Abstract
Use of telemetry data to inform fisheries conservation and management is becoming increasingly common; as such, fish typically must be sedated before surgical implantation of transmitters into the coelom. Given that no widely available, immediate-release chemical sedative currently exists in North America, we investigated the feasibility of using electricity to sedate Lake Trout Salvelinus namaycush long enough for an experienced surgeon to implant an electronic transmitter (i.e., 180 s). Specifically, our study objectives were to determine (1) whether some combination of electrical waveform characteristics (i.e., duty cycle, frequency, voltage, and pulse type) could sedate Lake Trout for at least 180 s; and (2) whether Lake Trout that were sequentially exposed to continuous DC and pulsed DC had greater rates of spinal injury and short-term mortality than control fish. A Portable Electrosedation System unit was used to sedate hatchery and wild Lake Trout. Dual-frequency pulsed-DC and two-stage approaches successfully sedated Lake Trout and had similar induction and recovery times. Lake Trout sedated using the two-stage approach did not have survival rates or spinal abnormalities that were significantly different from those of control fish. We concluded that electricity was a viable alternative to chemical sedatives for sedating Lake Trout before surgical implantation of an electronic transmitter, but we suggest that Lake Trout and other closely related species (e.g., Arctic Char Salvelinus alpinus) may require morphotype-specific electrical waveforms due to their morphological diversity.
Received January 28, 2017; accepted April 24, 2017 Published online June 20, 2017
The ability to sedate fish is a valuable tool for hatchery or aquaculture settings (e.g., enumeration of fish, spawning, and transportation; Cooke et al. Citation2004; Zydlewski et al. Citation2008), population assessment (e.g., collection of length–weight data or sex determination; Soto and Burhanuddin Citation1995; Matsche Citation2013), and research purposes (e.g., implantation of acoustic telemetry transmitters; Wilson et al. Citation2017). Sedation allows fishery biologists to handle fish more efficiently and safely while also reducing physical damage and stress to fish caused by handling (Summerfelt and Smith Citation1990).
Many different chemical (Peake Citation1998; Taylor and Roberts Citation1999; Carter et al. Citation2011) and physical (Madden and Houston Citation1976) methods can be used to sedate fish. Marking and Meyer (Citation1985) described an ideal sedative as one that (1) has an induction time of less than 15 min and preferably less than 3 min; (2) has a short recovery time (5 min or less); (3) is nontoxic to fish and humans; (4) has no persistent effects on fish physiology and behavior; (5) leaves no residues and does not require withdrawal time; (6) has no cumulative negative impacts from repeated exposures; and (7) is inexpensive. Of the chemical sedatives available today, none can be considered ideal by these criteria. For example, tricaine methanesulfonate (MS-222) requires fish to be held for a 21-d withdrawal period before release, which may be inconsistent with study objectives and infeasible for field-related activities (Vandergoot et al. Citation2011). Alternatives exist that may be more appropriate for field work, such as AQUI-S 20E (AQUI-S, Lower Hutt, New Zealand), a eugenol-based investigational new animal drug (INAD), which can be used by participants in the National INAD Program as an immediate-release sedative for freshwater fishes treated as part of “field-based fishery management,” while all other fish sedated with this product are subject to a 72-h withdrawal period (Johnson et al. Citation2016). Additionally, exposure to chemical sedatives may have sublethal impacts on sensory abilities of fishes. For instance, MS-222 destroyed cilia on the olfactory sensory epithelia of Channel Catfish Ictalurus punctatus (Lewis et al. Citation1985), although exposure to MS-222 and 2-phenoxyethanol (another chemical sedative; e.g., Madison et al. Citation1972; Stasko et al. Citation1976) did not alter olfactory-mediated behavior of Pacific salmonids (Quinn et al. Citation1988). Furthermore, Readman et al. (Citation2013) recommended that ethical best practices should avoid several commonly used chemical sedatives, including MS-222 and benzocaine, after Zebrafish Danio rerio demonstrated an aversion to low concentrations of these compounds. Such issues have led researchers to seek an immediate-release sedative with no negative physiological or behavioral consequences for the fish (Trushenski et al. Citation2013).
Electricity, which is routinely used to collect fishes in the field (Reynolds and Kolz Citation2012), offers several advantages as a sedative relative to chemical options. For example, induction and recovery times are typically short, no withdrawal period is required, and equipment is easy to handle and not harmful to humans when used properly. Indeed, electricity has emerged as a preferred sedative for use with several species of sturgeon (Lake Sturgeon Acipenser fulvescens and Shortnose Sturgeon A. brevirostrum: Henyey et al. Citation2002; Atlantic Sturgeon A. oxyrinchus: Balazik et al. Citation2013; Pallid Sturgeon Scaphirhynchus albus: Johnson et al. Citation2016) and has been successfully applied to other warmwater and coolwater fishes (Walleye Sander vitreus: Vandergoot et al. Citation2011; Largemouth Bass Micropterus salmoides: Trushenski et al. Citation2012b; hybrid Striped Bass [White Bass Morone chrysops × Striped Bass Morone saxatilis]: Trushenski et al. Citation2012a). Although mortality has generally been low (e.g., Ainslie et al. Citation1998; Gaikowski et al. Citation2001), exposure to electricity may have sublethal consequences for fishes (e.g., internal hemorrhaging and spinal injuries; Dalbey et al. Citation1998; Dolan and Miranda Citation2004; Zydlewski et al. Citation2008). Furthermore, the reaction of fish to electricity varies depending on the intensity of the electrical field, the duration of exposure, and body morphometry (Ross and Ross Citation2008). Therefore, species-specific investigations into the efficacy of electricity as a sedative (hereafter, “electrosedation”), which should include both sublethal and lethal effects of exposure, are required because a single combination of waveform, frequency, and exposure time may not be suitable for all fishes (Walker et al. Citation1994; Zydlewski et al. Citation2008; Hudson et al. Citation2011).
