Abstract
This article presents the development and evaluation of a very compact facility for exposing humans to concentrated ambient ultrafine particles (da < 0.15 μm). The human ultrafine particle concentrator (UFPC) operates at an intake flow rate of 1200 liters per minute (LPM). The concentrator is preceded by an ultrafine impactor which separates the accumulation mode from ultrafine mode particles under a very low pressure drop (1.5 kPa), a feature that is essential in enabling human inhalation studies of ultrafine concentrated ambient particulates (CAP). A key feature of the UFPC is a new cooling system, consisting of a programmable refrigerated circulator, which produces the supersaturation that is necessary to grow ultrafine PM to supermicrometer sizes so that they can be concentrated by means of conventional virtual impaction. The new cooling system allows for entirely automated operation of the UFPC. The UFPC was characterized in field experiments, in which the concentration enrichment of ultrafine particles was determined based on their number and mass concentration as well as on chemical composition including elemental carbon (EC), inorganic ions (sulfate and nitrate), and polycyclic aromatic hydrocarbons (PAH). Tests were conducted at minor-to-total flow ratios varying from 2.5–5% (hence at minor flow rates between 30–60 LPM). Measurements with the scanning mobility particle sizer (SMPS) showed a near-ideal increase in number concentrations (corresponding to the ratio of total-to-minor flow rate) of ultrafine particles after enrichment. The concentration enrichment was uniform across the entire particle diameter range of 15–660 nm. Similar results were obtained for EC and PAH concentrations (measured by an Aethalometer). Time-integrated filter-based tests, conducted to characterize the system for ultrafine PM mass and inorganic ion concentrations showed that the average enrichment factor was very close to the ideal values, indicating near-perfect collection efficiency with minimal particle losses.
INTRODUCTION
Atmospheric ultrafine particles (defined in this study as having diameters less than 0.15 μm) either are formed by gas-to-particle conversion processes, in which hot supersaturated vapors undergo condensation upon being cooled to ambient temperatures, or are directly emitted as products of incomplete combustion processes (CitationFinlayson-Pitts and Pitts 1986). Although the mass fraction of the ultrafine mode is negligible, this size range contains the highest number of ambient particles as well as the highest total surface area. Because of their increased number and surface area, ultrafine particles are particularly important in atmospheric chemistry and environmental health.
Toxicological and epidemiological evidence linking respiratory health effects and exposures to ultrafine particles has been increasing over the last several years. Recent epidemiological studies (CitationHeyder et al. 1996; CitationPeters et al. 1997) demonstrated a stronger association between health effects and exposures to ultrafine particles compared to accumulation mode or coarse particles. Toxicological studies by CitationDonaldson et al. (1998) and CitationOberdoerster et al. (1992) indicated that ultrafine particles exerted a stronger physiological effect than the same mass of coarse or fine particles. A recent study by CitationLi et al. (2003) indicated that ultrafine PM were most potent towards inducing cellular heme oxygenase-1 (HO-1) expression and depleting intracellular glutathione, both sensitive markers for oxidative stress, compared to concurrently collected accumulation and coarse mode PM. The same study showed that ultrafine particles, and to a lesser extent fine particles, localize in mitochondria where they induce major structural damage.
The pulmonary toxicity of ultrafine particles has also been demonstrated in several controlled laboratory exposure studies. CitationOberdoster et al. (1992) have shown that apparently “inert” dusts consisting of ultrafine particles can be highly toxic to the lung because of interstitial access across the alveolar epithelium where they interact with macrophages. It is conceivable that, when subjected to a high ultrafine particle number concentration, “overload” may occur, in which the alveolar macrophages themselves cause injury to the lungs by releasing excessive quantities of oxygen radicals, proteases, and certain proteins et al. 1992). Other investigators have suggested that ultrafine particles coated with neutralized strong acids may, upon deposition, cause tissue damage due to their acidity (CitationFerin et al. 1991). In addition, acids or catalytic metals on the surfaces of irritant particles can be more efficiently transferred to the lungs by ultrafines than by an equal mass of larger particles. This is both because of the larger surface area-to-mass ratio inherent in the smaller particles and because the ultrafines have a higher deposition efficiency in the alveolar region. Finally, investigators have suggested that trace metals distributed widely throughout the lung on ultrafine particles could catalyze the formation of oxidants within the lung, which in turn produce tissue damage (CitationGhio et al. 1996; CitationDreher et al. 1997).
