We are pleased that P. A. Lawless found our work, CitationLarsen et al. 2003, worthy of examination and we welcome this opportunity to further clarify our results. Lawless' interpretation is concisely summarized in; CitationLawless (2003):
For large particles, the tail of the pulse is relatively long, and if a smaller particle passes through the sensor volume while the tail still elevates the detector voltage, the smaller particle may produce a pulse that exceeds the counting threshold for large particles …
Because small particles in ambient air are much more numerous than large particles, it is the small particle concentration that will govern the appearance rate of correlated pairs.
For data reported in CitationLarsen et al. 2003 and redisplayed in , we can estimate from the top panel that the number density in the smallest size bin is (2 × 104)/(5 s) ≈ 4 × 103 particles per second, corresponding to a mean interarrival time of about 2.5×10−4 s or a quarter of a millisecond. On the other hand, direct observation of (5–10 μm) analog voltage pulses indicates only about a tenth of a microsecond (0.1 μs) “window” during which a voltage pulse between 25–60 mV could trigger a phantom large particle at the flow rate used. Assuming the Poisson process (as conjectured in CitationLawless 2003), the probability of a phantom is the ratio of the two characteristic time scales above. In other words, the probability of a small particle arriving within a fraction of a microsecond after the large particle is on the order of 10−3 or a tenth of a percent.Footnote 1 Because of lower concentrations, the probabilities of false detections in other size bins are below 0.1%. Conservatively then, the cumulative probability of a false detection from all bins is still well below a percent and cannot account for the observed deviations from pure randomness. Thus, the effect of CitationLawless 2003 is negligible in this case (but always useful to keep in mind).Footnote 2
FIG. 1 An empirical demonstration that false “small-turned-large” particle detections are rare. The first panel shows a 25 h time series of particle counts in the 0.3–0.5 μm bin. The ordinate gives number of particles in this size range, detected in a 5 s interval. The middle panel, taken from Figure 2 of CitationLarsen et al. 2003, shows a simultaneous time-series of particle counts in the 5–10 μm size bin. Three shaded regions—A, B, and C—were selected for their relatively constant concentrations of the smallest and most numerous aerosol particles. If small aerosol particles turn into phantom large particles, the two time series should be correlated, with large particle count being highest in region C (due to the higher concentration of small aerosol particles). No such effect is observed. Furthermore, the phantoms should yield a relative abundance of short delay times between large particles. To test this, the bottom panel displays the frequency that, after detecting one 5–10 μm particle, the next 5–10 μm particle is detected “Lags” later. Each lag is many hundreds of pulse decay times so, if phantoms dominate the signal, we would expect that for “Lags = 0” the frequency in region C should be larger than that for regions A or B. Again, this is not observed. We conclude that, therefore, the detected correlations are not due to phantom detections, conjectured in CitationLawless 2003.
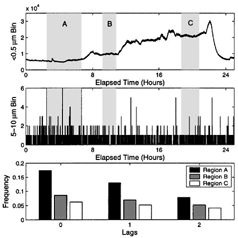
Our conclusion is further confirmed by examining , where we see that the proportionality hypothesized in CitationLawless 2003 between clustering rate and small particle concentration does not occur. Additionally, no correlation between concentration in other size bins and clustering rate is found. Finally, we modified experimental conditions as suggested at the conclusion of CitationLawless 2003 in our more recent work concerned with much finer temporal resolution and lower flow rates (unpublished). Preliminary analysis still indicates deviations from perfect randomness.
We must now bring the reader's attention to an important paper by CitationPreining 1983, which we missed in CitationLarsen et al. 2003 but were directed to by an anonymous reader. Preining's was the first attempt, to the best of our knowledge, to use an optical particle counter to detect deviations from pure randomness in ambient aerosols; he also found deviations from the Poisson process. Furthermore, the experiments reported in CitationPreining 1983 were conducted under a wider range of experimental conditions and with better resolution than those in CitationLarsen et al. 2003. The method of CitationPreining 1983 relied on the notion of a scale-dependent concentration; however, and the pitfalls of this notion are described both by CitationPreining 1983 and emphasized throughout CitationLarsen et al. 2003.
Near the end of his paper, CitationPreining 1983 writes:
Conditional distributions such as…the distributions of time intervals that occurred just after a particle of a certain size had arrived or a time interval of a certain duration had elapsed would give entirely new and deep insights into the structure of particulate clouds and thus provide a much needed quantitative tool in aerosol microphysics.
While Lawless finds turbulence-induced clustering counterintuitive, Preining attributes the deviations from Poisson behavior to poor mixing of aerosols. As is (perceptively) noted in CitationLawless 2003, we were hesitant to attribute the spatial correlations to an “ultimate cause” as there was no consensus even among the authors regarding the most likely physical origin. We agree with Lawless in the general attitude that instrumental effects cannot yet be ruled out entirely. The mere action of the counter pulling in air can disturb the “true” aerosol distribution by creating eddies in the tube, etc. Turbulence is a quick but not thorough mixer and it leaves clumps of particles behind.Footnote 3
Nevertheless, based on the so-called “mapping theorem” (pp. 17–21 of CitationKingman 1983), we suspect that the spatial correlations are truly there, not induced by the counter.Footnote 4
Acknowledgments
We would like to thank Glenn Shaw at the University of Alaska, Fairbanks for the continued loan of the optical particle counter used. This work was supported in part by the NSF grant ATM-0106271, the Michigan Space Grant Consortium the Goddard Earth Science Technology Center's Graduate Student Summer Program in Earth System Science. We would further like to thank Dr. Phil Lawless for writing the comment as well as an anonymous reader for directing us to CitationPreining 1983
Notes
1The entire sensor volume (∼1 mm) traversal time is on the order of 10 microseconds at our flow rates.
2For example, a second particle, while not causing a false detection, may nevertheless artificially lengthen the first particle's recorded residence time. This did not affect our analysis.
3 There is considerable literature on the intermittent nature of turbulent mixing, e.g., CitationSquires and Eaton 1991
4 This theorem is about invariance of a Poisson process under “reasonable” mappings such as fluid streamline narrowing while entering a tube.
REFERENCES
- Kingman , J. 1983 . Poisson Processes , Oxford : Clarendon Press .
- Larsen , M. , Cantrell , W. , Kannosto , J. and Kostinski , A. 2003 . Detection of Spatial Correlations Among Aerosol Particles . Aerosol Sci. Technol , 37 : 476 – 485 .
- Lawless , P. 2003 . Comment on Detection of Spatial Correlations Among Aerosol Particles . Aerosol Sci. Technol , 38 : 128
- Preining , O. 1983 . Optical Single-Particle Counters to Obtain the Spatial Inhomogeneity of Particulate Clouds . Aerosol Sci. Technol , 2 : 79 – 90 .
- Squires , K. and Eaton , J. 1991 . Preferential Concentration of Particles by Turbulence . Physics Fluids A , 3 : 1169 – 1178 .