INTRODUCTION
Background of Bone Histomorphology in Modern Biology and Paleontology
Bone microstructure (i.e., histomorphology) reflects developmental history, ecological behaviors, and physiological mechanisms in the vertebrates. Since Mantel (Citation1850a, Citationb) first found well-preserved microstructure in fossil bones, many paleontologists have studied bone histomorphology and its relationship to various ecological behaviors in both extant and extinct vertebrates (e.g., Bailleul et al., Citation2019; Cook et al., Citation1962; Enlow & Brown, Citation1957; Erickson et al., Citation2004; Kolb et al., Citation2015a; Nopcsa, Citation1933; Nopcsa & Heidsieck, Citation1934; Pawlicki et al., Citation1966; Woodward, Citation2019; Woodward et al., Citation2014). Histomorphology provides information that cannot be obtained from external skeletal morphology (Bailleul et al., Citation2019; Kolb et al., Citation2015a; Quekett, Citation1849; Wang et al., Citation2020), such as ecological niches (Hayashi et al., Citation2013), sex differences via medullary bone (Bloom et al., Citation1941; Schweitzer et al., Citation2005, Citation2007; Wang et al., Citation2020), ontogenetic growth patterns (Erickson et al., Citation2004; Woodward, Citation2019; Woodward et al., Citation2014), pathological healing (Ekhtiari et al., Citation2020), and stress distribution in bones caused by animal locomotion (Bromage et al., Citation2003; de Margerie et al., Citation2004; Suniaga et al., Citation2018). Therefore, bone histomorphology has been emphasized not only in paleontological, but also in extant biological, pathological, and veterinary medical research (Musumci, Citation2014).
Paleo-histomorphological studies, which examine fossil bones of extinct taxa, often focus on millimeter-scale structures such as cortical bone thickness (e.g., Gray et al., Citation2007; Hayashi et al., Citation2013; Taylor, Citation2000), the sub-millimeter scale for trabecular distribution in epiphyses (e.g., Bishop et al., Citation2018a, Citationb, Citationc), and the tenth-millimeter scale for lines of arrested growth (LAGs: e.g., Chabreck & Joanen, Citation1979; Curry, Citation1990; Erickson et al., Citation2004; Mitchell et al., Citation2017; Woodward, Citation2019; Woodward et al., Citation2014). To apply these histological studies to extinct taxa, many paleontologists have also examined the significance of bone histomorphology in extant taxa, including not only humans and domestic animals but also wild animals and captive animals at zoos. However, most studies on the modern bones of wild animals have primarily focused on the millimeter- to tenth-millimeter scale structures, as well as those of fossil bones.
In contrast to paleohistological studies, more micrometer to nanometer scale structures have been studied in medical studies using modern bones of humans and domestic animals (e.g., Carnelli et al., Citation2013; Skedros et al., Citation1994; Suniaga et al., Citation2018). For example, morphology and the density of osteons, which may relate to mechanical loading and body size, are the scale at which most studies have been conducted (Felder et al., Citation2017; Zedda & Babosová, Citation2021; Zedda et al., Citation2008), at a resolution of 10–500 μm (Wegst et al., Citation2014). The axial orientation of collagen and hydroxyapatite correlates with the compressive and tensile stress in bones and can be observed using Raman spectroscopy and linearly and circularly polarized light microscopy, at a resolution of 3–10 μm (Boyde & Riggs, Citation1990; Bromage et al., Citation2003; Schrof et al., Citation2014). Bone mineralization density, which relates to locomotor activity, can be measured using X-ray analytical methods, such as X-ray microtomography (Davis & Wong, Citation1996; Suniaga et al., Citation2018). If these methods are applied to paleohistological studies and the examination of bones from wild animals, it provides a necessary framework for studying the physiological mechanisms and ecological behaviors of extinct taxa. This allows us to improve our understanding of the physiological and functional aspects, as well as the diversity of bone microstructures. For this reason, as well as to get the best performance from the equipment used for observation, high quality thin sections are required in modern bones for providing fundamental information to study histomorphological significance in extinct taxa.
A common process involved in preparing thin sections of fossil bones is to embed them in plastic prior to sectioning. In the 1950s, Canada balsam resin was used for mounting the samples (Enlow, Citation1954). Later, Parlodion (purified pyroxylin) solution with butyl acetate or amyl acetate was used for embedding the bone samples to stabilize and support the sample during sectioning (Enlow, Citation1954). Later, Chinsamy and Raath (Citation1992) introduced another method for making thin sections, which involves embedding samples with epoxy resin, followed by grinding with carbide abrasive powder and waterproof abrasive paper. Until today, the thin sectioning methods developed for fossil bones have been widely adopted by paleontologists for both fossil (e.g., Amson et al., Citation2014; Assis et al., Citation2015; Fernández-Jalvo et al., Citation2010; Gray et al., Citation2007; Heck et al., Citation2020; Lamm, Citation2013; Orlandi-Oliveras et al., Citation2018; Stein & Sander, Citation2009; Straehl et al., Citation2013; Wolf et al., Citation2012) and dried modern bones (e.g., Atterholt & Woodward, Citation2021; Heck et al., Citation2020; Legendre & Botha-Brink, Citation2018; Meier et al., Citation2013; Montoya-Sanhueza & Chinsamy, Citation2017; Montoya-Sanhueza et al., Citation2020; Nacarino-Meneses et al., Citation2016; Straehl et al., Citation2013; Tajiri & Fujita, Citation2013; Urano et al., Citation2019), although some studies have employed another resin for embedding (e.g., polyester: Heck et al., Citation2020; Sander, Citation2000) and abrasive paper for grinding (Lamm, Citation2013).
Conventional Thin Sectioning Method
The conventional method for the comparison with the modified method (see Protocols/Methodology) followed Urano et al. (Citation2019), which is employed for thin-sectioning modern hard tissues, and partly on the approach presented by Chinsamy and Raath (Citation1992), which is employed for both modern and fossil bones. In this study, the thickness of sections was adjusted to 100 μm to facilitate observation of bone microstructure, especially collagen fiber orientation (Boyde & Riggs, Citation1990).
Small holes were drilled through the bone cortex to allow the epoxy resin to penetrate the marrow cavity. In cases where the bone samples were too large to embed, they were cut into a few centimeters in length using a fret saw. The bones were placed in acetone for 5 h to dehydrate and degrease them. The samples were allowed to dry in air and then embedded in epoxy resin (Devcon ET, ITW Co.). The bubbles in the resin were removed as much as possible prior to polymerization using a vacuum pump (VE-ALL, As One Co.). The resin was heat-polymerized in a 40°C incubator (PIC-100, As One Co.) for 48 h. The block of polymerized resin embedding the bone sample (hereafter, the block) was trimmed and cut into transverse sections using a low speed cutting machine (RCA-005 Refine Saw, Lo, Refine Tec Ltd). The section plane of the sample (hereinafter, primary plane) was ground with silicon carbide (#800, #1000, and #1500) and polished with alumina abrasive powder (#3000). The sample was cleaned using an ultrasonic cleaner (SWT710, Citizen Co.) for 1 min to remove the particles of bone and/or abrasive powder prior to the sample being ground and/or polished with the next grade. The primary plane was bound to a glass slide with an epoxy bond (Konishi Bond E Set, Konishi Co.) and fixed with clips for several days at room temperature. After solidification of the epoxy bond, the block was secondarily cut into sections of 200 μm thickness. The exposed section plane, as well as the primary plane, was ground and polished to obtain a final section thickness of 100 μm. Ultraviolet (UV) curable acrylate resin cured by UV light for 30 s was used to hold the coverslip.