In order to conduct the first ever studies of the health effects of real-world ultrafine particles on humans, we developed the ambient ultrafine PM concentrator (UFPC). The concentrator will be used to provide ultrafine PM at concentrations sufficient to perform inhalation toxicology studies designed to test hypotheses regarding the effects of spatial, seasonal, and compositional differences of ultrafine particles in the development or aggravation of cardiopulmonary diseases. These differences stem from the wide range of sources and formation mechanisms of ultrafine PM in the Los Angeles Basin as well as changing meteorological conditions, all of which produce highly variable diurnal and seasonal patterns of ultrafine PM (CitationKim et al. 2002; CitationFine et al. 2003).
MATERIALS AND METHODS
Description of the UFPC
The design of the UFPC is shown schematically in . The particle concentration principle of the UFPC is similar to that of previously developed, smaller scale concentrators (CitationKim et al. 2001a, Citationb) but with many significant design and operational improvements, including the design of the saturators and condensers that produce the condensational growth of ultrafine PM, the intake and output flows, and the overall concentration enrichment factor for ultrafine PM.
The major components of the UFPC include, (1) an ultrafine impactor to separate ultrafine from the accumulation mode PM, (2) 4 rectangular saturators (stainless steel containers), each 30×15×40 cm in size, (3) 12 parallel condensers, (4) 12 virtual impactors, and (5) 12 diffusion driers. The UFPC operates at a total sampling flow rate of 1200 LPM. Ambient aerosol is first drawn through a high-flow, low-pressure drop inertial impactor with a 50% cutpoint of 0.15 μm developed by CitationMisra et al. (2002) to remove accumulation mode particles. Removal of these particles makes it possible to examine the effects of ultrafine particles only. As it will be illustrated in the results and discussion sections of this article, the present system could also be used as a very effective concentrator for PM2.5, in which case the 0.15 μm impactor is replaced by a 2.5 μm commercially available impactor (PM2.5 High-Volume Sampler, Model TE-6070-2.5-HVS Mass Flow Control, Tisch Environmental, Cleves, OH, 45002). The ultrafine PM is then split into 4 saturators. In each saturator, the aerosol passes over a pool of warm, ultrapure, deionized water and the air becomes saturated at about 30–32°C. The water in each container is kept at this temperature by means of quartz immersions heaters (Model EW-03124-30 Immersion heater, Cole-Parmer Instrument Company, Vernon Hills, IL). Each heater produces a maximum heating output of 0.5 kW, which is adequate to heat and saturate at least 300 LPM of air (one-quarter of the total intake flow of the concentrator). The heating output of each immersion heater is adjusted by means of a voltage regulator. Following the saturators, the aerosol is drawn through 12 condensing tubes placed in parallel. In each condenser, the aerosol is cooled from 32°C to about 22°C, and the resulting supersaturation allows ultrafine particles to grow to supermicron size. The grown particles are then drawn through 12 virtual impactors connected in parallel and identical to that already used in our prototype ultrafine concentrator described by CitationKim et al. (2001a). The design of the saturators and the cooling system, which includes use of a voltage-controlled quartz heating rod for the saturation process and a programmable refrigerated circulator for cooling the condensers (discussed in a subsequent section), represents a major improvement in the UFPC over the originally developed ultrafine concentrators.
The minor-to-total flow ratio of each virtual impactor can be varied from 2 to 5% of the intake flow, thus the 12-virtual impactor ensemble supplies to the exposure chamber an ultrafine aerosol at a flow rate ranging from 24 to 60 LPM, enriched in concentration ideally by a factor of 50 to 20, respectively. Once concentrated, the particles pass through 12 diffusion dryers in parallel (each connected to the minor flow of one virtual impactor) to remove the excess water and return the particles to their original relative humidity and temperature conditions (and thus return them to their original size), prior to entering the exposure chamber. The concentration levels can be further adjusted by mixing the particle-enriched air stream with particle-free air. The additional air flow is especially necessary in exposures during which a subject exercises, thereby breathing at inhalation flows of 30–50 LPM compared to the 12–18 LPM inhalation flow at rest (CitationLippmann 1977). These exposures require a lower concentration enrichment level because the increase in breathing rate compensates for the lower concentration. The entire UFPC system has been placed inside a mobile trailer so that human exposure can be conducted in several locations in order to take advantage of the varying characteristics of ultrafine PM as mentioned above.
