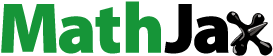
Abstract
In this study, the manners in which temperature (45–77 °C) and the addition of xylan (5 g/95 g lignin) influence the onset of precipitation and evolution of the particle size distribution during acid precipitation of softwood kraft lignin were investigated in situ. No systematic trend between the onset pH of precipitation and the temperature or the addition of xylan could be observed at these conditions: the average onset pH was found to be 9.3. However, the size of the agglomerates increased as the temperature was increased, but added xylan rendered a decrease in agglomerate size. A higher onset pH was measured at increased Na ion concentration. The results indicate that the ionization degree of the phenolic groups influences the precipitation at 1 M Na ions but it is also probable that the degree of ionization of the carboxylic groups (on kraft lignin and xylan) influences precipitation (particle numbers and sizes).
INTRODUCTION
Dependent on the species, around 15–30% of wood tissue consists of lignin: an aromatic macromolecule comprised of phenyl propane units that are cross-linked as the wood tissue is being formed. Many different chemical bonds can be found in native lignin, with the most abundant being the β–O–4 linkage (about 50–60%). It is mainly this linkage between the phenyl propane units that is cleaved by the reaction that occurs with the cooking chemicals during cooking in the kraft process for pulp production, and results in the formation of smaller kraft lignin fragments. Cleavage of the β–O–4 linkage also results in the formation of phenolic groups, which are deprotonated at alkaline conditions in the cooking process: the ionized phenolic groups cause the lignin fragments to dissolve and be transported out of the wood cell wall by diffusion. The remaining pulp is washed, yielding a washed pulp and black liquor: the latter contains not only the dissolved kraft lignin extracted from the wood tissue, but also polymeric and residual forms of xylan, degraded products from galactoglucomannans and inorganic cooking chemicals. In the present-day kraft pulp mills, the black liquor is combusted in order to recover the cooking chemicals as well as to produce electricity and steam for both internal and external use. Extracting lignin from the black liquor is a first step in converting the conventional kraft pulp mill into an integrated biorefinery and pulp mill. Two pulp mills (Domtar in Plymouth, USA and Stora Enso in Sunila, Finland) have recently installed the commercial LignoBoost™ technology,[Citation1,Citation2] enabling the extraction of high-purity kraft lignin. Today, a total of 0.1 Mt kraft lignin is produced annually worldwide by different extraction processes. However, the total annual production of kraft lignin worldwide is 50–60 Mt which means that there is a potential of considerably more being recovered as a material stream. Extracted kraft lignin can serve as a precursor in the production of high value products such as carbon fibers, adhesives and bio-oils, which all are under development.[Citation3–7]
It is well known that the ionization of phenolic groups plays a major role in the solubility of kraft lignin at alkaline pH. The apparent pKa value of lignin is a function of several parameters such as the chemical substitution pattern on the phenolic aromatic ring, temperature and solution conditions. The pKa value has been measured to be 10.5 (21 °C) for Indulin AT softwood kraft lignin.[Citation8] A study of a large number of different phenolic compounds related to kraft lignin, however, showed pKa values ranging from 6 to 11,[Citation9] indicating that the acidity of different phenolic alcohols in kraft lignin may potentially be rather diverse. Although the phenolic groups contribute most to the ionizable groups on lignin, with contents of about 2–5 mmol/g, low contents of carboxylic groups can also be found of around 0.1–0.8 mmol/g.[Citation10–14] It has been shown that the precipitation of kraft lignin is sensitive to pH at low concentrations of salt even in the acidic range, and the suggestion was made that this is due to the carboxylic groups on the kraft lignin molecules.[Citation15,Citation16] The pKa value for carboxylic groups on lignin has been reported to be around 5.5.[Citation17]
Fragments of xylan are present in black liquor and a previous study on the filtration of hardwood kraft lignin with different amounts of xylan showed that the presence of xylan had a negative influence on filtration after precipitation performed by lowering the pH.[Citation18] A recent work has confirmed this particular influence of xylan; it was suggested that the xylan co-precipitates with the lignin and forms agglomerates.[Citation19] Both softwood and hardwood xylan have glucuronic acid side groups, with a pKa of about 3.4,[Citation17] that will be ionized at alkaline and neutral conditions and thus influence the solubility of xylan. It is possible that the properties of the lignin agglomerates containing xylan are also influenced. The way in which xylan co-precipitates with lignin, and at which conditions, may be critical for controlling the performance of the separation process with respect to the purity and filterability of the material.
Despite the need for detailed understanding of the solubility and precipitation of kraft lignin in black liquor, not many papers can be found in the literature that investigate this with the aim of understanding the mechanisms involved. The subject has, nevertheless, been studied for several decades, with the focus of early work being placed mostly on the yield. It was found, among many things, that the yield in acid precipitation of kraft lignin from black liquors is dependent on the pH.[Citation20,Citation21]
In rather recent studies, the yield of precipitation from softwood black liquors has been studied; the influence of temperature, pH and the addition of salt,[Citation22] along with the molecular weight, were investigated using fractionated black liquors (24–38% total dry solids).[Citation23] Overall it was found that a high molecular weight, low pH, and low temperature increased the yield.
