Abstract
Background
Small airway dysfunction (SAD) is increasingly recognized as an important feature of pediatric asthma yet typically relies on spirometry-derived FEF25–75 to detect its presence. Multiple breath washout (MBW) and oscillometry potentially offer improved sensitivity for SAD detection, but their utility in comparison to FEF25–75, and correlations with clinical outcomes remains unclear for school-age asthma. We investigated SAD occurrence using these techniques, between-test correlation and links to clinical outcomes in 57 asthmatic children aged 8–18 years.
Methods
MBW and spirometry abnormality were defined as z-scores above/below ± 1.96, generating MBW reference equations from contemporaneous controls (n = 69). Abnormal oscillometry was defined as > 97.5th percentile, also from contemporaneous controls (n = 146). Individuals with abnormal FEF25–75, MBW, or oscillometry were considered to have SAD.
Results
Using these limits of normal, SAD was present on oscillometry in 63% (resistance at 5–20 Hz; R5-R20; >97.5th percentile), on MBW in 54% (Scond; z-scores> +1.96) and in spirometry FEF25–75 in 44% of participants (z-scores< −1.96). SAD, defined by oscillometry and/or MBW abnormality, occurred in 77%. Among those with abnormal R5-R20, Scond was abnormal in 71%. Correlations indicated both R5-R20 and Scond were linked to asthma medication burden, baseline FEV1 and reversibility. Additionally, Scond correlated with FENO and magnitude of bronchial hyper-responsiveness. SAD, detected by oscillometry and/or MBW, occurred in almost 80% of school-aged asthmatic children, surpassing FEF25–75 detection rates.
Conclusions
Discordant oscillometry and MBW abnormality suggests they reflect different aspects of SAD, serving as complementary tools. Key asthma clinical features, like reversibility, had stronger correlation with MBW-derived Scond than oscillometry-derived R5-R20.
Introduction
Asthma is characterized by airway inflammation, airflow limitation and bronchial hyper-responsiveness (BHR). The small (or peripheral) airways, defined as airways with an internal diameter < 2 mm, have been recognized as a major site for both airway inflammation (Citation1,Citation2) and airflow limitation in asthma (Citation3). Spirometry remains the gold standard method to determine presence and severity of obstruction to airflow in asthmatic subjects, and whilst the limitation of FEV1 in detecting small airway obstruction has been long recognized (Citation4), recent studies have highlighted the ability of FEF25–75 in this setting across both adult (Citation5) and pediatric (Citation6) cohorts. Detecting this “silent” small airway dysfunction (SAD) is also possible using multiple breath washout (MBW) and oscillometry which reflect differing aspects of peripheral lung function: MBW assesses gas mixing, a process involving all the airways, whilst oscillometry reflects underlying lung mechanics across a wide range of airway generations within the airway tree. For this reason, insights provided by the different methods are unlikely to be identical but are rarely directly compared.
Direct comparison in adult asthma has been performed within the ATLANTIS study (Citation5), which reported greater rates of abnormality and utility for FEF25–75, than that observed for MBW and IOS indices. Pediatric literature focusing on detection of SAD is scant. A recent pediatric study evaluated the performance of spirometry indices to detect an a priori classification of SAD in patients being managed at a single tertiary pediatric hospital (Citation6). Due to COVID restrictions, oscillometry, present at the facility, was unable to be performed at the time of spirometry assessment. FEF25–75 abnormality was defined as < −1.65 z-scores and identified in the highest proportion of those with SAD (41%), and the author concluded that spirometry was an adequate tool to screen for SAD in this setting. He suggested that additional complementary tests, such as oscillometry, could be considered to confirm its presence, and that chest CT was not indicated in pediatric asthma. Formal agreement between oscillometry and FEF25–75, alongside other promising tests for SAD (e.g., MBW), have not been performed to date in pediatric asthma.
Published data is limited to comparing one of the two tests to FEF25–75 (Citation7) which suggest greater rates of abnormality than detected with FEF25–75 (Citation8,Citation9). To better understand the significance of SAD detection using these tools, it is important to relate MBW and oscillometry indices not only to current asthma control but also to other important aspects of the underlying disease process such as airway inflammation, bronchodilator response (BDR) and BHR. Fractional concentration of exhaled nitric oxide (FENO) is a marker of type 2 driven airway inflammation and has been shown to predict subsequent loss of asthma control when preventer medications are weaned (Citation10). BHR is an established predictor of whether childhood asthma persists into adulthood (Citation11), although it may persist despite remission, and FENO and SAD, as assessed by oscillometry, have been shown to predict BHR (Citation12).
The aim of our study was to directly compare rates of abnormality in MBW and oscillometry indices, to that observed with low FEF25–75 values, in a cohort of asthmatic school-aged children in a Swedish secondary care setting using equipment-specific thresholds for abnormality derived from concurrent healthy cohorts. Furthermore, we aimed at examining how SAD, detected using these tools, related to important asthma outcomes such as asthma control, baseline and reversible airway obstruction (as assessed by spirometry), degree of airway inflammation (FENO) and BHR.
