Abstract
A high loading sampler for the chemical characterization of fine particles (PM 2.5 ) was developed and validated through laboratory and field experiments. This speciation sampler consists of two identical serially connected impaction stages to remove particles larger than 2.5 μm, following by a chamber to allow use of one or two all-glass honeycomb diffusion denuders, and a holder for a 47 mm filter. Two configurations of the sampler allow sampling at flows of 10 lpm and 16.7 lpm. System performance was evaluated in laboratory experiments using artificially generated polydisperse aerosols. This novel sampler provides a much larger mass loading capacity than previous impactors that use flat, rigid substrate surfaces. The polyurethane foam (PUF) substrate maintains adequate performance characteristics (retention of size cut-off, sharpness of cut-off curve, and minimal particle bounce and re-entrainments) at loadings of at least 35 mg. This is equivalent to 728 μg/m3 for a 48 h sampling period (or 500 h of sampling at 70 μg/m3). System performance was also evaluated in a series of field intercomparison experiments for both flow configurations (10 and 16.7 lpm). Measurements of PM 2.5 mass and sulfate concentrations showed excellent agreement between the US EPA Federal Reference Method (FRM) Sampler and the speciation sampler.
INTRODUCTION
Abundant epidemiological studies have demonstrated the adverse health effects of particulate matter (PM), even at levels far below the National Air Quality Standards. Health effects include both respiratory and cardiovascular mortality and morbidity (CitationGold et al. 1999; CitationKlemm et al. 2000; CitationMurphy et al. 1999; CitationPeters et al. 2000; CitationPope et al. 1999; CitationSimpson et al. 1997; CitationSoukup et al. 2000). Despite the abundance of epidemiological evidence, the specific mechanisms leading to these adverse health effects have not been yet defined.
Therefore, there is a growing need for methods that can be used to measure the different chemical components of fine particulate matter. Various speciation samplers were developed for the physico-chemical characterization of PM2.5 (CitationDemokritou et al. 2001; CitationKoutrakis et al. 1993; CitationSioutas et al. 1996). Such a speciation sampler consists of a conventional impactor to remove particles larger than a cutpoint, following by one or more diffusion denuders to remove selected pollutant gases. Finally the particles are collected on a 47 mm filter placed downstream of the denuder.
Various diffusion denuder systems were developed over the years, such as the annular denuder and the honeycomb glass denuder (CitationKoutrakis et al. 1989, 1993). The later denuder system has a higher capacity than that of an annular denuder. This all-glass honeycomb denuder is a cylinder with a height of 3.8 cm containing hexagonal glass tubes. Two or more denuders can be used in series.
The two limitations associated with the existing speciation samplers are limited collection capacity of the impaction substrate, and particle bounce-off/re-entrainment. In order to minimize particle bounce-off/re-entrainment, impaction substrates are usually coated with adhesives such as mineral oil or grease, both of which have a limited loading capacity (CitationDemokritou et al. 2001; CitationJohn et al. 1991; CitationSehmel 1980; CitationWall et al. 1990). Some researchers have also used a cyclone as the particle separation device to increase loading capacity to as much as 6 mg (CitationKenny et al. 2000). The Well Impactor Ninety-Six (WINS) Impactor, which is used in the EPA Federal Reference Method sampler to collect PM2.5 particles, was found to have a loading capacity of only about 1.5 mg (CitationKenny et al. 2000).
Recently, a new impaction substrate was developed that eliminates the use of adhesives such as grease or mineral oil, while minimizing particle bounce-off and re-entrainment losses. This chemically inert, polyurethane foam substrate (PUF; density = 0.02 g/cm3, Merryweather foam, OH) can be used to collect larger quantities of particles than previously used substrates. For this substrate, without using adhesives such as grease or mineral oil, particle bounce and re-entrainment losses were found to be not significant. This PUF substrate was used in both a high volume cascade impactor (CitationDemokritou et al. 2002b) and a high volume low cutpoint impactor (CitationKavouras et al. 2000) for physicochemical and toxicological characterization of atmospheric aerosols.
