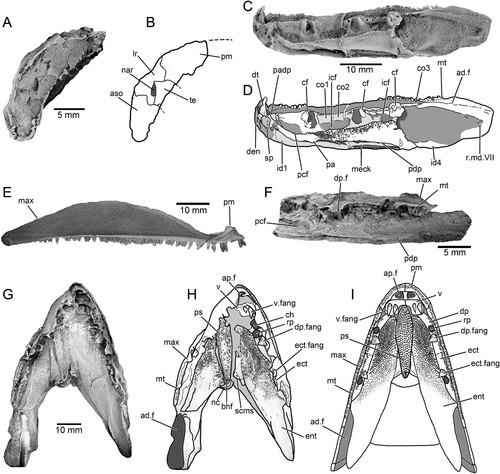
FIGURE 5. Harajicadectes zhumini, body and fins. A, photograph and B, drawing of body scales, opercular-gular elements and pectoral girdle, NMVP 229368. C, squamation and D, lepidotrichia from the rear of the body, immediately anterior to the base of the caudal fin, CPC 39953. E–F, squamation from the anterior of the body with partial head, operculum, and pectoral fin, CPC 39952. G–H, mesial view of mid-body squamation with detached pectoral fin and basal scute, CPC 39954.
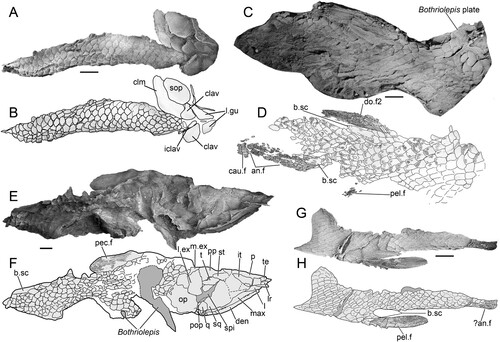
GEOLOGICAL SETTING
The Harajica Sandstone Member of the Parke Siltstone () was described in detail by Jones (Citation1972). Young (Citation1985) reported a measured section with the fish remains mainly restricted to a 15 m zone 75–90 m above the underlying Deering Siltstone, the basally branching member of the Pertnjara Group. Young (Citation1985) initially estimated a Givetian–Frasnian age for the Harajica fish assemblage. The underlying Deering Siltstone produced a single fish impression with coarse tubercular ornament from a nearby locality (Dare Plain; locality 2 of Young, Citation1985). A better specimen (Young, Citation1988) from a similar horizon near the base of the Pertnjara Group at Mount Winter is a spine referable to the placoderm Wuttagoonaspis. The Wuttagoonaspis assemblage is widely distributed across the Early–Middle Devonian of central Australia (Young & Goujet, Citation2003). In the western part of the Amadeus Basin it is known from the base of the Pertnjara Group and upper part of the Mereenie Sandstone (Young & Schultze, Citation2005; Young & Turner, Citation2000). Young (Citation1991) noted that a Geminospora lemurata spore assemblage, previously reported from Dare Plain, elsewhere ranges down into the Eifelian, which was consistent with a Givetian age for the Amadeus Basin occurrence. Taking into account other microvertebrate evidence, Young and Turner (Citation2000: fig. 4) correlated the Harajica fauna with the Antarctic Aztec fish assemblage (Macrovertebrate zone 6d), of probable Givetian age.
MATERIALS AND METHODS
All fossils are preserved as natural molds within blocks of red sandstone, often intermingled with specimens of Bothriolepis. CPC24700 and 24702 were collected by Gavin Young in 1973. CPC 33948-33954, all NMVP specimens, along with AMF 99703 and 99704 found by Alex Ritchie, were collected during a 1991 field excursion for the Conference on Australasian Vertebrate Evolution, Palaeontology and Systematics led by Gavin Young. NTM P6410 and P6411 were collected in 2016 during a Flinders University expedition led by John Long. NTM P6410 is selected as the holotype as it combines a diagnostic posterior skull roof, squamation and a reasonably complete body outline. It establishes that the other disjunct fossil material from the site belongs to a single tetrapodomorph taxon.
The fossils were scrubbed with water and brushes to remove fine sandstone grains covering impressions of the specimens, while larger regions of surrounding matrix were excised with a carbide rod and an ARO Model 8315 engraving pen. Casting was undertaken in black latex and whitened in ammonium chloride sublimate for presentation. Photographs were taken with a Nikon D80 camera with a Tamaron 90 mm macro lens.
A phylogenetic analysis was performed to determine the phylogenetic position of Harajicadectes among the basally branching Tetrapodomorpha. We amended the data matrix presented in Cloutier et al. (Citation2020) to examine the relationships of Elpistostege, which in turn was an expanded version of the matrix published by Zhu et al. (Citation2017) to examine the phylogenetic position of Hongyu. Our revised matrix comprises 202 characters and 44 taxa, including 39 tetrapodomorphs plus an outgroup of 5 Devonian dipnomorphs (Diabolepis, Youngolepis, Powichthys, Porolepis, and Glyptolepis). All characters were treated as unordered in this analysis and a detailed description of the characters is presented as Supplemental File 1. In addition to the inclusion of Harajicadectes, fresh observations by one of us (TC) resulted in recodings within seven rhizodontid taxa (see Appendix S2 in Supplemental File 1). For the analysis itself conducted with PAUP, tree bisection and reconnection was used as the branch-swapping algorithm, with zero branch lengths collapsed using a random-addition sequence method with 10,000 replicates. The full character-by-taxon matrix is available as a NEXUS file in the electronic supplement (Supplemental File 2) and on MorphoBank (project 4724; http://morphobank.org/permalink/?P4724). The data logs detailing the scripts and workflow used for running the analysis (Supplemental File 3) along with the tree file (Supplemental File 4) are also presented.
Institutional Abbreviations—AMF, Australian Museum, Sydney; CPC, Commonwealth Palaeontological Collection, Geoscience Australia, Canberra, Australia; NMVP, National Museum of Victoria, Melbourne, Australia; NTM, Museum and Art Galleries of the Northern Territory, Darwin, Australia.
Anatomical Abbreviations—Anatomical terminology follows that of Andrews (Citation1985) and Ahlberg and Johanson (Citation1997). ad.f, adductor fossa; an.f, anal fin; ap.f, apical fossa; ar, articular; aso, anterior supraorbital; b.sc, basal scute; bhf, buccohypophyseal foramen; cau.f, fragmentary caudal fin; cf, coronoid fang; ch, choana; clav, clavicle; clm, cleithrum; co, coronoid; den, dentary; do.f2, 2nd dorsal fin; dp, dermopalatine; dp.fang, dermopalatine fang; dt, dentary tusks; ect, ectopterygoid; ect.fang, ectopterygoid fang; ent, entopterygoid; ext, extratemporal; icf, intercoronoid fossa; iclav, interclavicle; id, infradentaries; it, intertemporal; j, jugal; l, lachrymal; l.ex, lateral extrascapular; l.gu, lateral gular; lr, lateral rostral; m.ex, median extrascapular; max, maxilla; meck, Meckelian bone; mp, median postrostral; mt, marginal teeth; n, nasals; nar, external nasal opening; nc, rim of notochordal canal; op, operculum; orb, orbit; p, parietal; pa, prearticular; padp, parasymphysial dental plate; pcf, precoronoid fossa; pdp, prearticular dental plate; pec.f, pectoral fin; pel.f, pelvic fin; pf, pineal foramen; pm, premaxilla; po, postorbital; pop, preoperculum; pp, postparietal; ps, parasphenoid; pso, posterior supraorbital; pt, post-temporal; q, quadratojugal; r.md.VII, foramen for ramus mandibularis of nervus facialis; rp, replacement pit; scms, subcranial muscle scar; slc, sensory line canal; sop, suboperculum; sp, symphysial pit; spi, spiracular opening; sq, squamosal; st, supratemporal; t, tabular; te, (anterior) tectal; v, vomer; v.fang, vomerine fang.
