Abstract
The concentration and composition of PM 2.5 from May to September of 2000 and monthly trends in ambient fine-particulate material concentrations from October 1999 through December 2000 at the National Energy Technology Laboratory's airmonitoring site in Pittsburgh are reported. Twenty four-hour integrated samples were collected using the Particle Concentrator-Brigham Young University Organic Sampling System (PC-BOSS), a multichannel integrated diffusion denuder sampler designed for routine determination of the chemical composition of ambient particulate matter. The fine-particulate pollutants determined were sulfate estimated as ammonium sulfate, nonvolatile organic material, semivolatile organic material lost from particles during sampling, elemental carbon, nitrate estimated as ammonium nitrate, including ammonium nitrate lost from particles during sampling and elemental content determined by PIXE (for a limited number of samples). Episodes with elevated sulfate and organic material (both semivolatile and nonvolatile) concentrations were seen throughout this period. For the purpose of this discussion, an episode was defined as all times when 3 h average TEOM monitor PM 2.5 concentrations exceeded 30 μg/m3. The use of estimated back-trajectories indicated that during the periods for which these elevated concentrations were observed, pollutants were transported predominantly from the Southwest from the Ohio River Valley to the sampling site. For days when fine particulate episodes occurred, back-trajectory computations were derived for time intervals for which PM 2.5 TEOM concentrations exceeded 30 μg/m3. However, for nonepisode days, back trajectories were computed over a 24 h period. Average PC-BOSS–constructed PM 2.5 concentration (including semivolatile components lost from particles during sampling) for the period from October 1999 through December 2000 was 19 μg/m 3 , excluding crustal material concentration.
INTRODUCTION
Air pollution has become one of the serious environmental concerns in urban areas, especially in view of the adverse health effects that have been associated with ambient fine particles (CitationDockery and Pope 1994; CitationVedal 1997; CitationPope 2000; CitationSchwartz 2001). On July 17, 1997, the United States Environmental Protection Agency revised the National Ambient Air Quality Standards to address ambient air concentrations of particulate matter with aerodynamic diameter of 2.5 μm or less (PM2.5). The proposed new standard establishes a 24 h average concentration limit of 65 μg/m3 and an annual mean concentration limit of 15 μg/m3 to protect human health.
The Office of Science and Technology of the National Energy Technology Laboratory (NETL) has participated in the larger United States Department of Energy Fossil Energy PM2.5 project by establishing, equipping, and operating a PM2.5 sampling and analysis station at the Pittsburgh NETL site. The driving force behind the monitoring effort was the need for a better understanding of the relationships between ambient concentrations and composition of PM2.5 measured at a stationary outdoor monitoring site and the meteorological conditions that contribute to those concentrations. Development of a strategy for mitigating air pollution must include the acquisition of air-quality data, monitored systematically to capture seasonal fluctuations. The need for systematic monitoring of air quality in Pittsburgh was another motivating criteria for support of this project by the Office of Science and Technology of the National Energy Technology Laboratory (NETL) of the US Department of Energy in Pittsburgh, Pennsylvania.
In this article, 24 h ambient fine particulate concentrations and composition are presented. Fine particulate concentrations were correlated with meteorological data that included 24 h back-trajectory computations for all sampling periods to characterize the meteorological relationships in a quantitative manner.
EXPERIMENTAL
The Sampling Site
The air monitoring site is located on an open hill at the NETL Pittsburgh facility at 40.31 North in latitude and 79.98 West in longitude. The specific location of the sampling station was chosen to take advantage of an existing meteorological tower which supplies full weather data for the program. The NETL site is not near any major road or freeway, so high concentrations of fresh automotive emissions are not expected. There are also no major point sources close to the site. Two small experimental combustors, located at the NETL facility just 200 m east of the site occasionally burn a variety of fuels (227 kg/h of coal and about 18 kg/h of various other fuels). About 600 m ESE is a coal-fired steam plant which burns up to 860 kg/h of fuel to provide heating for the NETL facility. Both these sources are downwind of the NETL site the great majority of the time. While they make infrequent contribution to nitrogen and sulfur oxides and CO concentrations at the NETL site, they are not expected to be a significant contributor to PM2.5 at the site (CitationAnderson et al. 2003). Major coal-fired power plants, iron and coke processing facilities, and chemical manufacturing plants are located along the Ohio River Valley to the west and southwest of the NETL site. Some coke processing facilities, an integrated steel mill, and two well-controlled power plants are also located along the Monongahela River to the east and southeast of the facility. These are all potential sources of transported pollutants to the site. The prevailing wind directions at the site are generally from the southwest to northwest directions. Thus, impacts from emissions from the Ohio River Valley and urban centers to the southwest, west, and northwest are expected.