Description of Cooling System for the UFPC
In our original ultrafine concentrator prototypes (CitationKim et al. 2001a, b), the cooling media in the condensers was a mixture of ice, salt, and water, cooling the aluminum sampling lines in a commercially available Coleman cooler. For the development of the larger scale UFPC Human Exposure Facility, it became imperative to devise a new cooling method, which would be self-sustaining, require minimum maintenance, and, very importantly, would provide much more uniform cooling over the entire length of the condensing tubes. Towards that goal, we employed two refrigerated circulating chillers rated at 900 Watts maximum cooling capacity each (Programmable Refrigerated Circulator, Model 9710, Polyscience, Niles, IL). Each chiller was chosen to provide sufficient cooling load to accommodate 600 LPM of air (thus for 6 of the 12 condenser tubes of the UFPC). Each of the 12 condensing tubes consisted of two concentric tubes made of stainless steel, with internal diameters of 2.54 cm (inner tube) and 5 cm (outer tube) and a length of 80 cm. The condensing tubes were placed vertically on top of the saturators, as shown in . The chillers circulated the coolant, a mixture of ethylene glycol and water in a ratio of 1:1, through the condensers. The total coolant flow through each of the condensers is about 2 LPM and can be controlled using a valve. Each condenser tube was insulated on the outside of the steel shell with commercially available polyethylene insulation.
The grown aerosol leaves the condensing tubes and immediately enters the virtual impactors. These virtual impactors are the same as those described by CitationKim et al. (2001a) and were designed to have a 1.8 μm 50% cutpoint at an intake flow rate of 105 LPM, under a pressure drop of at 110″ H2O (27 kPa) across the major flow. The pressure drop in the minor (or concentrated) flow is minimal (i.e., less than 0.2 kPa).
The performance of the Model 9710 refrigerated circulator was evaluated in order to determine whether the circulator was able to provide an optimum and, very importantly, a stable temperature for the growth of particles over a prolonged period of time, thus achieving a steady-state coolant temperature circulating through the condensers. The evaluation was performed by using one of the Model 9710 circulators to cool six condensers (corresponding to a flow of 600 LPM) in the UFPC. Each of the 6 condensers was followed by a virtual impactor at its outlet, where the grown ultrafine particles were concentrated. The minor flow of each virtual impactor was connected to a diffusion dryer, and the flow from all six diffusion dryers was then merged into one minor flow (of 30 LPM). The performance of the circulator was then evaluated by periodically checking the enrichment in terms of particle number concentration measured before and after the concentrator by means of a Condensation Particle Counter (CPC, Model 3022A, TSI Inc., St. Paul, MN). The circulator reservoir temperature was also recorded periodically. The saturator temperature in these experiments was maintained at 28–30°C. The coolant flow rate in these experiments was set at 2 LPM per condenser in order to provide adequate residence time in the circulator reservoir for cooling.
Field Characterization of the UFPC
Following the evaluation of the cooling system, the performance of the UFPC was field tested using real-world ambient Los Angeles aerosols during the period of May–August 2002. Measurements of concentration-enriched ultrafine aerosols were compared to direct ambient measurements made with a scanning mobility particle sizer (SMPS; Model 3936, TSI Inc., St. Paul, MN). Three 15 min average ambient aerosol concentrations were first measured by the SMPS. The SMPS was then connected downstream of the ultrafine concentrator and immediately upstream of the exposure chamber and three additional 15 min readings were taken. Repeated runs were conducted by switching the SMPS back and forth between the ambient air and the outlet of the UFPC. Tests were conducted at minor flows rations of 2.5, 3.3, and 5%, which correspond to ideal enrichment factors of 40, 30, and 20, respectively. With the exception of the 5% minor flow configuration, for which concentration enrichment was determined for the entire SMPS particle size range (15 to 650 nm), the UFPC was preceded by the 0.15 μm cutpoint impactor and thus no data are presented for particles larger than this mobility diameter. In addition to the particle size distribution measurements, an Aethalometer (Model AE-21 (UV+BC), Thermoandersen, Smyrna, GA) was also used to measure concentration enrichment factors based on ultrafine PM elemental carbon (EC) and certain polycyclic aromatic hydrocarbons (PAHs) concentrations. The latter compounds are measured by means of optical absorption at 370 nm in the near ultraviolet. Many aromatic organic species have strong absorbance at this wavelength, and the UV channel of the instrument corresponds very strongly to such PAHs as those present in tobacco smoke, fresh diesel exhaust, and wood smoke. The experimental procedure was otherwise identical to that described above for the SMPS measurements. The instrument was connected upstream of the UFPC (right after the 0.15 μm impactor) for measuring the ambient concentrations of ultrafine EC and PAHs. Then it was connected downstream of the UFPC diffusion driers for taking measurements of the enriched aerosol. Repeated measurements were conducted by switching the Aethalometer back and forth in between ambient and concentrated aerosol.