SYSTEMATIC PALEONTOLOGY
OSTEICHTHYES Huxley, Citation1880
SARCOPTERYGII Romer, Citation1955
TETRAPODOMORPHA Ahlberg, Citation1991
Genus HARAJICADECTES gen. nov.
HARAJICADECTES ZHUMINI, gen. nov. et sp. nov.
()
FIGURE 7. Harajicadectes zhumini. Reconstructed skull and pectoral girdle in A, dorsal and B, lateral view.
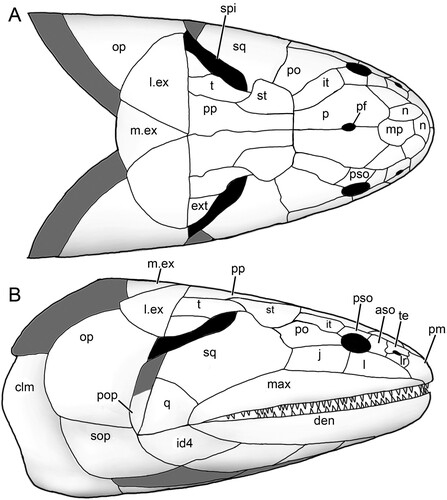
‘rhipidistian crossopterygian’: Young, Citation1985:246.
‘rhipidistian osteichthyan’: Young, Citation1991:107.
‘osteolepid indet.’: Young, Long and Turner, Citation1993:248.
‘articulated sarcopterygians’: Young and Turner, Citation2000:107.
‘rhipidistian crossopterygian’: Clement, Citation2009:615.
LSID. zoobank.org:pub:A21E263E-9B2B-4C9B-9B1B-74B53C2CF535
Holotype—NTM P6410: partial skull and much of the body outline in dorsal view with squamation preserved.
Paratype—CPC 39948: partial skull, right post-temporal and right lower jaw.
Referred Material—AMF 99703: pectoral fin elements; AMF 99704: crushed skull with mandibles in dorsal view; CPC 24700: section of squamation and fragmentary jaw elements. CPC 24702: denticulated tooth surface, either a partial entopterygoid or prearticular dental plate. CPC 39949: skull in palatal view (39949A) and a detached fragment of the snout tip (39949B); CPC 39950: partial palate; CPC 39952: partial skull, left pectoral fin and scales from the anterior half of body; CPC 39953: rear part of the body, comprising scales, median fins, and a fragmentary pelvic fin; CPC 39954: detached pectoral fin with basal scute superimposed over flank squamation preserved in visceral aspect; NMVP 229354: partial palate and scales from the anterior body; NMVP 228722: partial skull roof, left post-temporal, and right lower jaw in dorsal view. NMVP 229368: partial opercular-gular series, pectoral girdle, and body scales; NMVP 253964: right mandible in lingual view; NMVP 253965: fragmentary right mandible and dermopalatine (253965A), and an associated complete left maxilla in mesial view (253965B); NTM P6411: section of vertebral column and associated scales.
Etymology—“Harajica Biter.” Named for the Harajica Sandstone Member and the ancient Greek dēktēs (“biter”) in reference to the animal’s large fangs and presumed predatory habits. The species honors Professor Min Zhu of the Institute of Vertebrate Paleontology and Paleoanthropology, Beijing, China, for his numerous contributions to early vertebrate paleontology.
Type Locality and Horizon—Harajica Sandstone Member of the Parke Siltstone. Locality 6 of Young (Citation1985), about 2 km southwest of the southern end of Stokes Pass, Amadeus Basin, Northern Territory. Givetian–Frasnian in age ().
Diagnosis—Tetrapodomorph fish with greatly enlarged spiracular openings, comprising over 20% of the total length of the skull-roof, bordered by the tabular, extratemporal, and squamosal. Parietal and postparietals of roughly equal length. Elongate intertemporal that widens anteriorly. Posterior nasals narrower than the anterior and posterior supraorbitals. Median extrascapular tapers anteriorly into a V-shaped recess formed by the large rounded lateral extrascapulars. Elongate lanceolate parasphenoid with denticles larger on the anterior part of the bone. Scales display ridged ornamentation and lack cosmine. Anterior squamation cycloid, abruptly shifting to a rhombic shape on the rear flank.
DESCRIPTION
General Features
The holotype (NTM P6410) preserves a head and fusiform body outline with a total length of 26.5 cm (). The posterior end of the fossil terminates shortly before the caudal fin, suggesting an original total length of roughly 30 cm, assuming similar proportions to those of Canowindra (Thomson, Citation1973) or Gogonasus (Long et al., Citation2006; Long & Trinajstic, Citation2017). Assuming minimal distortion of the incompletely preserved skull bones, the holotype skull length is about 4.8 cm from the snout to the posterior margin of the post-parietals. The largest skull, AMF 99704, has a length of 7.6 cm, suggesting a fish 45–50 cm long (). Only a few small patches of cranial dermal ornament are preserved, with faint impressions suggesting wavy ridges on the skull roof and a vermiculate pattern of short ridges and pores on the snout (). Sensory canals are also rarely preserved, restricted to short sections of lateral line canals on the lateral extrascapular of NTM P6410 () and AMF 99704 () and on the squamosal of CPC 33948 (). A reconstruction of the complete skull, opercular-gular series, and pectoral girdle in articulation is presented in .
Skull
Snout—Various regions of the snout are preserved throughout different specimens of Harajicadectes (, , ), with the median post rostral and nasals best shown in NMV P228722 (). The median post rostral is an anteroposteriorly elongate hexagon, approximately half the length of the parietals, and is the width of a single parietal. It is laterally bordered on each side by smaller single nasal bones, which closely match the median post-rostral in shape. Lateral abutment of the median post rostral by single nasal bones is also present in Eusthenodon (Jarvik, Citation1952) and Cladarosymblema (Fox et al., Citation1995), although the configuration and number of nasals varies within these taxa. At least two small sub-hexagonal nasals anteriorly straddle the median post-rostral. Both are approximately half the length of the median post rostral but equal in width. A small bone may sit in between these two anterior nasals, and is approximately 1/6 the width of each. A small rectangular anterior tectal and lateral rostral bones are situated between the anterior suborbital and premaxilla (, ). A single pair of external nostrils was present, with the only unambiguous nostril visible on CPC 39949B, entirely enclosed by the anterior tectal and lateral rostral ().
Skull Roof—The parietal and postparietals of Harajicadectes () are of roughly equal anteroposterior length. This is a condition also seen in tristichopterids (Schultze & Reed, Citation2012; Young et al., Citation2013) and elpistostegalians (Vorobyeva, Citation1980; Cloutier et al., Citation2020), whereas more basally-branching forms such as Gogonasus (Long et al., Citation1997), Marsdenichthys (Holland et al., Citation2010), and canowindrids (Long, Citation1985b) typically possess postparietals that are significantly longer than the parietals.
The parietal shield () is well preserved in AMF 99704 and the paratype CPC 39948, while only the parietals are visible in NMV P228722. The parietals are elongate, sub-rectangular bones, being three times as long as wide and slightly smaller or equal in length to the postparietals, a condition seen in some specimens of Gyroptychius (Thomson, Citation1965) and tristichopterid taxa such as Mandageria (Johanson & Ahlberg, Citation1997) and Eusthenopteron (Jarvik, Citation1980). The anterior margin of each parietal varies between being pointed, to laterally border the median post rostral, or concave to surround the posterior-most nasal bone. The median suture between the parietals is visible in CPC 39948 and NMV P228722, with the latter clearly showing a small circular opening for the pineal foramen, as opposed to the large kite-shaped pineal bone of tristichopterids such as Eusthenodon (Jarvik, Citation1952) or Mandageria (Johanson & Ahlberg, Citation1997). In megalichthyids the pineal foramen is closed (Fox et al., Citation1995; Janvier et al., Citation2007; Thomson, Citation1964). The posterior end of both parietals is wider than the anterior edge of both postparietals. Parietal pit lines are not preserved.
