Abstract
A single-cylinder engine diesel exhaust exposure system was constructed to conduct laboratory health effects studies. The single-cylinder engine was selected to provide a cost-effective and easy-to-operate biological exposure system as an alternative to larger engines operated on chassis or test stand dynamometers. The engine was a 5500-watt diesel generator operated at load by connection to a bank of 11 500 watt light sources. The engine was isolated from the rodent and cell exposure chambers to ameliorate excessive noise and heat in the exposure room. Exhaust was diluted approximately 100:1 and routed to a 1 m3 flow-through exposure chamber where the exhaust composition was assessed in detail. Measurements included particle mass, particle size distribution (based on both number and mass), carbon monoxide, nitrogen oxides, particle carbon (organic and elemental), particle sulfate/nitrate, metals, total and speciated volatile hydrocarbons, and speciated semivolatile and particle-phase polycyclic aromatic hydrocarbons. The exhaust composition was compared with published diesel exhaust compositions and that from a 2000 Model Cummins 5.9L ISB engine, which was operated on a variable duty cycle on a dynamometer using the same fuel and lube oil as the single-cylinder engine. The exhaust composition from the single-cylinder engine was consistent with “typical” diesel exhaust and the larger on-road engine. It yielded particle mass with a mass median diameter of ∼0.1 𝛍m that was composed of ∼70% elemental carbon, ∼35% organic carbon, ∼4–5% sulfate/nitrate, and small amounts of metals. The volatile and speciated organic/inorganic gases were in the range of literature values, with specific characteristics of low particle bound aromatic compounds and higher amounts of volatile and oxygenated organics. Based on this evaluation, the single-cylinder engine and exhaust dilution system described here generates emissions that should be useful for studying health hazards that pertain to emissions from a broad range of diesel engines.
INTRODUCTION
Engine, fuel, and after-treatment technologies have progressively reduced diesel engine emissions (DEE), but they continue to be a health concern and thus a continued research focus (CitationLloyd and Cackette 2001; CitationMauderly 2001; CitationEPA 2002; CitationBunn et al. 2002). Though DEE health research has largely emphasized cancer effects, it has also included lung and airway inflammation, amplification of respiratory allergies, and reduced resistance to respiratory infection (CitationDiaz-Sanchez et al. 1997; CitationMauderly 1998; CitationSydborn et al. 2001; CitationHarrod et al. 2003). Both particulate matter (PM) and non-PM components of DEE appear to be important to observed health responses, but their relative contributions to different effects are uncertain. Recent evidence suggests that the composition of the PM and non-PM components will affect the toxic potency per unit of exposure to mobile source emissions (CitationSeagrave et al. 2002), illustrating the importance of characterizing exposures well in order to place their health effects in context.
Considering the range of research needs relating to DEE toxicity (CitationMauderly 2001), a wider range of health laboratories with capabilities to expose humans, animals, and cells to whole diesel emissions under realistic, standardized conditions is needed. Studies using real-time exposures to DEE have been limited to a few laboratories that can operate engines on test stands or chassis dynamometers. A simpler, less expensive system would be useful if it produced emissions similar to those generated by larger on-road and off-road engines. Of course, the composition of DEE is affected by many variables, and thus a single engine cannot model all conditions. However, if a system is well characterized the exposure can be placed in context of typical exhaust, and the relevance of the health outcomes can be better evaluated.
The goal of this work was to characterize emissions from a small, single-cylinder diesel engine (SCDE) for potential use in health studies. An engine was selected that has proven useful for conducting bench-scale studies of lubrication and producing results that could be extrapolated to larger on-road and off-road engines (CitationLue et al. 2001; CitationPerez and Boehman 2001). A dilution/exposure system was constructed, and the diluted emissions from the engine were characterized at the point of exposure. The emission composition was compared to that of DEE from a common contemporary on-road engine used in a previous inhalation study, to off-road DEE measured in an underground mine, and to other reported DEE composition.
EXPERIMENTAL METHODS
Engine and Engine Operation
A schematic of the DEE generation/exposure system is presented in . DEE was produced by a 5500 Watt SCDE generator (Yanmar, Model YDG 5500E) that contains a 406 cc displacement air-cooled engine. The engine was operated using Number 2 Diesel Certification Fuel (Phillips Chemical Company, Borger, TX) and 40 weight motor oil (Rotella T, Shell, Houston, TX). A bank of 11 500 watt halogen lights pulled electrical current from the SCDE to provide a constant 100% rated load during operation. This operating mode was selected based on similarity in the PM color on a filter when compared with PM samples from a multicylinder diesel engine (MCDE) operated on a cycle (see MCDE description below).