Methods
Participants with asthma
Over a seven-month period, all children aged 8 to 18 years, with a physician-confirmed asthma diagnosis, attending an outpatient clinic at Skaraborg Central Hospital, a regional pediatric hospital in Skövde, Sweden, were consecutively invited to participate in the study. Exclusion criteria included acute exacerbations within three weeks prior to evaluation, history of premature birth (<37 wk. gestation), and cardiac disease, diabetes, or other systemic disease. The study was approved by the Regional Ethics Committee in Gothenburg, Sweden, #DNR266-09. Written consent was obtained from all guardians and/or participants prior to examination.
Study protocol
The study protocol included two visits. The first visit included FENO, sulfur hexafluoride (SF6) MBW, impulse oscillometry (IOS) and spirometry testing (in that order). BDR testing was performed using inhalation of 400 mcg salbutamol (Airomir®, Teva Sweden AB, Helsingborg, Sweden) via pressurized metered dose inhaler (pMDI) and a non-electrostatic holding chamber (Vortex®, Pari Medical GmbH, Starnberg, Germany). A self-administered asthma control test (ACT for 4–11 years (Citation13) or 12–18 years (Citation14)) was conducted. A “medication score” was calculated (increasing score with higher asthma medication burden, details in supplementary file), based on reported current asthma medication. The second visit, conducted within two weeks, consisted of spirometry performed before and after isocapnoic dry air hyperventilation challenge (DACh). All participants withheld asthma medication for at least 12 h prior to study visits.
Measurements of airway inflammation
FENO was measured using an online chemiluminescent NO analyzer (CLD88, EcoMedics AG, Dürnten, Switzerland) at 50 ml/s, as per American Thoracic Society (ATS) and European Respiratory Society (ERS) guidelines (Citation15), with abnormality defined as > 20 parts per billion (ppb) (Citation16).
Measurements of lung function
MBW was performed according to current ERS/ATS consensus standards (Citation17) using an inert gas mixture containing 4.0% SF6, 4.0% helium, 21.0% oxygen, and balance nitrogen. Gas concentrations were recorded by respiratory mass-spectrometer (AMIS 2000, Innovision AS, Odense, Denmark), details in supplementary file. Results were reported as mean values for indices measuring global ventilation inhomogeneity (Lung clearance index, LCI, and slope index, SI), and concentration-normalized phase III slope (SnIII) analysis indices Scond and Sacin. SnIII values were multiplied by expiratory tidal volume (VT) to allow correction for lung size and breathing pattern variability (Citation17). IOS and spirometry was performed using a Jaeger MasterScreen system (CareFusion, Würzburg, Germany). IOS was performed with hands supporting the cheeks, according to ERS recommendations (Citation18). Indices reported were resistance at 5 Hz (R5), resistance at 5 minus 20 Hz (R5-20Hz) and area of reactance (AX). Spirometry was performed according to ATS/ERS technical standards (Citation19) and predictive values were calculated according to Global Lung Function Initiative (GLI) reference equations (Citation20).
A significant BDR was defined as ≥10% FEV1 improvement in relation to the pre BD FEV1. At the second visit, DACh was performed using an Aiolos Astmatest® system (Aiolos Medicinsk teknik, Karlstad, Sweden). Spirometry was performed at baseline and 2, 5 and 10 min after the challenge and finally 15 min after BD administration. BHR was defined as a maximal fall in FEV1 from baseline of ≥10% (Citation21).
Healthy control cohorts
Two different healthy control cohorts were recruited to derive predicted values for IOS and SF6 MBW indices, respectively. A healthy control was defined as a subject without a history of premature birth, asthma or other lower airway respiratory conditions, absence of cardiac problems or malformations of the chest or the airways, and without developmental problems. Subjects were not being tested within a three-week period of an airway tract infection. One hundred and forty-six (69 females) healthy children aged 6–18 years, performed IOS using the same equipment and procedure as used by the children with asthma. Predictive values and upper limit of normal (ULN) for IOS outcomes were defined as 50th and 97.5th percentiles in a quantile regression model. Sixty-nine (35 females) healthy children aged 7–18 years performed SF6 MBW using the same equipment and procedure as used by the children with asthma. Predicted values and ULN for SF6 MBW was defined as mean value and mean +1.96 SD in the healthy cohort. Detailed information about the two healthy control cohorts and the derived reference values are provided in a supplementary file.
Statistical analysis
Demographic data were presented as mean (standard deviation; SD), while remaining data were expressed as median (inter quartile range; IQR or range). Spearman’s rho (ρ) was used for bivariate analysis. MBW and spirometry outcomes were expressed as z-scores, while IOS outcomes were expressed in %predicted. Abnormality in MBW and spirometry outcomes was defined as z-score > +1.96 or < −1.96, based on findings in our MBW healthy control cohort (n = 69) and published GLI spirometry equations (Citation20). Abnormality for oscillometry was defined as values > 97.5th percentile from findings in the corresponding healthy control cohort (n = 146). Differing ways to define ULN were used since data were distributed in different ways in our healthy cohorts: MBW data demonstrated a normal distribution while IOS data were non-normally distributed with a clear heteroscedastic distribution when correlated to height, and therefore quantile regression analysis was used to identify the 97.5th percentile. The 97.5th percentile was used since it corresponds to a z-score value of +1.96. SAD was defined for each test (spirometry, MBW and IOS) as an abnormal value, based on the upper or lower limit of normal of health reference data. A p-value <0.05 (two-tailed) was regarded as statistically significant. AX was reported as its square root (SqAX) to avoid any amplification of its effect given both X5 and Fres (which contribute to AX) increase as peripheral airway resistance increases. This approach has been used before in the literature (Citation22,Citation23). Statistical analyses were performed using SPSS Statistics (v27, IBM, Armonk, United States).