Problems in Observation of Bone Histomorphology
We encountered three problems when observing the collagen fiber orientation in thin sections of extant taxa, which were prepared using the abovementioned method (modified from Chinsamy & Raath [Citation1992] and Urano et al. [Citation2019]).
Opaque Patches in Thin Sections—The first concern was a reddish-brown post-mortem stain retained in the thin section. This stain was found in many but not all of the samples from the several mammalian orders which we studied—see, for examples, the thin sections of Cape hyrax (Procavia capensis) tibia and fibula () and lesser Japanese mole (Mogera imaizumii) humerus (). This stain does not transmit light, and thus obstructs the observation of lamellae, osteons, and osteocyte lacunae in transmitted light microscopy. As these areas are opaque, they cannot be studied for collagen fiber orientation by polarized light microscopy, which requires completely transparent sections (Boyde & Riggs, Citation1990). We could not remove the stain with acetone, which rejected the possibility that it is due to a lipid that obstructs transmitted light (Weissleder, Citation2001: fig. 1b). In contrast, some samples did not contain the stain—see , which shows a section of raccoon (Procyon lotor) metacarpus that does not have the stain.
FIGURE 1. Transmitted light images of transverse thin sections of Cape hyrax (Procavia capensis) left tibia and fibula by a 35 mm film/slide scanner (OpticFilm 8100, Plustek Inc.). A, the section prepared with the method developed by Urano et al. (Citation2019); B, section soaked in acetone for 1 week; and C, section soaked in Quadrol for 1 week. The asterisk (*) indicates the heme pigment distributed in the bones. The directions of bones are above, anterior; right, lateral; left, medial; and bottom, posterior. Abbreviations: ep, epoxy resin; fb, fibula; LAG, line of arrested growth; and tb, tibia. All field widths equal 10.51 mm.
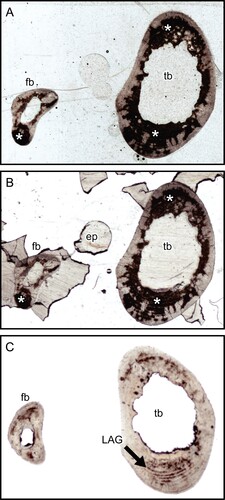
Birefringence of Epoxy Resin—The next concern was the birefringence of the epoxy resin, which was used for embedding samples and for bonding to the glass slides. Epoxy resin is inherently transparent. However, it develops a strain birefringence due to its deformation in the following stages of polymerization. This birefringence appears unevenly stronger on the thin section through the polarizing microscope than the natural birefringence of bone, which is the result of the negative intrinsic birefringence of hydroxyapatite crystals and the positive form birefringence of the oriented collagen in which the apatite is deposited (). Thus, this birefringence that disturbs polarized light prevents systematic study of the bone proper. Furthermore, the resin used in bonding bone to the glass slides may cause a problem with the distribution of microstructural observations (; see for comparison); for example, Araldite (García-Martínez et al., Citation2011; Marín-Moratalla et al., Citation2013; Nacarino-Meneses et al., Citation2016; Orlandi-Oliveras et al., Citation2018; Straehl et al., Citation2013; Wolf et al., Citation2012), EpoThin (Atterholt & Woodward, Citation2021; Schweizer et al., Citation2007), and Devcon (de Margerie et al., Citation2004; Urano et al., Citation2019) generate significant birefringence compared with polyester resin (Sander, Citation2000; Sawada et al., Citation2014; Stein et al., Citation2013).
FIGURE 2. Transverse thin section of lesser Japanese mole (Mogera imaizumii) left humerus prepared by the method of Urano et al. (Citation2019: see the heading Conventional Thin Sectioning Method). A, C, E, transmitted, and B, D, F, polarized light microscopic images. The asterisk (*) indicates the heme pigment distributed in the bone. The directions of bone are above, anterior; right, medial; left, lateral; and bottom, posterior. Abbreviation: ca, canal. The field widths equal 5.59 mm (A, B), 2.15 mm (C, D), and 845 μm (E, F).
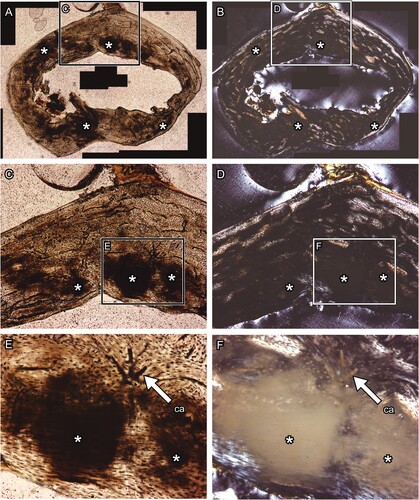
FIGURE 3. Transverse thin section of raccoon (Procyon lotor) left metacarpus produced with no Quadrol treatment. A and C, transmitted, and B and D, polarized light microscopic images. Panels C and D are magnified images in the frames on panels A and B, respectively. The directions of bone are above, anterior; right, lateral; left, medial; and bottom, posterior. The field widths equal 3.57 mm (A, B) and 2.15 mm (C, D).
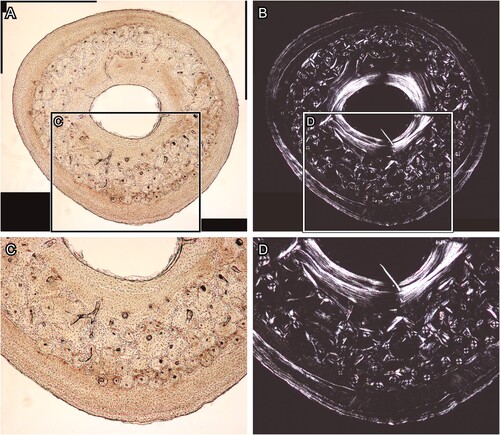
Abrasive Powder for Grinding and Polishing Section Surface—The last concern was the contamination with abrasive powder in the thin sections. Through backscattered electron mode scanning electron microscopy (BSE-SEM) observations, we found that the abrasive powder, which was used for both grinding to adjust sample thickness and polishing the section surface, was impacted into the section surfaces (). Furthermore, the abrasive powder is also difficult to polish smoothly, and a rough surface can be observed by BSE-SEM (). These features caused disturbance in observation of microstructures such as osteons, lacunae, and degree of mineralization under BSE-SEM (; see for comparison).
FIGURE 4. Transverse section of chicken (Gallus gallus) ulna, which was polished by silicon carbide and alumina abrasive powder. A and C, the BSE compositional images, and B and D, the BSE topographic images taken in the same area in the previous panels. Panels C and D are magnified images in the frames on panels A and B, respectively. Abbreviations: ab, abrasive powder; and ca, canal. The field widths equal 1.59 mm (A, B) and 706 μm (C, D).
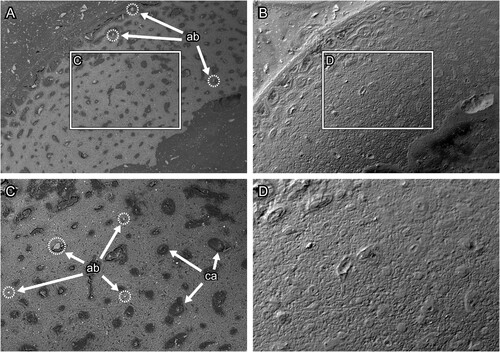
The above problems need to be solved. This study aimed to modify the bone thin sectioning method that facilitates detailed observation of histomorphological study.