The intertemporal is elongate, being three times as long as wide, and is approximately equal in length to the supratemporal and tabular of the postparietal shield. Towards the anterior end, the intertemporal widens, with a transverse anterior margin. This differs from the narrow, pointed anterior end of the intertemporal in tristichopterids (e.g., Eusthenopteron, Eusthenodon, Mandageria, Cabonnichthys, Edenopteron; Ahlberg & Johanson, Citation1997; Jarvik, Citation1944, Citation1952; Johanson & Ahlberg, Citation1997; Young et al., Citation2019). The anterior end is also pointed in Cladarosymblema (Fox et al., Citation1995), whereas an anteriorly broad intertemporal is seen in Marsdenichthys (Holland et al., Citation2010) and Medoevia (Lebedev, Citation1995). However, in the latter two taxa the intertemporal is relatively shorter compared with Harajicadectes. The ‘posterior postorbital’ in an equivalent position in Canowindra is also broader anteriorly and pointed posteriorly (Long, Citation1985b).
Both posterior and anterior supraorbitals are short, but broad. The posterior supraorbital has a blunt or rounded posterior end, distinct from the pointed corresponding region of the posterior supraorbital in Eusthenodon (Jarvik, Citation1952), Mandageria (Johanson & Ahlberg, Citation1997), and Cabonnichthys (Ahlberg & Johanson, Citation1997). The right posterior supraorbital on CPC 33948 appears to be either broken or could be two separate bones (). A strip of narrow, fluted bone delineates the anterior margin of the postparietals (). Each postparietal has a narrow anterior section, and broadens posteriorly so the rear half of the bone is more than double the anterior breadth. The postparietals are joined at a gently undulating median suture, and the posterior margins are relatively straight, as in the Tristichopteridae (Jarvik, Citation1996; Johanson & Ahlberg, Citation1997; Young et al., Citation2013, Citation2019). In contrast, in Gogonasus (Long et al., Citation2006), canowindrids (Long, Citation1985b, Citation1987), and the megalichthyid Litoptychius (Schultze & Chorn, Citation1998), the posterior margin of the postparietal shield displays some form of curvature or projection. A faint pitline is visible crossing the left postparietal in NMVP 228722.
The supratemporals are located laterally to the anterior portion of each postparietal, with the best-preserved examples visible on CPC 33948 () and AMF 99704 (). Each supratemporal is relatively large and roughly semi-circular, exhibiting a wide convex lateral border. This width is over double that of the anterior breadth of a postparietal. It is of roughly equal length to the tabular, in contrast to Glyptopomus (Jarvik, Citation1950) and Jarvikina (Vorobjeva, Citation1977) where the supratemporal is approximately double the length of the tabular.
The tabulars are elongate and sub-rectangular, about half the width of the supratemporal. This is distinct amongst tetrapodomorph fish except for the basally-branching rhizodontid Gooloogongia (Johanson & Ahlberg, Citation2001). However, unlike Gooloogongia, the tabulars in Harajicadectes flank greatly enlarged external openings for the spiracular chambers. As in rhizodontids and ‘osteolepidids,’ the tabulars are bordered laterally by the extratemporals. These are triangular, and slightly wider than the tabulars. Canowindra also has a triangular extratemporal.
Cranial preservation of the holotype (, ), CPC 33948 () and CPC 39952 () reveal the natural boundary between the skull roof and the cheek unit. The most conspicuous character is the greatly enlarged spiracular opening, accounting for between 25–30% of the total length of the skull roof. The opening is bordered posteromesially by the tabular and extratemporal, anterolaterally by the squamosal (and preopercular if present). The bony slit appears to have been open-ended posteriorly.
The spiracle is a minute slit in the majority of early osteichthyans (Jarvik, Citation1980). Among Devonian forms, enlarged spiracular openings are present in the marine tetrapodomorph Gogonasus (Long et al., Citation2006), elpistostegalian-grade stem-tetrapods (Brazeau & Ahlberg, Citation2006; Cloutier et al., Citation2020; Daeschler et al., Citation2006) and the actinopterygian Pickeringius (Choo et al., Citation2019). In Gogonasus (Long et al., Citation2006), the tabulars have ventrally directed laminae forming a sloping wall to the enlarged spiracles, but these (if present) are not displayed in the tabulars of Harijicadectes.
Cheek—The maxilla is preserved in articulation in a number of specimens (, ), and NMV P253965 () includes a complete disarticulated example in mesial view. It is an elongate bar-like bone, approximately equal in length to the parietal and postparietal combined. While there is individual variation in proportions, in all specimens the bone gently tapers anteriorly to a similar extent to the maxilla of Marsdenichthys (Holland et al., Citation2010), but substantially less than that of Megalichthys (Miall, Citation1885), Rhizodopsis (Traquair, Citation1881), Gogonasus (Long et al., Citation1997), and Latvius (Jessen, Citation1973). In mesial view (), the bone flares dorsally at the contact with the premaxilla, indicating the presence of a substantial infraorbital overlap surface. Posteriorly, the maxilla tapers slightly towards a rounded tip. Numerous sharp, conical teeth are positioned along the ventral margin except for the posterior 15%. More than 45 teeth are present on NMV P253965. The internal surface of this specimen shows a continuous ridge diverging from the tooth row with an anterodorsally directed flange at the anterior extremity. This feature, which contributes to the posterior margin of the choana, is also present in Cladarosymblema (Fox et al., Citation1995), Medoevia (Lebedev, Citation1995), and Gogonasus (Long et al., Citation1997).
The lachrymal (, ) has a steep posterior suture with the jugal and has a short, posterodorsal concavity that contributes to the relatively small orbit. Based on CPC 33948 (), the jugal is rectangular in shape and of similar size to the lachrymal, with a narrow anterodorsal contribution to the orbit. The postorbital () is preserved only in CPC 33948 and AMF 99704. Unlike Osteolepis and Gogonasus (Long et al., Citation1997) the postorbital does not extend posteriorly as far as the spiracular slit.
The squamosal is incompletely known, with partial examples preserved on CPC 33948 (), CPC 39952 (), and AMF 99704 (). It is a large bone, comparable in size to the entire postparietal shield. The entire posterodorsal margin forms most of the anterolateral margin of the spiracular slit, with a short anteromedial concavity to accommodate the lateral curvature of the supratemporal. The quadratojugal, visible only in CPC 39952 (), is a triangular bone, roughly 1/7th the size of the squamosal with which it sutures anterodorsally. An unambiguous preopercular has not been identified in the available material.
Extrascapulars—The extrascapular series (, ) consists of paired lateral and a single median element. One complete lateral extrascapular is preserved in position in CPC 33948 () and NMVP 228722 () and a partial median extrascapular is visible on CPC 39952. The lateral units are large, semicircular bones, of comparable size to the parietal shield. NMVP 228722 shows ornamental grooves radiating from the ossification center of the bone. The median extrascapular is a triangular bone, about half the size of the laterals, which tapers towards an anterior point.
Palate and Ethmosphenoid—CPC 33949A () preserves the best example of the palatal anatomy. The parasphenoid is an elongate, lanceolate element with a denticulated surface that tapers towards a rounded posterior extremity. The anterior tip is not clearly visible, but the lateral contours and posterior embayment formed by the vomers indicate that it gently tapers towards the front. The denticles are distinctly larger on the anterior half of the bone, with a slightly raised single row of denticles along the posterolateral margins surrounding a central depression that housed the buccohypophyseal foramen.