Dilution/Exposure System
The SCDE/exposure system was placed in a basement below the exposure laboratory to isolate temperature and noise. Stainless steel plumbing was utilized to transit and dilute the engine exhaust. The exhaust line was welded directly from the engine to a 5.1 cm diameter tube housed in fiberglass insulation. After transiting approximately 5 meters (transit time < 2 s), the exhaust was diluted, and a portion of the diluted exhaust was removed (dumped) prior to a secondary dilution stage. Typically these primary dilution and dump lines operate at ∼500–700 L/min. All exhaust was diluted with ambient air preconditioned by passage through a charcoal/HEPA filter bank to remove volatile and particle-bound contaminants, and then tempered to ∼20°C by passage through a conditioning system. After the second dilution stage, the insulation ended, and the diluted exhaust transit line expanded to a 15.2 cm diameter flow-through manifold with several ports that allow extraction of exhaust to exposure systems.
Although several extraction ports were available on this manifold, only three are shown in . Two of these lines (full description only shown for one) transited material for whole-body exposure systems, and a separate line could be used for in vitro exposures. A series of dilution and air dumps preceded the whole-body and cell exposure system. Material was extracted from the manifold at approximately 50 L/min, and dilution air was added to achieve a desired total flow through the exposure system. The animal exposure chamber was a conventional 1 cubic meter whole-body inhalation chamber (Hazleton H-1000) operated at a flow rate of approximately 250 L/min. Temperature, relative humidity, and flow (orifice plate mated to electronic pressure transducer) were monitored and recorded at all times in the whole-body exposure system by LabView (version 6) software. DEE concentrations were adjusted manually for dilution and air dumps in the air transit system. The dilution and dump flow rates on the sample line going to the exposure chamber were typically operated at ∼30–50 L/min. DEE concentration was controlled by diluting to a predetermined PM concentration.
Contemporary On-Road Engine Emissions
Emissions from a 2000 model Cummins 5.9L ISB engine were collected from an exposure chamber at the same PM concentration used for the SCDE characterization. The operation and configuration of the Cummins engine and dilution system have been described (CitationMcDonald et al. 2001). In brief, the engine was mated to an eddy current dynamometer (Alpha-240, Kiel, FRG) and operated on a slightly modified (no motoring) EPA Engine Dynamometer Schedule for Heavy-Duty Diesel Engines (CitationU.S. EPA, 1981). The engine was operated with the same fuel and oil as used in the SCDE described above.
CHARACTERIZATION OF EXHAUST COMPOSITION
Sampling Approach
Samples were collected directly from the exposure chambers at dilutions that produced a PM concentration of 1 mg/m3. The analytes, sampling approach, analytical technique, and sampling conditions are summarized in and detailed below for each technique. All sample transit lines were composed of either stainless steel (for PM) or Teflon (for gases), and all sample flow rates were monitored with standards traceable to the National Institute of Standards and Technology (NIST). Flow rates <2 L/min were monitored with a dry-cell flow meter (Gillian, Sensidyne Inc., St. Petersburg, FL), while higher flow rates were measured with a hot wire anemometer (TSI, Inc., Model 4000).
TABLE 1 Summary of measurements, measurement conditions, and analytical techniques used to characterize single-cylinder exhaust composition
Particle Mass
PM concentration was measured gravimetrically by sampling for 30 min intervals on 47 mm Pallflex (Pall-Gelman) filters connected to the exposure chamber with a 20.3 cm long by 0.6 cm internal diameter (i.d.) probe connected to an aluminum in-line filter holder (In-Tox Products, Inc.). Pre- and postsample filter weights were measured using a Mettler MT5 balance. A static discharger was used prior to weighing to avoid any interference from electrical charge on the filters.
Real-Time Particle Mass
Real-time PM was measured using a DustTrak Nephelometer (TSI, Inc.) to assess system stability and to use as an indicator of chamber concentration in order to adjust dilutions for hitting the target mass concentration. The instrument withdrew material from the chamber through the same type of probe as described for PM. The instrument response was calibrated in advance against filter mass data.
Particle Size
Particle number size distribution from ∼14–700 nm was measured using a scanning mobility particle sizer (SMPS; Model 3080, TSI, Inc.). The SMPS was operated at a sample flow rate of 0.3 L/min and a sheath flow rate of 3 L/min. Particles were counted from the SMPS exit using a condensation particle counter (Model 3025, TSI, Inc.). SMPS data were analyzed with Aerosol Instrument Manager (Version 5.0, TSI, Inc.). PM size distribution from ∼0.05–10 μm was measured using a microorifice-uniform deposit impactor (MOUDI; MSP Corp.) using lightly greased aluminum impaction substrates, as described by CitationMarple et al. (1991). MOUDI samples were withdrawn from the chamber through a 20.3 cm long by 1.3 cm i.d. probe.