The Lake Trout Salvelinus namaycush is an ecologically and economically important species across its native range (Muir et al. Citation2012; Chavarie et al. Citation2015) and has recently been the focus of large-scale behavioral and movement studies (Binder et al. Citation2016; Guzzo et al. Citation2016; Marsden et al. Citation2016). Suppression programs in western North America, where Lake Trout have been widely introduced (Martinez et al. Citation2009), have conducted similar studies to inform removal efforts (Dux et al. Citation2011; Muhlfeld et al. Citation2012). Such studies require that Lake Trout be sedated before they are subject to intracoelomic implantation of electronic transmitters (Vandergoot et al. Citation2011; Wilson et al. Citation2017). Here, we report on the feasibility of using electrosedation to sedate Lake Trout for the purpose of implanting an electronic transmitter into the coelom. Specifically, our study objectives were to determine (1) whether some combination of electrical waveform characteristics (i.e., duty cycle, frequency, voltage, and pulse type) could sedate Lake Trout for at least 180 s; and (2) whether Lake Trout sequentially exposed to two different waveforms (i.e., continuous DC [CDC] and pulsed DC [PDC]) had greater rates of spinal injury and short-term (i.e., 22-d) mortality than control fish. We used a Portable Electrosedation System (PES) unit (Smith-Root, Inc.) to initially evaluate candidate waveforms on hatchery-raised Lake Trout; we subsequently evaluated lethal and sublethal consequences of a single-setting exposure for a separate sample of wild-caught Lake Trout from Lake Huron.
METHODS
During February 2014, electrosedation settings were evaluated using lean Lake Trout (n = 34; mean TL ± SE = 699 ± 8 mm) obtained from Sullivan Creek National Fish Hatchery (NFH; Brimley, Michigan) and humper Lake Trout (n = 17; mean TL = 536 ± 11 mm) obtained from Iron River NFH (Iron River, Wisconsin). Several differences exist between these two morphotypes, with lean Lake Trout generally reaching larger sizes and having less body fat than humper Lake Trout (e.g., Muir et al. Citation2014; Hansen et al. Citation2016). Both sources of hatchery fish were derived from wild Lake Superior Lake Trout. Subsequent experiments designed to examine lethal and sublethal effects of electrosedation were conducted in December 2015 using wild lean Lake Trout (n = 28; mean TL = 670 ± 18 mm) collected with gill nets from northern Lake Huron by commercial fishers. All fish were held at the Hammond Bay Biological Station (HBBS; Millersburg, Michigan) in either circular fiberglass tanks (1,893 L [500 gal]; 1.2-m diameter) or concrete raceways (18 × 1.8 × 0.8 m) with a constant supply of freshwater from Lake Huron.
A PES unit was used to evaluate which electrical settings (i.e., waveform type, intensity, and duration) were sufficient for sedating Lake Trout long enough to permit surgical implantation of an electronic transmitter into the coelom. This unit consisted of a battery-operated power supply box, which regulated the type, intensity, and duration of electricity administered, and an exposure chamber (1.0 × 0.4 × 0.4 m) with an anode and a cathode mounted on opposite ends (). The PES unit produced three different types of DC (): CDC (i.e., voltage increased from zero to a maximum programmed voltage level within 1 s and decreased to zero 1 s after a predetermined exposure duration), PDC (i.e., voltage began at a maximum programmed voltage, stayed constant for the entire pulse duration, and then dropped to zero), and dual-frequency PDC (i.e., bursts of brief pulses at high frequency, with each burst delivered at low frequency). Each pulse type could be modified by changing the duty cycle (i.e., percentage of time that a given waveform is at peak voltage; range = 1–99%), frequency (i.e., number of pulses per second; range = 5–100 Hz [in 5-Hz increments] and 250, 500, and 1,000 Hz), and voltage (range = 20–400 V [in 5-V increments]). The PES unit could also be programmed to deliver two different settings in succession (i.e., a two-stage approach). For example, a fish could be exposed to 100-V CDC for 10 s and then immediately exposed to 100-V PDC at 30 Hz and a 25% duty cycle for another 10 s.
FIGURE 1. Photograph of a lean Lake Trout undergoing electrosedation with a Portable Electrosedation System unit (Smith-Root, Inc.; A = power supply box; B = exposure chamber).
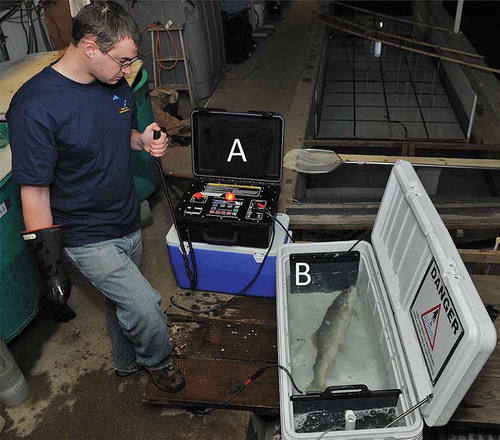
FIGURE 2. Illustrations of three different types of DC produced by the Portable Electrosedation System unit (Smith-Root, Inc.): (A) continuous DC, in which voltage increases from zero to a maximum programmed voltage level within 1 s and decreases to zero 1 s after a predetermined exposure duration; (B) pulsed DC, in which voltage begins at the maximum programmed voltage, stays constant for the entire pulse duration, and then drops to zero; and (C) dual-frequency pulsed DC, characterized by bursts of brief pulses at high frequency, with each burst delivered at low frequency.