The studies discussed above were all performed using solutions (or black liquors) having a relatively high concentration of lignin and inorganic salts. In another set of investigations, the initial formation of nuclei was studied, often using dilute lignin solutions. In one such study[Citation16] with Indulin ATR kraft lignin, the critical coagulation concentration (CCC) was investigated, predominately in the neutral and acidic regimes at lignin concentrations of about 0.1 g/L. The CCC showed a strong dependency on the pH and the valency of the counterion, however, showed to be independent on the temperature in investigated range (20–60 °C).
Another study investigated the onset of aggregation of kraft lignin macromolecules (Indulin AT) as a function of NaCl concentration, temperature and pH[Citation24]. This was measured as occurring at 0.31 M NaCl, at 75 °C and a pH of about 10.1, and was independent of the lignin concentration in the range 0.26–3.3 g/L. In contrast to the earlier study,[Citation16] the CCC was measured as being temperature-dependent, decreasing as the temperature increased at dilute concentrations of lignin.
In an additional study, the precipitation process on the nano-scale was investigated (lignin concentration 0.26–3.3 g/L) at a more detailed level.[Citation25] The structure of the resulting flocs was fractal, and the fractal dimension (1.9–2.45) was shown to be dependent on the stability ratio,[Citation26,Citation27] indicating whether the precipitation mode is diffusion or reaction limited. Flocs/primary particles in the range of 100 nm to 1–2 µm were formed. The CCC shifted from 1.3 to 1.75 M NaCl at 70 °C and pH 10.5, when a large molecular weight fraction (>100 kDa) was removed from the lignin, showing that the molecular weight of the lignin is critical for its solubility. It was suggested that the high molecular weight fraction was the first to precipitate when the conditions favored precipitation: this hypothesis was strengthened further in the study carried out[Citation23] mentioned above.
The influence of agitation on the properties of agglomerates has been studied during the acid precipitation of kraft lignin from black liquors using CO2 to lower the pH to 9.8 at 75 °C.[Citation28] In general, the agglomerates became larger in size and looser in structure at lower agitation speeds during acidification, and increased further in size during aging: the lower the speed, the larger the particles. It was observed that the agglomerates were made up of rather spherical primary particles of a specific size (1–2 µm), regardless of the intensity of the agitation. It was suggested that the properties of the primary particles formed were independent of the rate of agitation, even though it was clear that agitation affected the agglomerate structures on the micron scale that were composed of the primary particles. Similar agglomerate structures, composed of primary particles of similar size, have been observed in previous work, too.[Citation29]
To date, the influence of temperature on the onset of precipitation has only been investigated for very dilute systems. The FBRM technique used in this work enables particle size distribution measurements to be made at high concentrations of lignin, thereby allowing the influence of temperature on precipitation to be investigated at lignin concentrations of technical interest. Furthermore, the size distribution of particles formed on the micron scale at different conditions is of interest for the filterability of lignin (also in the presence of xylan). In particular, in situ knowledge of the effect of temperature on the particle size distribution is, to our knowledge, currently lacking. No detailed studies can be found in the literature that monitor the precipitation process all the way from highly alkaline to acidic conditions by lowering the pH, investigating the pH sensitivity in the whole relevant pH range.
This work aims at investigating the onset of precipitation and the precipitation process in terms of the development of the particle size distribution on the micron scale, over a wide pH range and at various conditions, with the emphasis placed on the effects of temperature and the addition of xylan.
MATERIALS
The lignin used in this work was a softwood LignoBoost™ lignin sourced from a Nordic pulp mill. The molecular weight distribution of the starting lignin material was measured using gel-permeation chromatography (GPC), (PL-GPC 50 plus) equipped with an ultraviolet detector (280 nm). The mobile phase was a DMSO/LiBR mixture (10 mM LiBR) and the column was a PolarGel-M column with a guard column (300 × 7.5 mm/50 × 7.5 mm, 8 µm). A 10-point calibration curve-fit was made with standards of Pullulan (type: polysaccharide calibration kit, Varian). The weight-averaged molecular weight was determined from four runs: 11.3 ± 0.09 (kDa) with polydispersity 3.6 ± 0.03. The initial sodium content of the lignin was determined to be 0.16 ± 0.02 wt% by atomic absorption spectroscopy (Thermoscientific iCE 3000). The same batch of lignin has also been characterized elsewhere[Citation30]: the content of phenolic and carboxylic groups was found to be 4.27 mmol/g and 0.45 mmol/g, respectively. The content of residual xylan in the lignin powder used was estimated to 0.35%, based on the xylose concentration measured by high-performance anion exchange chromatography (HPAEC) after acid hydrolysis with sulfuric acid, and the procedure can be found elsewhere.[Citation31] A Dionex ICS-5000 system, equipped with CarboPacTM PA1 columns and an electrochemical detector, was employed, and the eluents were NaOH and NaOH + NaAc (0.2 M). NaOH (Sigma Aldrich, purity ≥98%) and Na2SO4 (Fisher Chemical, purity >99.5%) were used to produce the aqueous model solutions for the experiments (see the section “Experimental Procedure”), and deionized water was used throughout all preparations and experiments. The acid solutions used were prepared by diluting concentrated sulfuric acid (Scharlau, 95–97%). The source of xylan was beech xylan (Lot 141202, P-XYLNBE, Megazyme) having a glucuronic acid content of 13.0 wt% (specified by the supplier).
EXPERIMENTAL
In this work, the pH, temperature and presence of xylan on precipitation of kraft lignin has been investigated. An overview of the experimental conditions studied can be found in .