In addition to the continuous monitoring procedures described above, the UFPC was characterized in time-integrated experiments, in which ultrafine PM was collected on 47 mm Teflon filters (PTFE Teflon, 2 μm pore, Gelman Science Ann Arbor, MI) placed before and after the UFPC. The sampling flows were 30 LPM for both filters and were purposely matched in order to eliminate the potential effects of adsorption and volatilization artifacts, which depend greatly on the filter face velocity (CitationAppel et al. 1984; CitationZhang and McMurry 1987). A total of 12 such experiments were performed, all at a minor-to-total flow ratio of 5% (i.e., minor flow of 60 LPM), thereby corresponding to an ideal enrichment factor of 20. The Teflon filters were weighed before and after each experiment in a temperature and relative humidity-controlled room to determine mass concentrations of ambient and concentrated ultrafine aerosols. Subsequently, the filters were extracted with a mixture of 5 mL of deionized water and 0.15 mL ethanol and the concentrations of inorganic particle-bound ions (nitrate and sulfate) were measured for ambient and concentrated PM by means of ion chromatography.
RESULTS AND DISCUSSION
Evaluation of the Cooling System
The result of the evaluation of one of the Model 9710 Refrigerated Circulators is provided in . The minor flow of the virtual impactors was 30 LPM (i.e., 5% of the major flow of a 600 LPM system), corresponding to an ideal enrichment of 20. The average enrichment factor obtained for the data presented in is 19.42 (±1.55), which is almost identical to the ideal value. This value corresponds to the average of all the enrichment values recorded every 15 min using the CPC for the entire length of the 150 min experiment, longer than a typical exposure which lasts about 2 h in the current experimental design. The data in also indicate that the temperature of the circulator reservoir reached a steady-state value of −10.4°C after 120 min of the experiment. These results demonstrate that each of the two Model 9710 circulators have sufficient cooling power to cool 6 condensers (corresponding to a total aerosol flow of 600 LPM) over long periods of time (well in excess of the 2 h needed in the current scheme). Thus, the applicability and robustness of the new cooling system was confirmed and two circulators are employed to provide adequate cooling to operate the UFPC at 1200 LPM.
Field Evaluation of the UFPC
The results of the complete field evaluation of the UFPC are shown in . First, particle number-based enrichment for different minor flow ratios, as measured by an SMPS for ultrafine PM, is presented in . The minor flows in these experiments were 60, 40, and 30 LPM, corresponding to the minor-to-total flow ratios of 5, 3.3, and 2.5%, respectively. The major flow was adjusted to keep the total flow at 1200 LPM for each minor flow. The enrichment values are averaged over three measurements and were obtained by dividing the particle number concentration values for a particular particle size in the enriched aerosol by the corresponding value in the ambient aerosol. As evident from the figure, the overall enrichment value for the minor-to-total flow of 5% was around 19.11 (±1.43), thus very close to the ideal value of 20. A very important observation made for this minor flow was the ability of the UFPC to concentrate particles to as low as 15 nm (which represents the minimum particle diameter detected by our SMPS) by a factor of 19–20. For the minor-to-total flow ratios of 3.3 and 2.5%, the measured overall concentration enrichment values were 29.5 (±2.2) and 39.6 (±1.8), respectively, for the particle range between 15 to 180 nm. These were again almost identical to the ideal values of 30 and 40. The data plotted in suggest that the concentration enrichment decreases as the particle mobility diameter decreased below 20 nm for the 2.5% and 3.3% minor flow ratio configurations. This decrease may be due to possible inhomogeneities in the condensers, causing saturated air to be exposed to different supersaturation ratios, some too small to activate the smaller particles. The decrease could also be occurring due to diffusion losses of the smaller particles, which would be compounded by lower flow rates. Another possibility may be that particles smaller than 20 nm grow to a size either close to or slightly smaller than the cutpoint of the virtual impactors. Also, as the minor-to-total flow ratio decreases, particle capturing efficiency of the virtual impactors may be decreasing around the 50% cutpoint due to an overall increase of the cutpoint diameter with decreasing minor-to-total flow ratio (CitationLoo and Cork 1988). Clearly, the produced supersaturation is sufficient to activate even these very small particles, as evident from the fact that they do become concentrated, and at a 5% minor-to-total flow ratio their enrichment is near its ideal value.