The PC-BOSS Sampler
shows the schematic of the Particle Concentrator-Brigham Young University Organic Sampling System (PCBOSS) used in the Pittsburgh NETL study. The inlet to the sampler was a Bendix cyclone (CitationChan and Lippmann 1977) with a particle cut of 2.3–2.5 μm aerodynamic diameter at an inlet flow of 150 L/min. Following the inlet was a mixing chamber to ensure complete mixing of the inlet aerosol prior to diverting 16 L/min of the total flow to the side flow manifold. The side flow manifold held a single-filter pack containing a 47 mm diameter Whatman Nuclepore (0.4 μm pore, Whatman Inc., Clifton, NJ, USA) and a 47 mm diameter charcoal-impregnated glass fiber filter (CIG, Schleicher and Schuell, Dassel, Germany). The side flow allowed for the direct determination of the particle concentrator efficiency and losses (CitationLewtas et al. 2001; CitationModey et al. 2001). The remainder of the sampled air stream entered a virtual-impactor particle concentrator. The design and evaluation of the concentrator have been previously described (CitationSioutas et al. 1994; CitationDing et al. 2002). The particle concentrator separates 75% of the gas phase material into the major flow and leaves particles larger than the cut point (about 0.1 μm) along with a significantly reduced fraction of the gas phase material in the minor flow. Thus, the particle concentrator gives rise to a concentrated stream of 0.1 to ∼2.5 μm particles in the minor flow of the PC-BOSS.
The minor flow air containing concentrated particles entered the BOSS diffusion denuder (CitationEatough et al. 1993). The denuder was comprised of parallel strips of charcoal-impregnated cellulose fiber filter (CIF, Schleicher and Schuell, Keene, NH, USA), which were separated at the long edges by 2 cm diameter glass rods. With a significant fraction of gas-phase species such as HNO3, SO2, O3, and volatile organic compounds (VOC) in the sampled air removed in the particle concentrator prior to passage of the aerosol through the denuder, the efficiency of the concentrator plus denuder for removal of SO2, and gas phase HNO3 and organic compounds is expected to exceed 99% (CitationEatough et al. 1999; CitationDing et al. 2002; CitationLewtas et al. 2001). As a result, positive sampling artifacts from the collection of gas-phase compounds by quartz filters after the denuder are negligible.
The denuder was followed by two parallel filter packs, each drawing approximately 16 L min−1 of air. One of the parallel filter packs contained a 47 mm quartz filter (Gelman Sciences, Ann Arbor, MI, USA) followed by a 47 mm charcoal-impregnated glass (CIG) fiber filter (Schleicher and Schuell, Dassel, Germany). This combination of filters was used to determine fine particulate carbonaceous material, sulfate, and nonvolatile nitrate on the quartz filter, as well as semivolatile organic material (SVOM) lost from the particles on the quartz filter and trapped on the sorbent CIG. The other parallel filter pack contained a 47 mm diameter ringed support Teflon filter (Whatman Inc., Clifton, NJ, USA) followed by a Nylasorb filter (Gelman Sciences, Ann Arbor, MI, USA). The Teflon filter was used to determine sulfate and nonvolatile nitrate, whereas the Nylasorb filter was used for the determination of nitrate lost from particles during sampling. With this combination of techniques, negative sampling artifacts are avoided. All quartz filters were baked at 800°C for 10 h before use. The CIG sorbent filters used in this study were baked in an oven at 340°C for 24 h in an atmosphere of nitrogen. These treatments were to ensure that the filters contained no residual organic compounds prior to being used for sampling. The Nuclepore, Teflon, and Nylasorb filters were used as received from the manufacturer without any pretreatment.