TABLE 1. Overview of the experiments conducted.
Experimental Set-up and Measurements of Particle Size Distribution
The precipitation experiments were performed in a jacketed precipitation vessel (of volume 0.5 L and inner diameter 96 mm) connected to a heating circuit. This allows the interior volume to be heated indirectly and the temperature can thus be controlled during the experiments. The top of the vessel was covered by a lid to minimize evaporation losses, but the vessel was open during the time samples of liquid were taken from the vessel. The vessel was equipped with baffles along its walls. Agitation was provided by a 45° inclined pitch-blade impeller with a diameter of 50 mm and blade width 10 mm; the blades were mounted 15 mm from the bottom of the vessel, producing a downward flow. The agitation speed was 250 rpm for all the experiments. A test was performed in order to examine how well the mixing device could distribute added acid evenly in these mixing conditions. The pH indicator phenolphthalein was added to deionized water in the precipitation vessel and the pH was adjusted to 9.7 with sulfuric acid and NaOH: the solution, which was now pink, turned completely transparent in the 5 s following the addition of 200-µL 6-M sulfuric acid, and reached a pH of 4.0. The result indicates that the mixing was good.
The Focused Beam Reflectance Measurement (FBRM®) probe was positioned at a 35° angle to the direction of the flow and toward the leading side of a baffle, yet still relatively close to the impeller blades. This position minimizes clogging of the probe window while enabling impingement of the particles in the flowing suspension on the probe tip window. The FBRM unit was a model G400 from Mettler Toledo and reports particle chord lengths in the range 1–1000 µm. The FBRM technique measures chord lengths of particles, allocating them to pre-defined chord length bins (the “primary” setting was used in this work), and reports the numbers of chord lengths in each bin as counts (#/s) during a chosen sampling period. Consequently, the chord length distribution can be measured in real-time and in situ, allowing the evolution of the chord length distribution to be monitored throughout the experiment.
Experimental Procedure
The lignin was dissolved for a minimum of 16 h in a 1 M NaOH solution. Prior to the preparation of experimental solutions the dry content of the lignin powder used was measured for each experiment. The mixture was filtered after dissolution to remove undissolved or insoluble material: less than 1.2% of the dry lignin powder mass was filtered off. Filtration was carried out using a filter funnel connected on top of a conical glass flask that, in turn, was connected to a vacuum suction. The filter paper used was a Sartorius stedim Type 184, regenerated cellulose, with a nominal pore size of 0.45 µm. The ratio of lignin to water in all the pre-filtered experimental solutions prepared was kept constant at 0.10 g lignin/g water. The concentration of lignin in the pre-filtered solutions prepared for the precipitation experiments at 1 M Na ion concentration varied between 8.8 and 8.9 wt% due to variations in the amounts of removed undissolved or insoluble material in the different experiments. Preparation of the solution for the 4 M Na ion concentration experiment (Exp. 11) included adjusting the Na ion concentration by the addition of Na2SO4 to the pre-filtered solution of 1 M Na ion solution to reach a concentration of 1.5 M Na2SO4. The lignin concentration obtained in this case was 7.5 wt%.
The pre-filtered solution, with a pH value of 13.4, was added to the precipitation vessel and the heating circuit was turned on. In the cases where xylan was to be added, the procedure was to first lower the pH to 12.6, then add the xylan and, finally, turn on the heating. This was done in order to reduce potential degradation of the xylan, yet ensure that the pH was sufficiently high to avoid any precipitation of lignin occurring prior to the addition of xylan. Xylan was added in the proportion 5 g xylan/95 g (dry) kraft lignin. 6 M sulfuric acid solution was used for the purpose of acidification, which was performed step-wise and by adding it dropwise into the precipitation vessel, with the pH being allowed to equilibrate between each addition. The pH of the liquid was measured at different times during the experiments by removing a sample of about 10 ml from the precipitation vessel. This was cooled to 25 °C and the pH measured by a pH meter of type Mettler Toledo SevenCompact™ S220 equipped with automatic temperature correction and an InLab Max Pro-ISM pH electrode (Mettler Toledo) calibrated at room temperature. After the pH measurement, the sample was reheated to the experimental temperature and returned to the vessel. No influence on the chord length distribution could be detected when the sample was returned to the vessel.
The onset of precipitation was defined as the point at which a distinct increase in counts could be observed relative to the initial count level (base line) and to which the count level did not return immediately (which may happen when a relatively large amount of acid is added but the conditions is far away from the onset conditions). Since 1 µm is the smallest chord length reported by the equipment used in this study the onset of precipitation was defined as when the equipment detects the first stable particles equal or larger than 1 µm.
ESTIMATION OF CHARGE DENSITY
It is relevant for further analysis to estimate the charge density of the lignin at the onset condition, which can be made from the ionization degree of ionizable groups and the content of those groups (phenolic and carboxylic groups). An estimate of how much the degree of ionization may have decreased at given pH (e.g., the onset pH) can be made from the mass action equation for the protonation reaction in EquationEquation (1)(1)
(1) , where the equilibrium constant,
, is expressed in terms of the activity of protons,
and the ionization degree,
, of the ionizable group of interest.[Citation32] In this case, it is the fraction of the deprotonated phenolic groups:
(1)
(1)
The temperature dependency of the equilibrium constant may be accounted for approximately by the van’t Hoff expression:
(2)
(2)
where is the enthalpy of ionization of phenolic groups, which may be assumed to be that for phenol, 20 kJ/mol.[Citation33]
is the gas constant and
is a reference temperature, here chosen to be 298 K.