In this study, a speciation sampler that is suitable for high loading applications was developed. Main feature of this sampler is its ability to collect large quantities of particles on its PUF substrates with minimal particle bounce and re-entrainment.
METHODS
Design Considerations for Impactors
Inertial impactors have been used extensively for particle collection and size classification (Citationde la Mora et al. 1990; CitationMarple et al. 1987, 1991). A conventional impactor consists of a nozzle for the acceleration of particle-laden gas and a flat, rigid impaction surface (substrate). The basic mechanism for inertial deposition of particles is based on the momentum of the accelerated aerosol particles, and thus their ability to cross the streamlines above the impaction zone or stagnation point. In this region, the streamlines are narrowly spaced. Particles with aerodynamic diameters larger than the impactor's size cutpoint have enough momentum to cross the streamlines and impact onto the substrate while smaller particles, which have insufficient momentum to cross the streamlines, remain suspended in the sample air, and are not collected.
According to impaction theory, the Stoke's number, Stk, is the governing parameter for impaction and is defined as follows:
Inertial impactors have been studied extensively, both theoretically and experimentally. Various guidelines for the critical design parameters were obtained from a numerical fit of the Navier-Stokes fluid flow equations (CitationMarple 1970; CitationMarple and Liu 1974). It has been shown that, for slit-shaped nozzle impactors using a rigid, flat surface as an impaction substrate, the aerodynamic diameter of particles collected with 50% efficiency, d 50, also known as cutpoint or separation point, corresponds to a value of 0.7 for the square root of Stokes number, √Stk. In the case of the new speciation sampler, a nonrigid porous medium (PUF) was used as an impaction substrate. For this substrate, it was shown in experimental impaction studies that d 50 corresponds to a value of √Stk that is much lower than 0.7 (CitationDemokritou et al. 2002b; CitationKavouras et al. 2000; CitationKavouras and Koutrakis 2001). As a result, for the same nozzle geometry and flowrate, lower cutpoints can be achieved as compared to those for rigid, flat plate substrates, and the pressure drop is substantially reduced for the same size cutpoint. In addition, as has been previously reported, the use of polyurethane foam substrates improves the performance of inertial impactors by minimizing bounce-off and re-entrainment losses, as compared to coated and uncoated rigid, flat substrates. Another advantage of a foam substrate is its ability to collect large amounts of particles per unit surface area (CitationDemokritou et al. 2002b; CitationKavouras et al. 2000; CitationKavouras and Koutrakis 2001).
Description of the Speciation Sampler
The speciation sampler (SPESAM) is shown on . It consists of a two identical impaction stages placed in series to remove particles larger than 2.5 μm. One or two all-glass, honeycomb diffusion denuders (with appropriate coatings) can be placed downstream of the impactor to collect selected pollutant gases. Particulate matter is collected downstream on a 47 mm filter. All components (except the denuders) are made of aluminum and are Teflon-coated to minimize losses of gases on the internal surfaces. Different slit acceleration nozzle sections and impaction substrates are used for the 10 and 16.7 lpm configurations of the sampler. The 10 and 16.7 lpm airflow rates were selected to match the airflow characteristics of the Harvard/EPA-Annular denuder System (HEADS) and the Federal Reference Method (FRM), respectively. Both the impactor section and filter holder components are connected to the sampler body with spring clips. The critical impactor design parameters are shown in . The impaction substrate holder for the PUF substrate consists of a hollow aluminum disk with inside diameters of 2.8 and 3.3 cm for the 10 and 16.7 lpm configurations, respectively. Furthermore, the thickness of the polyurethane foam is 0.64 cm to ensure that particles do not penetrate through the PUF and reach the bottom surface of the substrate holder.
TABLE 1 Characteristics of the two-stage impactor
Laboratory Characterization of the Sampler
The experimental setup that was used for the characterization of the impaction section is shown in . The sampler's particle collection efficiency was examined as a function of particle size. Alternating measurements were performed upstream and downstream of the sampler. Both single and double impaction stage arrangements were characterized.