Supplemental Data
Besides the PC-BOSS sampler from Brigham Young University, the monitoring site also had a tapered element oscillating microbalance (TEOM) monitor for continuous PM2.5 mass monitoring and a sequential Federal Reference Method (FRM) Sampler (Rupprecht and Patashnick, Albany, NY, USA) for 24 h PM2.5 mass. Data from each of these instruments were provided by NETL for comparison with those obtained from the PC-BOSS.
Sampling Protocol
Daily samples have been collected with one PC-BOSS sampler for over a year at the National Energy Technology Laboratory PM2.5 characterization site in Pittsburgh. The initial six months of data (from November 1999 through April 2000) for this program have been previously reported (CitationModey and Eatough 2003). This article gives the data from May 2000 through December 2000, thus spanning the summer and fall time periods, and interprets the data for the entire sampling period. Samples for the determination of fine particulate composition and concentration were collected from 12:00 noon EDT to 12:00 noon EDT the next day with the PC-BOSS. Periodically this same PC-BOSS was also used for measurement of the denuder efficiency for collection of gas-phase organic species.
Sample Analysis
Analysis for Nitrate and Sulfate
Sulfate and nitrate analysis was by ion chromatography. All ion chromatographic analyses were performed using a Dionex LC20 chromatograph equipped with a CD20 conductivity detector. The eluent was 3.5 mM Na2CO3/1.0 mM NaHCO3 solution at a flow rate of 1.2 mL/min, and the suppressor was an anion self-regenerating suppressor. One 2 cm2 punch of the quartz filters was extracted by ultrasonication with deionized water in a monovette (Sarstedt, Newton, NC, USA). The extracted solution was analyzed for sulfate and nitrate. Whole Teflon filters and half of each Nuclepore filter were also extracted by ultrasonication in deionized water in a monovette and analyzed for sulfate and nitrate. Whole Nylasorb filters were extracted ultrasonically in the ion chromatography eluent solution and analyzed for nitrate. For multiple analysis of a single sample, precision for sulfate and nitrate determinations was within ± 5%
Analysis for Collected Carbonaceous Material
A 2 cm2 portion of the quartz and CIG filters were analyzed for carbonaceous material by thermal desorption of the collected materials using temperature-programmed volatilization (CitationEatough et al. 1993; CitationEllis and Novakov 1982). The carbonaceous materials were converted to CO2, which was then determined on-line using a nondispersive infrared (NDIR) detector on an ULTRAMAT 3-gas analyzer (Siemens Inc., Roswell, GA, USA). The NDIR detector was calibrated daily using three certified CO2 standards (Airgas, Los Angeles, CA, USA) which spanned the analysis concentration range. Organic compounds evolved from the particles and collected by the CIG filter will be referred to in this article as particulate semivolatile. The CIG filters were heated from 50°C to 340°C at a ramp rate of 15°C/min in a stream of nitrogen. The maximum temperature for the CIG analysis was imposed by the fact that degradation of the filter charcoal began 10–20°C below the maximum analysis temperature. Collected semivolatile organic compounds lost from the particles were evolved from the CIG between 200°C to 300°C. The quartz filters were heated from 50°C to 800°C at a ramp rate of 28°C/min in a stream of N2/O2 (70:30% v/v). Soot or elemental carbon was estimated from the high temperature (usually above 460°C) peak (CitationEllis and Novakov 1982) on the thermogram obtained from the quartz filter analysis. Because of the presence of high concentrations of organic material that evolve at a temperature just below that for soot, the uncertainty in this estimate is probably about 30–50%. The shape of the thermograms indicated that all collected organic compounds and soot were evolved from the collection matrix at the maximum temperatures used. No evidence for the high-temperature evolution of CO2 from inorganic carbonates was seen. Blank determinations were made for both quartz and CIG filters. The variability in blank filters was ±3 μg C/filter, corresponding to an uncertainty in the blank correction of ±0.4 μg C/m3.
Analysis for Crustal Material
Selected Nuclepore filter samples were analyzed for elemental content by proton induced X-ray emission (PIXE) analysis. Crustal material was estimated from the aluminum and silicon concentrations using average crustal abundances of these elements (CitationWeast 2001).