A worn right vomer is visible in its natural position, bearing two pronounced conical fangs. The vomer consists of a broad, lateral section with a straight posterior margin, lacking the tapering posterior projections present in Eusthenopteron (Jarvik, Citation1980) and mandageriines (Young et al., Citation2013, Citation2019). A narrow anteromedial ramus projects towards a presumed contact with the opposite vomer, forming an embayment to accommodate the anterior tip of the parasphenoid. The vomer of Megalichthys also possesses a medially directed extension (Jarvik, Citation1966). In that taxon the bone gently tapers towards the midline of the palate, rather than being sharply separated into a narrow medial and broad lateral sections as in Harajicadectes.
The denticulated surfaces of both entopterygoids are preserved, although heavily fractured due to post-mortem compression. A wedge-shaped mass of bone to the right of the parasphenoid (left in palatal view) might represent a discrete element (), perhaps comparable to the ‘accessory vomers’ first described from the Canowindra tristichopterids Mandageria and Cabonnichthys (Ahlberg & Johanson, Citation1997; Johanson & Ahlberg, Citation1997). In the absence of an unambiguous counterpart on the left side and without supporting evidence from additional specimens, this is currently interpreted as a fractured section of the entopterygoid. Denticles are larger in the anterior parts of the entopterygoids, particularly along the lateral margins, becoming progressively finer and more scattered towards the posterior, with the rear quarter of the bone being almost devoid of ornamentation. The dermopalatines and ectopterygoids are preserved, but the suture between them is difficult to discern. Each bone bears a single stout, conical fang, mesial to a row of fine denticles.
Small sections of the ethmosphenoid floor are visible adjacent to the parasphenoid, although insufficiently preserved to accurately determine the overall shape. A broken section to the left (right in palatal view) of the parasphenoid and partially underlain by the entopterygoid bears a prominent subcranial muscle scar. A roughened semicircular surface immediately posterior of the parasphenoid likely represents the rim of the notochordal chamber.
Lower Jaw—The labial surface of the mandible is partially visible in the holotype (), CPC 39952 (), and a variety of isolated heads (). The lingual surface is reasonably complete in NMV P253964 (), the only specimen that preserves the entire outline of the mandible, and partially visible in NMV P253965A (). NMV P253964 has a straight occlusal margin and a gently convex ventral surface. It tapers only very gently from back to front, so the anterior jaw tip is only marginally narrower than the rear. The occlusal margin has small conical teeth, arranged in at least two rows, from the anterior tip to over the anterior-third of the adductor fossa. A single large, recurved symphysial tusk is present at the anterior of the dentary, 4–5 times the size of the marginal teeth.
The prearticular of NMV P253964, while missing the rear end, is seen to expand posteriorly, gently narrowing anteriorly and bending dorsally, terminating shortly before the median symphysis. Part of the denticulated prearticular plate is preserved, the denticles being substantially larger along the dorsal edge. A highly fragmentary parasymphysial plate is preserved immediately anterior to the prearticular. Three coronoids appear to be present, but it is difficult to discern the sutures between them and the third in the series is poorly preserved. A continuous vertical lamina bearing a row of closely packed denticles runs along the entire coronoid series. Positioned labially to this lamina, each coronoid bears large, stout fangs and adjacent replacement pits.
Body and Fins
Scales—The scales of Harajicadectes are found in direct association with cranial material in the holotype () and CPC 39952 (), enabling the identification of headless, scale-bearing specimens (CPC 39951, 39953, 39954, NMVP 229368, NTM P6411) as this taxon. The squamation is cycloid in the anterior and mid-sections of the postcranium (), abruptly shifting to a rhombic shape in the vertical rows immediately behind the level of the second dorsal fin (). This shift in scale morphology from rounded to angular is unusual for a tetrapodomorph fish, but is also recorded in Marsdenichthys (Holland et al., Citation2010) and Rhizodopsis (Andrews & Westoll, Citation1970).
The exposed external surfaces of the scales lack cosmine and are ornamented, as in rhizodontids (Holland et al., Citation2007), tristichopterids (Jarvik, Citation1952), Marsdenichthys (Holland et al., Citation2010), Medoevia (Lebedev, Citation1995), Rhizodopsis (Schultze & Heidtke, Citation1986) and Canowindra (Thomson, Citation1973). Each scale has approximately 25-35 anteroposteriorly directed sub-parallel ridges (), which appear wider and less densely packed than those of Marsdenichthys (Holland et al., Citation2010). This morphology differs from that of Mandageria, Cabonnichthys, and Notorhizodon, in which scale ornamentation is composed of widely separated parallel grooves (Young, Citation2008; Young et al., Citation2013). Of a selection of isolated tetrapodomorph scales figured by Burrow and Turner (Citation2012) from Emsian?/Eifelian Cravens Peak Beds, Queensland, two specimens (ANU V3534 and V3535) display similar ornamentation to Harajicadectes.
In Harajicadectes, the course of these ridges becomes rounded, following the curvature of the scale border. The concentric intersecting lines characteristic of Marsdenichthys and Rhizodopsis (Holland et al., Citation2010) are absent.
Fins—No endoskeletal fin elements are preserved in any of the material. A left pectoral fin is present on CPC 39952 () while CPC 39954 displays a probable right pectoral fin with an associated basal scute which appears to have detached and flipped post-mortem to lie against the internal surfaces of squamation from the right side of the body (). The fin is paddle-shaped with a rounded tip, with over 19 unsegmented proximal lepidotrichia that transition into finer rays towards the distal tip. The base of the fin is poorly preserved and the disposition of the fleshy lobe and basal scute is unclear. The fin is narrower than the pectoral fin, with over 12 elongate proximal lepidotrichia which progressively divide into over 25 fine distal fin rays. Fragmentary scales, smaller than the adjacent trunk scales, cover the proximal fin rays, suggesting the presence of a fleshy basal lobe, although its size and shape are indeterminate.
CPC 39953 preserves the anal and second dorsal fins along with small fragments of the pelvic and caudal fin. No lepidotrichia of the first dorsal fin are visible, although CPC 39952 preserves a basal scute (). The second dorsal and anal fins are elongate and narrow based, with associated basal scutes longer than the fin bases. The anal fin is positioned posterior to the second dorsal fin, at the transition zone from the cycloid scales of the mid-body to the rhombic scales on the posterior. It has a rounded tip; the distal end of the dorsal fin is damaged.
PHYLOGENETIC ANALYSIS
To assess the phylogenetic relationships of Harajicadectes within Tetrapodomorpha, the taxon was scored using the dataset of Cloutier et al. (Citation2020). A heuristic search resulted in 336 trees with a length of 438 steps, which are presented as strict consensus and 50% majority rules trees (). As with analyses conducted with earlier versions of this matrix (Cloutier et al., Citation2020; Zhu et al., Citation2017), Rhizodontida, Kenichthys and Tungsenia are resolved as successive outgroups to the remainder of the Tetrapodomorpha, with the enigmatic Hongyu chowi maintaining its position as an aberrant rhizodont (Zhu et al., Citation2017). Above the Rhizodontida, the strict consensus tree presents a large polytomy consisting of Harajicadectes alongside various osteolepidid-grade taxa and the clades Canowindridae, Megalichthyidae, and Eotetrapodiformes.
FIGURE 8. Proposed phylogenetic interrelationships among early Tetrapodomorpha incorporating new data from Harajicadectes, collated from 336 most parsimonious trees. A, strict consensus tree, numbers represent Bremer support values. B, 50% majority-rule consensus tree, numbers are node support bootstrap values.