PROTOCOLS AND METHODOLOGY
Materials and Equipment
Four mammalian and one avian limb bones (humerus, ulna, metacarpus, femur, tibia, and fibula), representing five species were used in this study: a nine-banded armadillo (Dasypus novemcinctus) that was road-killed; a Cape hyrax (Procavia capensis) that died at a zoo; a lesser Japanese mole (Mogera imaizumii) that had been stored as dried specimens in Y. Yokohata laboratory (University of Toyama, Toyama, Japan); a raccoon (Procyon lotor) that was exterminated as an invasive species in Japan; and a chicken (Gallus gallus) that was purchased commercially.
The materials, chemicals, and instruments used in testing the modified methods for resolving the problems due (1) opaque stain, (2) epoxy resin birefringence, and (3) contamination with abrasive powder are listed in . The thin sections prepared using the conventional method (see the heading Conventional Thin Sectioning Method) and the modified method proposed in this study (see the heading Modified Thin Sectioning Method) were compared.
TABLE 1. List of materials, chemicals, and instruments used for the modified thin-sectioning method proposed in this study. See Figure 5 for the procedure of the modified thin sectioning method.
Analytical Approach: Evaluation of the Quality of Thin Sections
A linearly polarizing microscope (Eclipse E200, Nikon Co.) was employed to evaluate the birefringence of bone mineral (i.e., orientation of collagen fibers and hydroxyapatite), as well as that of epoxy resin, and the distribution of opaque stain within bone sections. The histological images were photographed under both transmitted and polarizing light, with consistent illuminating settings maintained to facilitate a direct comparison of the visibility of histological structures across the sections.
BSE-SEM (S-3400N, Hitachi Ltd; EVO-10, Zeiss Co. Ltd) was employed to evaluate roughness and abrasive powder residues on the polished surface of thin sections prepared by two different methods (see the heading Conventional Thin Sectioning Method and Modified Thin Sectioning Method). BSE topographic images were taken for the epoxy-embedded thin sections, with each surface polished by abrasive powder or paper, respectively. For comparison, we examined the surfaces of thin sections that were (i) cut by the diamond saw, (ii) soaked in dichloromethane, (iii) embedded in epoxy resin and polished using abrasive paper, and (iv) treated with resin dissolution followed by polishing with abrasive powder, respectively (Figure S1, Supplementary File 1). Additionally, the BSE compositional images were taken to evaluate the abrasive powder residues on the surface.
Modified Thin Sectioning Method: Preparation of Bone Slice Samples
To solve the problems described in the Introduction (see the heading Problems in Observation of Bone Histomorphology), we refined the method for preparing thin sections (). The preparation order can be adjusted as appropriate. The bone sample was first cut in the transverse plane by a water-cooled diamond saw at a low speed of approximately 150 rpm without embedding in epoxy resin. For secondary cutting, the thickness of the slice was adjusted to 0.5–1 mm (hereinafter, the slice sample). After the process of removal of the stain (see the heading Removal of the Pigment), grinding, and polishing (see the heading Grinding and Polishing Sample Surfaces), the thin sections were mounted with DPX mounting medium (C18H18N2.2Br). During this process, care was taken to ensure that no air bubbles and dust were trapped with the sample. The mounted samples were placed in an oven at 45°C overnight.
FIGURE 5. Diagram of a modified method of thin sectioning preparation developed in this study. The dashed frames and arrows indicate the procedures if a bone sample is required to be embedded in epoxy resin.
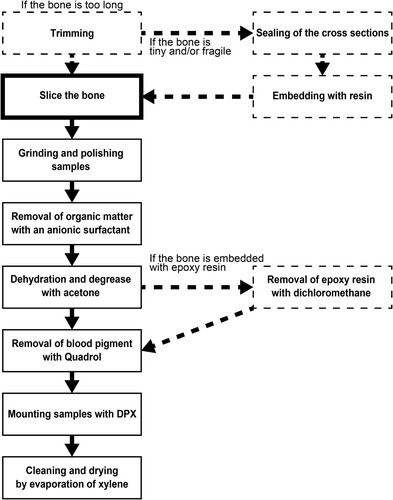
In cases where the bone sample was too tiny, slender, or fragile, it was difficult to cut out the slice sample without embedding in resin. Therefore, fragile bone samples were embedded with resin prior to cutting the slice sample, but the resin was removed in the post-processing (see the heading Removal of Epoxy Resin from the Samples). However, the penetration of the resin into the bone was prevented as much as possible. The cut section of the bone was sealed with tape prior to embedding in the resin, to avoid penetration of resin into the marrow cavity and canals of the bone. The possible organic matter that remained in the bone, such as bone marrow, was removed with an anionic surfactant (α-olefin sulfonate).
Removal of the Pigment
Since the stain in the sections was not removable by organic solvents, it was speculated that the stain is another biological pigment. The possible pigment contained in the cortex is hemoglobin and/or hemosiderin (heme), which is released from erythrocytes during post-mortem decay and degeneration. To remove the pigment, we used N,N,N′,N′-tetrakis (2-hydroxypropyl) ethylenediamine (Quadrol) in H2O solution (hereinafter, Quadrol). Quadrol is the main component that leaches heme from the tissue in the ‘Clear, Unobstructed Brain Imaging Cocktails and computational analysis (CUBIC)’ method for soft tissue transparency (Jing et al., Citation2018; Susaki et al., Citation2014; Tainaka et al., Citation2014). Urea and octyl phenyl, which constitutes the Scale1/Cubic reagent used for tissue transparency in the CUBIC method, were deemed unnecessary for hard tissue observation, as they are employed to prevent soft tissue cell expansion due to osmotic pressure and other factors.
Slice samples were put in a jar. Quadrol was added until the solution covered the entire slice samples. Thereafter, the jar was kept at room temperature for 1 week or more. Next, the bone slice samples were gently removed from the jar. They are prone to breaking due to the viscosity of the Quadrol. It is expected that the thinner the section is prepared, the more effective will be the removal of the stain. However, if there is concern of sample breakage, the Quadrol treatment can be done prior to polishing the sample to 100 μm in thickness. Thereafter, the samples were washed well with ethanol or methanol and xylene to remove the viscous Quadrol.
The Effect of Quadrol in Removing the Iron Pigment—Comparison of the images of the thin sections taken before and after immersing in Quadrol () showed that the reddish-brown stain patches were mostly removed by Quadrol although they may have persisted along the LAGs (). No decalcification or damage was observed in the sections. In histomorphological observation, bone lacunae and laminae were clearly observed in transmitted light (; see for comparison). Under polarized light, the section prepared using the modified method appears to be overall brighter than that prepared using the conventional method (; see for comparison). Moreover, the collagen fiber orientation was clearly observed in the section prepared in the modified method due to the removal of the pigment (; see for comparison).
FIGURE 6. Transverse thin section of lesser Japanese mole (Mogera imaizumii) left humerus prepared by the method developed in this study (see the heading Modified Thin Sectioning Method). A, C, E, transmitted and B, D, F, polarized light microscopic images. The directions of bone are above, anterior; right, medial; left, lateral; and bottom, posterior. Abbreviation: ca, canal. The field widths equal 4.18 mm (A, B), 2.15 mm (C, D), and 845 μm (E, F).