Polydisperse particles were generated by atomizing an aqueous suspension of 2–20 μm hollow glass spheres (density: 1.1 g/cm3, Polysciences, Inc., Warrington, PA) with a Retec Model X-70/N nebulizer at 7 psi of filtered air. For stability of atomization, the nebulizer was maintained at constant conditions. The aerosol was diluted in a mixing chamber (volume of 1.15 × 10−2 m3) with particle-free dry air to evaporate the water from aerosolized glass spheres. The test aerosol was then introduced at the top of a vertical cylindrical duct. Additional filtered room air was also drawn through the top of the duct. Total airflow was 10.0 or 16.7 lpm, depending on the sampler flow configuration. Turbulence was induced near the top of the duct using a rectangular plate, to assure uniform concentration throughout the duct. The sampler was connected at the bottom of this duct.
Particle concentrations were measured for one minute durations using isokinetic sampling probes, alternating between upstream and downstream of the sampler. An Aerodynamic Particle Sizer (APS, Model 3310, TSI Inc., St. Paul, MN) was used to measure particle number concentrations in the size range of 0.6 to 20 μm. The alternating measurements were repeated at least five times to ensure adequate precision. The upstream sampling port was placed approximately eight-duct diameters downstream from the duct entrance. The downstream sampling port was similarly placed on a second duct connected to the outlet of the impaction system. The reproducibility of the measurements was ±5%. The fixed sampling flow rate of 5 lpm drawn by the APS was taken into account when adjusting the flow rate through the isokinetic probes and through the sampler. For each particle size, the sampler's collection efficiency was determined as follows:
The collection efficiency data were fitted using the Boltzmann sigmoidal function (Sigma Plot, SPSS Inc.) as follows:
Inertial particle losses within the sampler may be caused by turbulent flow in the acceleration nozzle region, at flow turns, and at the flow exit/entrance between stages. Particle losses were determined by measuring particles upstream and downstream of the stage, with the substrate removed. It is worthwhile to point out that the collection efficiency for each flow configuration took into account the effects of particle losses.
Particle Loading Capacity Experiment
Further experiments were performed using the same experimental apparatus to investigate the effect of substrate particle loading on the collection efficiency curve. As the loading was built up over time on the substrate, consecutive particle number concentrations were measured upstream and downstream of the sampler, as described above. Particle collection efficiency was calculated based on EquationEquation (3) as a function of loaded mass on the PUF substrates. Loaded mass, M, on substrate was calculated as follows:
Field Validation Experiments
A series of 48 h field validation experiments were performed on the roof of the Harvard Countway Library, in Boston, during September 2002. A PM2.5 FRM sampler, using the WINS system (Rupprecht and Patashnick, Albany, NY) was colocated with four SPESAM samplers. Two were the 10 lpm flow version of the SPESAM and the other two were the 16.7 flow lpm version. The FRM (16.7 lpm) was selected for collocation because it is the U.S. Environmental Protection Agency (EPA) reference sampler for PM2.5.
All SPESAM samplers were assembled without the denuders. Both the FRM and the SPESAM Samplers used the same 47 mm diameter 2 μm pore-size Teflon filters (R2PJ047, Pall Life Sciences, Ann Arbor, MI) to collect particles. The internal surfaces of all the samplers were cleaned between experiments. In addition, the SPESAM's PUF substrates and FRM's oiled substrates were also changed between each sample. Laboratory and field blanks were routinely used to check for any potential filter contamination during assembly and transport of the samplers.
Filters were weighed before and after sampling in accordance with U.S. EPA guidelines (Citation1997). After equilibration for at least 48 h at 40 ± 5% RH and 21 ± 2°C, filters were weighed on a Mettler-Toledo model MT-5 Microbalance. Before weighing, filters were held between 210Po ionizing units (Staticmaster Model 2U500, NRD Inc., Grand Island, NY) for more than 10 seconds, to reduce electrostatic charges to the Boltzmann equilibrium distribution. Following the off-weight measurements, the Teflon filters were subsequently extracted with 0.15 ml of ethanol (to wet the Teflon membrane) plus 5 ml of 1.5 mM NaOH and analyzed for sulfate using Ion Chromatography (IC) (Dionex DX120, Sunnyvale, CA).