Gas Analysis
Total nitrogen oxides (NOx = NO + NO2) were measured using a chemiluminescent analyzer (API Model 200A) that was zeroed with ultrazero air and calibrated with NIST traceable span gases. CO concentrations were determined using a photoacoustic gas analyzer (Innova 1312). The manufacturer performed the initial calibration, and calibration was checked on site against National Institute of Standards Technology (NIST) traceable gases. Total hydrocarbons (THC) were measured using a real-time flame ionization detector (Analytical Instruments, Model 300H) calibrated against a certified propane standard.
Metals
Samples for metal determination were collected through a 15.2 cm long by 1.3 cm i.d. diameter probe onto a Teflon (Pall-Gelman, 2.0 μm pore size, 47 mm diameter) filter that was housed in a Teflon coated filter cartridge (URG, Inc.). Metals were analyzed at Carlsbad Environmental Monitoring and Research Center (Carlsbad, NM) by inductively coupled plasma mass spectrometry (Perkin Elmer Elan 6000) following EPA method 200.8. Filters (sample plus field blanks, which went through same filter handling process as samples) were digested by microwave (Model MDS 2100, CEM Corp.) in a cocktail of nitric acid (7 mL), hydrochloric acid (3 mL), ultrapure water (5 mL), hydrofluoric acid (5 mL), and hydrogen peroxide (2 mL). Samples were digested for 30 min, allowed to cool, and transferred to 50 mL centrifuge cones to be diluted to 50 mL with ultrapure water. This digestate was analyzed directly without dilution. Quantitation was conducted with standards purchased from CPI International.
Carbon and Inorganic Ion
Organic carbon/elemental carbon (OC/EC) and SO−2 4/NO− 3 were measured on quartz filters (Pall-Gelman, QAO, 47 mm diameter, heat treated) collected using the same type of probe as for PM sampling. Prior to sampling, quartz filters were precleaned by baking for 6 h in a furnace at 900°C. Desert Research Institute (DRI; Reno, NV) analyzed the OC/EC and ions. Samples and field blanks were shipped under the same conditions to assess background or contamination. At DRI, filters were divided, and a 1 cm2 punch from one-half of the filter was used for OC/EC analysis, while the other half was used for ion analysis. OC/EC was determined by thermal/optical reflectance (CitationChow et al. 1993) and inorganic ions by ion chromatography (CitationChow and Watson 1999). The OC measurement only quantitated the carbon component of organic carbon species; thus, mass concentration reported by this method did not reflect contributions from hydrogen, oxygen, and other elements associated with OC species in the air. To estimate the actual OC mass concentration, a correction factor of 1.2 was applied as recommended by CitationPierson and Brachaczek (1983) for organic mass (OM) emitted from vehicles. The OC concentration reported here is thus defined as OM.
Speciated Volatile Organics
Volatile organic speciation of alkanes, alkenes, alkynes, and aromatic compounds was conducted by DRI according to canister sampling/analysis methods as described (CitationZielinska et al. 2001). In brief, samples were collected in evacuated stainless steel SUMMA® canisters that were analyzed by gas chromatography with flame ionization detection (Hewlett-Packard 5890 Series II) using three separate methods to target the following: (1) methane, (2) light hydrocarbons with good resolution of compounds from ∼C2–C5, and (3) C2–C12 hydrocarbons with less resolution of the low molecular weight compounds but a larger range of compounds.
Volatile carbonyl compounds were collected on C18 Sep-Pak (Waters, Inc., Midford, MA) cartridges impregnated with 2,4-dinitrophenylhydrazine. These cartridges trap the carbonyl compounds as hydrazones which are eluted after sampling with 2 mL of analytical grade acetonitrile and analyzed by high-pressure liquid chromatography (Hewlett Packard 1050) with ultraviolet detection at 365 nm. The solvents and analytical conditions used for the HPLC analysis are listed in .
TABLE 2 Analytical conditions for analysis of carbonyl compounds
Speciated Semivolatile/Particle Bound Organics
Material for speciated particle and semivolatile organic analysis was collected on a Teflon-impregnated glass fiber (Pallflex, 100 mm) filter backed by a 5.1 cm × 10.2 cm polyurethane foam (PUF) (Tisch Environmental, Inc.)/XAD-4 (10 g) (Fisher Scientific, Pittsburg, PA)/PUF sandwich cartridge. The PUF was cleaned prior to use with a sequential soxhlet extraction (24 h) with a 90:10 hexane/ether mix, then by acetone. XAD-4 was cleaned by rinsing it with methanol, followed by soxhlet extraction with dichloromethane, followed by acetone. After sampling, PUF/ XAD-4/PUF cartridges were soxhlet extracted for 24 h in hexane/ether (90:10) followed by 12 h in acetone. Filters were soxhlet extracted for 12 h in dichloromethane followed by 12 h in acetone. All solvents were analytical grade (Fisher Scientific, Pittsburg, PA). Prior to extraction, samples were spiked with a suite of deuterated internal standards closely resembling the chemical structures and behavior of the analytes of interest. These internal standards mimicked the behavior of the target analytes throughout the extraction process. The internal standards used included deuterated naphthalene, phenanthrene, acenaphthene, chrysene, benzo(a)pyrene, and dibenz(a,h)anthracene. After extraction, solvents were concentrated by rotary evaporation and filtered through a 0.2 μm acrodisc filter (Waters, Inc.). Extracts were then evaporated to ∼100 μL under a gentle nitrogen stream and brought to 0.1 mL (for personal samples) or 1 mL (for ambient samples) with acetonitrile prior to analysis.