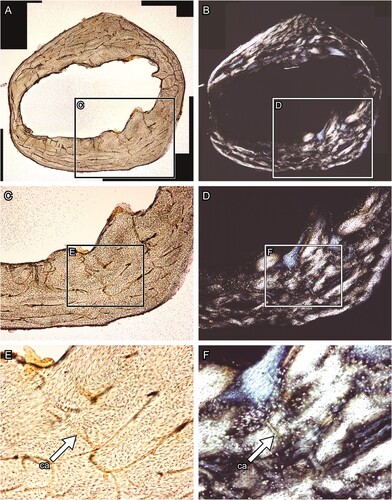
Removal of Epoxy Resin from the Samples
Chemical degradation of epoxy resin after curing is difficult, although some supercritical fluids have been used as solvents for epoxy resin (e.g., Dark et al., Citation1974; Jiang et al., Citation2010; Kuo et al., Citation2019; Li et al., Citation2019; Liu et al., Citation2012; Oliveux et al., Citation2015). However, these methods require supercritical test equipment and maintenance of high temperature and pressure, which is unsuitable for bone samples as they would be heated, decalcified, and degraded under such conditions. We used dichloromethane, which can degrade and dissolve epoxy resin at room temperature. Huan et al. (Citation2011) advocated the method of dissolving resin to extract metal parts, using chemical compounds including dichloromethane. However, the solution contains formic acid and sulphonic acid, which would dissolve bone samples. In this study, thus, we used only dichloromethane. This requires careful handling in a well-ventilated room or under a fume hood because it is volatile and harmful. However, since the solution is hydrophobic and denser than water, pouring additional water prevents the volatilization and waste of dichloromethane. Slices of 500 μm in thickness embedded in epoxy resin were placed in dichloromethane for 2 h. The samples were rinsed with water after dissolving the epoxy before further grinding and polishing.
A block in which the bone is surrounded by epoxy resin needs large amounts of dichloromethane and time to dissolve the epoxy resin. To spare dichloromethane, other methods of bone isolation from epoxy resin are required. Here, we used a chicken humerus embedded in 30 g of epoxy resin (). The embedded sample was soaked in acetone for 24 h to soften the resin. Next, the sample was placed in dichloromethane with water, for 24 h. While changing the solution, removable resin debris was dissected away before further treatment with dichloromethane and acetone. This process was repeated several times to remove the epoxy resin as much as possible.
FIGURE 7. A, Chicken (Gallus gallus) humerus embedded by epoxy resin (the block), which is used for removal of epoxy resin (see the heading Removal of Epoxy Resin from the Samples). The block has been soaked in acetone and dichloromethane for: B, one; C, two; and D, three cycles. All scale bars equal 3 cm.
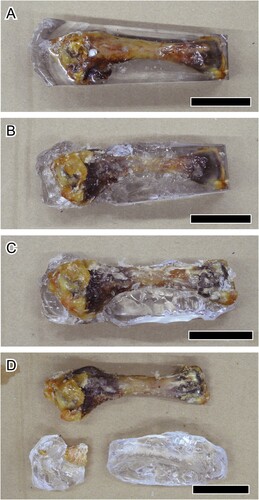
The Effect of Dichloromethane on Epoxy Resin Removal—The slice samples treated with dichloromethane were observed by a polarizing microscope (). Soaking in dichloromethane showed a faster removal of epoxy resin than with acetone. However, some epoxy resin in the sections remained in the narrow, intricate areas such as vessel canals and marrow cavities. Nevertheless, thin sections were undamaged in this method (Figure S1, Supplementary File 1).
In the block (), the epoxy resin was almost completely removed after three cycles of the method. A little epoxy resin remained on the periosteal surface of metaphysis and cartilage. The epoxy resin in and on the soft tissues was not removed even after the repeated cycles. Soft tissues such as cartilage were torn off when the epoxy resin was manually detached by hand. On the other hand, the bone was not broken despite being placed in acetone and dichloromethane for several cycles.
An Alternative to Epoxy Resin—For fragile bones, however, resin-embedding is the only method available so far to stabilize the sample during the cut. Instead of epoxy resin, we recommend (poly-) methyl methacrylate (PMMA or MMA), which has been used in sectioning modern bone for research. It has not yet been shown to be a problem for polarized light microscopy. MMA and PMMA also can take a fine polish or can be micro-milled to a flat surface, and have therefore been used extensively for BSE-SEM and correlative microscopic methods (Boyde, Citation1984, Citation2019).
Grinding and Polishing Sample Surfaces
We followed a part of the “Standard Thin-Sectioning Procedure” of Lamm (Citation2013) for grinding and polishing the sample surfaces. In this study, loose, unbound abrasive powder was never used, to avoid the impact of the powder onto the section surfaces.
The slice sample was ground and polished on both sides with a rotary grinding plate (the grinder: LaboPol-4, Struers Ltd; Beta Grinder-Polisher, Buehler Ltd) and waterproof silicon carbide papers (CarboMet, Buehler Ltd) from rough to fine (P1000, P2500, and P4000) until the slice thickness was adjusted to 100 μm, which was measured by the digital caliper (0–200 mm; accuracy, 0.01 mm; CD-20APX, Mitsutoyo Co. Ltd) and the micrometer (0–25 mm; accuracy, 0.001 mm; MDC-25MXT, Mitsutoyo Co. Ltd). Rubber gloves protect fingers and fix the slice sample while grinding and polishing. Finally, the polished slice sample was dehydrated and defatted in acetone for several hours.
Evaluation of Section Surfaces—The BSE-SEM images of section surfaces under the various conditions are provided in Supplementary File 1 (Figure S1, Supplementary File 1). The surface microstructure of thin sections polished with waterproof silicon carbide paper was more clearly observed than that of thin sections polished with abrasive powder (; see for comparison). In the thin section produced by the conventional method, the abrasive powder was impacted into the bone and epoxy resin (). The bone surface was roughly polished such that the microstructures such as the osteocyte lacunae and osteons could not be observed (). In the thin section produced by the modified method, on the other hand, the surface is smoothly polished and free from contamination with abrasive powder (). The modified method facilitates the observation of bone microstructures such as lacunae and osteons in the BSE compositional images (). The lacunae were arranged concentrically around the canal and the contrast made it possible to distinguish osteon units by the degree of mineralization. However, scratch marks caused by polishing were observed. On the other hand, the difference in the polishing methods could not be clearly distinguished in the images observed under the polarizing microscope (, ). These results support and recommend the grinding method proposed by Lamm (Citation2013).
FIGURE 8. Transverse section surface of chicken (Gallus gallus) ulna, which was polished by silicon carbide paper. A and C, the BSE compositional images and B and D, the BSE topographic images taken in the same area in the previous panels. Figures C and D are magnified images in the frames on figures A and B, respectively. Abbreviations: ca, canal; lc, lacuna; and os, osteon. The field widths equal 1.59 mm (A, B) and 706 μm (C, D).
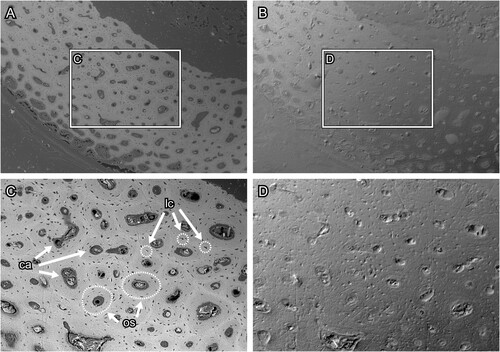
Pitfalls to Avoid: Limitations
Thin sectioning methods developed for fossil bones have been applied to modern bones in many histological studies. However, the material properties differ between modern and fossil bones. Consequently, it causes problems for observing more microstructures and ultrastructures in modern bones. This study modified the thin-sectioning preparation for modern bones of wild animals, where the blood pigment diffuses within the bone disturbing the histological observation. Therefore, the modified thin sectioning method is expected to advance the development of histological studies in modern bones of wild animals, which contribute to providing fundamental information to paleohistological studies with fossil bones.