RESULTS AND DISCUSSION
PM 2.5 Composition
Fine particulate material is composed of a wide range of species, but in this article particulate composition is assumed to be carbonaceous material both elemental and organic, sulfate as ammonium sulfate, and nitrate as ammonium nitrate. Levels of NH4 + in ambient fine particulate material collected at the NETL site correlated with the equivalent sum of concentrations of SO4 2− and NO3 −, with linear regression slopes of ∼1.0 (CitationMartello et al. 2001). This suggested that most of the acid nitrate and sulfate aerosols are neutralized by ammonium ions in the fine-particulate fraction. Therefore, on the basis of these findings, nitrate and sulfate associated with the fine-particulate fraction at the NETL site were estimated as their ammonium salts. The organic material, both nonvolatile and semivolatile, was assumed to be 61% carbon (CitationTurpin and Lim 2001).
Constructed PM2.5 was calculated as the sum of concentrations of organic material determined on the quartz and sorbent charcoal-impregnated filter underneath the quartz, average estimated ammonium sulfate on the quartz and Teflon filters, average estimated ammonium nitrate on the quartz and Teflon filters, elemental carbon or soot determined on the quartz filter, and estimated ammonium nitrate determined on the Nylasorb filter, thus, incorporating negative artifacts in the PM2.5 calculation. All these concentrations were corrected for particle concentrator efficiency.
Elemental data from which crustal material was estimated was only available for a fraction of the data. However, these data suggested an average crustal material concentration of 0.5 μg m−3 based on the Al and Si content determined on the filters. In our previous report (Modey and Eatough 2002) for data obtained at this site from November 1999 to April 2000, we reported a semiannual average of 19.1 μg m−3 for constructed PM2.5 without crustal material. Results reported here are therefore a continuation of our one-year everyday involvement in the program. From 11 May 2000 to 10 December 2000, average constructed PM2.5, including semivolatile material lost from particles and the estimated crustal material, was 18.5 μg m−3. The average percentage composition, including a 0.5 μg m−3 average crustal material, was as follows: sulfate, estimated as ammonium sulfate, 40%; nonvolatile organic material, 34%; semivolatile organic material lost from particles during sampling, 17%; soot, 3%; retained nitrate as ammonium nitrate, 4%; ammonium nitrate lost from particles during sampling, 2%; and crustal material, 3%.
gives the monthly average maximum and minimum concentrations obtained for each daily measured PM2.5 component for May through December 2000. The average PM2.5 component concentrations for November 1999 through December 2000 are given in . Ammonium sulfate concentrations were elevated during May through October and highest in August. Nonvolatile organic material concentration was moderately high throughout the study period, but semivolatile organic material lost from particles was highest during January through April. Clearer pictures of the variation in each of these components from May through December 2000 are seen in , where ammonium sulfate, nonvolatile organic material, and lost semivolatile organic material are seen to be consistently higher throughout the month of August. also shows a comparison of the PC-BOSS–constructed mass, FRM mass, and TEOM monitor mass for the months of May–September 2000. The FRM and TEOM monitor data are not yet available for the November through December data. Details on the daily variations for the first six months of the study have been previously published (CitationModey and Eatough 2003).
FIG. 2 Monthly average 24 h daily PM2.5 concentrations in μg/m3 from November 1999 through December 2000.
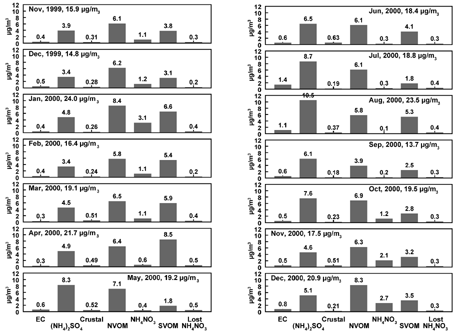
FIG. 3 Comparison of 24 h PC-BOSS (PM2.5), TEOM monitor, and FRM PM measurements and PC-BOSS (NH4)2SO4, NVOM, and SVOM concentrations from May through December 2000.