The pH or activity of the protons at the actual temperature, T, can be estimated from the pH measured at 25 °C via the ionization constant for water, , in the mass action equation in EquationEquation (3)
(3)
(3) , assuming the activity of water to be 1, and using a similar van’t Hoff expression as in EquationEquation (2)
(2)
(2) to account for temperature dependency:
(3)
(3)
Further assumptions are also made: pKw is 14 at 25 °C; the enthalpy of ionization of water is 55.8 kJ/mol, as at 25 °C,[Citation34] and its temperature dependency can be neglected; the activity coefficient of the protons remains constant within the temperature range investigated (25–77 °C). The concentration of hydroxide ions may be assumed to be that at 25 °C, at any temperature in the range of interest, at alkaline conditions.[Citation8] The activity coefficient of the hydroxide ions is also assumed to be independent of temperature in the range of interest, i.e., the activity of the hydroxide ions, , remains constant in the temperature interval.
RESULTS
The Onset of Precipitation
The Onset pH
A peak indicating the formation of unstable agglomerates was observed close to the onset precipitation pH. This occurred at lower ionic strengths and higher temperatures (65 °C and 77 °C), both with and without the addition of xylan; an example of this can be found in . Thus, agglomerates are formed that disintegrate rather quickly into small particles (size <1 µm) and/or re-dissolve. It was also found that this course of events was faster at 77 °C than 65 °C. In this pH range, the solubility is obviously controlled by the protonation/deprotonation of the phenolic groups. In the present study, acid of high concentration was added dropwise, with the consequence that, for a very short period of time, the local pH was below/far below the onset pH. This made it possible to obtain an initial local precipitation, because agglomerates became unstable as the pH gradient evened out due to agitation and the agglomerates disintegrate/re-dissolve. In order to verify that the reduction in counts was due to an actual decrease in concentration and not to changes in the light-scattering properties at the specific conditions, samples were taken at different times, i.e., prior to the peak, at the peak and after it. Filtering the samples showed that the mass of the particles collected was in approximate agreement with the trends observed by the FBRM measurements. Further experiments are, however, necessary in order to obtain a more detailed understanding of this phenomenon.
Figure 1 The onset of precipitation, and the formation and disintegration of particles that follow (Exp. 8).
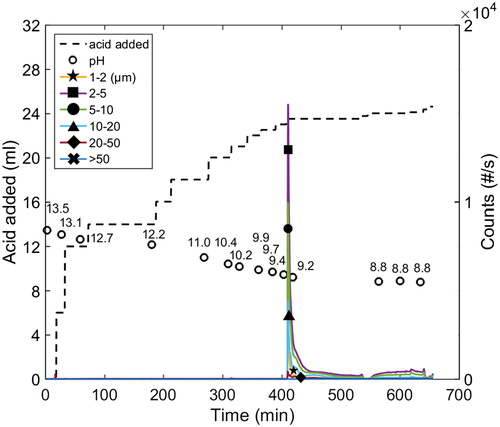
As mentioned in the section “Experimental Procedure,” the onset of precipitation in this study is defined as the point when stable particles/agglomerates equal or larger than 1 µm are formed. However, it should be mentioned here that nano-sized lignin particles may in fact be formed at higher pH values than those measured here and that the FBRM unit is not able to detect: the minimum size it can measure is 1 µm.
The onset pH varied between 9.1 and 9.4 in all of the experiments at a Na ion concentration of 1 M (see ). This span was regarded as being within the experimental error of the estimations of the onset pH at the conditions investigated. No systematic trends with respect to either temperature or the addition of xylan were discerned for the conditions investigated. The average onset pH was found to be 9.3, a value that is in the range of apparent pKa values expected for kraft lignin.[Citation8] When the Na ion concentration was increased to 4 M at 77 °C, however, the onset pH was above 11.3, i.e., significantly higher than at 1 M. This indicates that the onset of precipitation has a strong dependency on the Na ion concentration and will be investigated further in detail in a subsequent study.
TABLE 2. Data of the onset of precipitation.
A comparison of CCC data found in literature with the onset of precipitation (particles ≥1 µm) using the methodology used in this work is shown in . Despite differences in not only measuring methodology, but also regarding source and concentration of lignin as well as mixing/no mixing, temperature and ionic species composition, it can be concluded that the pH at the CCC /onset pH of precipitation increases with increasing Na ion concentration. Furthermore, other parameters such as temperature, ion species composition, mixing and lignin source also differed between the data sources. The CCC of 1.3 M NaCl found at 70 °C and pH 10.5[Citation25] is the point at which the formation of particles is diffusion-limited, forming particles ≥1 µm. In that work, it was shown that at 1 M NaCl, the sizes of particles converged to 500 nm over the experimental time of 40 min. That observation, indicate that it is possible that submicron particles had been formed already at pH values above the onset pH of 9.3 in the current work, however, was kinetically stabilized or metastable until the pH had been lowered to the onset pH. Consequently, the onset pH of 9.3 at 1 M Na ion concentration found in the current work may correspond to the CCC.