Why Does the Pigment Deposit in the Modern Bone Tissue?—It is clear that the stain is blood-derived pigment and that Quadrol is an effective agent for its removal. To our knowledge, blood-derived pigment deposition in bone tissue has not been reported in the literature. In most prospective histological studies on bones of extant domestic animals and humans, the carcasses are frozen immediately after the death or the bones are removed from the carcass and fixed (for example, with neutral buffered formaldehyde) immediately after death. In contrast, many other histological studies use carcasses that have already died in the wild or in a zoo, and left for some time after death without freezing treatment. The thin section of the armadillo femur used in this study, which had certainly been dead for a matter of weeks before collection, had massive amounts of the presumed blood pigment in the untreated bones, and the thin section of the femur prepared in the conventional method appeared to be reddish-brown in color under macroscopic view (Figure S2, Supplementary File 1). Similarly, blood pigment appeared in the thin sections of Cape hyrax as well. The Cape hyrax used in this study had died at the zoo and was left at room temperature for at least 24 h after death until the pathological autopsy was carried out. During this time, therefore, the blood-derived pigment is expected to have diffused into the bone tissue quite soon after death. In contrast, such heme pigment was not observed in the racoon bone samples used in this study, which was frozen soon after its death for the extermination of invasive species in Japan (). Therefore, whether heme diffuses in the bone largely depends on the duration of the process from postmortem to cryopreservation or blood removal. Additionally, one of us has very frequently seen iron pigmentation in immature mammalian dental enamel where its color depends on the maturation cycle banding (Boyde, pers. comm.). Since there is no collagen in enamel, but abundant apatite, it may be that it binds to the mineral rather than the organic matrix.
Do Fossil Bones Retain the Heme Pigment?—Heme is an organic complex composed of ferrous and protoporphyrin proteins. Thus, the stain may be removed from bones during taphonomic processes and fossilization as any protein is supposedly degraded. However, some previous studies have shown that blood-like stains persist in fossil bone sections (Amson et al., Citation2015; Castanet, Citation2006; Kolb et al., Citation2015b; Köhler & Moyà-Solà, Citation2009; Moncunill-Solè et al., Citation2016; Nopcsa & Heidsieck, Citation1934; Sawada et al., Citation2014; Schweitzer et al., Citation1997). Note that these patches are possibly caused by not only the pigment but also air-bubbles and other factors. To identify whether the opaque patch is iron pigment, chemical elemental analysis is required. The Quadrol reagent used in this study works by eluting heme proteins by solubilizing them (Susaki et al., Citation2014). If the stain contains iron derived from heme, but which was degraded in the taphonomic process, it would not be expected to be removed by Quadrol. To analyze hydroxyapatite orientation and X-ray diffraction (XRD) in fossil bones that retain blood-like stains, additional methods to remove the stain from the fossil bone sections may be required.
CONCLUSIONS AND FUTURE DIRECTIONS
Compared with the conventional method, the modified method for preparing thin bone sections herein resolved the problems raised in the Introduction (see the heading Problems in Observation of Bone Histomorphology). Under a polarizing microscope, the bone microstructures were clearer, more transparent, and brighter than those in the section embedded and bounded with epoxy resin (, ). However, neither the heme pigment nor the impregnated epoxy resin was always completely removed from the thin sections by this method. Therefore, the number of solvents to be used and the soaking duration in each solvent needs to be optimized, as is found to be appropriate.
This modified method cannot be applied directly to fossil bones. Heme iron undergoes a transformation into an iron mineral during taphonomic processes; therefore, it cannot be removed by Quadrol treatment. The integrity of the resin in fossil bones depends on factors, including the sample size (Lamm, Citation2013), the thickness of the sections (Heck et al., Citation2020), and possibly the elasticity of the material and resin. In addition, abrasive material should be chosen case-by-case by adjusting to the hardness of the sample (i.e., mineral composition). Therefore, further examinations are required to address the removal of iron minerals within fossil bones and suitable resin for embedding fossils in terms of visibility of microstructures and ultrastructures.
AUTHOR CONTRIBUTIONS
DN launched the research project, prepared the specimens and chemicals used in this study, and thin sections, created figures, interpretation, and drafted the manuscript. AB raised and discussed the issues of conventional thin sectioning preparation, designed the solution, prepared the specimen, chemicals used in this study, and instruments for thin sectioning, and composed parts of the manuscript. Both authors edited the manuscript.
SUPPLEMENTARY FILES
Supplementary_File_1.docx: Figures S1 and S2
Supplemental Material
Download MS Word (1.8 MB)DISCLOSURE STATEMENT
No potential conflict of interest was reported by the author(s).
ACKNOWLEDGMENTS
We are grateful to S. Fujiwara (Nagoya University Museum, Aichi, Japan) for supervising the first author. S. Fujiwara, J. Hatase (Hiroshima City Asa Zoological Park, Hiroshima, Japan), K. Sone (Nagoya Biodiversity Center, Aichi, Japan), and Y. Yokohata (University of Toyama, Toyama, Japan) provided us the bone materials. K. Nakamura (Hokkaido University, Hokkaido, Japan) and Y. Koketsu (Nagoya University, Aichi, Japan) advised the method to make thin sections in paleontological and petrological studies. Y. Koketsu and D. Mills (Queen Mary University of London, London, UK) supported the equipment used in this study. We are grateful to A. Huttenlocker (University of Southern California, California, U.S.A.), H. N. Woodward (Oklahoma State University Center for Health Sciences, Oklahoma, U.S.A.), M. Whitney (Harvard Museum of Comparative Zoology, Harvard University, Massachusetts, U.S.A.), and an anonymous reviewer for the critical and beneficial comments as editors and reviewers. Japan Society for the Promotion of Science (JSPS) fellowship (grant no. 21J14927) supported this research.
DATA AVAILABILITY STATEMENT
The high-resolution images of thin sections used in this study are available on Morphobank: http://morphobank.org/permalink/?P4522.
LITERATURE CITED
- Amson, E., de Muizon, C., Laurin, M., Argot, C., & de Buffrénil, V. (2014). Gradual adaptation of bone structure to aquatic lifestyle in extinct sloths from Peru. Proceedings of the Royal Society B, 281(1782), 20140192. DOI: 10.1098/rspb.2014.0192.
- Amson, E., de Muizon, C., Domning, D. P., Argot, C. & de Buffrénil, V. (2015). Bone histology as a clue for resolving the puzzle of a dugong rib in the Pisco Formation, Peru. Journal of Vertebrate Paleontology, 35(3), e922981. DOI: 10.1080/02724634.2014.922981.
- Assis, S., Keenleyside, A., Santos, A. L., & Cardoso, F. A. (2015). Bone diagenesis and its implication for disease diagnosis: Relevance of bone microstructure analysis for the study of past human remains. Microscopy and Microanalysis, 21(4), 805–825. DOI: 10.1017/S1431927615000768.
- Atterholt, J. & Woodward, H. N. (2021). A histological survey of avian post-natal skeletal ontogeny. PeerJ, 9:e12160. DOI: 10.7717/peerj.12160.
- Bailleul, A. M., O’Connor, J., & Schweitzer, M. H. (2019). Dinosaur paleohistology: review, trends and new avenues of investigation. PeerJ, 7:e7764. DOI: 10.7717/peerj.7764.