The limit of detection (LOD), precision, and coefficient of variation (CV) were calculated for the SPESAM samplers. LOD was calculated based on three times the standard deviation of the concentrations of the field blanks. Precision was obtained as the RMS difference for the paired values, divided by √2. CV was calculated as the precision divided by the mean concentration.
RESULTS AND DISCUSSION
Laboratory Tests
show the impactor collection efficiency as a function of the aerodynamic diameter for both the 10 and 16.7 lpm sampling flow rates. For the 10 lpm, single-impaction stage configuration, values of d 50 and s g were 3.2 μm and 1.45, respectively. When a second identical stage was added in series, d 50 decreased to 2.6 μm, while s g decreased to a value of 1.35, indicating an improvement in the sharpness of the cutoff curve. Similarly, for the 16.7 lpm, single impaction stage configuration, the d 50 and s g were 2.7 μm and 1.40, respectively. When a second identical stage was added in series, d 50 decreased to 2.4 μm, and sg decreased to a value of 1.29, again indicating a sharper collection efficiency curve. In addition to providing sharper separation characteristics, the use of two impaction stages in series instead of one will also result to an increase on the loading capacity of the system.
FIG. 3 Collection efficiency curves as a function of aerodynamic diameter for SPESAM samplers, (a) 10 LPM sampling flow and (b) 16.7 LPM sampling flow.
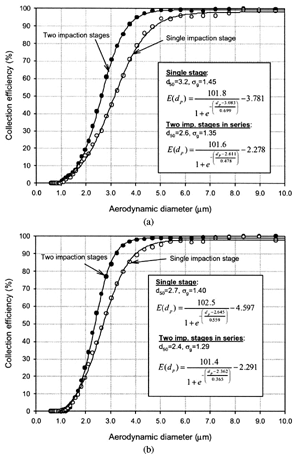
As expected, based on results of previous studies (CitationDemokritou et al. 2002a, b; CitationKavouras et al. 2000; CitationKavouras and Koutrakis 2001; CitationHuang and Tsai 2003; CitationHuang et al. 2001), the use of PUF as an impaction substrate does not follow the traditional rigid, flat surface impaction theory. For the single-stage sampler, √Stk50 corresponding to the cutpoint of the impactor should be 0.7 for a flat rigid surface (CitationHinds 1999). For the PUF substrate, as shown in , the value of √Stk50 is only about 0.4. This result is consistent with previous studies with PUF samplers (CitationDemokritou et al. 2002b). This observed increased collection efficiency is most likely because the penetration of air into the pores of the substrate causes higher inertial forces on particles near the impaction surface than with a rigid, flat plate for the same nozzle acceleration velocity. In addition, this small penetrating flow carries particles into the porous substrate, and those particles will be subjected to filtration. This is expected to result in a less sharp collection efficiency curve compared to that of a flat surface substrate (CitationHuang and Tsai 2003; CitationHuang et al. 2001). However, in this case the use of two impaction stages in series instead of one, as it is mentioned above, improved the sharpness of the collection efficiency curve.
illustrates the particle losses for each flow configuration for the two-stage impaction arrangement as a function of aerodynamic diameter. It is worthwhile to note that particle losses onto the impactor's internal walls and onto the nozzles are incorporated into the collection efficiency curves (). For the particles with an aerodynamic diameter smaller than 2.5 μm, losses were approximately 5%. For coarse particles (PM2.5−10) losses, which are most likely due to turbulence effects, there is an increase with increasing size to a maximum of approximately 22%.
Particle Loading Experiments
shows the collection efficiency curve of the 16.7 lpm configuration as a function of the mass loading (0, 2.8, 15.0, and 35.0 mg). From this figure, it is evident that particle loading does not significantly change the particle collection efficiency curve of the sampler for up to at least 35 mg of loaded mass. A mass loading of 35 mg corresponds to 500 h of sampler operation at an average ambient PM2.5 concentration of 70 μg/m3 (or 48 h at 728 μg/m3). This loading capacity is greater than is necessary for all except the most highly polluted ambient atmospheres.