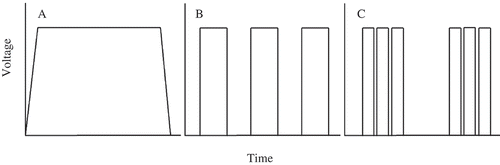
Water conductivity and temperature were measured in the exposure chamber with an ExStik EC410 conductivity meter (Extech Instruments, Nashua, New Hampshire). Power density (Pw) was calculated to estimate the amount of power to which fish were exposed for a given setting,
where Cw is the ambient conductivity (µS/cm) of the water, V is the voltage used for a particular setting, and D is the distance (cm) between the electrodes in the exposure chamber. Ambient water conductivity averaged 160 µS/cm and water temperature was 8.0°C during the initial pilot work (February 2014); ambient conductivity was 164 µS/cm measured at 6.6°C during the subsequent trials. The distance between the anode and the cathode in the exposure chamber was 76 cm during all trials. Power density was 100 µW/cm3 during the pilot work and was 102 µW/cm3 when we evaluated the two-stage approach (December 2015).
Initial pilot work was conducted on a subset of hatchery Lake Trout during February 2014 to evaluate candidate settings that had previously proven effective for other fishes. For example, we tested varying voltages of CDC that effectively sedated Rainbow Trout Oncorhynchus mykiss, Coho Salmon O. kisutch, and Bull Trout S. confluentus in prior studies (Kynard and Lonsdale Citation1975; Hudson et al. Citation2011). Similarly, we evaluated dual-frequency PDC waveforms that had previously sedated salmonids (Sharber et al. Citation1994; Tipping and Gilhuly Citation1996; Tesch et al. Citation1999). We did not evaluate PDC settings alone because a previous study suggested that PDC settings were not sufficient to sedate Lake Trout for intracoelomic surgical procedures (Gaikowski et al. Citation2001). However, preliminary work conducted at HBBS by Smith-Root, Inc., and HBBS staff suggested that a two-stage approach using CDC and PDC warranted further investigation (Martin O’Farrell, Smith-Root, Inc., personal communication). Candidate settings were evaluated by exposing a single fish and observing whether the fish reached stage-IV anesthesia (i.e., total loss of equilibrium, muscle tone, and responsiveness to visual and tactile stimuli but maintenance of a slow, steady opercular rate; Summerfelt and Smith Citation1990).
During the pilot trials, two general types of electrosedation setting were identified for sedating Lake Trout. The first type was a two-stage approach that exposed fish to CDC for 20 s, immediately followed by PDC for an additional 20 s; the second type involved exposing fish to dual-frequency PDC for 20 s. We evaluated four specific settings based on either the two-stage approach or the dual-frequency PDC approach by using a subset of lean Lake Trout (n = 7 fish/setting, or 28 total; ). Specifically, we quantified the induction time (i.e., time elapsed from the end of exposure to the point at which fish entered stage-IV anesthesia) and recovery time (i.e., time elapsed from induction until fish regained and maintained equilibrium) for fish that were exposed to each setting. Induction and recovery times were compared among settings by using ANOVA (α = 0.05). Similar settings were also applied to humper Lake Trout but did not sedate these fish to a satisfactory level during pilot trials; therefore, we focused our efforts on lean Lake Trout.
TABLE 1. Descriptions of four Portable Electrosedation System unit (Smith-Root, Inc.) settings that were used to electrosedate lean Lake Trout during February 2014 pilot work (CDC = continuous DC; PDC = pulsed DC). The asterisk indicates the setting that was used during 2015 trials.
Follow-up trials were conducted in December 2015 to evaluate short-term survival and determine whether wild Lake Trout sedated with the two-stage electrosedation settings experienced deleterious sublethal effects (i.e., spinal injury). We focused on the two-stage approach identified by our initial work rather than on the dual-frequency PDC because the CDC and PDC settings are easily replicated with other commercially available electrofishing control boxes. A similar two-stage approach was used successfully to sedate Pacific salmonids in hatchery settings (e.g., Zydlewski et al. Citation2008). Lean Lake Trout (n = 17) were sedated via the two-stage approach described above (i.e., two-stage A setting in ) and were immediately transferred to a mock surgery table. Fish were held on the surgical table for 180 s to mimic surgical implantation of an electronic transmitter, and a unique PIT tag was inserted into the coelom for identification purposes. We recognize that surgery duration can be influenced by a variety of factors (e.g., suture material and surgeon experience; Cooke et al. Citation2003), but we ultimately used a 180-s threshold for two reasons: (1) it was a conservative reflection of our collective experience performing hundreds of acoustic transmitter implantation surgeries on fishes (e.g., Walleye and Lake Trout); and (2) other published studies have reported surgical durations of 180 s or less (e.g., Collins et al. Citation2013). All fish received a constant supply of freshwater across their gills while on the surgical table. After 180 s, fish were transferred to individual recovery tanks, and recovery time was recorded as described above. Once recovered, Lake Trout were moved to a concrete raceway, and survival was assessed for 22 d postsedation. Additional fish (n = 11) held in an adjacent raceway served as a control group during the study. Control fish were handled in a similar manner to treatment fish during capture and transport to HBBS, but they were not subjected to electrosedation or simulated surgeries.