Results
Fifty-seven children were recruited from the outpatient children asthma clinic at Skaraborg Hospital over a seven-month period. Demographic data are summarized in . Boys were over-represented (40/57, 70%) in the cohort and 65% were at ≥ step 3 of GINA management and 40% were inadequately controlled (ACT-score <20). Comparing females to males, there were a greater proportion of females with inadequate asthma control (59 vs. 33% of males), while other demographic data were similar. All children completed the full study protocol, apart from IOS in one child due to technical issues.
Table 1. Demographic data in the entire asthma cohort and based on gender.
Derived reference values for MBW and oscillometry
A detailed description of the two locally collected healthy control cohorts are presented in supplementary file. SF6 MBW: The definition of MBW abnormality was based on findings from 69 healthy children (35 females). Gender did not have any significant effect on any of the derived MBW indices. A bivariate correlation matrix was constructed to identify potential predictors, including, age, weight, height, and BMI (body mass index). No significant correlation was found between anthropometric data and any MBW index. Predicted values for MBW were reported as the mean value in the healthy cohort (). Upper limit of normal (ULN) and lower limit of normal (LLN) were defined as mean ± 1.96 standard deviation (SD), as shown in Figures E1–E4 in supplementary file.
Table 2. Reference values for sulfur hexafluoride (SF6) multiple breath washout (MBW) and impulse oscillometry (IOS) outcomes derived from two separate healthy cohorts.
IOS: The definition of IOS abnormality was based on findings from 146 healthy children (69 females). None of the reported IOS indices (R5, R20, R5-R20, AX and SqAX) significantly differed between boys and girls. The strongest correlation with potential predictors (i.e., weight, height, BMI, and age) was observed for height across all IOS outcomes (Spearman’s ρ ranging from −0.73 for R20 to −0.85 for R5, p < 0.001 for all). Therefore, height was used as a predictor for IOS outcomes. IOS outcomes were treated as non-parametric data, and quantile regression was used to derive the predicted median and 97.5th percentile for IOS outcomes, the equations are given in .
Lung function, airway inflammation and bronchial hyper-responsiveness
Lung function and proportion of abnormality in each index, are summarized in and . Increased FENO, indicating type 2 airway inflammation, occurred in 42% of the subjects: median (range) FENO 14 (4 to 208) ppb. Greatest rates of abnormality for spirometry indices were 44% for FEF25–75, 33% for FEV1/FVC ratio, 18% for FEV1, but all had normal FVC. A significant FEV1 reversibility occurred in 53%. BHR was present in 43% (females 71% versus males 31%, p = 0.09) and improvement with BD following DACh occurred in 48%. Maximum FEV1 fall after DACh was, 8.1% (−1.0 to 54.6%), median (range).
Figure 1. Prevalence of abnormal lung function outcomes in a cohort of 57 children aged 8–18 years with asthma.
R5-R20, resistance at 5 minus 20 Hz. SqAX, square root of area under the reactance curve. FEF25–75, forced expiratory flow between 25 and 75% of FVC. FEV1, forced expiratory volume in one second. FVC, forced vital capacity. FEF75, forced expiratory flow at 75% of FVC. R5, resistance at 5 Hz. LCI, lung clearance index.
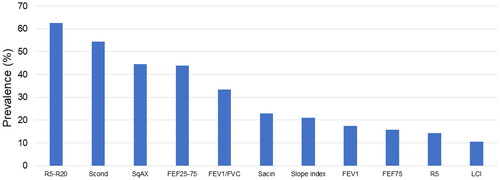
Table 3. Lung function outcomes and proportion (percentage) with abnormal findings in 57 school-aged children with asthma.
Prevalence of SAD as assessed by IOS and MBW
For IOS, R5-R20 was abnormal in a greater proportion (63%) than any spirometry index. SqAX (45%) was abnormal in a similar proportion to FEF25–75, whilst much lower rates of abnormality occurred for R5 (14%). Two thirds had an abnormality in ≥1 IOS index. As with R5-R20, Scond (54%) was abnormal in a greater proportion of subjects than any spirometry index, followed by Sacin (23%), SI (21%) and finally LCI (11%). Sixty-one percent had an abnormality in ≥1 MBW index. SAD as defined by IOS and/or MBW abnormality, occurred in 77%.
Associations between lung function and clinical characteristics
No IOS or MBW index correlated with current asthma control (ACT). Scond significantly correlated with all remaining clinical markers of disease (): with FENO (ρ 0.39, p < 0.01), medication score (ρ 0.41, p < 0.01), baseline FEV1 (ρ −0.42, p < 0.001), FEV1 reversibility (ρ 0.67, p < 0.001), and maximum FEV1 fall after DACh (ρ 0.43, p < 0.001). LCI and SI demonstrated similar correlations, apart from the maximum FEV1 fall after DACh, whilst Sacin additionally failed to correlate with FENO. R5, R5-R20, and SqAX correlated significantly with medication score, baseline FEV1 and FEV1 reversibility, with strongest correlations between R5 and baseline FEV1 (ρ −0.65, p < 0.001) and between R5-R20 and SqAX versus baseline FEV1 (ρ −0.60, p < 0.001). FEF25–75, correlated with ACT score (ρ 0.30, p < 0.05), medication score (ρ −0.37, p < 0.01), and both baseline FEV1 and FEV1 reversibility (ρ 0.87 and ρ −0.66, p < 0.001 for both).