FIG. 5 Particle loading test for 16.7 lpm SPESAM sampler. (a) Change of the collection efficiency versus loaded mass. (b) Change of 50% cutoff aerodynamic diameter versus loaded mass. (c) Collection efficiency versus loaded mass for da = 1.3 μm and 9.7 μm, respectively.
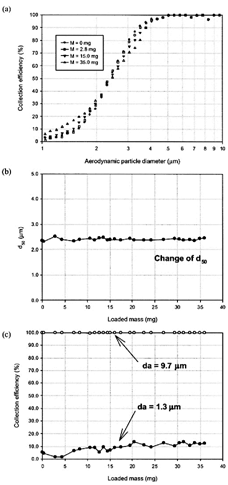
also shows the cutoff diameter (d 50) for the 16.7 lpm configuration, as a function of loaded mass on PUF. The cutpoint is not significantly changed during the particle loading experiment. The d 50 changed at most by only 0.12 μm (<5.0%) for up to 35 mg of loaded mass. It is worth pointing out that for an oiled flat substrate impactor, such as the WINS PM2.5 sampler, d 50 decreased from 2.45 to 2.25 μm for a particle loading of only ∼1.5 mg (CitationKenny et al. 2000). This change is most likely due to the formation of a “hummock-like deposit” during particle loading, which is a distinctive feature for rigid, flat impaction substrates. This type of deposit does not occur when using PUF substrates because the particles are deposited inside the pores of the foam.
presents the collection efficiency for the particle aerodynamic diameters of 1.3 μm and 9.7 μm as a function of particle loaded mass. The 9.7 and 1.3 μm particle sizes represent particles significantly larger and smaller than the cutpoint, respectively (d 50 = 2.5 μm). The collection efficiencies for the 9.7 μm particle remain unchanged and close to 100% for loading up to 35 μg. This is an indication that there is no particle bounce and re-entrainment which is usually more pronounced for large particle sizes. For the 1.3 μm particle size, the collection efficiency was relatively low (approximately 10%) and also remained unchanged with the loading.
It is obvious that the developed impaction system maintained its impaction characteristics at high loading conditions. Thus, particle-bounce for the PUF substrate is minimal for a much higher mass loading than for the commonly used flat, rigid substrates. For example, for the greased coated impaction substrate, particle bounce became significant for a particle mass loading of only about 1 mg, due to a change in the impaction surface characteristics (CitationTsai and Cheng 1995).
Field Study
Mean concentration, precision, the 48 h limits of detection (LODs), and coefficient of variation (CV) are shown in . The LODs for the 10 lpm SPESAM configuration were found to be 0.65 μg/m3 and 0.02 μg/m3 for PM2.5 mass and sulfate, respectively. Similarly, for the 16.7 lpm configuration, LODs were found to be 0.23 μg/m3 and 0.01 μg/m3 for PM2.5 mass and sulfate, respectively. The mean ambient PM2.5 concentration and sulfate concentration in Boston were all much higher than the LOD values.
TABLE 2 Mean concentration, precision, limit of detection, and coefficient of variation for field blank
shows the intercomparison between the SPESAM system and FRM for the PM2.5 mass concentration for both the 10 and 16.7 lpm configurations. Linear regression analysis indicates that for both flow configurations the slope and intercept were not significantly different from unity and zero, respectively. In addition, R2 values are very close to unity. These results demonstrate excellent agreements for PM2.5 mass for the SPESAM and FRM.
FIG. 6 Comparison of PM2.5 mass concentration for the SPESAM system with FRM, (a) 10 LPM configuration and (b) 16.7 LPM configuration.
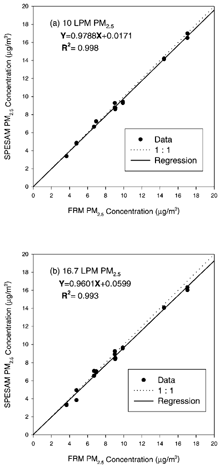
shows the intercomparison between the SPESAM system and FRM, for the sulfate concentration for both the 10 and 16.7 lpm configurations. Analysis of linear regression also indicates that for both flow configurations, the slope and intercept were not significantly different from unity and zero, respectively. In addition, R2 values are very close to unity. These results also suggest an excellent correlation between the two sampling systems.