FIG. 3 Concentration enrichment as a function of particle diameter for three minor-to-total flow ratios (r).
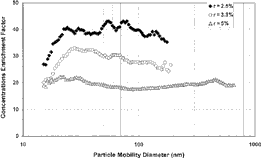
FIG. 4 Size distribution for ambient and concentrated aerosol using UFPC (minor-to-total flow ratio = 5%).
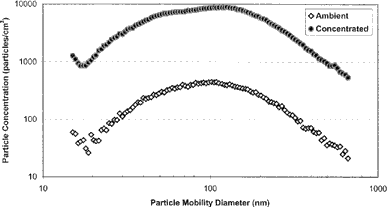
FIG. 5 Measurements of ambient and enriched concentrations of EC and PAHs versus time (minor-to-total flow ratio = 5%).
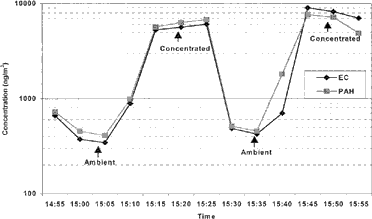
FIG. 6 Measurements of ambient and enriched concentrations of EC and PAHs versus time (minor-to-total flow ratio = 3.3%).
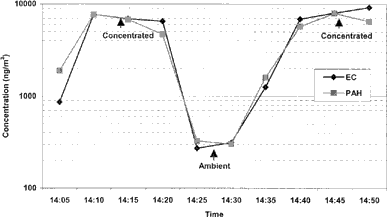
FIG. 7 Measurements of ambient and enriched concentrations of EC and PAHs versus time (minor-to-total flow ratio = 2.5%).
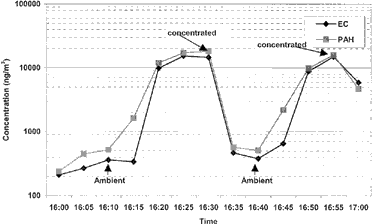
FIG. 8 Comparison of ambient and enriched ultrafine PM mass concentration for a minor-to-total flow ratio of 5%.
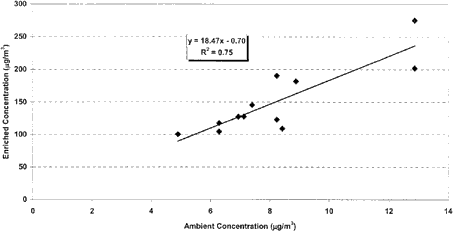
FIG. 9 Comparison of ambient and enriched ultrafine nitrate and sulfate mass concentration for a minor-to-total flow ratio of 5%.
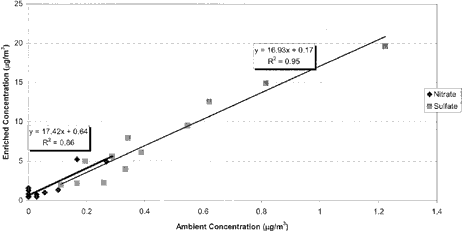
Overall, the data presented in show that the enrichment values obtained for different minor-to-total flow ratios correspond to the maximum obtainable enrichment values. These results unequivocally indicate that the desired enrichment is observed for ultrafine particles with minimal losses, implying that no particle coagulation occurred during the enrichment process. If coagulation had occurred, the measured number concentrations after enrichment would have dropped.