Splitless injections (1 μL) were made onto a gas chromatograph-mass spectrometer (HP 5890 GC, 5972 MS) equipped with a phenylmethylsilicone fused-silica capillary column (30 m, 0.25 mm × 0.25 Mm; DB-5 ms, J&W Scientific). The operating conditions were as follows: 65°C for 2 min; 12°C/min to 180°C; 8°C/min to 320°C; hold at 320°C for 10 min.
Samples were quantified by comparing the response of the deuterated internal standards to the analyte of interest. When a deuterated internal standard was not available, quantitation was based on the most similar internal standard compound structure and retention time. Analyte response was referenced to calibration curves created from standard solutions made with authentic standards (Aldrich, Inc., Milwaukee, WI) and the NIST Standard Reference Material (SRM 2260).
Data Analysis
lists the chemical components sorted by chemical class and designated as gaseous, semivolatile, or particle-associated. Elements (metals and other ICPMS analytes) measured at low amounts or below the analytical uncertainty are not presented. In addition, several individual components were summarized by chemical class and not listed individually. Data not presented are available in the Appendix to this article. The semivolatile components were defined as organic compounds collected and analyzed on the Filter/PUF/XAD-4/PUF sampling train having a vapor pressure equal to or less than benz(a)anthracene (vapor pressure: 1.1 × 10−7 mm Hg at 25°C). If the vapor pressure was not known, the compound was defined as semivolatile if it eluted before benz(a)anthracene on the gas chromatogram. The data were sorted according to class, analytical method, and measurement uncertainties calculated based on the following formula:
TABLE 3 Chemical composition of diluted emissions from a single-cylinder diesel generator operated at 100% loadFootnote a
RESULTS
shows the fractional abundance of the primary PM constituents (EC, OM, inorganic ions [mostly sulfate], and metals) in diluted exhaust from SCDE and MCDE, and in “typical” diesel exhaust as defined by CitationEPA (2002). The data reported by CitationEPA (2002) are an average encompassing typical exhaust, and thus represent means of a wide range of values. As shown, the PM from the SCDE operated at full load had a bulk particle composition similar to that of both “typical” diesel exhaust and the MCDE. This PM consisted primarily of EC and OM, with small amounts of inorganic ions (∼1% of PM) and metals (sum ∼0.2% of PM). The only observable metals above detection limits were barium, copper, lead, and zinc. Calcium, iron, and silicon were all detected, but concentrations were within the range of analytical uncertainty.
FIG. 2 Fractional abundance of the primary components of diesel exhaust particulate matter in diluted exhaust from (1) a Cummins multicylinder engine operated on an engine cycle (CitationMcDonald et al. 2001), (2) the single-cylinder engine operated at steady state, and (3) “typical” diesel exhaust as defined by the CitationEnvironmental Protection Agency (2002).
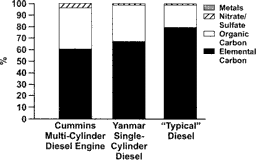
compares several DEE components, including OM and EC, among the SCDE, MCDE, and an underground mine atmosphere that contained DEE contributions from a range of vehicles. The underground mine data were taken from CitationMcDonald et al. (2002). The mine atmosphere consisted of a DEE and suspended rock dust mixture, with the majority of the mass (>90%) being DEE; samples were collected with a 2.5 micron cut point to reduce the dust contribution. The PM concentrations in the mine were within 20% of the concentrations to which the SCDE and MCDE were diluted, allowing direct comparisons among the three. The mine atmosphere contained OM and EC concentrations in the same range as those in the SCDE and MCDE chamber atmospheres.