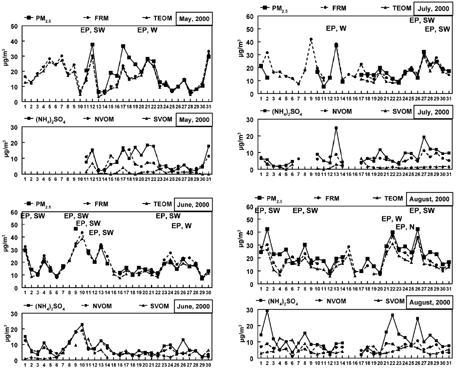
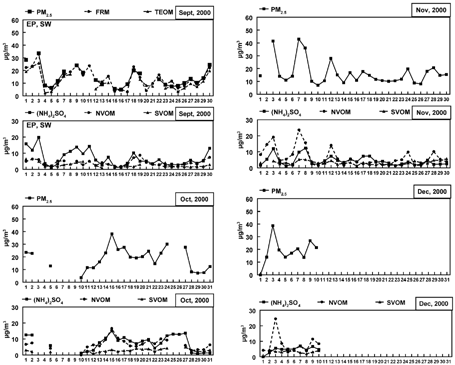
TABLE 1 Summary of concentrations of fine particulate composition from May 2000 through December 2000. Concentrations are in μg m−3. Number of days in each month for which samples were available for analysis are shown in parentheses
shows a comparison of data from May through September 2000 obtained from the PC-BOSS, the TEOM monitor, and the sequential FRM sampler. The PC-BOSS nonvolatile mass is calculated based on concentrations of PM2.5 components determined on the Quartz (Q1, ) and Teflon (T1, ) filters. PM2.5 concentrations obtained from the TEOM monitor are uniformly lower than concentrations determined using either the PC-BOSS or the FRM. The difference is due to the contribution of semivolatile components not retained by the heated filter (50°C) of the TEOM monitor but determined with the PC-BOSS based on analysis of filters C1 and N1 shown in . For 12% of the samples, the FRM concentrations are slightly higher than the PC-BOSS–constructed PM2.5; about 10% are equal to PC-BOSS–constructed PM2.5, and the majority of the FRM concentrations are much lower than the constructed PM2.5 concentrations determined with the PC-BOSS.
At least two factors are expected to influence these observed trends. First, the few cases where the FRM PM2.5 is higher than the PC-BOSS–constructed PM2.5 could be due to higher concentrations of crustal material in the fine particulate fraction than estimated from the PC-BOSS data, or alternatively, could be attributed to whether or not elimination of water from particulate-material trapped on the filters used in the FRM sampler was complete prior to postweighing of the FRM filters. Even though the EPA protocols are intended to remove water prior to postweighing the filters, not all particle-bound water may be removed (CitationLong et al. 2003). Water is not included in the PC-BOSS–constructed PM2.5 calculations. Secondly, loss of semivolatile organic material from the FRM filter is also likely to lead to the observed trends in which the FRM concentrations are lower than constructed PC-BOSS PM2.5 concentrations.
gives the linear regression analysis summary for fine-particulate concentrations determined using the PC-BOSS, FRM, and TEOM where all three concentrations are available. These data include an average 0.5 μg/m3 crustal material concentration for the PC-BOSS data. A two-tailed two-sample t-test on data from the PC-BOSS, TEOM monitor, and FRM at the 5% rejection region is also given in . The results show expected agreements and disagreements in the paired data sets: the FRM underestimates PM2.5 (p-value 0.019, zero intercept slope = 0.84); the TEOM monitor also underestimates PC-BOSS–constructed PM2.3 (p-value 0.0000055, zero intercept slope = 0.72); the FRM give higher concentrations than the TEOM monitor results (p-value 0.015, zero intercept slope = 0.85); the PC-BOSS nonvolatile mass is a better estimate of FRM PM2.5 (p-value 0.364, zero intercept slope = 1.01) than PC-BOSS–constructed PM2.5.
TABLE 2 Intersampler statistical summary of PM2.5 concentrations for the period May 2000 through September 2000. Linear regression R2, slopes, and intercepts are given for both calculatedFootnote a and a zero interceptFootnote b
Annual Trends in the Data
shows monthly trends in fine-particulate composition from November 1999 to December 2000. Nonvolatile organic material (NVOM) dominated the fine-particle composition during the winter and spring months (November through April), but in summer and fall (May through October) the fine-particle composition was dominated by ammonium sulfate. Semivolatile organic material (SVOM) concentrations were higher from January through April and also in August. Ammonium nitrate concentrations were very low throughout the year but were highest during January. The average annual constructed 2000 PM2.5 concentration was 19 μg/m3 with the addition of an average of 0.5 μg/m3 crustal material. The annual average standard for PM2.5 is currently 15 μg/m3. The annual average nonvolatile PM2.5 concentration was 14 μg/m3. Semivolatile ammonium nitrate and SVOM account for the remaining 4 μg/m3 above the current annual standard. Therefore, SVOM is an important fine-particulate component that deserves consideration in any future amendment to the current PM2.5 standard. Elemental data from which crustal material concentration could be calculated was only available for a small fraction of the data. However, as noted above, this fraction of the data suggested an average crustal material concentration of 0.5 μg/m3 based on the Al and Si content determined on the Nuclepore filters.