- Bishop, P. J., Hocknull, S. A., Clemente, C. J., Hutchinson, J. R., Farke, A. A., Beck, B. R., Barrett, R. S., & Lloyd, D. G. (2018a). Cancellous bone and theropod dinosaur locomotion. Part I—an examination of cancellous bone architecture in the hindlimb bones of theropods. PeerJ, 6:e5778. DOI: 10.7717/peerj.5778.
- Bishop, P. J., Hocknull, S. A., Clemente, C. J., Hutchinson, J. R., Barrett, R. S., & Lloyd, D. G. (2018b). Cancellous bone and theropod dinosaur locomotion. Part II—a new approach to inferring posture and locomotor biomechanics in extinct tetrapod vertebrates. PeerJ, 6:e5779. DOI: 10.7717/peerj.5779.
- Bishop, P. J., Hocknull, S. A., Clemente, C. J., Hutchinson, J. R., Farke, A. A., Barrett, R. S., & Lloyd, D. G. (2018c). Cancellous bone and theropod dinosaur locomotion. Part III—Inferring posture and locomotor biomechanics in extinct theropods, and its evolution on the line to birds. PeerJ, 6:e5777. DOI: 10.7717/peerj.5777.
- Bloom, W., Bloom, M. A. & Mclean, F. C. (1941). Calcification and ossification. Medullary bone changes in the reproductive cycle of female pigeons. Anatomical Record, 81(4), 443–466. DOI: 10.1002/ar.1090810404.
- Boyde, A. (1984). Methodology of calcified tissue specimen preparation for SEM. In G. R. Dickson (Ed.), Methods of calcified tissue preparation (pp. 251–307). Elsevier.
- Boyde, A. (2019). Scanning electron microscopy of bone. In A. Idris (Ed.), Bone research protocols. Methods in molecular biology, 1914 (pp. 571–616). Humana Press. DOI: 10.1007/978-1-4939-8997-3_31.
- Boyde, A. & Riggs, C. M. (1990). The quantitative study of the orientation of collagen in compact bone slices. Bone, 11(1), 35–39. DOI: 10.1016/8756-3282(90)90069-b.
- Bromage, T. G., Goldman, H. M., McFarlin, S. C., Warshaw, J., Boyde, A., & Riggs, C. M. (2003). Circularly polarized light standards for investigations of collagen fiber orientation in bone. Anatomical Record (Part B: New Anat.), 274B(1), 157–168. DOI: 10.1002/ar.b.10031.
- Carnelli, D., Vena, P., Dao, M., Ortiz, C., & Contro, R. (2013). Orientation and size-dependent mechanical modulation within individual secondary osteons in cortical bone tissue. Journal of the Royal Society Interface, 10(81), 20120953. DOI: 10.1098/rsif.2012.0953.
- Castanet, J. (2006). Time recording in bone microstructures of endothermic animals; functional relationships. General Palaeontology, 5(3–4), 629–636. DOI: 10.1016/j.crpv.2005.10.006.
- Chabreck, R. H. & Joanen, T. (1979). Growth rates of American alligators in Louisiana. Herpetologica, 35(1), 51–57. DOI: 10.2307/1352272.
- Chinsamy, A. & Raath, M. A. (1992). Preparation of fossil bone for histological examination. Palaeontologica Africana, 29, 39–44.
- Cook, S. F., Brooks, S. T., & Ezra-Cohn, H. E. (1962). Histological studies on fossil bone. Journal of Paleontology, 36(3), 483–494.
- Curry, K. A. (1990). Ontogenetic histology of Apatosaurus (Dinosauria: Sauropoda): New insights on growth rates and longevity. Journal of Vertebrate Paleontology, 19(4), 654–665. DOI: 10.1080/02724634.1999.10011179.
- Dark, W. A., Conrad, E. C., & Crossman Jr., L. W. (1974). Liquid chromatographic analysis of epoxy resins. Journal of Chromatography, 91, 247–260. DOI: 10.1016/S0021-9673(01)97904-X.
- Davis, G. R. & Wong, F. S. (1996). X-ray microtomography of bones and teeth. Physiological Measurement, 17(3), 121–146. DOI: 10.1088/0967-3334/17/3/001.
- de Margerie, E., Robin, J-P., Verrier, D., Cubo, J., Groscolas, R., & Castanet, J. (2004). Assessing a relationship between bone microstructure and growth rate: a fluorescent labelling study in the king penguin chick (Aptenodytes patagonicus). Journal of Experimental Biology, 207(5), 869–879. DOI: 10.1242/jeb.00841.
- Ekhtiari, S., Chiba, K., Popovic, S., Crowther, R., Wohl, G., Wong, A. K. O., Tanke, D. H., Dufault, D. M., Geen, O. D., Parasu, N., Crowther, M. A., & Evans, D. C. (2020). First case of osteosarcoma in a dinosaur: a multimodal diagnosis. Lancet Oncology, 21(8), 1021–1022. DOI: 10.1016/S1470-2045(20)30171-6.
- Enlow, D. H. (1954). A plastic-seal method for mounting sections of ground bone. Stain Technology, 29(1), 21–22. DOI: 10.3109/10520295409115431.
- Enlow, D. H. & Brown, S. O. (1957). A comparative histological study of fossil and recent bone tissues. Part II. Texas Journal of Science, 9, 136–214.
- Erickson, G. M., Makovicky, P. J., Currie, P. J., Norell, M. A., Yerby, S. A., & Brochu, C. A. (2004). Gigantism and comparative life-history parameters of tyrannosaurid dinosaurs. Nature, 430, 772–775. DOI: 10.1038/nature02699.
- Felder, A. A., Phillips, C., Cornish, H., Cooke, M., Hutchinson, J. R., & Doube, M. (2017). Secondary osteons scale allometrically in mammalian humerus and femur. Royal Society Open Science, 4(11), 170431. DOI: 10.1098/rsos.170431.
- Fernández-Jalvo, Y., Andrews, P., Pesquero, D., Smith, C., Marín-Monfort, D., Sánchez, B., Geigl, E., & Alonso, A. (2010). Early bone diagenesis in temperate environments part I: Surface features and histology. Palaeogeography, Palaeoclimatology, Palaeoecology, 288(1–4), 62–81. DOI: 10.1016/j.palaeo.2009.12.016.
- García-Martínez, R., Marín-Moratalla, N., Jordana, X., & Köhler, M. (2011). The ontogeny of bone growth in two species of dormice: Reconstructing life history traits. Comptes Rendus Palevol, 10(5–6), 489–498. DOI: 10.1016/j.crpv.2011.03.011.
- Gray, N-M., Kainec, K., Madar, S., Tomko, L., & Wolfe, S. (2007). Sink or swim? Bone density as a mechanism for buoyancy control in early cetaceans. Anatomical Record, 290(6), 638–653. DOI: 10.1002/ar.20533.
- Hayashi, S., Houssaye, A., Nakajima, Y., Chiba, K., Ando, T., Sawamura, H., Inuzuka, N., Kaneko, N., & Osaki, T. (2013). Bone inner structure suggests increasing aquatic adaptations in Desmostylia (Mammalia, Afrotheria). PLoS ONE, 8(4), e59146. DOI: 10.1371/journal.pone.0059146.
- Heck, C. T., Volkmann, G., & Woodward, H. N. (2020). Polyester or epoxy: assessing embedding product efficacy in paleohistological methods. PeerJ, 8:e10495. DOI: 10.7717/peerj.10495.
- Huan, H., Wang, W., & Wang, J. (2011). Huan yang shuzhi rongjie ji ji qi zhibei fangfa [Epoxy resin dissolving agent and preparation method thereof] (China Patent No. CN101649068B). China National Intellectual Property Administration.