In addition to the tests performed above, the aerosol size distribution was also measured before and after enrichment for the minor-to-total flow ratio of 5% (corresponding to an enrichment of 20) on the basis of particle mobility diameter. shows the concentration enrichment as a function of particle mobility diameter obtained by measuring the size distributions of ambient aerosols upstream of the concentrator and immediately downstream of the diffusion dryers of the concentrator with the SMPS. The data in establish that the size distribution was well preserved during the concentration enrichment process. The number of median diameter and geometric standard deviation for the ambient aerosol are 96.7 nm and 2.0, respectively, which are almost identical to the values of 96.9 nm and 2.1 obtained for the concentrated aerosol. These results confirm that drying by diffusion returns the concentrated droplets to their original size with minimal distortion of the size distribution. This also indicates that the process of growth occurred without any coagulation, which would also have distorted the size distribution.
The data for EC and PAHs measurements are presented in corresponding to the minor flows of 60, 40, and 30 LPM. The experimental procedure was identical to that described above for the SMPS measurements, i.e., the Aethalometer was connected upstream of the UFPC (right after the 0.15 μm impactor) and downstream of the UFPC, and repeated measurements were conducted. The enrichment values calculated from these figures correspond to the average ratio of the higher concentrations obtained for the enriched aerosol to the lower ambient concentrations observed between two successive 15 min measurements. The data recording period of the Aethalometer during these measurements was 5 min. The intermediate values in between the higher and lower observed concentrations represent a 5 minute period covering a combination of sampling enriched and ambient aerosol, and thus these data were not used in calculating the enrichment factors. As the data plotted in indicate, a near-ideal enrichment was observed for all the minor flows. The average EC enrichment was 19.55 (±2.5), 28.9 (±0.67), and 42.5 (±4.1) corresponding to the minor-to-total flow ratios of 5, 3.3, and 2.5%, respectively. The Aethalometer PAH signal showed enrichments of 16.7 (±0.22), 25.1 (±1.86), and 38.21 (±3.52) corresponding to the minor-to-total flow ratios of 5, 3.3, and 2.5%, respectively. This demonstrates that the UFPC can concentrate hygroscopic (EC) and some semivolatile constituents of the ambient aerosol such as PAHs by near-ideal enrichment factors.
In addition to the measurements made by the SMPS and Aethalometer described above, time-integrated filter-based measurements were also performed to evaluate the performance of the UFPC. As described earlier, one 47 mm Teflon filter (2 μm pore, PTFE Teflon, Gelman Science, Ann Arbor, MI) was placed upstream of the concentrator's saturators, immediately downstream of the 0.15 μm impactor, and the other filter was placed downstream of the diffusion dryers of the UFPC (i.e., at the outlet of the concentrator). All filters were sampling at a flow of 30 LPM. The mass, sulfate, and nitrate concentrations of enriched and ambient ultrafine aerosol are compared in and , respectively. Ammonium sulfate is a hygroscopic constituent of the ambient aerosol, while ammonium nitrate, in addition to being hygroscopic, is also semivolatile in nature. The average enrichment factors were found to be 18.3, 20.6, and 17.8 for ultrafine PM mass, sulfate, and nitrate, respectively. These results are very close to ideal enrichment, indicating near-perfect collection efficiency with minimal particle losses even for semivolatile (and hygroscopic) species like ammonium nitrate. This observation is of significance since ammonium nitrate is one of the main constituents of ambient aerosol and, when it is heated during the saturation, process, losses due to volatilization may be possible. However, these results establish that no such losses occurred during saturation, and the UFPC can concentrate ammonium nitrate by near-ideal enrichment. These results are further supported by the study conducted by CitationChang et al. (2000), which showed the losses due to volatilization are actually decreased by the concentration enrichment process.