Figure 2. Collection of data on the CCC/onset of precipitation (particles of sizes ≥1 μm). Black triangles: no mixing, 25 °C, lignin concentration 0.1 g/L.[Citation16] Green star: no mixing, 70 °C, lignin concentration 0.3-3.3 g / L [25]. Blue squares: mixing 150 rpm, 45 °C, Lignin concentration 130 g /L.[Citation35] Red circles (see this work). *This data point is valid at 25–60 °C.
![Figure 2. Collection of data on the CCC/onset of precipitation (particles of sizes ≥1 μm). Black triangles: no mixing, 25 °C, lignin concentration 0.1 g/L.[Citation16] Green star: no mixing, 70 °C, lignin concentration 0.3-3.3 g / L [25]. Blue squares: mixing 150 rpm, 45 °C, Lignin concentration 130 g /L.[Citation35] Red circles (see this work). *This data point is valid at 25–60 °C.](/cms/asset/65da47d0-63ce-4445-ac36-0738755b3922/lwct_a_1488870_f0002_c.jpg)
Charge Density of Lignin at the Onset
Initially, the phenolic groups are protonated gradually as the pH decreases. An estimate of how much the degree of ionization may have decreased at the average onset pH of 9.3 (25 °C) at 1 M Na ion concentration can be made from EquationEquations (1)–(3) combined with the associated assumptions presented in the section Estimation of Charge Density. The results obtained in this study for pH 9.3 at 25 °C are presented in . While the estimates are rather rough, they give the magnitude of the degree of ionization that can be expected around the onset pH, based on apparent pKa values reported previously for softwood Indulin AT kraft lignin[Citation8] and that of monomeric coniferyl alcohol.[Citation9] It should be kept in mind that the estimated degrees of ionization assuming a pKa corresponding to coniferyl alcohol are probably too high, as this phenolic compound is relatively acidic[Citation9] compared to the phenolic structures expected in kraft lignin.[Citation14] Moreover, since the electrostatic interactions between a charged macromolecule/particle and protons in the solution increase with molecular weight/size, assuming the remaining molecular properties to be constant, a lower pH is rendered at the surface of the macromolecule/particle than in the bulk: this increases the apparent pKa (see the pKa reported for kraft lignin[Citation8]). The results reported in indicate that the degree of ionization probably has to be decreased to a very low level for the precipitation onset of micron-sized particles to occur at 1 M Na ion concentration.
According to the estimates shown in , and the total density of the phenolic group of 4.27 mmol/g reported for this lignin,[Citation30] the charge density provided by phenolic groups may be 0-0.9 mmol/g at the conditions investigated for the onset of precipitation at 1 M Na ion concentration. However, the carboxylic groups on lignin should be ionized fully at pH 9.3, and thus contribute 0.45 mmol/g[Citation30] to the charge density. This indicates that both phenolic and carboxylic groups can be expected to contribute to the total charge density in the same order of magnitude already at the precipitation onset pH for the conditions stipulated above. Observations showing that the pH still affects the solubility of kraft lignin in the acidic pH range were made in earlier works as well[Citation16]: it was attributed to decreasing the interactions of electrostatic origin between the lignin particles as carboxylic groups were protonated, or possibly due to increased hydrogen bonding caused by the protonated form of the carboxylic groups.
The Course of Precipitation and Chord Length Distributions
One typical example of the course of the formation of particles of various size classes when acid was added is found in (Exp. 8). The kinetics of particle formation was fast just after a volume of acid was added, and decreased as the formation of particles pursue toward what appears to be steady state at a given condition. This indicates that each condition is associated with a certain equilibrium condition: a behavior that has been observed in previous studies investigating the yield at various conditions,[Citation22,Citation23] where the yield increased when the pH was lowered.
Figure 3 The course of precipitation in the neutral to acidic pH range at 77 °C, 1 M and xylan added (Exp. 8). This is the sequence following on the sequence of the early stages presented in Figure 1.
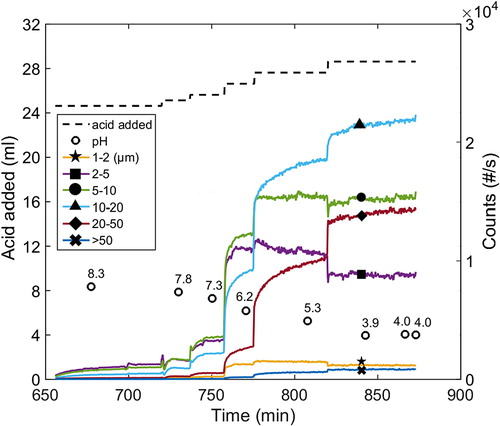
The evolvement of the chord length distribution over the course of precipitation in the neutral to acidic pH range at 77 °C and 1 M Na ion concentration, both with and without additions of xylan, is shown in ; the corresponding data for 65 °C is shown in . It is evident that larger particles are formed at 77 °C than at 65 °C, both with and without xylan added, which is in agreement with earlier work.[Citation36]
Figure 4 Profiles of chord length distributions at 77 °C and 1 M Na ion concentration. Dashed lines: with xylan added (Exp. 8). Solid lines: pure lignin references (Exp. 9).