TABLE 4 Comparison of the particle carbon and speciated semivolatile/particle bound organics in emissions from two engines and an underground mine measured at similar particle mass concentrations
The most abundant gases measured were CO (10 ppm), NOx (12 ppm), and THC (4 ppm). The sum of measured volatile organic species amounted to ∼3 ppm, which is in reasonable agreement with the THC measurement, considering the THC calibration/response was based on propane. The volatile THC was composed primarily of C1–C2 volatile organics, but measurable amounts of several compounds from several chemical classes were observed. The compound classes were present in an order of alkene > carbonyl > alkane > alkyne > aromatic > cycloalkane > cycloalkene (). These proportions are in reasonable agreement with published proportions of volatile organics in diesel exhaust (e.g., CitationSchauer et al. 1999; CitationSchmitz et al. 2000). CitationSchauer et al. (1999) reported carbonyl> alkene> alkane>aromatic emissions from two medium-duty diesel engines operated on chassis dynamometers using commercial California reformulated diesel fuel, while CitationSchmitz et al. (2000) reported alkene > carbonyl > aromatic > alkyne > alkane emissions from a contemporary light-duty diesel operated with unspecified European fuel. The specific emission rates were different between these studies, but the aromatic, olefin, alkane, and alkene concentrations were within 15% of each other. This contrasts with the emissions from the SCDE, where aromatic emissions were approximately five times lower than the most abundant organic chemical class. Several previous studies (reviewed in CitationEPA 2002) have shown that while emissions of aromatic compounds from diesel combustion are related to several factors (fuel, engine, engine operation, etc.), they are most strongly related to fuel aromatic content. The Schauer and Schmitz studies did not give information on the total aromatic content of their diesel fuels. It is possible that the aromatic content of the fuel used for the present study (29 volume %) was lower than that of the fuels used in those studies. shows a comparison of four volatile aromatics (benzene, toluene, ethyl benzene, m- & p-xylene) measured in emissions at 1000 μg/m3 PM from the SCDE or the MCDE. As mentioned previously, the SCDE and MCDE were operated with the same fuel, and there is good agreement for toluene, ethyl benzene, and m- & p-xylene, and reasonable agreement (factor of two) for benzene. The difference in benzene likely reflects the difference in the operation of the two engines (100% steady-state load versus a cycle).
FIG. 3 Volatile organic compound classes emitted from the single-cylinder diesel engine presented as a fraction of the sum of all measured volatile organic compounds.
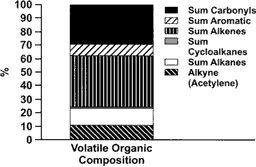
FIG. 4 Concentration of benzene, toluene, ethyl-benzene, and m- and p-xylene from the Yanmar single-cylinder diesel engine exhaust and a Cummins 5.9L (CitationMcDonald et al. 2001). Both engines were operated with the Number 2 diesel certification fuel, and exhausts were diluted to a particle mass concentration of 1000 μg/m3
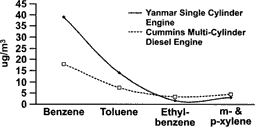
The semivolatile/particle-phase organic components measured included several classes of polycyclic aromatic hydrocarbons (PAH). Some of these compounds are present exclusively as gases and some are primarily present in the particulate phase. Several of these compounds in the intermediate vapor pressure range are present in the gas and particle phases at the same time, and are termed semivolatile. The portion of these compounds present in the gas or particle phases will depend on the environmental conditions. For this study, no effort was made to directly measure the distinction between the amounts of compound present in the gas or particle phase, as the sampling approach collected both fractions simultaneously, and the solvent extracts were combined for final analysis. As indicated above, an estimation of phase is given in based on vapor pressure and compound retention time on a gas chromatogram. Similar to previously reported PAH emissions from diesel exhaust (e.g., CitationLowenthal et al. 1994; CitationSchauer et al. 1999), the PAH concentrations were dominated by the semivolatile species, especially the naphthalene class of compounds. In fact, almost all of the nonoxygenated particle-phase PAH were at or below the analytical detection limits (∼5.8 ng/m3), as demonstrated by concentrations within the range of uncertainty.
PAH concentrations in the diluted SCDE, MCDE exhaust, and the underground mine atmosphere were similar with the total measured PAH within 20% of each other (). While most of the individual compounds varied more than this, most of the PAH concentrations were at least within a factor of two of each other.
Particle size was characterized by both mass (0.05–10 microns [50% cut point]) and number distributions (). Mass distributions were presented as the fractional mass amount (dm/m total) for each incremental size (dlogdp). Since the MOUDI stages are 50% cut points, and the mass on each stage overlapped the higher and lower cut points, aerodynamic diameters are represented as the geometric means between impactor cut points. The mass distribution showed that >85% of the PM was below 1 micron in aerodynamic diameter with a mass median diameter of ∼100 nm. The number count median diameter was 80 nm. This number median diameter was slightly less than the mass median diameter because the smaller diameter particles weigh less than larger diameter particles, contributing less to the total mass amount.
DISCUSSION
A SCDE exposure system was constructed, and the emissions were characterized in sufficient detail to place this system in context of “typical” DEE and exhaust from a MCDE. The ultimate goal of this work was to evaluate the utility of this system for health studies that aim to represent exposures to on- or off-road diesel vehicle emissions. The comparisons made among the SCDE, MCDE, and an underground mine facilitated this evaluation. While the specific emission rates from the range of vehicles encompassed by these comparisons varied by orders of magnitude, the common concentrations of DEE PM in the mine and the inhalation chambers allowed a unique comparison of material concentrations in animal laboratory exposures and human occupational exposures.