Characteristics of subjects with SAD
The clinical characteristics of subjects with and without abnormalities in specific lung function indices are summarized in Table E3. Abnormality in IOS indices was associated only with larger FEV1 reversibility. In contrast, MBW indices were associated with many clinical outcomes, where abnormal Scond were associated with a higher FENO, higher medication score, increased FEV1 reversibility and increased airway response to DACh. Raised Sacin was the only lung function outcome associated with worse asthma control (i.e., lower ACT-score).
Discordance between oscillometry and MBW
All IOS indices (%predicted) were significantly correlated with each other (ρ ≥ 0.7, p < 0.001), with the strongest correlation between R5-R20 and SqAX (ρ 0.89, p < 0.001). Among MBW indices (z-scores), the correlations were weaker (ρ 0.23 to −0.59) but statistically significant (p < 0.01), except for Scond versus Sacin (ρ 0.23, p = 0.087), Table E4. Among MBW indices, Scond showed strongest correlations with IOS indices (ρ 0.42 to 0.47, p ≤ 0.001). In 71% of the subjects R5-R20 and Scond findings were concordant (i.e., both abnormal or both normal).
Discussion
This is, to the best of our knowledge, the most comprehensive assessment of SAD in a cohort of pediatric school-age asthma subjects performed to date. In contrast to current evidence in adult asthma, rates of abnormality in both oscillometry and MBW exceeded those detected by FEF25–75 from spirometry. In almost 80% of childhood asthmatics, there was evidence of SAD, as detected by abnormality in either oscillometry and/or MBW. Whilst R5-R20 detected greater rates of abnormality, Scond showed the strongest and greatest number of associations with clinically important characteristics. Discordance between oscillometry and MBW indices suggested these tools are complementary tools, highlighting the importance of including both in the assessment of SAD in pediatric asthma.
R5-R20, derived from IOS, and Scond, from MBW, had the highest rates of abnormal findings, outperforming other indices, including FEF25–75 from spirometry which was abnormal in only 44%. This rate of FEF25–75 abnormality was almost identical to that reported within a recent retrospective analysis of pediatric asthma subjects attending a tertiary referral clinic (Citation6), although it is important to note that we used a z-score cut off of < −1.96 to be consistent with the approaches used for MBW and IOS, in contrast to Hopp et al that used a z-score cut off of < −1.65. For the benefit of cohort comparison, in our cohort applying this < −1.65 threshold increased the rate of abnormality in FEF25–75 to 56%, suggesting that our cohort contained a higher incidence of FEF25–75 detected SAD than the Hopp et al cohort. By contrast, SAD as detected by abnormality in oscillometry and/or MBW occurred in 77% of subjects. The prevalence of abnormal Scond and R5-R20 were substantially higher than that of LCI and R5 in this cohort, which are the most commonly reported MBW and oscillometry indices, respectively, in previous pediatric studies examining detection of SAD. Moreover, abnormality in LCI and R5 were outperformed by many, if not all, indices for that tool. Discordance in abnormality classification between R5-R20 and Scond (≈30% of subjects) suggests these indices reflect different aspects of peripheral airway dysfunction.
R5-R20, Scond and FEF25–75 significantly correlated with medication score, baseline FEV1 and FEV1 reversibility. In addition, Scond correlated with both airway inflammation and airway BHR. In parallel, abnormal Scond was associated with increased FENO, increased medication score, increased FEV1 reversibility and increased BHR. In contrast, abnormal R5-R20 was only associated with increased FEV1 reversibility. Finally, asthma control correlated with FEF25–75 and was worse in children with raised Sacin from MBW.
Comparison to previous literature of MBW and oscillometry in pediatric asthma
Several findings were consistent with existing studies focusing on one of the two tools. In our cohort of predominantly mild to moderate asthmatics, LCI was normal in almost 90% of subjects, in agreeance with previous studies of mild asthma (Citation24,Citation25). LCI was more frequently abnormal in severe asthma (Citation26–28). The greater rates of abnormality of Scond, compared to LCI, is consistent with previous reports describing better discrimination between asthma and healthy controls (Citation29,Citation30). The reason for the lack of consensus regarding correlation between MBW indices and asthma control in the literature remains unclear: higher LCI (Citation31) and Scond (Citation8,Citation29) have been reported in uncontrolled asthma, whilst abnormal Sacin was the only MBW lung function outcome accompanied by worse asthma control in our study. IOS indices have previously outperformed spirometry indices such as FEF25–75 in the literature, in detecting asthma control (Citation26,Citation32), and predicting future loss of asthma control (Citation33), and future lung function impairment (Citation34). However, this was not the case in our study, where FEF25–75 was the only SAD measure that significantly correlated with asthma control score, although demonstrating a weak correlation (ρ 0.30, p < 0.05).