- Jiang, G., Pickering, S. J., Lester, E. H., & Warrior, N. A. (2010). Decomposition of epoxy resin in supercritical isopropanol. Industrial and Engineering Chemistry Research, 49(10), 4535–4541. DOI: 10.1021/ie901542z.
- Jing, D., Zhang, S., Luo, W., Gao, X., Men, Y., Ma, C., Liu, X., Yi, Y., Bugde, A., Zhou, B. O., Zhao, Z., Yuan, Q., Feng, J. Q., Gao, L., Ge, W-P., & Zhao, H. (2018). Tissue clearing of both hard and soft tissue organs with the PEGASOS method. Cell Research, 28, 803–818. DOI: 10.1038/s41422-018-0049-z.
- Köhler, M. & Moyà-Solà, S. (2009). Physiological and life history strategies of a fossil large mammal in a resource-limited environment. PNAS, 106(48), 20354–20358. DOI: 10.1073/pnas.0813385106.
- Kolb, C., Scheyer, T. M., Veitschegger, K., Forasiepi, A. M., Amson, E., Van der Geer, A. A. E., Van den Hoek Ostende, L. W., Hayashi, S., & Sánchez-Villagra, M. R. (2015a). Mammalian bone palaeohistology: a survey and new data with emphasis on island forms. PeerJ, 3:e1358. DOI: 10.7717/peerj.1358.
- Kolb, C., Scheyer, T. M., Lister, A. M., Azorit, C., de Vos, J., Schlingemann, M. A. J., Rössner, G. E., Monaghan, N. T., & Sánchez-Villagra, M. R. (2015b). Growth in fossil and extant deer and implications for body size and life history evolution. BMC Evolutionary Biology, 15, 19. DOI: 10.1186/s12862-015-0295-3.
- Kuo, L., Zhang, L., & Xu, Z. (2019). Decomposition behavior and mechanism of epoxy resin from waste integrated circuits under supercritical water condition. Journal of Hazardous Materials, 374, 356–364. DOI: 10.1016/j.jhazmat.2019.04.028.
- Lamm, E. (2013). Preparation and sectioning of specimens. In K. Padian & E. Lamm (Eds.), Bone histology of fossil tetrapods (pp. 55–160). University of California Press. DOI: 10.1525/california/9780520273528.003.0004.
- Legendre, L. J. & Botha-Brink, J. (2018). Digging the compromise: investigating the link between limb bone histology and fossoriality in the aardvark (Orycteropus afer). PeerJ, 6:e5216. DOI: 10.7717/peerj.5216.
- Li, K., Zhang, L., & Xu, Z. (2019). Decomposition behavior and mechanism of epoxy resin from waste integrated circuits under supercritical water condition. Journal of Hazardous Materials, 374, 356–364. DOI: 10.1016/j.jhazmat.2019.04.028.
- Liu, Y., Gong, X., Wu, S., & Li, L. (2012). Decomposition of epoxy resin E-44/METHPA by tetrahydronaphthalene. In, 2012 International Conference on Biomedical Engineering and Biotechnology (pp. 1304–1306). IEEE. DOI: 10.1109/iCBEB.2012.124.
- Marín-Moratalla, N., Jordana, X., & Köhler, M. (2013). Bone histology as an approach to providing data on certain key life history traits in mammals: Implications for conservation biology. Mammalian Biology, 78(6), 422–429. DOI: 10.1016/j.mambio.2013.07.079.
- Mantel, G. (1850a). On a dorsal dermal spine of the Hylaeosaurus, recently discovered in the strata of Tilgate Forest. Philosophical Transactions of the Royal Society of London, 140, 391–392. DOI: 10.1098/rstl.1850.0018.
- Mantel, G. (1850b). On the Palorosaurus; an undescribed gigantic terrestrial reptile whose remains are associated with those of the Iguanodon and other saurian in the strata of Tilgate Forest, Sussex. Philosophical Transactions of the Royal Society of London, 140, 379–390. DOI: 10.1098/rstl.1850.0017.
- Meier, P. S., Bickelmann, C., Scheyer, T. M., Koyabu, D., & Sánchez-Villagra, M. R. (2013). Evolution of bone compactness in extant and extinct moles (Talpidae): exploring humeral microstructure in small fossorial mammals. BMC Evolutionary Biology, 13, 55. DOI: 10.1186/1471-2148-13-55.
- Mitchell, J., Sander, P. M., & Stein, K. (2017). Can secondary osteons be used as ontogenetic indicators in sauropods? Extending the histological ontogenetic stages into senescence. Paleobiology, 43(2), 321–342. DOI: 10.1017/pab.2016.47.
- Moncunill-Solè, B., Orlandi-Oliveras, G., Jordana, X., Rook, L., & Köhler, M. (2016). First approach of the life history of Prolagus apricenicus (Ochotonidae, Lagomorpha) from Terre Rosse sites (Gargano, Italy) using body mass estimation and paleohistological analysis. Comptes Rendus Palevol, 15(1–2), 227–237. DOI: 10.1016/j.crpv.2015.04.004.
- Montoya-Sanhueza, G. & Chinsamy, A. (2017). Long bone histology of the subterranean rodent Bathyergus suillus (Bathyergidae): ontogenetic pattern of cortical bone thickening. Journal of Anatomy, 230(2), 203–233. DOI: 10.1111/joa.12547.
- Montoya-Sanhueza, G., Bennet, N. C., Oosthuizen, M. K., Dengler-Crish, C. M., & Chinsamy, A. (2020). Long bone histomorphogenesis of the naked mole-rat: Histodiversity and intraspecific variation. Journal of Anatomy, 238(6), 1259–1283. DOI: 10.1111/joa.13381.
- Musumci, G. (2014). Past, present and future: overview on histology and histopathology. Journal of Histology & Histopathology, 1, 5. DOI: 10.7243/2055-091X-1-5.
- Nacarino-Meneses, C., Jordana, X., & Köhler, M. (2016). First approach to bone histology and skeletochronology of Equus hemionus. Comptes Rendus Palevol, 15(1–2), 267–277. DOI: 10.1016/j.crpv.2015.02.005.
- Nopcsa, B. F. (1933). On the histology of the ribs in immature and half-grown trachodont dinosaurs. Proceedings of the Zoolgical Society of London, 103(1), 221–226. DOI: 10.1111/j.1096-3642.1933.tb01588.x.
- Nopcsa, B. F. & Heidsiek, E. (1934). Über eine Pachyostotische Rippe aus der Kreide Rügens [A pachyostotic rib from the Rügen chalk]. Acta Zoologica, 15(2–3), 431–455. DOI: 10.1111/j.1463-6395.1934.tb00661.x.
- Oliveux, G., Dandy, L. O., & Leeke, G. A. (2015). Degradation of a model epoxy resin by solvolysis routes. Polymer Degradation and Stability, 118, 96–103. DOI: 10.1016/j.polymdegradstab.2015.04.016.
- Orlandi-Oliveras, G., Nacarino-Meneses, C., & Köhler, M. (2018). Bone histology provides insights into the life history mechanisms underlying dwarfing in hipparionins. Scientific Reports, 8, 17203. DOI: 10.1038/s41598-018-35347-x.
- Pawlicki, R., Korbel, A., & Kubiak, H. (1966). Cells, collagen fibrils and vessels in dinosaur bone. Nature, 5049, 655–657. DOI: 10.1038/211655a0.