This study showed that a SCDE operated at 100% load yielded emissions reasonably consistent with what is considered “typical” DEE, and the composition was similar to emissions from the MCDE and to the mine atmosphere. The SCDE configuration and operation described here should provide a valuable and reasonably cost-effective research tool for studies investigating the relationship between inhaled DEE and health. However, researchers that implement this type of system should be aware that no single DEE generation system or operating mode can represent the full range of environmental DEE.
The primary PM constituents (carbon [OM/EC], ions) emitted from the SCDE were similar both to “typical” exhaust (CitationEPA 2002) and MCDE-generated exhaust. It is important to note that the similar proportions of total OM imply, but do not confirm, that the OM compositions are similar. OM from DEE is composed of thousands of individual components, and all of the OM constituents from DEE have not been completely identified by any laboratory to date due to technical challenges. The particle-bound PAH measured here accounted for a small portion of the total OM but are an important metric because of their potential importance in health studies and because they are among the most studied class of emissions from diesel exhaust.
The composition of the PAH from the SCDE was similar to that of published PAH emissions, emissions from a MCDE engine, and an underground mine atmosphere. Concentrations of particle-phase PAH compounds such as benzo(a)pyrene at or below the limits of detection were characteristic of all of these conditions. Benzo(a)pyrene has received considerable attention as a component of diesel exhaust because of its mutagenic potential, but it is actually emitted in low amounts relative to other PAH and is close to the analytical detection limits for many heavy-duty diesel vehicles (e.g., CitationZielinska et al. 1998; CitationMcDonald et al. 2002). Several of the oxygenated PAH were measured at concentrations above many of the nonoxygenated compounds, which may be of interest because of the specific toxicity of quinoid and other oxygenated compounds (e.g., CitationBolton et al. 2000). For example, the sum of these compounds was higher than that of phenanthrenes. CitationSchauer et al. (1999) measured some of these compounds, but data published on oxygenated PAH from DEE are sparse, so additional comparisons are not available.
Metal emissions in DEE arise from fuel/lube additives, engine/exhaust system wear, and metals taken into the engine from resuspended soil, asphalt, or other airborne materials (CitationDocekal et al. 1992; CitationLighty et al. 2000). Emissions of metals from on-road vehicles have ranged from a fraction of a percent to up to ∼5% of the total DEE PM (CitationEPA 2002). In the SCDE system described herein, metal emissions are low because a new engine was placed indoors, and the intake air was not enriched in resuspended metals. This same phenomenon is likely observed in all engine studies conducted with new engines on test stands in controlled environments, resulting in DEE studies that have traditionally been conducted with proportions of metals lower than what is typical of on-road emissions, including resuspended dust. The metals observed here (barium, copper, lead, zinc, calcium, iron, silicon) were all typical of DEE (CitationDocekal et al. 1992; CitationEPA 2002), and the total percent metal emitted (∼0.2% of total PM) was similar to several studies conducted on chassis dynamometers (e.g., CitationLowenthal et al. 1994; summarized in CitationEPA 2002).
The composition of the volatile organic compounds from the SCDE was generally similar to published results. The magnitude of the differences between summed chemical classes (e.g., aromatic versus alkane) was greater here than observed by CitationSchauer et al. (1999) and CitationSchmitz et al. (2000), but concentrations were consistent with exhaust generated from a 2000 Cummins 5.9L ISB engine operated on a variable cycle. Several air toxics known to be present in diesel exhaust and likely or possible human carcinogens (CitationEPA 2002) are among the over 60 volatile organics measured here. These toxics include benzene, formaldehyde, 1,3-butadiene, acetaldehyde, and acrolein. Similar to other reports on these compounds in DEE, their concentrations in SCDE exhaust were relatively low. For example, 1,3 butadiene was only 0.64 μg/m3, or 0.06% as a fraction of the PM emissions. In comparison, 1,3 butadiene was 0.1% as a fraction of the PM emissions in the work by CitationSchauer et al. (1999) from a larger engine operated on a dynamometer.
Particle size distribution is an important characteristic of diesel exhaust because of the strong interest in the health effects of ultrafine- and nanoparticle-size particles that encompass much of the DEE PM in environmental samples (CitationKittelson 1998). The particle size distribution reported by CitationKittelson (1998) was virtually identical to the PM size distribution the SCDE exhaust in the present study. Though not explicitly tested here, this size distribution may be attributed to both the similar particle formation dynamics in the SCDE relative to large engines and to the dilution approach used here (dilute ∼ 100X within 1–2 s). The particle number median diameter was larger than described by CitationKittelson (1998); Kittelson described a typical number median diameter in the 20 nm range compared to 80 nm measured from the SCDE. However, the particle number size distribution was similar to the “signature size distributions” described by CitationHarris and Maricq (2001), who showed particle number distributions with medians in the 70–100 nm range. Particle size measurements from diesel exhaust range widely throughout the literature because these measurements depend on several factors such as dilution rate, humidity, concentration, temperature, etc. (CitationEPA 2002).