Our study provides the most complete assessment of pediatric asthma during clinical stability, due to limitations to recruitment of previous cohorts, where these two tools were compared. Knihtilä et al. (Citation31) did not specify doctor diagnosis of asthma in their cohort: the authors described differing R5, R5-R20 and AX derived from IOS between healthy controls and 5–10-year-olds with mild asthma symptoms, but no MBW index (LCI, Scond or Sacin) differed between the groups. Smith et al. (Citation8) enriched their cohort with a large proportion of subjects with severe asthma (65% of the cohort), and found that FEV1 was the most useful tool to detect asthma control. Wawszczak et al. (Citation35) included subjects during asthma exacerbation (48% of the cohort), and reported raised LCI, Scond, Sacin, R5-R20 and AX in these subjects, compared to the stable asthma group.
Comparison to detection of small airway dysfunction within adult asthma
There is no current gold-standard definition of SAD. In the ATLANTIS study (Citation5) in adult asthma the optimal combination of biomarkers, physiological tests, and imaging markers to measure SAD is comprehensively studied. They reported FEF25–75 abnormality in 68%. Our data presented here is important as it shows the disparity between adult and pediatric asthma, well recognized across many other aspects of asthma assessment. In the ATLANTIS study, R5-R20 and Scond detected 42% and 30% abnormality, respectively, far less than FEF25–75, in contrast to our pediatric data where both significantly outperformed FEF25–75. This supports the ongoing use of these tools in the assessment of pediatric SAD, as proposed by two world leading asthma and allergy organizations in a recent joint manifesto (Citation3). Oscillometry is more widely used in current lung function laboratories, with IOS incorporated into existing equipment for conventional lung function testing. MBW is more restricted in its availability but the greater correlation of Scond with clinical outcomes in our cohort, in comparison to R5-R20, supports an ongoing role in research into the importance of SAD in this setting.
Ability of MBW and IOS indices to reflect SAD
Since no “gold standard” of SAD exists, it is worth revisiting the evidence that MBW and oscillometry reflect SAD. Patchiness of disease distribution in asthmatic lungs has been demonstrated in both modeling (Citation36) and imaging studies (Citation37), with extent correlated to disease severity and medication burden (Citation38). Modeling studies demonstrated that these changes require small airway obstruction (Citation39) and altered small airway behavior (Citation36). Functional CT imaging work demonstrated penetration of 5 Hz oscillometry signals into less ventilated regions, whilst 20 Hz signals travel to better-ventilated regions (Citation40), supporting R5-R20 utility in this setting, but also suggesting that it primarily may reflect better ventilated lung regions. The low prevalence of abnormal LCI in our study may relate to the fact that it reflects differences in specific ventilation among lung units, but is not sensitive to the sequential filling and emptying, generated by differences in time constants among such lung units, reflected by Scond (Citation41). The higher sensitivity of Slope index, abnormal in twice as many subjects as LCI, may reflect the fact that in its calculation it divides the washout curve into components reflecting a theoretical fast and slow ventilated compartment (Citation17), which better reflects the mechanism behind Scond. For these reasons, we consider MBW indices such as Scond to be more truly reflective of non-uniform distribution of peripheral airway narrowing/obstruction, and may be the more clinically informative index, given its stronger and wider range of correlation than R5-R20 to other clinical asthma measures within our cohort.
Sacin reflects ventilation inhomogeneity arising within the entrance of the acini and structural asymmetries in these distal lung units (Citation42). Abnormal Sacin occurred in 23% of the subjects and was the only IOS and MBW measure associated with uncontrolled asthma. Higher Sacin has been reported during asthma exacerbation (Citation35). Prevalence of Sacin abnormality was higher in our previously published adult asthma cohort (42%) (Citation22), and occurred predominantly in those with a positive smoking history. Within the large adult multi-center ATLANTIS study (Citation5), Sacin was abnormal in 41% at GINA step 5 therapy, and correlated with exacerbation frequency, but not ACT. Taken together, these results suggest that Sacin abnormality may be clinically relevant and potentially progress over time, although longitudinal studies are needed to confirm this. We hypothesize that initial patchy distribution of disease within conducting airways (driving raised Scond) gradually involves larger parts of the lung with disease progression, such that Sacin abnormality appears later on in disease progression, as Sacin is largely determined by the phase III slope of the first washout breath which is weighted to the contribution of the best-ventilated lung units.
Strengths and limitation
The major strengths of this study are: (1) use of both MBW and oscillometry, including the gold standard MBW method for gas signal analysis (respiratory mass spectrometry) and (2) definition of limits of normal determined by contemporaneous equipment-specific reference data. In the supplementary file, raw data plots are provided to support our approach to defining upper limits of normal across an age range where normal lung function may vary. However, the study has limitations. We did not incorporate an CT imaging-based assessment of structural changes of SAD, and the exact locations of pathology within the small airways that abnormalities in FEF25–75, MBW and IOS reflect are not known. Ongoing efforts to understand this using advanced modeling techniques will hopefully provide further insight (Citation43,Citation44). For pediatric studies this reflect concerns about radiation exposure during this critical age range for development, and therefore the utility of tests without associated radiation is important to know. There also remains challenges with applying this imaging based “gold standard” tool due to the ongoing debate about where true thresholds lie for defining abnormal gas trapping as a marker of SAD (Citation45). In addition, in other obstructive lung conditions, such as Cystic Fibrosis, MBW has been shown to have much stronger correlation with structural changes on CT, in comparison to spirometry (Citation46), supporting its use in situations where concerns regarding CT use exist. We withheld asthma medications for 12 h prior to lung function assessment at each visit. The exact time that medication is withheld prior to testing may vary across studies and how that affects rates of abnormality remains unclear.