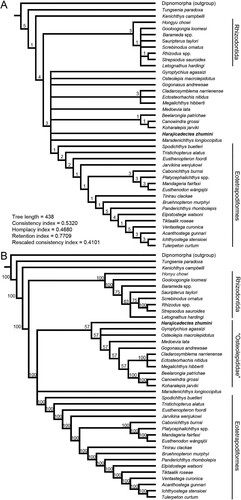
In contrast, the 50% majority tree replaces this polytomy with a trichotomy consisting of Eotetrapodiformes, the problematic Marsdenichthys Long (Citation1985a) and a clade whose basal section contains various ‘osteolepidid-grade’ taxa which form successive outgroups to the Canowindridae and Megalichthyidae. The position of Marsdenichthys within previous analyses has been highly unstable, having been placed as the sister-taxon to the Tristichopteridae (Long, Citation1985a), within the Canowindridae (Swartz, Citation2012), the sister-taxon to the Eotetrapodiformes (Zhu et al., Citation2017), or clustering among the osteolepidid-grade forms in a position basal to Gogonasus (Holland & Long, Citation2009; Long et al., Citation2006).
In the 50% majority consensus tree, Harajicadectes is resolved as the most basally-branching member of this ‘osteolepidid-clade.’ Effectively a reconstituted Osteolepididae sensu lato, this clade was previously resolved in Cloutier et al. (Citation2020) and Clement et al. (Citation2021), contrasting with earlier analyses that resolved this cluster of taxa either as polytomy (Zhu et al., Citation2017) or as multiple outgroups of varying composition to the Eotetrapodiformes, with the Canowindridae occupying either a basal (Swartz, Citation2012) or derived (Lu et al., Citation2012) position in the cluster. This analysis recovers poor support for this clade with only a single unambiguous character, namely the shift in marginal denticle bands of the coronoids (Character 98) from a single or absent row to a narrow band with 2–4 rows of denticles.
DISCUSSION
Resolving the Relationships of Harajicadectes
In our cladistic analysis, Harajicadectes sits above the Rhizodontida and is resolved among a paraphyletic grade of Middle–Late Devonian osteolepidid-grade taxa, either within a large polytomy or as the most basally-branching representative of a series of outgroups to the Canowindridae and Megalichthyidae, although support for this outcome is low. Beyond that, the interrelationships of the new taxon are poorly resolved with no other tetrapodomorph in the analysis exhibiting a consistent sister-group relationship with it. It is likely that convergent evolution is obscuring the relationships of this taxon.
Overall, Harajicadectes presents a highly distinct mosaic of characters which elsewhere manifests in disparate tetrapodomorph taxa. Much of the anatomy of Harajicadectes is characteristic of taxa basal to the Eotetrapodiformes, including the presence of an extratemporal, an ethmosphenoid that is of equal length or shorter than the otoccipital and the pronounced posterodorsal process of the maxilla. Multiple rows of denticles on the coronoids are also present in osteolepidid-grade taxa and Megalichthyidae (Clement et al., Citation2021), but absent in Rhizodontida and Eotetrapodiformes. The short, tapering anterior edge of the median extrascapular is implied by the shape of the broad post-temporals, a feature elsewhere present in Canowindridae (Long, Citation1987; Thomson, Citation1973; Young et al., Citation1992), Rhizodontida (Johanson & Ahlberg, Citation1997; Long & Ahlberg, Citation1999) and in Mandageria (Johanson & Ahlberg, Citation1997). The body squamation transitions from round anterior scales to rhombic scales towards the tail. Among other tetrapodomorph fishes, this configuration is only found in Marsdenichthys (Long, Citation1985a).
The Devonian fossil faunas of central Australia remain poorly sampled and Harajicadectes represents the first fossil osteichthyan taxon from this region based on reasonably complete articulated remains. Within the Harajica Sandstone Member, the only other osteichthyan remains belong to the dipnoan Harajicadipterus youngi, another highly distinctive taxon whose close interrelationships are difficult to resolve (Clement, Citation2009). East Gondwana experienced a high degree of vertebrate endemism during the Early Devonian, giving way to a more cosmopolitan fauna by the Late Devonian (Young et al., Citation2010). The Harajica fossil fauna, containing two highly distinct and presumably regionally endemic sarcopterygians, alongside the abundant cosmopolitan placoderm Bothriolepis (Young, Citation1985) may represent a transitional phase during this faunal shift.
Spiracular Function and the Devonian Atmosphere
Of considerable note are the enlarged spiracular openings in Harajicadectes. These are similar in relative size to those seen in elpistostegalian fishes (Clack, Citation2007; Cloutier et al., Citation2020; MacIver et al., Citation2017) and Gogonasus (Long et al., Citation2006). They are considerably larger than those in Eusthenopteron, which is thought to retain the plesiomorphic tetrapodomorph condition (Clack, Citation2007).
The separation of taxa with enlarged spiracular notches throughout tetrapodomorph phylogeny suggests that this character is likely to have arisen multiple times. In fact, enlarged openings are also known in several actinopterygian taxa including the Late Devonian Pickeringius (Choo et al., Citation2019) and the extant bichirs, Polypterus. It was recently confirmed that Polypterus perform spiracle-mediated aspiration and possess a pulmonary circulatory system similar to that found in lungfish and tetrapods (Graham et al., Citation2014). Furthermore, during the Middle–Late Devonian there were other taxa, namely lungfishes, that also seemed to be developing air-breathing adaptations alongside the appearance of enlarged spiracles in the groups mentioned above (Clement & Long, Citation2010; Clement et al., Citation2016; Long, Citation1993). The synchronized appearance of these adaptations in parallel in multiple lineages of osteichthyans is noteworthy and can perhaps be related to increases in global oxygen levels during the Middle Devonian (Cannell et al., Citation2022).
There have been numerous efforts attempting to reconstruct global oxygen levels throughout the Paleozoic. Researchers have contemplated the connection between global temperature and climate, oxygen levels and the evolution of early vertebrates and air-breathing behavior for a long time (Barrell, Citation1916). Berner and colleagues have proposed mathematical models based the geochemical cycles of carbon and sulfur that invariably show a decrease in global oxygen levels to below that of present day during the early to mid-Devonian, before sharply increasing again from the Late Devonian and throughout the Carboniferous (Berner, Citation2006, Citation2009; Berner & Canfield, Citation1989; Berner et al., Citation2007). However, while the estimated paleoatmospheric oxygen levels between models can vary considerably between studies, even more recent refinements (notably GEOCARBSULFOR) continue to find that global oxygen levels were well below those of present day until near the end of the Devonian (Krause et al., Citation2018).
The work of Scott and Glasspool (Citation2006) echoes the findings of Berner’s models of Paleozoic atmospheric oxygen levels via their work using the presence of charcoal as a proxy for fire. Low levels of charcoal present close to the Silurian/Devonian boundary suggest oxygen levels high enough for the ‘fire window’ (13–35% O2), compared with the following Middle–Late Devonian interval which exhibits a dearth of charcoal supporting predicted low global oxygen levels, before dramatic increases throughout the Carboniferous. Both He et al. (Citation2019) and Cannell et al. (Citation2022) show that there is now evidence for a global peak in oxygen during the Middle Devonian.
Increases in global atmospheric oxygen are commonly considered to have aided the vertebrate invasion of land (tetrapods) via supporting increased metabolic activity, boosting the effectiveness of lungs, and lowering desiccation risk (Clack, Citation2012; Graham et al., Citation2016). The parallel appearance of air-breathing adaptations, such as spiracular breathing, in several lineages of osteichthyans during a time of decreased paleoatmospheric oxygen could suggest that the ability to supplement gill respiration with aerial oxygen likely afforded some adaptive advantage to those taxa.