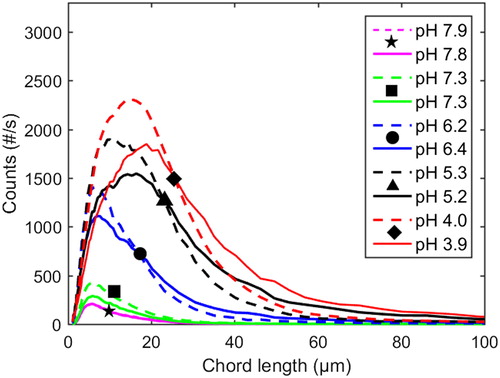
Figure 5 Profiles of chord length distributions at 65 °C and 1 M Na ion concentration. Dashed lines: with xylan added (Exp. 6). Solid lines: pure lignin references (Exp. 7).
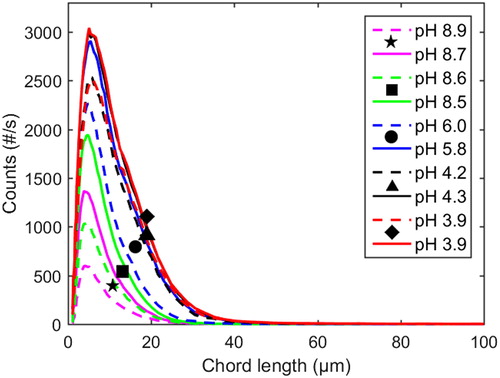
Generally, the numbers of particles of different sizes increases gradually as the pH decreases from neutral to acidic conditions, both with or without added xylan (see and ). The count values increased over the whole size distribution as the pH was decreased, implying that all the more lignin precipitated as the pH was subsequently decreased. There are several possible explanations for this evolvement of the chord length distribution. It could, for instance, be due to the growth of existing particles via the sorption of macromolecules onto their surfaces, thereby allowing them to grow larger, combined with the breakage/attrition of large particles into smaller particles caused by agitation. It may also be further nucleation directly from the solution which forms particles that grow/agglomerate to generate particles of various sizes in the size-range detectible by the FBRM unit. Breakage/attrition may be significant in this situation as well. A rigorous quantitative investigation is necessary to determine the mechanism(s) responsible for the behavior observed. Regardless of the mechanisms stated above, the observation that an increased degree of precipitation was caused by a subsequent lowering of the pH may originate from the heterogeneity of lignin solubility due to differences in the molecular properties in the population (e.g., molecular weight and content of ionizable groups). This may lead to all the more lignin being precipitated as the pH is subsequently decreased.[Citation23,Citation25]
The significance of the ionized carboxylic groups may increase as the phenolic groups approach complete protonation. Precipitation was found to be pH sensitive far below the onset pH at 65 °C, and particularly at 77 °C, both in terms of increase in numbers (counts) and size of particles (chord lengths): it is plausible that these observations are, to a large extent, a consequence of the protonation of carboxylic groups on lignin. Moreover, the effect was clear both with and without added xylan.
Compared to the case of no added xylan, a difference in the shape of the chord length distributions can be observed at 77 °C and pH values around 4–6 when xylan was added: with added xylan, the distribution shifted toward smaller particles (see ). At 65 °C, a similar shift was observed (), however, not as clear as at 77 °C, see the normalized chord length distributions in and .
Figure 6 Profiles of normalized chord length distributions at 77 °C and 1 M Na ion concentration. Each distribution is normalized to a number-based fraction of the total number at that point in time. Dashed lines: with xylan added (Exp. 8). Solid lines: pure lignin references (Exp. 9).
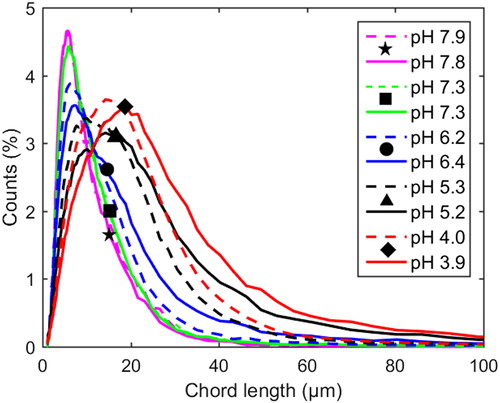
Figure 7 Profiles of normalized chord length distributions at 65 °C and 1 M Na ion concentration. Each distribution is normalized to a number-based fraction of the total number at that point in time. Dashed lines: with xylan added (Exp. 6). Solid lines: pure lignin references (Exp. 7).
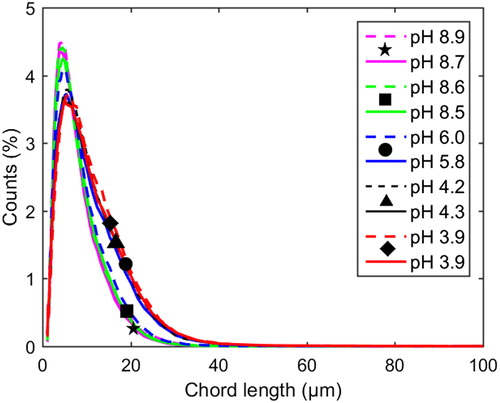
The shifts observed in the chord length distributions in the presence of added xylan at around pH 4–6, which are very clear in , may be a consequence of the incorporation of xylan into the particles as they form. This could cause a reduction in the further growth/agglomeration of particles due to increased electrostatic repulsive forces and/or steric effects, resulting in smaller particles being formed when compared to the case without added xylan. The explanation that ionized carboxylic groups influence precipitation in the pH range 4–6 at 1 M Na ion concentration (with and without added xylan) was strengthened further when observing the chord length distributions in the pH range 4–6 at 4 M Na ion concentration (xylan was added) (see ). At high ionic strength, the precipitation was much less sensitive to changes in the pH in this range than at 1 M Na ion concentration (compare the shifts in chord length distributions in the pH range 4–6 in to the corresponding shift in ). It is reasonable to assume that the ionized groups, whether they are on lignin or xylan, are screened sufficiently by counterions at such high ionic strengths and that the impact of carboxylic groups are of lesser importance. According to the measurements, most of the increases in the number and size of the particles at high ionic strength occurred instead in the pH range 8.5–11.1, where the degree of ionization of the phenolic groups is expected to influence precipitation strongly.