In summary, the DEE generation and exposure system described herein should provide a cost-effective approach to conducting inhalation exposures to diluted DEE having a composition falling well within the range of on- and off-road diesel vehicles.
Acknowledgments
The authors gratefully acknowledge the contributions of Desert Research Institute—especially Barbara Zielinska and Judith Chow—and the Carlsbad Environmental Monitoring and Research Center for sample analysis. Lovelace Respiratory Research Institute (LRRI) staff members Mitchell Irvin and Mark Gauna aided in system characterization, and Erin Caldwell assisted with exposure system construction. Paula Bradley and the LRRI Technical Communications staff assisted with edits and final manuscript formatting. This work was supported by the Office of Freedom Car and Vehicle Technologies, U.S. Department of Energy. This manuscript was prepared as an account of work sponsored by an agency of the U.S. Government. Neither the U.S. Government nor any agency thereof, nor any of their employees, makes any warranty, expressed or implied, or assumes any legal liability or responsibility for the accuracy, completeness, or usefulness of any information, apparatus, product, or process disclosed, or represents that its use would not infringe on privately owned rights. Reference herein to any specific commercial product, process, service by trade name, trademark, manufacturer, or otherwise does not necessarily constitute or imply its endorsement, recommendation, or favoring by the U.S. Government or any agency thereof. The views and opinions of the authors expressed herein do not necessarily state or reflect those of the U.S. government or any agency thereof. No copyright is asserted in the works of U.S. Government employees.
Notes
a Uncertainty estimates based on replicate precision and analytical detection limits.
b These are a partial list of the measured components of this chemical class.
a Underground mine data from CitationMcDonald et al. (2002). Mine samples were collected with a 2.5 micron size cut, but a portion of the mass (∼10%) was still attributed to resuspended rock dust.
b n = 2.
c n = 11.
d n = 8.
e n = 5.
f n = 7.
REFERENCES
- Bolton , J. L. , Trush , M. A. , Penning , T. M. , Dryhurst , G. and Monks , T. J. 2000 . Role of Quinones in Toxicology . Chem. Res. Toxicol. , 13 : 135 – 160 .
- Bunn , W. B. , Valberg , P. A. , Slavin , T. J. and Lapin , D. A. 2002 . What is New in Diesel . Int. Arch. Occup. Environ. Health , 75 ( Suppl. ) : S12 – S132 .
- Chow , J. C. , Watson , J. G. , Pritchett , L. C. , Pierson , W. R. , Frazier , C. A. and Purcell , R. G. 1993 . The DRI Thermal/Optical Reflectance Carbon Analysis System: Description, Evaluation and Applications in U.S. Air Quality Studies . Atmos. Environ. , 27A : 1185 – 1201 .
- Chow , J. C. and Watson , J. G. 1999 . “ Ion Chromatographic Analysis of Ambient Air Samples ” . In Elemental Analysis of Airborne Particles , Edited by: Landsberger , S. and Creatchman. , M. 67 – 96 . Newark, NJ : Gordon and Breach Publishers .
- Diaz-Sanchez , D. , Tsien , A. , Fleming , J. and Saxon , A. 1997 . Combined Diesel Exhaust Particulate and Ragweed Allergen Challenge Markedly Enhances Human In Vivo Nasal Ragweed-Specific IgE and Skews Cytokine Production to a T Helper Cell 2-Type Pattern . J. Immunol. , 158 : 2406 – 2413 .
- Docekal , B. , Krivan , V. and Pelz , N. 1992 . Trace Minor Element Characterization of Diesel Soot. (1996) . Fresenius J. Anal. Chem. , 343 : 873 – 878 .
- EPA (U.S. Environmental Protection Agency) . 2002 . Health Assessment Document for Diesel Engine Exhaust Prepared by the National Center for Environmental Assessment Washington DC for the Office of Transportation Air Quality; EPA/600/8-90/057F. Available from: National Technical Information Service Springfield VA; PB2002-107661
- Harris , S. J. and Maricq , M. M. 2001 . Signature Size Distributions for Diesel and Gasoline Engine Exhaust Particulate Matter . J. Aerosol Sci. , 32 : 749 – 764 .
- Harrod , K. S. , Jaramillo , R. J. , Rosenberger , C. L. , Wang , S. Z. , Berger , J. A. , McDonald , J. D. and Reed , M. D. 2003 . Increased Susceptibility to RSV Infection by Exposure to Inhaled Diesel Engine Emissions . Am. J. Respir. Cell Mol. Biol. , 28 : 451 – 465 .
- Kittelson , D. B. 1998 . Engines and Nanoparticles: A Review . J. Aerosol Sci. , 29 ( 5/6 ) : 575 – 588 .