CONCLUSIONS
Harajicadectes zhumini is a new taxon of fish-grade stem-tetrapod from the Middle–Late Devonian (Givetian–Frasnian) of central Australia. A phylogenetic analysis resolves the new taxon to within a paraphyletic assemblage of ‘osteolepidid-grade’ taxa although an unusual combination of characters makes its immediate relationships unclear. It is notable for having greatly enlarged spiracular openings, a feature present also in Gogonasus and the elpistostegalians. This character, suggestive of spiracular air-breathing, thus appeared independently in at least three lineages of Middle–Late Devonian tetrapodomorphs.
AUTHOR CONTRIBUTIONS
BC and TH designed the project, collated the manuscript, and created the figures. GY made the initial fossil discoveries and specimen documentation. AMC, BC, BK, GY, and JAL conducted fieldwork, including the recovery of the holotype specimen. BC, BK, TH, and JAL contributed to the anatomical description while AMC, BC, JAL, and TC conducted the phylogenetic analysis. BC and JAL analyzed the results of the analysis and wrote the discussion.
SUPPLEMENTARY FILES
Supplemental File 1.docx: appendices S1–S3, including character descriptions and codings (S1), coding modifications (S2) and phylogenetic results (S3).
Supplemental File 2.nex: character-by-taxon matrix used in the phylogenetic analysis.
Supplemental File 3.txt: data logs detailing scripts and workflow used for running the analysis.
Supplemental File 4.tre: tree files generated by the phylogenetic analysis.
Supplemental Material
Download Zip (84.9 KB)ACKNOWLEDGMENTS
J. Laurie provided access to material at Geosciences Australia while T. Ziegler did likewise for Museums Victoria specimens. L. Gibson assisted with photography at Melbourne Museum. C. Burke assisted preparation of the holotype at Flinders University, while the late D. Pickering oversaw preparation of additional material at Melbourne Museum. J. Gorter, J. McPherson, C. Thun, J. Lu, S. Arman, and others assisted during the 2016 field trip which acquired the holotype specimen. T. Senden and A. Sheppard provided access to facilities in the Research School of Physics and Department of Applied Mathematics, Australian National University, Canberra. M. Lee provided assistance with the phylogenetic analysis. The editors and reviewers provided invaluable feedback which greatly improved the final manuscript from the initial submission. This work was supported by the Australian Research Council via DECRA project DE1610024, and Discovery Grants DP0558499, DP0772138, DP160102460, and DP22100825.
DISCLOSURE STATEMENT
No potential conflict of interest was reported by the author(s).
LITERATURE CITED
- Ahlberg, P. E. (1991). A re-examination of sarcopterygian interrelationships, with special reference to the Porolepiformes. Zoological Journal of the Linnean Society, 103, 241–287.
- Ahlberg, P. E. (2018). Follow the footprints and mind the gaps: a new look at the origin of tetrapods. Earth and Environmental Science Transactions of the Royal Society of Edinburgh, 109(1–2), 115–137. https://doi.org/10.1017/S1755691018000695.
- Ahlberg, P. E., & Johanson, Z. (1997). The second tristichopterid (Sarcopterygii, Osteolepiformes) from the Upper Devonian of Canowindra, New South Wales, Australia. Journal of Vertebrate Paleontology, 17, 653–673.
- Ahlberg, P. E., & Johanson, Z. (1998). Osteolepiforms and the ancestry of tetrapods. Nature, 395, 792–794.
- Andrews, S. M. (1985). Rhizodont crossopterygian fish from the Dinantian of Foulden, Berwickshire, Scotland, with a re-evaluation of this group. Transactions of the Royal Society of Edinburgh, Earth Sciences, 76, 67–95.
- Andrews, S, M., & Westoll, T. S. (1970). The postcranial skeleton of Rhipidistian fishes excluding Eusthenopteron. Transactions of the Royal Society of Edinburgh, 68, 391–489.
- Barrell, J. (1916). Influence of Silurian-Devonian climates on the rise of air-breathing vertebrates. Bulletin of the Geological Society of America, 27, 387–436.
- Berner, R. A. (2006). GEOCARBSULF: A combined model for Phanerozoic atmospheric O2 and CO2, Geochimica et Cosmochimica Acta, 70, 5653–5664.
- Berner, R. A. (2009). Phanerozoic atmospheric oxygen: new results using the geocarbsulf model. American Journal of Science, 309, 603–606.
- Berner, R. A., & Canfield, D. E. (1989). A new model for atmospheric oxygen over Phanerozoic time. American Journal of Science, 289, 333–361.
- Berner, R. A., Vandenbrooks, J. M., & Ward, P. D. (2007). Oxygen and evolution. Science, 316, 557–558.
- Brazeau, M. D., & Ahlberg, P. E. 2006. Tetrapod-like middle ear architecture in a Devonian fish. Nature, 439, 318–321.
- Burrow, C. J., & Turner, S. (2012). Fossil fish taphonomy and the contribution of microfossils to documenting Devonian vertebrate history. In J. A. Talent (Ed.), Earth and Life: Global biodiversity, extinction intervals and biogeographic perturbations through time (pp. 189–223). Springer’s Legacy Series, International Year of Planet Earth, Springer Science + Business Media B.V. https://doi.org/10.1007/978-90-481-3428-1_8
- Cannell, A., Blamey, N., Brand, U., Escapa, I. & Large, R. (2022). A revised sedimentary pyrite proxy for atmospheric oxygen in the Paleozoic: Evaluation for the Silurian-Devonian-Carboniferous period and the relationship of the results to the observed biosphere record. Earth-Science Reviews, 231, 104062. https://doi.org/10.1016/j.earscirev.2022.104062
- Chang, M. M., & Zhu, M. (1993). A new Middle Devonian osteolepidid from Qujing, Yunnan. Memoirs of the Association of Australasian Palaeontologists, 15, 183–198.
- Choo, B., Lu. J., Giles, S., Trinajstic, K. & Long, J. A. (2019). A new actinopterygian from the Late Devonian Gogo Formation, Western Australia. Papers in Palaeontology, 5(2), 343–363. https://doi.org/10.1002/spp2.1243
- Clack, J. A. (2007). Devonian climate change, breathing, and the origin of the tetrapod stem group. Integrative and Comparative Biology, 47, 510–523.
- Clack, J. A. (2012). Gaining Ground: The Origin and Evolution of Tetrapods. Bloomington U.S.A.: Indiana University Press.
- Clement, A. M. (2009). A new genus of lungfish from the Givetian (Middle Devonian) of central Australia. Acta Palaeontologica Polonica, 54, 615–626.
- Clement, A. M. & Long, J. A. (2010). Air-breathing adaptation in a marine Devonian lungfish. Biology Letters, 6, 509–512.
- Clement, A. M., Long. J. A., Tafforeau, P., & Ahlberg, P. E. (2016). The dipnoan buccal pump reconstructed in 3D and implications for air breathing in Devonian lungfishes. Paleobiology, 42, 289–304.
- Clement, A. M., Cloutier, R., Lu, J., Perilli, E., Maksimenko, A., & Long, J. A. (2021). A fresh look at Cladarosymblema narrienense, a tetrapodomorph fish (Sarcopterygii: Megalichthyidae) from the Carboniferous of Australia, illuminated via X-ray tomography. Peer J, 9, e12597. https://doi.org/10.7717/peerj.12597
- Cloutier, R., Clement, A. M., Lee, M. S. Y., Noël, R., Béchard, I., Roy, V., & Long, J. A. (2020). Elpistostege and the origin of the vertebrate hand. Nature, 579, 549–554. https://doi.org/10.1038/s41586-020-2100-8
- Daeschler, E. B., Shubin, N. H. & Jenkins, F. A. (2006). A Devonian tetrapod-like fish and the evolution of the tetrapod body plan. Nature, 440, 757–763.