In summary
This study outlines important differences in SAD between adult and pediatric asthma. Complimentary information was provided by peripheral airway function indices of oscillometry and MBW, in school-age children across a wide range of asthma severity, and outperformed FEF25–75, recently recommended based on adult data to detect SAD in preference to these tools. In this, the most extensive assessment of pediatric SAD to date, SAD occurred in almost 80% of subjects and was best detected by R5-R20 (from oscillometry) and Scond (from MBW). Scond, due to its stronger association with clinical features of asthma seems more informative than oscillometry-derived R5-R20. This supports the specific proposal by two world leading asthma and allergy organizations in a recent joint manifesto for tools such as MBW and oscillometry to be used to confirm the presence of SAD (Citation3), and for physicians to recognize this as concerning when present. More effective targeting of small airways in childhood asthma may offer ability to improve disease progression, given the lower prevalence of Sacin abnormality in our pediatric cohort compared to adult cohorts. Commercial equipment for MBW and oscillometry is increasingly available, and it is important for future work to confirm the findings of this study in other pediatric asthmatic cohorts. Longitudinal studies, including these additional indices, are required to better understand the evolution of SAD in asthma and whether targeted interventions may improve long-term asthma outcomes.
Key findings
In school-aged asthma, multiple breath washout (MBW) derived Scond, and oscillometry derived R5-R20 are superior to spirometry derived FEF25–75 in detecting small airway dysfunction. Scond correlated with the highest number of clinical asthma outcomes, including airway inflammation and bronchial hyper-responsiveness.
Supplemental Material
Download MS Word (513.5 KB)Acknowledgments
We wish to express our gratitude to associate Professor Per Gustafsson at department of Pediatrics, Skaraborg Central Hospital, Sweden for assistance with study design, conducting the study and being an advisor in the process of data analysis. We also want to thank all children and parents participating in the study.
Declaration of interest
SK has been hired as a lecturer by Intramedic AB. ACO is a shareholder and board member of PExA AB (www.PEXA.se). No conflicts of interest are disclosed by any of the other authors.
Additional information
Funding
References
- Hamid Q, Song Y, Kotsimbos TC, Minshall E, Bai TR, Hegele RG, et al. Inflammation of small airways in asthma. J Allergy Clin Immunol. 1997;100(1):44–51.
- Wenzel SE, Szefler SJ, Leung DY, Sloan SI, Rex MD, Martin RJ. Bronchoscopic evaluation of severe asthma. Persistent inflammation associated with high dose glucocorticoids. Am J Respir Crit Care Med. 1997;156(3 Pt 1):737–743. doi:10.1164/ajrccm.156.3.9610046.
- Braido F, Scichilone N, Lavorini F, Usmani OS, Dubuske L, Boulet LP, Mosges R, Nunes C, Sánchez-Borges M, Ansotegui IJ, et al. Manifesto on small airway involvement and management in asthma and chronic obstructive pulmonary disease: an Interasma (Global Asthma Association – GAA) and World Allergy Organization (WAO) document endorsed by Allergic Rhinitis and its Impact on Asthma (ARIA) and Global Allergy and Asthma European Network (GA2LEN). Asthma Res Pract. 2016;2:12. doi:10.1186/s40733-016-0027-5.
- Woolcock AJ, Vincent NJ, Macklem PT. Frequency dependence of compliance as a test for obstruction in the small airways. J Clin Invest. 1969;48(6):1097–1106. doi:10.1172/JCI106066.
- Postma DS, Brightling C, Baldi S, Van den Berge M, Fabbri LM, Gagnatelli A, et al. Exploring the relevance and extent of small airways dysfunction in asthma (ATLANTIS): baseline data from a prospective cohort study. Lancet Respir Med. 2019;7(5):402–416.
- Hopp R, Lee J, Bohan H. An a priori approach to small airway dysfunction in pediatric asthmatics. Children (Basel). 2022;9(10):1454. doi:10.3390/children9101454.
- Hopp RJ, Wilson MC, Pasha MA. Small airway disease in pediatric asthma: the who, what, when, where, why, and how to remediate. a review and commentary. Clin Rev Allergy Immunol. 2022;62(1):145–159. doi:10.1007/s12016-020-08818-1.
- Smith CJ, Spaeder MC, Sorkness RL, Teague WG. Disparate diagnostic accuracy of lung function tests as predictors of poor asthma control in children. J Asthma. 2020;57(3):327–334. doi:10.1080/02770903.2019.1566471.
- Tirakitsoontorn P, Crookes M, Fregeau W, Pabelonio N, Morphew T, Shin H-W, Galant SP. Recognition of the peripheral airway impairment phenotype in children with well-controlled asthma. Ann Allergy Asthma Immunol. 2018;121(6):692–698. doi:10.1016/j.anai.2018.08.023.
- Chang DV, Teper A, Balinotti J, Castro Simonelli C, Garcia-Bournissen F, Kofman C. Exhaled nitric oxide predicts loss of asthma control in children after inhaled corticosteroids withdrawal. Pediatr Pulmonol. 2019;54(5):537–543. doi:10.1002/ppul.24268.