- Lighty , J. S. , Veranth , J. M. and Sarofim , A. F. 2000 . Combustion Aerosols: Factors Governing Their Size and Composition and Implications to Human Health . J. Air & Waste Manage. Assoc. , 50 : 1565 – 1618 .
- Lloyd , A. C. and Cackette , T. A. 2001 . Diesel Engines: Environmental Impact and Control . J. Air Waste Manage. Assoc. , 51 : 809 – 847 .
- Lowenthal , D. H. , Zielinska , B. , Chow , J. C. , Watson , J. G. , Gautam , M. , Ferguson , D. H. , Neuroth , G. R. and Stevens , K. D. 1994 . Characterization of Heavy-Duty Diesel Vehicle Emissions . Atmos. Environ. , 28 ( 4 ) : 731 – 743 .
- Lue , Y.-F. , Yeh , Y.-Y. and Wu , C.-H. 2001 . The Emission Characteristics of a Small D.I. Diesel Engine Using Biodiesel Blended Fuels . J. Environ. Sci. Health A , 36 ( 5 ) : 845 – 859 .
- Marple , V. A. , Rubow , K. L. , Anath , G. P. and Fissan , H. J. 1991 . Micro-Orifice Uniform Deposit Impactor . J. Aerosol Sci. , 17 : 489 – 494 .
- Mauderly , J. L. 1998 . “ Toxicology of Diesel Engine Emissions ” . In Health Effects of Particulate Matter in Ambient Air , Edited by: Vostal , J. J. 573 – 580 . Pittsburgh, PA : Air & Waste Management Association and the Czech Medical Association .
- Mauderly , J. L. 2001 . Diesel Emissions: Is More Health Research Still Needed? . Toxicol. Sci. , 62 : 6 – 9 .
- McDonald , J. D. , Costanzo , J. , Barr , E. B. , Mauderly , J. L. , Schauer , J. J. , Zielinska , B. , Sagebiel , J. C. , Chow , J. C. , Grosjean , D. and Grosjean , E. Characterization of Laboratory Exposure Atmospheres for Health Effects Studies. CD-ROM . Proceedings of Air and Waste Management Annual Conference . June 24–28 2001 , Orlando, FL.
- McDonald , J. D. , Zielinska , B. , Sagebiel , J. C. and McDaniel , M. R. 2002 . Characterization of Fine Particulate Material in Ambient Air and Personal Exposure Samples from an Underground Mine . Aerosol Sci. and Technol. , 36 ( 11 ) : 1033 – 1044 .
- Perez , J. and Boehman , A. Penn State Multi-Discipline Tribology Group and Energy Institute Studies . Proceedings of the 2001 Diesel Engine Emissions Reduction Workshop . Office of Transportation Technologies, U.S. Department of Energy . DOE/EE-DEER2001-CD
- Pierson , W. R. and Brachaczek , W. W. 1983 . Particulate Matter Associated with Vehicles on the Road 2 . Aerosol Sci. Technol. , 2 : 1 – 40 .
- Schauer , J. J. , Kleeman , M. J. , Cass , G. R. and Simonet , B. R. T. 1999 . Measurement of Emissions from Air Pollution Sources. 2. C1 Through C30 Organic Compounds from Medium Duty Diesel Trucks . Environ. Sci. Technol. , 33 : 1578 – 1587 .
- Schmitz , T. , Hassel , D. and Weber , F.-J. 2000 . Determination of VOC-Components in the Exhaust of Gasoline and Diesel Passenger Cars . Atmos. Environ. , 34 : 4639 – 4647 .
- Seagrave , J. C. , McDonald , J. D. , Gigliotti , A. P. , Nikula , K. J. , Seilkop , S. K. , Gurevich , M. and Mauderly , J. L. 2002 . Mutagenicity and In Vivo Toxicity of Combined Particulate and Semivolatile Organic Fractions of Gasoline and Diesel Engine Emissions . Toxicol. Sci. , 70 : 212 – 226 .
- Sydborn , A. , Blomberg , A. , Parnia , S. , Stenfors , N. , Sandstrom , T. and Dahlen , S.-E. 2001 . Health Effects of Diesel Exhaust Emissions . Eur. Respir. J. , 17 : 733 – 746 .
- U.S. EPA . Title 40 Code of Federal Regulations 81.1, Part 86 , pp. 186 – 241 . . 1981
- Zielinska , B. , McDonald , J. and Hayes , T. 1998 . Northern Front Range Air Quality Study Volume B:Source Measurements , Reno, NV : Desert Research Institute .
- Zielinska , B. , Sagebiel , J. , Harshfield , G. and Pasek , R. 2001 . Volatile Organic Compound Measurements in the California/Mexico Border Region During SCOS97 . Sci. Total Environ. , 276 : 19 – 31 .