Lake Trout were examined for evidence of spinal injury after two-stage electrosedation trials (December 2015) by using computed tomography (CT) scans. Fish were euthanized at 22 d postexposure via a lethal dose of MS-222 and were immediately frozen. The CT scans were performed at the College of Veterinary Medicine, Ohio State University, with the fish in dorsal recumbency using an eight-detector, helical CT scanner (GE LightSpeed Ultra 8-Slice; GE Healthcare, Waukesha, Wisconsin). Settings of the CT scans were 100 kV, 125 mA, 0.8-s tube rotation time, 1.25-mm slice thickness, 0.875 pitch, and a gantry angle of 0°. Data were acquired in helical fashion, and images were reconstructed with a standard soft-tissue filter algorithm (with display settings of window level [WL] = 40 and window width [WW] = 400) and a bone filter algorithm (with display settings of WW = 2,500 and WL = 250). The entire fish was included within the scanning boundaries. Images were reviewed by a veterinary radiologist at an imaging station with medical-grade monitors using an OsiriX DICOM viewer (OsiriX 5.8.5, 32-bit; http://www.OsiriX-viewer.com/index.html). Images were interpreted without knowledge of which fish belonged to the control and treatment groups, and the order was randomized before review. Spinal damage was classified per Reynolds and Kolz (Citation2012) based on a scale of 0 to 3, where 0 indicates that no spinal damage is apparent; 1 indicates compression (distortion) of the vertebrae only; 2 indicates misalignment of the vertebrae, including compression; and 3 indicates fracture of one or more vertebrae or complete separation of two or more vertebrae. Counts of live and dead fish and counts of individuals with and without spinal injuries were compared between control and treatment groups by using Fisher’s exact test (α = 0.05).
RESULTS
The four settings evaluated () during our pilot trials effectively sedated lean Lake Trout. All fish were sedated during a single exposure, and after exposure the fish experienced involuntary movements before transitioning to stage-IV anesthesia. Mean induction times were similar among waveforms, approximately 100 s (F3, 24 = 1.49, P = 0.24; ), while mean recovery times varied among waveforms (~200–400 s; F3, 24 = 3.83, P = 0.02; ).
FIGURE 3. Induction and recovery times for lean Lake Trout electrosedated with a Portable Electrosedation System unit (Smith-Root, Inc.) at four different settings (see for a description of each setting). The upper and lower boundaries of the box represent the 25th and 75th percentiles, respectively. The solid line in the middle of each box represents the median, the whiskers represent range of data, and the dots indicate outliers. Lowercase letters indicate significant differences.
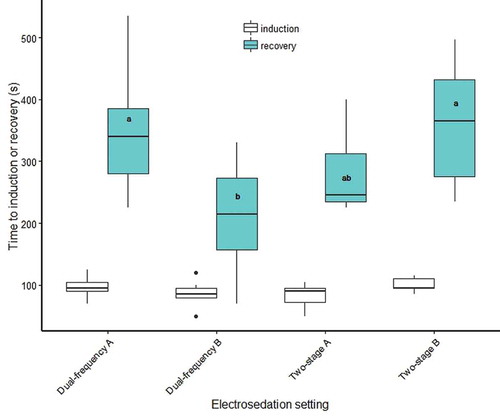
Lake Trout exposed to the two-stage approach of electrosedation (i.e., two-stage A setting in ) were effectively sedated and showed no immediate mortality. Ninety-four percent (16/17) of fish were sedated during the first complete exposure to our two-stage approach, while one fish required a second exposure before being induced. After induction occurred, 18% (3/17) of tested Lake Trout transitioned out of stage-IV anesthesia before the 180-s surgical window had elapsed. Fish took 73 ± 19 s (mean ± SE) on average to regain equilibrium after being transferred from the surgical board to the recovery tanks. Survival between the two groups did not differ significantly (P > 0.99; ). No mortalities occurred in the treatment group after 22 d, whereas one (9%) Lake Trout in the control group died. However, this fish appeared to have been injured during capture, so it was excluded from the analysis.
TABLE 2. Numbers of Lake Trout in control and treatment groups that died during the 22-d holding period or had spinal injuries after being exposed to a two-stage electrosedation approach (i.e., 20 s of 60-V continuous DC followed by 20 s of 60-V pulsed DC [30 Hz and 25% duty cycle]) administered via a Portable Electrosedation System unit (Smith-Root, Inc.). Numbers do not include fish that escaped during the 22-d holding period.
Lake Trout exposed to the two-stage approach of electrosedation showed minor spinal abnormalities similar to those of control fish (P > 0.99; ). Four fish in the treatment group (23%) escaped from the raceways after exposure and could not be examined for spinal injuries. Minor spinal injuries (score = 1) were observed in two fish from the treatment group and two fish from control group ().
FIGURE 4. Computed tomography (CT) scan of a lean Lake Trout used to evaluate different electrosedation settings for immobilizing fish prior to the implantation of electronic transmitters. The inset (image within the white outlined box) depicts a CT scan of the transverse plane of a vertebral bone exhibiting a minor spinal injury. Within the inset, the white arrow highlights a defect in the left lateral aspect of the vertebral body, and the black arrow points to a normal vertebral body margin on the right (R) side. The ventral aspect of the vertebral body is at the bottom of the image.