- Sears MR, Greene JM, Willan AR, Wiecek EM, Taylor DR, Flannery EM, Cowan JO, Herbison GP, Silva PA, Poulton R, et al. A longitudinal, population-based, cohort study of childhood asthma followed to adulthood. N Engl J Med. 2003;349(15):1414–1422. doi:10.1056/NEJMoa022363.
- Arıkoglu T, Unlu A, Yıldırım DD, Kuyucu S. The airway hyperresponsiveness to methacholine may be predicted by impulse oscillometry and plethysmography in children with well-controlled asthma. J Asthma. 2018;55(11):1166–1173. doi:10.1080/02770903.2017.1407337.
- Liu AH, Zeiger R, Sorkness C, Mahr T, Ostrom N, Burgess S, Rosenzweig JC, Manjunath R. Development and cross-sectional validation of the Childhood Asthma Control Test. J Allergy Clin Immunol. 2007;119(4):817–825. doi:10.1016/j.jaci.2006.12.662.
- Nathan RA, Sorkness CA, Kosinski M, Schatz M, Li JT, Marcus P, et al. Development of the asthma control test: a survey for assessing asthma control. J Allergy Clin Immunol. 2004;113(1):59–65.
- Baraldi E, de Jongste JC. Measurement of exhaled nitric oxide in children, 2001. Eur Respir J. 2002;20(1):223–237. doi:10.1183/09031936.02.00293102.
- Dweik RA, Boggs PB, Erzurum SC, Irvin CG, Leigh MW, Lundberg JO, Olin A-C, Plummer AL, Taylor DR. An official ATS clinical practice guideline: interpretation of exhaled nitric oxide levels (FENO) for clinical applications. Am J Respir Crit Care Med. 2011;184(5):602–615. doi:10.1164/rccm.9120-11ST.
- Robinson PD, Latzin P, Verbanck S, Hall GL, Horsley A, Gappa M, Thamrin C, Arets HGM, Aurora P, Fuchs SI, et al. Consensus statement for inert gas washout measurement using multiple- and single- breath tests. Eur Respir J. 2013;41(3):507–522. doi:10.1183/09031936.00069712.
- Oostveen E, MacLeod D, Lorino H, Farre R, Hantos Z, Desager K, et al. The forced oscillation technique in clinical practice: methodology, recommendations and future developments. Eur Respir J. 2003;22(6):1026–1041.
- Miller MR, Hankinson J, Brusasco V, Burgos F, Casaburi R, Coates A, et al. Standardisation of spirometry. Eur Respir J. 2005;26(2):319–338.
- Quanjer PH, Stanojevic S, Cole TJ, Baur X, Hall GL, Culver BH, Enright PL, Hankinson JL, Ip MSM, Zheng J, et al. Multi-ethnic reference values for spirometry for the 3-95-yr age range: the global lung function 2012 equations. Eur Respir J. 2012;40(6):1324–1343. doi:10.1183/09031936.00080312.
- Parsons JP, Hallstrand TS, Mastronarde JG, Kaminsky DA, Rundell KW, Hull JH, Storms WW, Weiler JM, Cheek FM, Wilson KC, et al. An official American Thoracic Society clinical practice guideline: exercise-induced bronchoconstriction. Am J Respir Crit Care Med. 2013;187(9):1016–1027. doi:10.1164/rccm.201303-0437ST.
- Kjellberg S, Houltz BK, Zetterström O, Robinson PD, Gustafsson PM. Clinical characteristics of adult asthma associated with small airway dysfunction. Respir Med. 2016;117:92–102. doi:10.1016/j.rmed.2016.05.028.
- Schultz ES, Hallberg J, Gustafsson PM, Bottai M, Bellander T, Bergström A, Kull I, Gruzieva O, Thunqvist P, Pershagen G, et al. Early life exposure to traffic-related air pollution and lung function in adolescence assessed with impulse oscillometry. J Allergy Clin Immunol. 2016;138(3):930–932.e5. doi:10.1016/j.jaci.2016.04.014.
- Steinbacher M, Pfleger A, Schwantzer G, Jauk S, Weinhandl E, Eber E. Small airway function before and after cold dry air challenge in pediatric asthma patients during remission. Pediatr Pulmonol. 2017;52(7):873–879. doi:10.1002/ppul.23724.
- Zwitserloot A, Fuchs SI, Müller C, Bisdorf K, Gappa M. Clinical application of inert gas Multiple Breath Washout in children and adolescents with asthma. Respir Med. 2014;108(9):1254–1259. doi:10.1016/j.rmed.2014.07.003.
- de Gouveia Belinelo P, Nielsen A, Goddard B, Platt L, Da Silva Sena CR, Robinson PD, Whitehead B, Hilton J, Gulliver T, Roddick L, et al. Clinical and lung function outcomes in a cohort of children with severe asthma. BMC Pulm Med. 2020;20(1):66. doi:10.1186/s12890-020-1101-6.
- Nuttall AG, Beardsmore CS, Gaillard EA. Ventilation heterogeneity in children with severe asthma. Eur J Pediatr. 2021;180(11):3399–3404. doi:10.1007/s00431-021-04101-3.
- Irving S, Fleming L, Ahmad F, Biggart E, Bingham Y, Cook J, Hall P, Jamalzadeh A, Nagakumar P, Bossley C, et al. Lung clearance index and steroid response in pediatric severe asthma. Pediatr Pulmonol. 2020;55(4):890–898. doi:10.1002/ppul.24675.