CONCLUSIONS
In this study, a high loading capacity sampler has been developed which can be used for the chemical characterization of fine particles (PM2.5). The two-stage impaction section of the sampler uses PUF as an impaction substrate. Based on laboratory experiments, the 50% size cutoffs are 2.6 and 2.4 μm (aerodynamic diameter), for the 10 and 16.7 lpm flow configurations, respectively. For mass loading up to at least 35 mg, insignificant changes in size cutoff, and minimum particle bounce and re-entrainment were found. Its high mass loading capacity makes the speciation sampler suitable for high loading applications. The SPESAM sampler was also evaluated in a field study by comparison with the U.S. EPA FRM sampler. The results of the intercomparison field study indicate excellent agreement between the two methods. The new system will be used extensively in our PM physicochemical studies across the US in the near future.
Acknowledgments
The evaluation of the SPESAM sampler was supported by the EPA/HARVARD Center on ambient particle health effects (grant R827353/01/0).
REFERENCE
- de la Mora , F. , Hering , S. U. , Rao , N. and McMurry , P. H. 1990 . Hypersonic Impaction of Ultrafine Particles . J. Aerosol Sci. , 21 : 169 – 187 .
- Demokritou , P. , Kavouras , I. G. , Ferguson , S. T. and Koutrakis , P. 2002a . Development and Experimental Evaluation of a Personal Environmental Monitoring System for Simultaneous Measurements of Particulate Matter and Criteria Gases . Aerosol Sci. Technol. , 35 : 741 – 752 .
- Demokritou , P. , Kavouras , I. G. , Ferguson , S. T. and Koutrakis , P. 2002b . Development of a High Volume Cascade Impactor for Toxicological and Chemical Characterization Studies . Aerosol Sci. Technol. , 36 : 925 – 933 .
- Demokritou , P. , Kavouras , I. G. , Harrison , D. and Koutrakis , P. 2001 . Development and Evaluation of an Impactor for a PM2.5 Speciation Sampler . J. Air Waste Manage. Assoc. , 51 : 514 – 523 .
- Gold , D. R. , Damokosh , A. I. , Pope , C. A. , Dockery , D. W. , McDonnell , W. F. , Serrano , P. , Retama , A. and Castillejos , M. 1999 . Particulate and Ozone Pollutant Effects on the Respiratory Function of Children in Southwest Mexico City . Epidemiol. , 10 : 8 – 16 .
- Hinds , W. C. 1999 . Aerosol Technology , New York : John Wiley & Sons Inc. .
- Huang , C.-H. and Tsai , C.-J. 2003 . Mechanism of Particle Impaction and Filtration by the Dry Porous Metal Substrates of an Inertial Impactor . Aerosol Sci. Technol. , 37 : 486 – 493 .
- Huang , C.-H. , Tsai , C.-J. and Shih , T.-S. 2001 . Particle Collection Efficiency of an Inertial Impactor with Porous Metal Substrates . J. Aerosol Sci. , 32 : 1035 – 1044 .
- John , W. , Fritter , D. N. and Winklmayr , W. 1991 . Resuspension Induced by Impacting Particles . J. Aerosol Sci. , 22 : 723 – 736 .
- Kavouras , I. G. , Ferguson , S. T. , Wolfson , J. M. and Koutrakis , P. 2000 . Development and Validation of a High Volume Low Cut-Off Inertial Impactor (HVLI) . Inhal. Toxicol. , 12 : 35 – 50 .
- Kavouras , I. G. and Koutrakis , P. 2001 . Use of Polyurethane Foam as the Impaction Substrate/Collection Medium in Conventional Inertial Impactors . Aerosol Sci. Technol. , 34 : 46 – 56 .
- Kenny , L. C. , Gussmann , R. and Meyer , M. 2000 . Development of a Sharp-cut Cyclone for Ambient Aerosol Monitoring Applications . Aerosol. Sci. Technol. , 32 : 338 – 358 .
- Klemm , R. J. , Mason , R. M. , Heilig , C. M. , Neas , L. M. and Dockery , D. W. 2000 . Is Daily Mortality Associated Specifically with Fine Particles? Data Reconstruction and Replication of Analyses . J. Air Waste Manag. Assoc. , 50 : 1215 – 1222 .