These results are all consistent with the concentrations of semivolatile material measured on the C1 and N1 filters of the PC-BOSS (). For the selected data, semivolatile material measured with the PC-BOSS averaged 16% of the PC-BOSS–constructed PM2.5, corresponding to the 0.84 slope for comparison of the PC-BOSS–constructed PM2.5 and FRM results. Likewise, the PC-BOSS nonvolatile and FRM results are in agreement. The TEOM monitor mass was lower than either the PC-BOSS or FRM results due to increased loss of semivolatile material from the heated filter of the TEOM monitor. However, contrary to these observations, statistical analysis of PC-BOSS and FRM data sets without the inclusion of the estimated crustal concentration indicated that a comparison between the FRM and PC-BOSS–constructed PM2.5 was not statistically significant (p-value 0.157). Thus, the two data sets were in agreement. Such an agreement could only be justified as resulting from the presence of quantities of semivolatile material, including water possibly retained on the filters for postweighing but not included in the constructed PC-BOSS calculations. The PC-BOSS semivolatile measurements do not agree with this justification. These arguments therefore justify the inclusion of estimated crustal material for the attainment of closure.
Meteorological Influence on Fine Particulate Concentration and Composition
Factors such as direction of movement of air mass are expected to influence the concentrations of fine-particulate matter impacting the site. Estimated back-trajectories (Hysplit4 Model 1997) have been used in previous studies to characterize the transport of pollutants to the NETL site (CitationAnderson et al. 2002). Similar computations were employed in this study as well to determine the transport route of pollutants to the site. During the period from May through August, the concentrations of PC-BOSS–constructed PM2.5 experienced occasional 1–3 day episodes ().
There were short-term high concentrations identified within a 24 h sampling period with well-defined meteorological transport patterns to the site. Back-trajectory computations during these episode days were derived from the time intervals during which 3 h PM2.5 TEOM concentrations exceeded 30 μg/m3. However, for the nonepisode days, back-trajectories were computed over a 24 h period. The episodes identified by this criteria are given in with the identified transport direction. The episodes are also identified in . They were all associated with transitions from locally high pressure to low pressure regimes. The concentrations of fine-particulate TEOM monitor mass during the high-pressure periods were generally low. This indicated that the pollution associated with the PM2.5 episodes did not originate from the local region around the NETL site, but that pollutants were transported into the area during the transition from high to a low pressure regime with the approach of a frontal system. For example, for an episode that occurred on 12 May, the high-pressure region prior to the episode was centered over Western Virginia and Pennsylvania, and the episode occurred with the approach of a warm frontal passage from the north. Transport at the start of the episode was from the Ohio River Valley from the southwest of the NETL site ( and ).
TABLE 3 Relationship between direction of transport of pollutants to the NETL site and fine-particulate concentrations (μg/m3) from May 2000 through September 2000
Similar patterns in pressure and fine-particulate concentration peaks with persistent southwest transport of pollutants were seen during May through September, as indicated in and . During these transport episodes, fine-particulate material was dominated by ammonium sulfate (), indicating that PM2.5 included significant contribution from photochemical processes during long-range transport. The average concentration of ammonium sulfate associated with these southwest transport conditions was 17 μg/m3 with much lower concentrations before and after the episodes. In addition, concentrations of sulfate were lower with transport from the southwest in the absence of an episode (). The concentrations of nonvolatile organic material (but not semivolatile organic material) were also elevated during the episodes.