Figure 8 Profile of chord length distribution during the course of precipitation illustrated in Figure 10. Conditions: 77 °C, 4 M Na ion concentration and xylan added (Exp. 11).
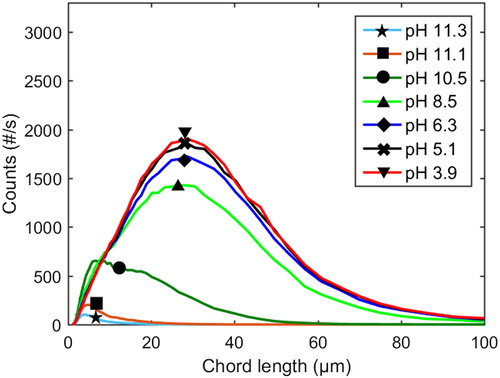
CONCLUSIONS
The following conclusions were drawn from this work:
The onset of precipitation, as defined in this study (particle chord lengths ≥1 µm), showed no significant dependency on temperature or the addition of xylan in the temperature range of 45–77 °C, at Na ion concentrations of 1 M and measured to occur, on average, at pH 9.3 (measured at 25 °C). The pH of the onset of precipitation at 4 M Na ion concentration and 77 °C was shown to be much higher than the corresponding pH at 1 M Na ion concentration: about 2 pH units.
It was found that the sizes of particles increased when the temperature was increased from 65 to 77 °C at neutral to acidic conditions and 1 M Na ion concentration, both with and without added xylan. This was most pronounced at pH values around 4–6.
With xylan added, the chord length distribution measured shifted to smaller particles at pH values around 4–6: the shift was clear at 77 °C but also visible at 65 °C.
The degree of precipitation was shown to be pH dependent: both the number and size of the particles increased with decreasing pH, confirming that more and more lignin precipitated as the pH was subsequently decreased.
Screening at the higher ionic strength resulted in a higher onset pH and a higher yield at a given pH compared to the lower ionic strength experiments. It was also found that, at the higher ionic strength, there was no significant increase in the yield in the pH range 4–6.
ACKNOWLEDGMENTS
The authors thank Dr Huyen Nguyen Lyckeskog, at Forest Products and Chemical Engineering (Chalmers University of Technology) for her assistance in measurements using atomic absorption spectroscopy.
Additional information
Funding
REFERENCES
- Öhman, F.; Wallmo, H.; Theliander, H. A novel method for washing lignin precipitated from kraft black liquor––laboratory trials. Nordic Pulp & Paper Research Journal 2007, 22(1), 9–16.
- Öhman, F.; Theliander, H.; Tomani, P.; Axegard, P. Method for separating lignin from black liquor. Google Patents 2013.
- Kadla, J. F.; Kubo, S.; Venditti, R. A.; Gilbert, R. D.; Compere, A. L.; Griffith, W. Lignin-based carbon fibers for composite fiber applications. Carbon 2002, 40(15), 2913–2920.
- Lora, J. H.; Glasser, W. G. Recent industrial applications of lignin: a sustainable alternative to nonrenewable materials. Journal of Polymers and the Environment 2002, 10(1/2), 39–48.
- Gellerstedt, G.; Sjöholm, E.; Brodin, I. The wood-based biorefinery: a source of carbon fiber? The Open Agriculture Journal 2010, 4(1), 119–124.
- Nguyen, T. D. H.; Maschietti, M.; Belkheiri, T.; Åmand, L.-E.; Theliander, H.; Vamling, L.; Olausson, L.; Andersson, S.-I. Catalytic depolymerisation and conversion of kraft lignin into liquid products using near-critical water. The Journal of Supercritical Fluids 2014, 86, 67–75.
- Baker, D. A.; Gallego, N. C.; Baker, F. S. On the characterization and spinning of an organic‐purified lignin toward the manufacture of low‐cost carbon fiber. Journal of Applied Polymer Science 2012, 124(1), 227–234.
- Norgren, M.; Lindström, B. Dissociation of phenolic groups in kraft lignin at elevated temperatures. Holzforschung 2000, 54(5), 519.
- Ragnar, M.; Lindgren, C. T.; Nilvebrant, N.-O. pKa-Values of guaiacyl and syringyl phenols related to lignin. Journal of Wood Chemistry and Technology 2000, 20(3), 277–305.
- Robert, D. R.; Bardet, M.; Gellerstedt, G.; Lindfors, E. L. Structural changes in lignin during kraft cooking part 3. On the structure of dissolved lignins. Journal of Wood Chemistry and Technology 1984, 4(3), 239–263.
- Argyropoulos, D. S. Quantitative phosphorus-31 NMR analysis of six soluble lignins. Journal of Wood Chemistry and Technology 1994, 14(1), 65–82.