SUMMARY AND CONCLUSIONS
An ultrafine particle concentrator (UFPC) for human exposures was developed and evaluated. The UFPC is capable of concentrating particles up to 40 times ambient concentrations while preserving particle size distributions, thereby enabling toxicological and human clinical studies which were heretofore impossible. Removal of particles above 0.15 μm (if desirable) is achieved by passing the air through an inertial impactor with 50% cutpoint at 0.15 μm, which allows for exposures to only ultrafine particles. The concentration enrichment process is accomplished by first growing ultrafine PM to supermicron size (a size that can easily be concentrated using a virtual impactor) by means of supersaturation/condensation, followed by virtual impaction. The concentrated ultrafine particles are reduced to their original size distribution by passing through diffusion dryers containing silica gel prior to entering the exposure chamber.
The UFPC was evaluated for particle enrichment by taking particle size distribution measurements of the concentration-enriched ultrafine aerosol and comparing them to direct ambient measurements. Results indicated ideal enrichment could be obtained for all of the minor flows ratios and that the concentrator could enrich the particles by the desired factor while preserving particle size distributions.
Measurement of concentrated and ambient ultrafine PM was also performed using an Aethalometer to test for enrichment of EC and PAHs. The average EC enrichment observed was 19.55 (±2.5), 28.9 (±0.67), and 42.5 (±4.1), corresponding to the minor-to-total flow ratios of 5, 3.3, and 2.5%, respectively, while the PAHs were enriched by factors of 16.7 (±0.22), 25.1 (±1.86), and 38.21 (±3.52). Time-integrated tests in which the concentration enrichment was determined based on ultrafine PM mass as well as nitrate and sulfate indicated a near-ideal enrichment with the average ratio of enrichment factor being 18.3, 20.6, and 17.8 for ultrafine PM mass, sulfate, and nitrate, respectively. Thus, field experiments indicated that the concentration enrichment process is not affected by particle size and chemical composition, at least for particles larger than 0.02 μm in diameter.
The development of particle concentrator technologies has fundamentally changed the research into the casual factors associated with exposure to PM. Historically investigators had to use ambient concentrations to conduct studies, and this reality made it difficult to identify and quantify dose-response relationships and to determine the underlying mechanisms of the health effects associated with PM exposure. The UFPC moves research on ambient air exposures into the realm of mechanistic studies, which previously could only be addressed using artificial laboratory-generated aerosols, which clearly lack the complexity of the ambient aerosol. We thus believe that this technological advance will have long-term significance in identifying the bases for health effects from exposure to PM.
Acknowledgments
This work was supported in part by the Southern California Particle Center and Supersite (SCPCS), funded by the U.S. EPA under the STAR program, and by the California Air Resources Board. Although the research described in this article has been funded in part by the United States Environmental Protection Agency through grants # 53-4507-0482 and 53-4507-7721 to USC, it has not been subjected to the Agency's required peer and policy review and therefore does not necessarily reflect the views of the Agency and no official endorsement should be inferred.
REFERENCES
- Appel , B. R. , Tokiwa , Y. , Haik , M. and Kothny , E. L. 1984 . Artifact Particulate Sulfate and Nitrate Formation on Filter Media . J. Air Waste Mgmt. Assoc. , 42 : 409 – 416 .
- Chang , M. C. , Sioutas , C. , Kim , S. , Gong , H. Jr. and Linn , W. S. 2000 . Reduction of Nitrate Losses from Filter and Impactor Samplers by Means of Concentration Enrichment . Atmos. Environ. , 34 : 85 – 98 .
- Donaldson , K. , Li , X. Y. and MacNee , W. 1998 . Ultrafine (Nanometer) Particle Mediated Lung Injury . J. Aerosol Sci. , 29 : 553 – 560 .
- Dreher , K. L. , Jaskot , R. H. , Lehmann , J. R. , Richards , J. H. , McGee , J. K. , Ghio , A. J. and Costa , D. L. 1997 . Soluble Transition Metals Mediate Residual Oil Fly Ash Induced Acute Lung Injury . J. Toxicol. Environ. Health , 50 : 285 – 305 .
- Ferin , J. , Oberdörster , G. , Soderholm , S. C. and Gelein , R. 1991 . Pulmonary Tissue Access of Ultrafine Particles . J. Aerosol Med. , 4 : 57 – 68 .
- Fine , P. M. , Shen , S. and Sioutas , C. 2003 . Inferring the Sources of Fine and Ultrafine Particulate Matter at Downwind Receptor Sites in the Los Angeles Basin Using Multiple Continuous Measurements . Aerosol Sci. Technol. , accepted February 2003
- Finlayson-Pitts , B. J. and Pitts , J. N. 1986 . Atmospheric Chemistry: Fundamentals and Experimental Techniques , New York : Wiley .