- Keen C, Olin AC, Wennergren G, Gustafsson P. Small airway function, exhaled NO and airway hyper-responsiveness in paediatric asthma. Respir Med. 2011;105(10):1476–1484. doi:10.1016/j.rmed.2011.04.004.
- Vilmann L, Buchvald F, Green K, Nielsen KG. Fractional exhaled nitric oxide and multiple breath nitrogen washout in preschool healthy and asthmatic children. Respir Med. 2017;133:42–47. doi:10.1016/j.rmed.2017.10.027.
- Knihtilä H, Kotaniemi-Syrjänen A, Pelkonen AS, Mäkelä MJ, Malmberg LP. Small airway function in children with mild to moderate asthmatic symptoms. Ann Allergy Asthma Immunol. 2018;121(4):451–457. doi:10.1016/j.anai.2018.07.026.
- Shi Y, Aledia AS, Tatavoosian AV, Vijayalakshmi S, Galant SP, George SC. Relating small airways to asthma control by using impulse oscillometry in children. J Allergy Clin Immunol. 2012;129(3):671–678. doi:10.1016/j.jaci.2011.11.002.
- Shi Y, Aledia AS, Galant SP, George SC. Peripheral airway impairment measured by oscillometry predicts loss of asthma control in children. J Allergy Clin Immunol. 2013;131(3):718–723. doi:10.1016/j.jaci.2012.09.022.
- Knihtilä H, Kotaniemi-Syrjänen A, Mäkelä MJ, Bondestam J, Pelkonen AS, Malmberg LP. Preschool oscillometry and lung function at adolescence in asthmatic children. Pediatr Pulmonol. 2015;50(12):1205–1213. doi:10.1002/ppul.23188.
- Wawszczak M, Kulus M, Peradzyńska J. Peripheral airways involvement in children with asthma exacerbation. Clin Respir J. 2021;16(2):97–104. doi:10.1111/crj.13456.
- Venegas JG, Winkler T, Musch G, Vidal Melo MF, Layfield D, Tgavalekos N, Fischman AJ, Callahan RJ, Bellani G, Harris RS, et al. Self-organized patchiness in asthma as a prelude to catastrophic shifts. Nature. 2005;434(7034):777–782. doi:10.1038/nature03490.
- Cadman RV, Lemanske RF, Evans MD, Jackson DJ, Gern JE, Sorkness RL, Fain SB. Pulmonary 3He magnetic resonance imaging of childhood asthma. J Allergy Clin Immunol. 2013;131(2):369–376.e1-5. doi:10.1016/j.jaci.2012.10.032.
- Altes TA, Mugler JP, Ruppert K, Tustison NJ, Gersbach J, Szentpetery S, Meyer CH, de Lange EE, Teague WG. Clinical correlates of lung ventilation defects in asthmatic children. J Allergy Clin Immunol. 2016;137(3):789–796.e7. doi:10.1016/j.jaci.2015.08.045.
- Campana L, Kenyon J, Zhalehdoust-Sani S, Tzeng Y-S, Sun Y, Albert M, Lutchen KR. Probing airway conditions governing ventilation defects in asthma via hyperpolarized MRI image functional modeling. J Appl Physiol (1985). 2009;106(4):1293–1300. doi:10.1152/japplphysiol.91428.2008.
- Bell AJ, Foy BH, Richardson M, Singapuri A, Mirkes E, van den Berge M, Kay D, Brightling C, Gorban AN, Galbán CJ, et al. Functional CT imaging for identification of the spatial determinants of small-airways disease in adults with asthma. J Allergy Clin Immunol. 2019;144(1):83–93. doi:10.1016/j.jaci.2019.01.014.
- Robinson PD, Goldman MD, Gustafsson PM. Inert gas washout: theoretical background and clinical utility in respiratory disease. Respiration. 2009;78(3):339–355. doi:10.1159/000225373.
- Verbanck S, Paiva M. Model simulations of gas mixing and ventilation distribution in the human lung. J Appl Physiol (1985). 1990;69(6):2269–2279. doi:10.1152/jappl.1990.69.6.2269.
- Foy B, Kay D, Siddiqui S, Brightling C, Paiva M, Verbanck S. Increased ventilation heterogeneity in asthma can be attributed to proximal bronchioles. Eur Respir J. 2020;55(3):1901345. doi:10.1183/13993003.01345-2019.
- Foy BH, Soares M, Bordas R, Richardson M, Bell A, Singapuri A, Hargadon B, Brightling C, Burrowes K, Kay D, et al. Lung computational models and the role of the small airways in asthma. Am J Respir Crit Care Med. 2019;200(8):982–991. doi:10.1164/rccm.201812-2322OC.
- Bayfield KJ, Weinheimer O, Boyton C, Fitzpatrick R, Middleton A, Kennedy B, Blaxland A, Jayasuriya G, Caplain N, Issa H, et al. Implementation and evaluation of ultra-low dose CT in early cystic fibrosis lung disease. Eur Respir J. 2023;62(1):2300286. doi:10.1183/13993003.00286-2023.
- Gustafsson PM, De Jong PA, Tiddens HA, Lindblad A. Multiple-breath inert gas washout and spirometry versus structural lung disease in cystic fibrosis. Thorax. 2008;63(2):129–134. doi:10.1136/thx.2007.077784.