- Froass, P. M.; Ragauskas, A. J.; Jiang Jian, E. NMR studies part 3: analysis of lignins from modern kraft pulping technologies. Holzforschung––International Journal of the Biology, Chemistry, Physics and Technology of Wood 1998, 52(4), 385.
- Norgren, M.; Lindström, B. Physico-chemical characterization of a fractionated kraft lignin. Holzforschung 2000, 54, 528.
- Crestini, C.; Lange, H.; Sette, M.; Argyropoulos, D. S. On the structure of softwood kraft lignin. Green Chemistry 2017.
- Lindström, T. The colloidal behaviour of kraft lignin. Colloid and Polymer Science 1979, 257(3), 277–285.
- Lindström, T. The colloidal behaviour of kraft lignin part II. Coagulation of kraft lignin sols in the presence of simple. Colloid and Polymer Science 1980, 258(2), 168–173.
- Laine, J.; Lövgren, L.; Stenius, P.; Sjöberg, S. Potentiometric titration of unbleached kraft cellulose fibre surfaces. Colloids and Surfaces A: Physicochemical and Engineering Aspects 1994, 88(2–3), 277–287.
- Wallmo, H.; Theliander, H.; Jönsson, A.-S.; Wallberg, O.; Lindgren, K. The influence of hemicelluloses during the precipitation of lignin in kraft black liquor. Nordic Pulp & Paper Research Journal 2009, 24(2), 165–171.
- Durruty, J.; Mattsson, T.; Theliander, H. Local filtration properties of kraft lignin: the influence of residual xylan. Separation and Purification Technology 2017, 179, 455–466.
- Alén, R.; Patja, P.; Sjöstrom, E. Carbon Dioxide Precipitation of Lignin from Pine Kraft Black Liquor. Tappi 1979, 62(11), 108–110.
- Uloth, V. C.; Wearing, J. T. Kraft lignin recovery: acid precipitation versus ultrafiltration. Part I. Laboratory test results. Pulp and Paper Canada 1989, 90, 67–71.
- Zhu, W.; Theliander, H. Precipitation of lignin from softwood black liquor: an investigation of the equilibrium and molecular properties of lignin. BioResources 2015, 10(1), 1696–1714.
- Zhu, W.; Westman, G.; Theliander, H. Lignin separation from kraft black liquor by combined ultrafiltration and precipitation: a study of solubility of lignin with different molecular properties. Nordic Pulp & Paper Research Journal 2016, 31(2), 270–278.
- Norgren, M.; Edlund, H.; Wågberg, L.; Lindström, B.; Annergren, G. Aggregation of kraft lignin derivatives under conditions relevant to the process, part I: phase behaviour. Colloids and Surfaces A: Physicochemical and Engineering Aspects 2001, 194(1–3), 85–96.
- Norgren, M.; Edlund, H.; Wågberg, L. Aggregation of lignin derivatives under alkaline conditions. Kinetics and aggregate structure. Langmuir 2002, 18(7), 2859–2865.
- Reerink, H.; Overbeek, J. T. G. The rate of coagulation as a measure of the stability of silver iodide sols. Discussions of the Faraday Society 1954, 18, 74–84.
- Evans, D. F.; Wennerström, H. Colloidal stability. In The Colloidal Domain: Where Physics, Chemistry, Biology, and Technology Meet, 2nd ed.; Evans, D. F.; Wennerström, H., Eds.; Danvers, MA: Wiley-VCH, 1999, 401–442.
- Kannangara, M.; Marinova, M.; Fradette, L.; Paris, J. Effect of mixing hydrodynamics on the particle and filtration properties of precipitated lignin. Chemical Engineering Research and Design 2016, 105, 94–106.
- Durruty, J.; Mattsson, T.; Theliander, H. Local and average filtration properties of kraft softwood lignin. Nordic Pulp and Paper Research Journal 2015, 30(1), 132–140.
- Aminzadeh, S.; Lauberts, M.; Dobele, G.; Ponomarenko, J.; Mattsson, T. M.; Sevastyanova, O. E.; Lindström, M. Membrane filtration of kraft lignin: Structural characteristics and antioxidant activity of the low-molecular-weight fraction. Industrial Crops and Products 2018, 112, 200–209.
- Jedvert, K.; Saltberg, A.; Lindström, M. E.; Theliander, H. Mild steam explosion and chemical pre-treatment of Norway spruce. BioResources 2012, 7(2), 2051–2074.
- Israelachvili, J. N. Electrostatic forces between surfaces in liquids. In Intermolecular and Surface Forces 3rd ed.; Israelachvili, J. N., Ed.; Boston: Academic Press, 2011, 291–340.
- Zavitsas, A. A. Acid ionization constants of phenol and some hydroxy-methylphenols between 20.degree. and 60.degree. Journal of Chemical & Engineering Data 1967, 12(1), 94–97.
- Olofsson, G.; Olofsson, I. The enthalpy of ionization of water between 273 and 323 K. The Journal of Chemical Thermodynamics 1977, 9(1), 65–69.
- Sewring, T.; Theliander, H. A study of kraft lignin acid precipitation in aqueous solutions using focused beam reflectance measurement (FBRM(R. )). Journal of Science and Technology for Forest Products and Processes 2018, 6(5).
- Öhman, F.; Theliander, H. Filtration properties of lignin precipitated from black liquor. Tappi Journal 2007, 6(7), 3–9.