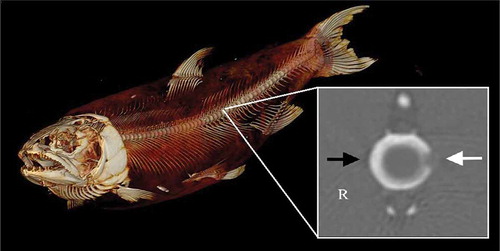
DISCUSSION
The two-stage electrosedation settings evaluated herein were effective at sedating the majority of Lake Trout long enough for an experienced surgeon to implant an electronic transmitter and had no apparent lethal or sublethal effects. Similar to published work on a range of other freshwater fishes (Henyey et al. Citation2002; Vandergoot et al. Citation2011; Trushenski and Bowker Citation2012), Lake Trout recovered quickly, with no mortality observed 22 d after exposure. Gaikowski et al. (Citation2001) concluded that exposure to PDC (i.e., 0.6–0.8 V/cm, 50 Hz, and 36% duty cycle for 15–30 s) adequately sedated Lake Trout of varying sizes (i.e., 350–830 mm TL) to allow routine fish handling tasks to be performed. However, mean recovery times observed by Gaikowski et al. (Citation2001) for Lake Trout of sizes comparable to those used in our study and fish tagged by other studies (e.g., Binder et al. Citation2016; Marsden et al. Citation2016) were generally less than the 180-s threshold needed for implanting an electronic transmitter. A small fraction of Lake Trout sedated using our two-stage approach showed minor spinal injuries, but the incidence of such injuries was not different from that in the control group. This suggested that injuries were not caused by the electrosedation procedure, similar to spinal injury rates observed for similarly sized Lake Trout sedated using PDC (Gaikowski et al. Citation2001) and for Walleyes sedated with either CDC or PDC (Vandergoot et al. Citation2011).
The electrosedation settings we found useful for sedating Lake Trout differed from those reported by researchers that have conducted similar experiments with other fishes. For instance, we tried two different CDC techniques (e.g., see different techniques used by Jennings and Looney Citation1998; Hudson et al. Citation2011), and in contrast to studies of other salmonid (e.g., Kynard and Lonsdale Citation1975; Gunstrom and Bethers Citation1985), acipenserid (Henyey et al. Citation2002; Balazik et al. Citation2013; Johnson et al. Citation2016), percid (Vandergoot et al. Citation2011), and moronid (Jennings and Looney Citation1998) fishes, these techniques did not effectively immobilize Lake Trout for implantation of electronic transmitters. As previously mentioned, PDC used independently also did not sedate Lake Trout long enough to allow implantation of an electronic transmitter (Gaikowski et al. Citation2001); however, when combined with CDC in a two-stage approach, the desired threshold of sedation was achieved. A two-stage approach has successfully been used to immobilize and euthanize Chinook Salmon O. tshawytscha (Zydlewski et al. Citation2008), although voltage and exposure time differed between our two-stage approach and that used on Chinook Salmon. Using our two-stage approach, Lake Trout required a longer exposure time and had a greater postexposure induction time than observed in other electrosedation studies. For instance, Walleyes required a 3-s exposure to PDC and were typically induced to stage-IV anesthesia immediately after exposure (Vandergoot et al. Citation2011; M. D. Faust, personal observation), whereas Lake Trout required longer exposure and experienced involuntary movements before being fully induced to stage-IV anesthesia.
Most electrosedation studies have acknowledged that species-specific waveforms are necessary to sedate fish long enough for a particular procedure to be performed (e.g., Gaikowski et al. Citation2001; Vandergoot et al. Citation2011), but we suggest that Lake Trout and related species, such as Arctic Char S. alpinus, may require a morphotype-specific approach due to the high degree of morphological diversity (Muir et al. Citation2016). For instance, although the two-stage and dual-frequency PDC approaches successfully sedated adult lean Lake Trout, these settings failed to satisfactorily sedate adult humper Lake Trout during pilot trials. Several differences exist between the two morphotypes, with lean Lake Trout generally reaching larger sizes and having less body fat than humper Lake Trout (e.g., Muir et al. Citation2014; Hansen et al. Citation2016). Both characteristics may have contributed to the inability of our settings to sedate the humper Lake Trout. Researchers wanting to use technologies such as acoustic telemetry to study morphotypes other than lean Lake Trout should evaluate electrosedation settings (e.g., waveform, voltage gradients, and duration) in addition to those reported here.
Although we focused our application of electrosedation to Lake Trout for implanting electronic transmitters into the coelom, the settings described here may also be useful for other purposes that require fish to be sedated for a similar amount of time. For instance, application of externally attached pop-up satellite archival tags (PSATs) to study movement and habitat use was previously limited to large fishes, but recent technological advances have led to the miniaturization of such transmitters and an ability to use PSATs on smaller fishes (e.g., Galuardi and Lutcavage Citation2012). Although methods for external attachment of electronic transmitters can be performed quickly without sedation (<30 s; Colotelo et al. Citation2013; Raby et al. Citation2015), PSATs typically are attached via a harness while the fish are sedated (Jepsen et al. Citation2015). Therefore, our electrosedation settings may be of utility to researchers exploring the use of PSATs or similar external electronic transmitters to study Lake Trout.