- Fox, R. C., Campbell, K. S. W., Barwick, R. E., & Long, J. A. (1995). A new osteolepiform fish from the Lower Carboniferous Raymond Formation, Drummond Basin, Queensland. Memoirs of the Queensland Museum, 38, 97–221.
- Graham, J. B., Wegner, N. C., Miller, L. A., Jew, C. J., Chin Lai, N., Berquist, R. M. L., Frank, L. R., & Long, J. A. (2014). Spiracular air breathing in polypterid fishes and its implications for aerial respiration in stem tetrapods. Nature Communications, 5, 6. https://doi.org/10.1038/ncomms4022
- Graham J. B., Jew, C. J., & Wegner, N. C. (2016). Modeling variable Phanerozoic oxygen effects on physiology and evolution. In R. C. Roach, P. H. Hackett, and P. D. Wagner (Eds.), Hypoxia: Translation in Progress (pp. 406–426), Springer US, New York.
- He, R., Lu, W., Junium, C. K., Van Straeten, C. A., & Lu, Z. (2019). Paleo-redox content of the Mid-Devonian Appalachian Basin and its relevance to biocrises. Geochimica et Cosmochimica Acta, 287, 328–340. https://doi.org/10.1016/j.gca.2019.12.019
- Holland, T. J., Warren, A., Johanson., Z., Long. J., Parker, K., & Garvey, J. (2007). A new species of Barameda (Rhizodontida) and heterochrony in the rhizodontid pectoral fin. Journal of Vertebrate Paleontology, 27, 295–315.
- Holland, T. J., & Long, J. A. (2009). On the phylogenetic position of Gogonasus andrewsae Long 1985, within the Tetrapodomorpha. Acta Zoologica, 90, 285–296.
- Holland, T., Long, J., & Snitting, D. (2010). New information on the enigmatic tetrapodomorph fish Marsdenichthys longioccipitus (Long, 1985). Journal of Vertebrate Paleontology, 30, 68–77.
- Hunt, J. R., & Young, G. C. (2012). Depositional environment, stratigraphy, structure, and palaeobiology of the Hatchery Creek Group (Early–?Middle Devonian) near Wee Jasper, New South Wales. Australian Journal of Earth Sciences, 59, 355–371.
- Huxley, T. H. (1880). On the application of the laws of evolution to the arrangement of the Vertebrata, and more particularly of the Mammalia. Proceedings of the Zoological Society, London, 43, 649–662.
- Janvier P., Clément, G., & Cloutier, R. (2007). A primitive megalichthyid fish (Sarcopterygii,Tetrapodomorpha) from the Upper Devonian of Turkey and its biogeographical implications. Geodiversitas, 29, 249–268.
- Jarvik, E. (1944). On the exoskeletal shoulder-girdle of teleostomian fishes, with special reference to Eusthenopteron foordi Whiteaves. Kungliga Svenska Vetenskapsakademiens Handlingar, 21, 1–32.
- Jarvik, E. (1950). On some osteolepiform crossopterygians from the Upper Old Red Sandstone of Scotland. Kunglinga svenska Vetenskapsakademiens Handlingar, 2, 1–35.
- Jarvik, E. (1952). On the fish-like tail in the ichthyostegid stegocephalians with descriptions of a new stegocephalian and a new crossopterygian from the Upper Devonian of East Greenland. Meddelelser om Grønland, 114, 1–90.
- Jarvik, E. (1966). Remarks on the structure of the snout in Megalichthys and certain other rhipidistid crossopterygians. Arkiv för zoology 19, 41–98.
- Jarvik, E. (1980). Basic Structure and Evolution of Vertebrates. Vols 1, 2. Academic Press, London.
- Jarvik, E. (1996). The Devonian tetrapod Ichthyostega. Fossils and Strata, 40, 1–213.
- Jessen, H.-J. (1973). Weitere Fischreste aus dem Oberen Plattenkalk der Bergisch-Gladbach-Paffrather Mulde (Oberdevon, Rheinisches Schiefergebirge). Palaeontographica Abteilung A-palaozoologie-stratigraphie, 143, 159–187.
- Johanson, Z., & Ahlberg, P. E. (1997). New tristichopterid (Osteolepiformes; Sarcopterygii) from the Mandagery Sandstone (Famennian) near Canowindra, N.S.W., Australia. Transactions of the Royal Society of Edinburgh, 88, 39–53.
- Johanson, Z., & Ahlberg, P. E. (1998). A complete primitive rhizodont from Australia. Nature, 394, 569–573.
- Johanson, Z., & Ahlberg, P. E. (2001). Devonian rhizodontids and tristichopterids (Sarcopterygii; Tetrapodomorpha) from East Gondwana. Transactions of the Royal Society of Edinburgh: Earth Sciences, 92, 43–74.
- Jones, B. G. (1972). Upper Devonian to lower Carboniferous stratigraphy of the Pertnjara Group, Amadeus Basin, central Australia. Journal of the Geological Society of Australia, 19, 229–249.
- Jones, B. G. (1991). Fluvial and lacustrine facies in the Middle to Late Devonian Pertnjara Group, Amadeus Basin, Northern Territory, and their relationship to tectonic events and climate. In R. J. Korsch and J. M. Kennard (Eds.) Geological and Geophysical studies in the Amadeus Basin, Central Australia. BMR Bulletin 236 (pp. 333–348). Bureau of Mineral Resources, Canberra.
- Krause, A. J., Mills, B. J., Zhang, S., Planavsky, N. J., Lenton, T. M. P., & Poulton, S. W. (2018). Stepwise oxygenation of the Paleozoic atmosphere. Nature Communications, 9, 1–10. https://doi.org/10.1038/s41467-018-06383-y
- Lebedev, O. A. (1995). Morphology of a new osteolepidid fish from Russia. Bulletin du Museum National d’histoire Naturelle. 4e Serie. Section C. Sciences de la Terre. Paleontologie, Geologie, Mineralogie, 17, 287−341.
- Long, J. A. (1985a). The structure and relationships of a new osteolepiform fish from the Late Devonian of Victoria, Australia. Alcheringa, 9, 1–22.
- Long, J. A. (1985b). New information on the head and shoulder girdle of Canowindra grossi Thomson from the Upper Devonian Mandagery Sandstone, New South Wales. Records of the Australian Museum, 37, 91–99.
- Long, J. A. (1987). An unusual osteolepiform fish from the Late Devonian of Victoria, Australia. Paleontology, 30, 839–852.
- Long, J. A. (1993). Cranial ribs in Devonian lungfishes and the origin of dipnoan air-breathing. Memoirs of the Association of Australasian Palaeontologists, 15, 199–209.
- Long, J. A., Barwick, R. E., & Campbell, K. S. W. (1997). Osteology and functional morphology of the osteolepiform fish Gogonasus andrewsae Long 1985, from the Upper Devonian Gogo Formation, Western Australia. Records of the Western Australian Museum Supplement, 53, 1–89.
- Long, J. A., & Ahlberg, P. E. (1999). New observations on the snouts of rhizodont fishes (Palaeozoic Sarcopterygi). Records of the Western Australian Museum Supplement, 57, 169–173.
- Long, J. A., Young, G. C., Holland, T., Senden, T. J., & Fitzgerald, E. M. G. (2006). An exceptional Devonian fish from Australia sheds light on tetrapod origins. Nature, 444, 199–202.
- Long, J. A., & Trinajstic, K. (2010). The Late Devonian Gogo Formation Lägerstatte of Western Australia: Exceptional Early Vertebrate Preservation and Diversity. Annual Review of Earth and Planetary Sciences, 38, 255–279.