High concentrations of ammonium sulfate with moderately high concentrations of nonvolatile organic material were present when pollutants were transported from the west. Only sulfate was significantly elevated during the westerly transport episode periods (). Transport from the west will include emissions from the coal-fired power plants and the various coke-processing and steel-manufacturing plants located along the Ohio River Valley to the west of the NETL sampling site. 23–25 July, 27–30 August, and 16 September were time periods when pollutants were transported to the NETL site from the east () and may possibly include impacts from the coke-processing facilities and the steel mill along the Monongahela River to the east of the NETL site. When pollutants were transported from the east no episodes were observed. However, average concentrations were comparable to those observed during transport from the west. Transport from the northwest was also frequent, as given in , but with PC-BOSS–constructed PM2.5 being lower, averaging 13.5 μg/m3. There were also a few days when pollutants were transported from the north (with an associated episode occurring on 24 August), the northeast, the south, and the southeast, as indicated in , with generally lower concentrations during these transport days.
CONCLUSIONS
The concentration and composition of ambient fineparticulate material impacting the NETL site in Pittsburgh were dependent on meteorological and seasonal conditions. During high pressure conditions, or transport from all directions other than the east, west, or southwest, concentrations of fineparticulate material averaged lower than 15 μg/m3 and the composition was dominated by carbonaceous material. Under these meteorological conditions, particulate matter impacting the site probably originated from local mobile and stationary combustion sources. Highest average concentrations of fine-particulate matter were always associated with transitions from high to low pressure with transport of pollutants to the NETL site with approach of a frontal system from the west or southwest, thus suggesting transport of emissions built up from larger and distant sources during the high-pressure period. These transport conditions occasionally led to episodes with average PM2.5 concentrations exceeding 30 μg/m3. The fine particles during these episodes contained elevated concentrations of ammonium sulfate. Back-trajectory analysis indicated that pollutants during these high-episode days were most frequently transported from the Ohio River Valley to the southwest of the NETL sampling site.
Acknowledgments
The participation of Brigham Young University personnel was funded under a University Coal Research Grant # DE-FG26-99FT40581 from the United States Department of Energy, National Energy Technology Laboratory to Brigham Young University. We express sincere thanks to the Office of Science and Technology of the National Energy Technology Laboratory of the United States Department of Energy for continuing financial support for this project. We also express our appreciation to NETL staff Richard R. Anderson, Donald V. Martello, Paul C. Rohar, and Karl Waldner for their technical and field support and for use of data from the collocated TEOM and FRM monitors. Sincere thanks is also expressed to the Air Resources Laboratory of the National Oceanic Atmospheric Administration (NOAA) for permission to access the HYSPLIT transport and dispersion model on the NOAA Air Resources Laboratory's READY web server.
Reference to this report to any specific commercial product, process, or service is to facilitate understanding and does not necessarily imply its endorsement or favoring by the United States Department of Energy.
Notes
a For calculated intercept.
b For zero intercept.
REFERENCES
- Anderson , R. R. , Irdi , G. , Lynn , R. , Martello , D. V. , Rohar , P. C. , Tamilia , J. P. , Veloski , G. A. , Waldner , K. , White , C. M. and Eatough , D. J. 2003 . The DOE NETL OST PM2.5 Characterization Program . Environ. Sci. Technol. , Submitted
- Anderson , R. R. , Martello , D. V. , Rohar , P. C. , Strazisar , B. R. , Tamilia , J. P. , Waldner , K. , White , C. M. , Modey , W. K. , Mangelson , N. F. and Eatough , D. J. 2002 . Sources and Composition of PM2.5 at the National Energy Technology Laboratory in Pittsburgh During July and August 2000 . Energy Fuels , 16 : 261 – 269 .
- Chan , T. and Lippmann , M. 1977 . Particle Collecting Efficiencies of Air Sampling Cyclones: An Empirical Theory . Environ. Sci. Technol. , 11 : 377 – 382 .
- Ding , Y. , Pang , Y. and Eatough , D. J. 2002 . A High Volume Diffusion Denuder Sampler for the Routine Monitoring of Fine Particulate Matter: I. Design and Optimization of the PC-BOSS . Aerosol Sci. Technol. , 36 : 383 – 396 .
- Dockery , D. W. and Pope , C. A. III . 1994 . Acute Respiratory Effects of Particulate Air Pollution . Ann. Rev. Public Health. , 15 : 107 – 132 .