The use of electrosedation in fisheries work has increased in recent years. Researchers have experimented with a variety of electrical settings to achieve sedation levels required for their needs (e.g., surgical procedures and handling). Here, we provide a suite of electrosedation settings that are beneficial for use with lean Lake Trout, satisfying many of the criteria for an ideal sedative as described by Marking and Meyer (Citation1985). In studies that use fish implanted with electronic transmitters to understand the ecology and population dynamics of a stock, a critical assumption is that tagged fish are representative of the population at large (i.e., fish without electronic transmitters; Pine et al. Citation2003). Because the tagging process may bias fish behavior (Goddard et al. Citation1974; Wilson et al. Citation2017), future studies should continue to evaluate the lethal and sublethal effects of sedating fish with electricity to better understand these potential biases.
ACKNOWLEDGMENTS
This work was funded by the Great Lakes Fishery Commission by way of Great Lakes Restoration Initiative appropriations (GL-00E23010) and is Contribution 34 of the Great Lakes Acoustic Telemetry Observation System. Scott Miehls, Chris Holbrook, Eric Larson, and Chris Wright provided technical assistance during this study. Albert Lablanc provided Lake Trout caught in Lake Huron. Dale Bast provided fish from Iron River NFH; Crystal LeGault-Anderson provided fish from Sullivan Creek NFH. Dan Dembkowski reviewed an earlier version of this manuscript. Comments from an anonymous reviewer improved this manuscript. All sampling and handling of fish during research by the Great Lakes Science Center were carried out in accordance with American Fisheries Society guidelines for the care and use of fishes (Use of Fishes in Research Committee Citation2014). Any use of trade, product, or firm names is for descriptive purposes only and does not imply endorsement by the U.S. Government.
REFERENCES
- Ainslie, B. J., J. R. Post, and A. J. Paul. 1998. Effects of pulsed and continuous DC electrofishing on juvenile Rainbow Trout. North American Journal of Fisheries Management 18:905–918.
- Balazik, M. T., B. C. Langford, G. C. Garman, M. L. Fine, J. K. Stewart, R. J. Latour, and S. P. McIninch. 2013. Comparison of MS-222 and electronarcosis as anesthetics on cortisol levels in juvenile Atlantic Sturgeon. Transactions of the American Fisheries Society 142:1640–1643.
- Binder, T. R., S. C. Riley, C. M. Holbrook, M. J. Hansen, R. A. Bergstedt, C. R. Bronte, J. X. He, and C. C. Krueger. 2016. Spawning site fidelity of wild and hatchery Lake Trout, Salvelinus namaycush, in northern Lake Huron. Canadian Journal of Fisheries and Aquatic Sciences 78:18–34.
- Carter, K. M., C. M. Woodley, and R. S. Brown. 2011. A review of tricaine methanesulfonate for anesthesia of fish. Reviews in Fish Biology and Fisheries 21:51–59.
- Chavarie, L., K. Howland, L. Harris, and W. Tonn. 2015. Polymorphism in Lake Trout in Great Bear Lake: intra-lake morphological diversification at two spatial scales. Biological Journal of the Linnean Society 114:109–125.
- Collins, A. L., S. G. Hinch, D. W. Welch, S. J. Cooke, and T. D. Clark. 2013. Intracoelomic acoustic tagging of juvenile Sockeye Salmon: swimming performance, survival, and postsurgical wound healing in freshwater and during a transition to seawater. Transactions of the American Fisheries Society 142:515–523.
- Colotelo, A. H., G. D. Raby, C. T. Hasler, T. J. Haxton, K. E. Smokorowski, G. Blouin-Demers, and S. J. Cooke. 2013. Northern Pike bycatch in an inland commercial hoop net fishery: effects of water temperature and net tending frequency on injury, physiology, and survival. Fisheries Research 137:41–49.
- Cooke, S. J., B. D. S. Graeb, C. D. Suski, and K. G. Ostrand. 2003. Effects of suture material on incision healing, growth and survival of juvenile Largemouth Bass implanted with miniature radio transmitters: case study of a novice and experienced fish surgeon. Journal of Fish Biology 62:1366–1380.
- Cooke, S. J., C. D. Suski, K. G. Ostrand, B. L. Tufts, and D. H. Wahl. 2004. Behavioral and physiological assessment of low concentrations of clove oil anesthetic for handling and transporting Largemouth Bass (Micropterus salmoides). Aquaculture 239:509–552.
- Dalbey, S. R., T. E. McMahon, and W. Fredenberg. 1998. Effect of electrofishing pulse shape and electrofishing-induced spinal injury on long-term growth and survival of wild Rainbow Trout. North American Journal of Fisheries Management 16:560–569.
- Dolan, C. R., and L. E. Miranda. 2004. Injury and mortality of warmwater fishes immobilized by electrofishing. North American Journal of Fisheries Management 24:118–127.
- Dux, A. M., C. S. Guy, and W. A. Fredenberg. 2011. Spatiotemporal distribution and population characteristics of a nonnative Lake Trout population, with implications for suppression. North American Journal of Fisheries Management 31:187–196.
- Gaikowski, M. P., W. H. Gingerich, and S. Gutreuter. 2001. Short-duration electrical immobilization of Lake Trout. North American Journal of Fisheries Management 21:381–392.
- Galuardi, B., and M. Lutcavage. 2012. Dispersal routes and habitat utilization of juvenile Atlantic Bluefin Tuna, Thunnus thynnus, tracked with mini PSAT and archival tags. PLOS (Public Library of Science) ONE [online serial] 7(5):e37829.