- Quekett, J. (1849). On the intimate structure of bone, as composing the skeleton in the four great classes of animals, viz., mammals, birds, reptiles, and fishes, with some remarks on the great value of the knowledge of such structure in determining the affinities of minute fragments of organic remains. Transactions of the Microscopical Society of London, 2(1), 46–58. DOI: 10.1111/j.1365-2818.1849.tb05102.x.
- Sander, P. M. (2000). Longbone histology of the Tendaguru sauropods: implications for growth and biology. Paleobiology, 26(3), 466–488. DOI: 10.1666/0094-8373(2000)026<0466:LHOTTS>2.0.CO;2.
- Sawada, J., Nara, T., Fukui, J., Dodo, Y., & Hirata, K. (2014). Histomorphological species identification of tiny bone fragments from a Paleolithic site in the Northern Japanese Archipalago. Journal of Archaeological Science, 46, 270–280. DOI: 10.1016/j.jas.2014.03.025.
- Schrof, S., Varga, P., Galvis, L., Raum, K., & Masic, A. (2014). 3D Raman mapping of the collagen fibril orientation in human osteonal lamellae. Journal of Structural Biology, 187(3), 266–275. DOI: 10.1016/j.jsb.2014.07.001.
- Schweitzer, M. H., Marshall, M., Carron, K., Bohle, D. S., Busse, S. C., Arnold, E. V., Barnard, D., Horner, J. R., & Starkey, J. R. (1997). Heme compounds in dinosaur trabecular bone. Proceedings of the National Academy of Sciences of the United States of America, 94(12), 6291–6296. DOI: 10.1073/pnas.94.12.6291.
- Schweitzer, M. H., Wittmeyer, J. L., Horner, J. R., & Toporski, J. K. (2005). Soft-tissue vessels and cellular preservation in Tyrannosaurus rex. Science, 307(5717), 1952–1955. DOI: 10.1126/science.1108397.
- Schweitzer, M. H., Elsey, R. M., Dacke, C. G., Horner, J. R., & Lamm, E. (2007). Do egg-laying crocodilian (Alligator mississippiensis) archosaurs form medullary bone? Bone, 40(4), 1152–1158. DOI: 10.1016/j.bone.2006.10.029.
- Skedros, J. G., Bloebaum, R. D., Mason, M. W., & Bramble, D. M. (1994). Analysis of a tension/compression skeletal system: Possible strain-specific differences in the hierarchical organization of bone. Anatomical Record, 239(4), 396–404. DOI: 10.1002/ar.1092390406.
- Stein, K. & Sander, M. (2009). Histological core drilling: A less destructive method for studying bone histology. In M. A. Brown, J. F. Kane, & W. G. Parker (Eds.), Methods in fossil preparation: proceedings of the first annual fossil preparation and collections symposium (pp. 69–80). Petrified Forest National Park.
- Stein, M., Hayashi, S., & Sander, P. M. (2013). Long bone histology and growth patterns in ankylosaurs: Implications for life history and evolution. PLoS ONE, 8(7), e68590. DOI: 10.1371/journal.pone.0068590.
- Straehl, F. R., Scheyer, T. M., Forasiepi, A. M., & MacPhee, R. D. (2013). Evolutionary patterns of bone histology and bone compactness in xenarthran mammal long bones. PLoS ONE, 8(7), e69275. DOI: 10.1371/journal.pone.0069275.
- Suniaga, S., Rolvien, T., vom Scheidt, A., Fiedler, I. A. K., Hrishikesh, A. B., Huysseune, A., Witten, P. E., Amling, M., & Busse, B. (2018). Increased mechanical loading through controlled swimming exercise induces bone formation and mineralization in adult zebrafish. Scientific Reports, 8(1), 3646. DOI: 10.1038/s41598-018-21776-1.
- Susaki, E. A., Tainaka, K., Perrin, D., Kishino, F., Tawara, T., Watanabe, T. M., Yokoyama, C., Onoe, H., Eguchi, M., Yamaguchi, S., Abe, T., Kiyonari, H., Shimizu, Y., Miyawaki, A., Yokota, H., & Ueda, H. R. (2014). Whole-brain imaging with single-cell resolution using chemical cocktails and computational analysis. Cell, 157(3), 726–739. DOI: 10.1016/j.cell.2014.03.042.
- Tainaka, K., Kubota, S. I., Suyama, T. Q., Susaki, E. A., Perrin, D., Ukai-Tadenuma, M., Ukai, H., & Ueda, H. R. (2014). Whole-body imaging with single-cell resolution by tissue decolorization. Cell, 159(4), 911–924. DOI: 10.1016/j.cell.2014.10.034.
- Tajiri, R. & Fujita, T. (2013). Jushihomai to kenma ni yoru dobutsu soshiki kansatsu shiryo sakusei-ho: ko-soshiki to nan-soshiki no doji kansatsu [Observation methods of hard and soft animal tissues by grinding specimens impregnated with resin]. TAXA, Proceeding of the Japanese Society of Systematic Zoology, 35, 24–34.
- Taylor, M. A. (2000). Functional significance of bone ballastin in the evolution of buoyancy control strategies by aquatic tetrapods. Historical Biology, 14(1–2), 15–31. DOI: 10.1080/10292380009380550.
- Urano, Y., Sugimoto, Y., Tanoue, K., Matsumoto, R., Kawabe, S., Ohashi, T., & Fujiwara, S. (2019). The sandwich structure of keratinours layers controls the form and growth orientation of chicken rhinotheca. Journal of Anatomy, 235(2), 299–312. DOI: 10.1111/joa.12998.
- Wang, M., O’Connor, J. K., Bailleul, A. M., & Li, Z. (2020). Evolution and distribution of medullary bone: evidence from a new Early Cretaceous enantiornithine bird. National Science Review, 7(6), 1068–1078. DOI: 10.1093/nsr/nwz214.
- Wegst, U. G. K., Bai, H., Saiz, E., Tomsia, A. P., & Ritchie, R. O. (2014). Bioinspired structural materials. Nature Materials, 14(1), 23–26. DOI: 10.1038/nmat4089.
- Weissleder, R. (2001). A clearer vision for in vivo imaging. Nature Biotechnology, 19, 316–317. DOI: 10.1038/86684.
- Wolf, D., Kalthoff, D. C., & Sander, P. M. (2012). Osteoderm histology of the Pampatheriidae (Cingulata, Xenarthra, Mammalia): Implications for systematics, osteoderm growth, and biomechanical adaptation. Journal of Morphology, 273(4), 388–404. DOI: 10.1002/jmor.11029.
- Woodward, H. N., Horner, J. R. & Farlow, J. O. (2014). Quantification of intraskeletal histovariability in Alligator mississippiensis and implications for vertebrate osteohistology. PeerJ, 2:e422. DOI: 10.7717/peerj.422.
- Woodward, H. N. (2019). Maiasaura (Dinosauria: Hadrosauridae) tibia osteohistology reveals non-annual cortical vascular rings in young of the year. Frontiers in Earth Sciences, 7, 50. DOI: 10.3389/feart.2019.00050.
- Zedda, M. & Babosová, R. (2021). Does the osteon morphology depend on the body mass? A scaling study on macroscopic and histomorphometric differences between cow (Bos taurus) and sheep (Ovis aries). Zoomorphology, 140, 169–181. DOI: 10.1007/s00435-021-00516-6.
- Zedda, M., Lepore, G., Manca, P., Chisu, V., & Farina, V. (2008). Comparative bone histology of adult horses (Equus caballus) and Cows (Bos taurus). Anatomia Histologia Embryologia, 37(6), 442–445. DOI: 10.1111/j.1439-0264.2008.00878.x.