- Eatough , D. J. , Wadsworth , A. , Eatough , D. A. , Crawford , J. W. , Hansen , L. D. and Lewis , E. A. 1993 . A Multi-System, Multi-Channel Diffusion Denuder Sampler for the Determination of Fine Particulate Organic Material in the Atmosphere . Atmos. Environ. , 27A : 1213 – 1219 .
- Eatough , D. J. , Pang , Y. and Eatough , N. L. 1999 . Determination of PM2.5 sulfate and nitrate with PC-BOSS designed for routine sampling for semivolatile particulate matter . J. Air Waste Management Assoc. , 49 : 69 – 75 .
- Ellis , E. C. and Novakov , T. 1982 . Application of Thermal Analysis to the Characterization of Organic Aerosol Particles . Sci. Total Environ. , 23 : 227 – 238 .
- 1997 . Hysplit4 (Hybrid Single-Particle Lagrangian Integrated Trajectory) Model , Silver Spring MD, , USA : NOAA Air Resources Laboratory . Located at
- Lewtas , J. , Booth , D. , Pang , Y. , Reimer , S. , Eatough , D. J. and Gundel , L. 2001 . Comparison of Sampling Methods for Semi-Volatile Organic Carbon (SVOC) Associated with PM2.5 . Aerosol Sci. Technol. , 34 : 9 – 22 .
- Long , R. W. , Eatough , N. L. , Mangelson , W. T. , Fiet , K. , Smith , S. , Smith , R. , Eatough , D. J. , Pope , C. A. and Wilson , W. E. 2003 . The Measurement of PM2.5, Including Semi-Volatile Components, in the EMPACT Program: Results from the Salt Lake City Study . Atmos. Environ. , 37 : 4407 – 4417 .
- Martello , D. V. , Anderson , R. R. , White , C. M. , Casuccio , G. S. and Schlaegle , S. F. 2001 . “ Quantitative Scanning Electron Microscopy Methods to Characterize Ambient Air PM2.5 ” . In American Chemical Society 222nd National Meeting, Symposium on Fine Particulate (PM 2.5 ) Formation and Emission from Fuel Combustion , 606 – 608 . Chicago, IL : ACS Fuel Chemistry Division . Preprints
- Modey , W. K. , Pang , Y. , Eatough , N. L. and Eatough , D. J. 2001 . Fine Particulate (PM2.5) Composition in Atlanta, USA: Assessment of the Particle Concentrator-Brigham Young University Organic Sampling System, PC-BOSS, During the EPA Supersite Study . Atmos. Environ. , 35 : 6493 – 6502 .
- Modey , W. K. and Eatough , D. J. 2003 . Trends in PM2.3 Composition at the Department of Energy OST NETL PM2.5 Characterization Site in Pittsburgh, Pennsylvania, USA . Adv. Environ. Res. , 7 : 859 – 869 .
- Pope , C. A. III . 2000 . Epidemiology of Fine Particulate Air Pollution and Human Health: Biologic Mechanisms and Who's at Risk . Environ. Health Persp. , 108 ( suppl. 4 ) : 713 – 723 .
- Schwartz , J. 2001 . Is there Harvesting in the Association of Airborne Particles with Daily Deaths and Hospital Admissions? . Epidemiology. , 12 : 55 – 61 .
- Sioutas , C. , Koutrakis , P. and Olson , B. A. 1994 . Development and Evaluation of a Low Cut-Point Virtual Impactor . Aerosol Sci. Technol. , 21 : 223 – 235 .
- Tang , H. , Lewis , E. A. , Eatough , D. J. , Burton , R. M. and Farber , R. J. 1994 . Determination of the Particle Size Distribution and Chemical Composition of Semi-Volatile Organic Compounds in Atmospheric Fine Particles . Atmos. Environ. , 28 : 939 – 947 .
- Turpin , B. J. and Lim , H.-J. 2001 . Species Contributions to PM2.5 Mass Concentrations: Revisiting Common Assumptions for Estimating Organic Mass . Aerosol Sci. Technol. , 35 : 602 – 610 .
- Vedal , S. 1997 . Ambient Particles and Health: Lines that Divide . J. Air Waste Management Assoc. , 47 : 551 – 581 .
- Weast , R. C. , ed. 2001 . CRC Handbook of Chemistry & Physics , 102nd ed. , Boca Raton, FL : CRC Press .