- Goddard, C. I., J. W. Lilley, and J. S. Tait. 1974. Effects of MS-222 anesthetization on temperature selection in Lake Trout, Salvelinus namaycush. Journal of the Fisheries Research Board of Canada 31:100–103.
- Gunstrom, G. K., and M. Bethers. 1985. Electrical anesthesia for handling large salmonids. Progressive Fish-Culturist 47:67–69.
- Guzzo, M. M., P. J. Blanchfield, A. J. Chapelsky, and P. A. Cott. 2016. Resource partitioning among top-level piscivores in a sub-Arctic lake during thermal stratification. Journal of Great Lakes Research 42:276–285.
- Hansen, M. J., N. A. Nate, A. M. Muir, C. R. Bronte, M. S. Zimmerman, and C. C. Krueger. 2016. Life history variation among four Lake Trout morphs at Isle Royale, Lake Superior. Journal of Great Lakes Research 42:421–432.
- Henyey, G., B. Kynard, and P. Zhuang. 2002. Use of electronarcosis to immobilize juvenile Lake and Shortnose sturgeons for handling and the effects on their behavior. Journal of Applied Ichthyology 18:502–504.
- Hudson, J. M., J. R. Johnson, and B. Kynard. 2011. A portable electronarcosis system for anesthetizing salmonids and other fish. North American Journal of Fisheries Management 31:335–339.
- Jennings, C. A., and G. L. Looney. 1998. Evaluation of two types of anesthesia for performing surgery on Striped Bass. North American Journal of Fisheries Management 18:187–190.
- Jepsen, N., E. B. Thorstad, T. Havn, and M. C. Lucas. 2015. The use of external electronic tags on fish: an evaluation of tag retention and tagging effects. Animal Biotelemetry [online serial] 3:49.
- Johnson, J. L., J. T. Trushenski, and J. D. Bowker. 2016. Induction, recovery, and hematological responses of Pallid Sturgeon to chemical and electrical sedation. North American Journal of Fisheries Management 36:568–575.
- Kynard, B., and E. Lonsdale. 1975. Experimental study of galvanonarcosis for Rainbow Trout (Salmo gairdneri) immobilization. Journal of the Fisheries Research Board of Canada 32:300–302.
- Lewis, D. H., R. J. Tarpley, J. E. Marks, and R. F. Sis. 1985. Drug-induced structural changes in olfactory organ of Channel Catfish Ictalurus punctatus, Rafinesque. Journal of Fish Biology 26:355–358.
- Madden, J. A., and A. H. Houston. 1976. Use of electroanaesthesia with freshwater teleosts: some physiological consequences in the Rainbow Trout, Salmo gairdneri Richardson. Journal of Fish Biology 9:457–462.
- Madison, D. M., R. M. Horrall, A. B. Stasko, and A. D. Hasler. 1972. Migratory movements of adult Sockeye Salmon (Oncorhynchus nerka) in coastal British Columbia as revealed by ultrasonic tracking. Journal of the Fisheries Research Board of Canada 29:1025–1033.
- Marking, L. L., and F. P. Meyer. 1985. Are better anesthetics needed in fisheries? Fisheries 10(6):2–5.
- Marsden, J. E., T. R. Binder, J. Johnson, J. He, N. Dingledine, J. Adams, N. S. Johnson, T. J. Buchinger, and C. C. Krueger. 2016. Five-year evaluation of habitat remediation in Thunder Bay, Lake Huron: comparison of constructed reef characteristics that attract spawning Lake Trout. Fisheries Research 183:275–286.
- Martinez, P. J., P. E. Bigelow, M. A. Deleray, W. A. Fredenberg, B. S. Hansen, N. J. Horner, S. K. Lehr, R. W. Schneidervin, S. A. Tolentino, and A. E. Viola. 2009. Western Lake Trout woes. Fisheries 34:424–442.
- Matsche, M. A. 2013. A portable electro-immobilization and laparoscopy system for sex determination and gonadal biopsy in Largemouth Bass Micropterus salmoides. Journal of Fish Biology 83:1391–1400.
- Muhlfeld, C. C., J. J. Giersch, and B. Martoz. 2012. Seasonal movements of non-native Lake Trout in a connected lake and river system. Fisheries Management and Ecology 19:224–232.
- Muir, A. M., C. R. Bronte, M. S. Zimmerman, H. R. Quinlan, J. D. Glase, and C. C. Krueger. 2014. Ecomorphological diversity of Lake Trout at Isle Royale, Lake Superior. Transactions of the American Fisheries Society 143:972–987.
- Muir, A. M., M. J. Hansen, C. R. Bronte, and C. C. Krueger. 2016. If Arctic Charr Salvelinus alpinus is ‘the most diverse vertebrate’, what is the Lake Charr Salvelinus namaycush? Fish and Fisheries 17:1194–1207.
- Muir, A. M., C. C. Krueger, and M. J. Hansen. 2012. Re-establishing Lake Trout in the Laurentian Great Lakes: the past, present, and future. Pages 533–588 in W. W. Taylor, A. J. Lynch, and N. J. Leonard, editors. Great Lakes fishery policy and management: a binational perspective, 2nd edition. Michigan State University Press, East Lansing.
- Peake, S. 1998. Sodium bicarbonate and clove oil as potential anesthetics for nonsalmonid fishes. North American Journal of Fisheries Management 18:919–924.