- Ghio , A. J. , Stonehuerner , J. , Pritchard , R. J. , Piantadosi , C. A. , Quigley , D. R. , Dreher , K. L. and Costa , D. L. 1996 . Humic-like Substances in Air Pollution Particulates Correlate with Concentrations of Transition Metals and Oxidant Generation . Inhal. Toxicol. , 8 : 479 – 494 .
- Heyder , J. , Brand , P. , Heinrich , J. , Peters , A. , Scheuh , G. , Tuch , T. and Wichmann , E. 1–3 May 1996 . “ Size Distribution of Ambient Particles and Its Relevance to Human Health ” . In 2nd Colloquium on Particulate Air Pollution and Health 1–3 May , Park City, Utah
- International Commission on Radiological Protection . 1994 . Human Respiratory Tract Model for Radiological Protection; A Report of Committee 2 of the ICRP , Oxford : Pergamon Press .
- Kim , S. , Jaques , P. , Chang , M. C. , Froines , J. R. and Sioutas , C. 2001a . A Versatile Aerosol Concentrator for Simultaneous In Vivo and In Vitro Evaluation of Toxic Effects of Coarse, Fine and Ultrafine Particles: Part I: Laboratory Evaluation . J. Aerosol Sci. , 11 : 1281 – 1297 .
- Kim , S. , Jaques , P. , Chang , M. C. , Xiong , C. , Friedlander , S. K. and Sioutas , C. 2001b . A Versatile Aerosol Concentrator for Simultaneous In Vivo and In Vitro Evaluation of Toxic Effects of Coarse, Fine and Ultrafine Particles: Part II: Field Evaluation . J. Aerosol Sci. , 11 : 1299 – 1314 .
- Kim , S. , Shi , S. , Zhu , Y. , Hinds , W. C. and Sioutas , C. 2002 . Size Distribution, Diurnal and Seasonal Trends of Ultrafine Particles in Source and Receptor Sites of the Los Angeles Basin . J. Air Waste Mgmt. Assoc. , 52 : 174 – 185 .
- Li , N. , Sioutas , C. , Cho , A. , Schmitz , D. , Misra , C. , Wang , M. , Oberley , T. , Froines , J. R. and Nel , A. 2003 . Ultrafine Particulate Pollutants Induce Oxidative Stress and Mitochondrial Damage . Environ. Health Perspect. , 111 : 455 – 460 .
- Lippmann , M. 1977 . “ Regional Deposition of Particles in the Human Respiratory Tract ” . In Handbook of Physiology, Reaction to Environmental Agents , Edited by: Lee , D. H. K. , Falk , H. L. , Murphy , S. O. and Geiger , S. R. Bethesda, MD : American Physiological Society .
- Loo , B. W. and Cork , C. P. 1988 . Development of High Efficiency Virtual Impactors . Aerosol Sci. Technol. , 9 : 167 – 176 .
- Misra , C. , Kim , S. , Shen , S. and Sioutas , C. 2002 . A High Flow Rate, Low Pressure Drop Impactor for Inertial Separation of Ultrafine from Accumulation Mode Particles . J. Aerosol Sci. , 33 : 735 – 752 .
- Oberdörster , G. , Ferin , J. , Gelein , R. , Soderholm , S. C. and Finkelstein , J. 1992 . Role of Alveolar Macrophage in Lung Injury; Studies with Ultrafine Particles . Environ. Health Perspect. , 102 : 173 – 179 .
- Peters , A. , Dockery , D. W. , Heinrich , J. and Wichmann , H. E. 1997 . Short-term Effects of Particulate Air Pollution on Respiratory Morbidity in Asthmatic Children . Eur. Respir. J. , 10 : 872 – 879 .
- Whitby , K. T. and Svendrup , G. M. 1980 . California Aerosols: Their Physical and Chemical Characteristics . Adv. Environ. Sci. Technol. , 10 : 477
- Zhang , X. Q. and McMurry , P. H. 1987 . Theoretical Analysis of Evaporative Losses from Impactor Surfaces . Atmos. Environ. , 21 : 1779 – 1789 .