- Koutrakis , P. , Fasano , A. M. , Slater , J. L. , Spengler , J. D. , McCarthy , J. F. and Leaderer , B. P. 1989 . Design of a Personal Annular Denuder Sampler to Measure Atmospheric Aerosols and Gases . Atmos. Environ. , 23 : 2763 – 2773 .
- Koutrakis , P. , Sioutas , C. , Ferguson , S. and Wolfson , J. M. 1993 . Development and Evaluation of a Glass Honeycomb Denuder/Filter Pack Sampler to Collect Atmospheric Gases and Particles . Environ. Sci. Technol. , 27 : 2497 – 2501 .
- Marple , V. A. 1970 . A Fundamental Study of Inertial Impactors, Ph.D. Thesis , MN : University of Minnesota .
- Marple , V. A. and Liu , B. Y. H. 1974 . Characteristics of Laminar Jet Impactors . Environ. Sci. Technol. , 8 : 648 – 654 .
- Marple , V. A. , Rubow , K. L. and Behm , S. 1991 . A Microorifice Uniform Deposit Impactor (MOUDI): Description, Calibration and Use . Aerosol Sci. Technol. , 14 : 434 – 446 .
- Marple , V. A. , Rubow , K. L. , Turner , W. and Spengler , J. D. 1987 . Low Flow Rate Sharp Cut Impactors for Indoor Air Sampling: Design and Calibration . J. Air Pollut. Control Assoc. , 37 : 1303 – 1307 .
- Murphy , S. A. M. , BeruBe , K. A. and Richards , R. J. 1999 . Bioreactivity of Carbon Black and Diesel Exhaust Particles in Primary Clara and Type II Epithelial Cell Cultures . Occup. Environ. Med. , 56 : 813 – 819 .
- Peters , A. , Verrier , R. L. , Schawrtz , J. , Gold , D. R. , Mittleman , M. , Baliff , J. , Oh , J. A. , Allen , G. , Monahan , K. and Dockery , D. W. 2000 . Air Pollution and Incidence of Cardiac Arrhythmia . Epidemiol. , 11 : 11 – 17 .
- Pope , C. A. , Verrier , R. L. , Lovett , E. G. , Larson , A. C. , Raizenne , M. E. , Kanner , R. E. , Schwartz , J. , Villegas , M. , Gold , D. R. and Dockery , D. W. 1999 . Heart Rate Variability Associated with Particulate Air Pollution . Amer. Heart. J. , 138 : 890 – 899 .
- Sehmel , G. A. 1980 . Particle Resuspension: A Review . Environ. Int. , 4 : 107 – 127 .
- Simpson , R. W. , Williams , G. , Petroeschevsky , A. , Morgan , G. and Rutherford , S. 1997 . Associations Between Outdoor Air Pollution and Daily Mortality in Brisbane . Australia, Arch. Environ. Health. , 52 : 442 – 454 .
- Sioutas , C. , Wang , P. , Ferguson , S. and Koutrakis , P. 1996 . Laboratory and Field Evaluation of an Improved Glass Honeycomb Denuder/Filter Pack Sampler . Atmos. Environ. , 30 : 885 – 895 .
- Soukup , J. M. , Ghio , A. J. and Becker , S. 2000 . Soluble Components of Utah Valley Particulate Pollution Alter Alveolar Macrophage Function in vivo and in vitro . Inhal. Toxicol. , 12 : 401 – 414 .
- Tsai , C.-J. and Cheng , Y.-H. 1995 . Solid Particle Collection Characterization on Impaction Surface of Different Designs . Aerosol Sci. Technol. , 23 : 96 – 106 .
- U.S. EPA . 1997 . National Ambient Air Quality Standards for Particulate Matter: Final Report , New York : Fed. Regist. .
- Wall , S. M. , John , W. , Wang , H. C. and Coren , S. 1990 . Measurement of Kinetic Energy Loss for Particles Impacting Surfaces . Aerosool Sci. Technol. , 12 : 926 – 946 .