- Pine, W. E., K. H. Pollock, J. E. Hightower, T. J. Kwak, and J. A. Rice. 2003. A review of tagging methods for estimating fish population size and components of mortality. Fisheries 28:10–23.
- Quinn, T. P., A. F. Olson, and J. T. Konecki. 1988. Effects of anaesthesia on the chemosensory behavior of Pacific salmon. Journal of Fish Biology 33:637–641.
- Raby, G. D., S. G. Hinch, D. A. Patterson, J. A. Hills, L. A. Thompson, and S. J. Cooke. 2015. Mechanisms to explain purse seine bycatch mortality of Coho Salmon. Ecological Applications 25:1757–1775.
- Readman, G. D., S. F. Owen, J. C. Murrell, and T. G. Knowles. 2013. Do fish perceive anaesthetics as aversive? PLOS (Public Library of Science) ONE [online serial] 8 (9):e73773.
- Reynolds, J. B., and A. L. Kolz. 2012. Electrofishing. Pages 305–361 in A. V. Zale, D. L. Parrish, and T. M. Sutton, editors. Fisheries techniques, 3rd edition. American Fisheries Society, Bethesda, Maryland.
- Ross, L. G., and B. Ross. 2008. Anaesthetic and sedative techniques for aquatic animals, 3rd edition. Blackwell Scientific Publications, Oxford, UK.
- Sharber, N. G., S. W. Carothers, J. P. Sharber, J. C. de Vos Jr, and D. A. House. 1994. Reducing electrofishing-induced injury of Rainbow Trout. North American Journal of Fisheries Management 14:340–346.
- Soto, C. G., and Burhanuddin. 1995. Clove oil as a fish anaesthetic for measuring length and weight of Rabbitfish (Siganus lineatus). Aquaculture 136:149–152.
- Stasko, A. B., R. M. Horrall, and A. D. Hasler. 1976. Coastal movements of adult Fraser River Sockeye Salmon (Oncorhynchus nerka) observed by ultrasonic tracking. Transactions of the American Fisheries Society 105:64–71.
- Summerfelt, R. C., and L. S. Smith. 1990. Anesthesia, surgery, and related techniques. Pages 213–272 in C. B. Schreck and P. B. Moyle, editors. Methods for fish biology. American Fisheries Society, Bethesda, Maryland.
- Taylor, P. W., and S. D. Roberts. 1999. Clove oil: an alternative anaesthetic for aquaculture. North American Journal of Fisheries Management 61:150–155.
- Tesch, A. H., D. Aro, G. Clark, D. Kucipeck, and J. D. Mahan. 1999. Effects of varying voltage and pulse pattern during electrical immobilization of adult Chum Salmon on egg survival to the eyed egg stage. North American Journal of Aquaculture 61:355–358.
- Tipping, J. M., and G. J. Gilhuly. 1996. Survival of electroanesthetized adult steelhead and eggs of fall Chinook Salmon. North American Journal of Fisheries Management 16:469–472.
- Trushenski, J. T., and J. D. Bowker. 2012. Effect of voltage and exposure time on fish response to electrosedation. Journal of Fish and Wildlife Management 3:276–287.
- Trushenski, J. T., J. D. Bowker, S. J. Cooke, D. Erdahl, T. Bell, J. R. MacMillan, R. P. Yanong, J. E. Hill, M. C. Fabrizio, J. E. Garvey, and S. Sharon. 2013. Issues regarding the use of sedatives in fisheries and the need for immediate-release options. Transactions of the American Fisheries Society 142:156–170.
- Trushenski, J. T., J. D. Bowker, B. R. Gause, and B. L. Mulligan. 2012a. Chemical and electrical approaches to sedation of hybrid Striped Bass: induction, recovery, and physiological responses to sedation. Transactions of the American Fisheries Society 141:455–467.
- Trushenski, J. T., J. D. Bowker, B. L. Mulligan, and B. R. Gause. 2012b. Induction, recovery, and hematological responses of Largemouth Bass to chemo- and electrosedation. North American Journal of Aquaculture 74:214–223
- Use of Fishes in Research Committee (joint committee of the American Fisheries Society, the American Institute of Fishery Research Biologists, and the American Society of Ichthyologists and Herpetologists). 2014. Guidelines for the use of fishes in research. American Fisheries Society, Bethesda, Maryland.
- Vandergoot, C. S., K. J. Murchie, S. J. Cooke, J. M. Dettmers, R. A. Bergstedt, and D. G. Fielder. 2011. Evaluation of two forms of electroanesthesia and carbon dioxide for short-term anesthesia of Walleye. North American Journal of Fisheries Management 31:914–922.
- Walker, M. K., E. A. Yanke, and W. H. Gingerich. 1994. Use of electronarcosis to immobilize juvenile and adult Northern Pike. Progressive Fish-Culturist 56:237–243.
- Wilson, A. D. M., T. A. Hayden, C. S. Vandergoot, R. T. Kraus, J. M. Dettmers, S. J. Cooke, and C. C. Krueger. 2017. Does intracoelomic implantation of telemetry transmitters alter the short-term post-release behaviour of a migratory freshwater fish? Ecology of Freshwater Fish 26:292–300.
- Zydlewski, G. B., W. Gale, J. Holes, J. Johnson, T. Brigham, and W. Thorson. 2008. Use of electroshock for euthanizing and immobilizing adult spring Chinook Salmon in a hatchery. North American Journal of Aquaculture 70:414–424.