- Long, J. A., & Trinajstic, K. (2017). A review of recent discoveries of exceptionally preserved fossil fishes from the Gogo sites (Late Devonian, Western Australia). Earth and Environmental Science Transactions of the Royal Society of Edinburgh, 108(1), 111–117. https://doi.org/10.1017/S1755691018000178
- Lu, J., Zhu, M., Long, J. A., Zhao, W., Senden, T. J., Jia L. T., & Qiao, T. (2012). The earliest known stem-tetrapod from the Lower Devonian of China. Nature Communications, 3, 1160. https://doi.org/10.1038/ncomms2170
- MacIver, M. A., Schmitz, L., Mugan, U., Murphey, T. D., & Mobley, C. D. (2017). A massive increase in visual range preceded the origin of terrestrial vertebrates. Proceedings of the National Academy of Sciences U.S.A., 114(12), E2375-E2384. https://doi.org/10.1073/pnas.1615563114
- Miall, L. C. (1885). Description of the Remains of Megalichthys in the Leeds Museum. Leeds Philosophical and Literary Society. 16pp.
- Romano, C., Koot, M. A., Kogan, I., Brayard, A., Minikh, A. V., Brinkmann, W., Bucher, H., & Kriwet, J. (2014). Permian–Triassic Osteichthyes (bony fishes): diversity dynamics and body size evolution. Biological Reviews, 91, 106–147. https://doi.org/10.1111/brv.12161
- Romer, A. S. (1955). Herpetichthyes, Amphibioidei, Choanichthyes or Sarcopterygii? Nature, 176, 126.
- Schultze, H.-P., & Heidtke, U. (1986). Rhizodopside Rhipidistia (Pisces) aus dem Perm der Pfalz (W-Deutschland). Neues Jahrbuch für Geologie und Paläontologie – Monatshefte, 3, 165–170.
- Schultze, H.-P., & Chorn, J. (1998). Sarcopterygian and other fishes from the marine Upper Devonian of Colorado, U.S.A. Mitteilungen aus dem Museum für Naturkunde zu Berlin, Geowissenschaftliche Reihe, 1, 53–72.
- Schultze, H.-P., & Reed, W. (2012). A tristichopterid sarcopterygian fish from the upper Middle Devonian of Nevada. Historical Biology, 24, 425–440.
- Scott, A. C., & Glasspool, I. J. (2006). The diversification of Paleozoic fire systems and fluctuations in atmospheric oxygen concentration. Proceedings of the National Academy of Sciences U.S.A., 103, 10861–10865.
- Swartz, B. (2012). A marine stem-tetrapod from the Devonian of western North America. PLoS ONE, 7, e33683. https://doi.org/10.1371/journal.pone.0033683
- Thomson, K. S. (1964). Revised generic diagnosis of the fossil fishes Megalichthys and Ectosteothachius (family Osteolepidae). Bulletin of the Museum of Comparative Zoology, 131, 283–311.
- Thomson, K. S. (1965). Gyroptychius (Rhipidistia, Osteolepidae) from the Middle Devonian of Scotland. Annals and Magazine of Natural History, 7, 725–732.
- Thomson, K. S. (1973). Observations on a new rhipidistian fish from the Upper Devonian of Australia. Palaeontographica A, 143, 209–220.
- Traquair, R. H. (1881). On the cranial osteology of Rhizodopsis. Transaction of the Royal Society of Edinburgh, 30, 167–179.
- Vorobyeva, E. I. (1977). Morphology and nature of evolution of crossopterygian fishes. Trudy Paleontologischeskogo Instituta Akademia Nauk SSSR, 163, 1–239.
- Vorobyeva, E. I. (1980). Observations on two rhipidistian fishes from the Upper Devonian of Lode, Latvia. Zoological Journal of the Linnean Society, 70, 191–201.
- Young, B., Dunstone, R. L., Senden, T. J., & Young, G. C. (2013). A gigantic sarcopterygian (tetrapodomorph lobe-finned fish) from the Upper Devonian of Gondwana (Eden, New South Wales, Australia). PLoS ONE, 8, e53871. https://doi.org/10.1371/journal.pone.0053871
- Young, G. C. (1985). New discoveries of Devonian vertebrates from the Amadeus Basin, central Australia. BMR Journal of Australian Geology and Geophysics, 9, 239–254.
- Young, G. C. (1988). New occurrences of phyllolepid placoderms from the Devonian of central Australia. BMR Journal of Australian Geology and Geophysics, 10, 363–376.
- Young, G. C. (1991). Palaeozoic vertebrates. In R. K. Korsch & J. M. Kennard (Eds.). Geological and Geophysical studies in the Amadeus Basin, Central Australia. BMR Bulletin 236 (pp. 106–107). Bulletin of Mineral Resources, Canberra.
- Young, G. C. (2005). An articulated phyllolepid fish (Placodermi) from the Devonian of central Australia: implications for non-marine connections with the Old Red Sandstone continent. Geological Magazine, 142, 173–186.
- Young, G. C. (2008). Relationships of tristichopterids (osteolepiform lobe-finned fishes) from the Middle-Late Devonian of East Gondwana. Alcheringa, 32, 321–336.
- Young, G. C., & Gorter, J. D. (1981). A new fish fauna of Middle Devonian age from the Taemas/Wee Jasper region of New South Wales. Bureau of Mineral Resources Bulletin, 209, 83–147.
- Young, G. C., & Goujet., D. (2003). Devonian Fish Remains from the Dulcie Sandstone and Cravens Peak Beds, Georgina Basin, Central Australia. Records of the Western Australian Museum, 65, 1–85.
- Young, G. C., & Lu., J. (2020). Asia-Gondwana connections indicated by Devonian fishes from Australia: palaeogeographic considerations. Journal of Palaeogeography, 9(1), 8. https://doi.org/10.1186/s42501-020-00057-x
- Young, G. C., & Schultze, H.-P. (2005). New osteichthyans (bony fishes) from the Devonian of Central Australia. Mitteilungen aus dem Museum für Naturkunde in Berlin - Geowissenschaftliche Reihe, 8, 13–35.
- Young, G. C., & Turner, S. (2000). Devonian microvertebrates and marine-nonmarine correlation in East Gondwana: Overview. Courier Forschungsinstitut Senckenberg, 223, 453–470.
- Young, G. C., Long, J. A., & Turner, S. (1993). Faunal lists of Eastern Gondwana Devonian macrovertebrate assemblages. In Long, J.A. (Ed.) Palaeozoic Vertebrate Biostratigraphy and Biogeography (pp. 248–251). Belhaven Press, London.
- Young, G. C., Long, J. A., & Ritchie, A. (1992). Crossopterygian fishes from the Devonian of Antarctica: systematic, relationships and biogeographic significance. Records of the Australian Museum, 14, 1–77.
- Young, G. C., Burrow, C., Long, J. A., Turner, S., & Choo, B. (2010). Devonian macrovertebrate assemblages and biogeography of East Gondwana (Australasia, Antarctica). Palaeoworld, 19, 55–74. https://doi.org/10.1016/j.palwor.2009.11.005
- Young, G. C., Dunstone, R. L., Ollerenshaw, P. J., Lu, J., & Crook., B. (2019). New information on the giant Devonian lobe-finned fish Edenopteron from the New South Wales south coast. Australian Journal of Earth Sciences, 67(2), 221–242. https://doi.org/10.1080/08120099.2019.1651769
- Zhu, M., & Ahlberg, P. E. (2004). The origin of the internal nostril of tetrapods. Nature, 432, 94–97.
- Zhu, M., Ahlberg, P. E., Zhao, W-J., & Jia, L-T. (2017). A Devonian tetrapod-like fish reveals substantial parallelism in stem tetrapod evolution. Nature Ecology and Evolution, 1(10), 1470–1476. https://doi.org/10.1038/s41559-017-0293-5