Abstract
The enhanced uptake of sulfuric acid molecules by charged clusters as a result of dipole–charge interaction is critical to the ion-mediated nucleation of particles in the atmosphere. This article illustrates the effect of the structure of the gas-phase sulfuric acid and its hydrates on the uptake of the sulfuric acid molecules by the charged clusters/ultrafine particles. It is shown that the different structures of gas-phase sulfuric acid and its hydrates lead to very big (up to ∼ 230%) variations in the uptake coefficients due to the large difference in the dipole moments. On average, the hydration of gaseous sulfuric acid in the atmosphere is likely to enhance the uptake coefficients.
INTRODUCTION
It is important to understand the formation and properties of fine particles in the atmosphere due to their climate effect and health impact. Gaseous sulfuric acid, which is one of the key substances for atmospheric aerosol formation, tends to form hydrates—small clusters consisting of a few water molecules associated with the sulfuric acid molecule. The hydration effect has been studied in the past in the frame of classical binary homogeneous nucleation (BHN) theory (e.g., Jacker-Voirol and Mirabel 1989; CitationNoppel 1998); however, recent studies (e.g., CitationWeber et al. 1996; CitationClarke et al. 1998) indicated significant discrepancies between the observations of the fast nucleation events in the boundary layer and model predictions of the classical BHN theory. CitationYu and Turco (2000) suggested that, in addition to sulfuric acid and water, ions also play an important role in the formation of the atmospheric ultrafine particles, and they designed a kinetically consistent ion-mediated nucleation (IMN) theory that has provided new insight into the formation mechanism of atmospheric particles (CitationYu 2002, Citation2003).
According to the IMN theory, the charged molecular clusters/ particles have great growth advantage over corresponding neutral clusters/particles as a result of dipole–charge interactions. CitationNadykto and Yu (2003) showed that the enhancement in the attachment coefficient of the neutral molecule of free sulfuric acid to small ions/charged clusters may be as big as ∼ 10 in the lower troposphere, ∼ 15 in the polar stratosphere, and ∼ 20 in the mesosphere due to the high dipole moment of sulfuric acid molecule. Earlier measurement of sulfuric acid dipole moment (CitationKuczkowski et al. 1981; this work contains the only measurement to our knowledge reported in the literature) indicated that sulfuric acid molecule has a dipole moment of 2.73 D. Recent theoretical calculations by CitationAl Natsheh et al. (2003) suggested that the gas-phase sulfuric acid molecules may have different equilibrium structures that give different dipole moments. CitationAl Natsheh et al. (2003) also found that gas phase sulfuric acid hydrates have wide variations in the equilibrium structures and dipole moments. Since most of sulfuric acid molecules in the atmosphere are likely to be hydrated, it is important to understand how the variations in the structures and subsequent electrical properties of the hydrates influence the rate at which the charged clusters/ultrafine particles uptake the sulfuric acid vapor.
The present article discusses the effect of the structure variations and hydrates (mono-, di-, and trihydrates) of gas-phase sulfuric acid on the uptake of sulfuric acid by charged clusters/ultrafine particles of ∼ 0.5–3 nm in size.
STRUCTURE AND POLARITY OF GAS-PHASE SULFURIC ACID AND ITS HYDRATES
The hydration model employed in the classical BHN theory does not distinguish the difference between different configurations of the hydrates. However, hydrates of the sulfuric acid may have various geometrical configurations from close to spherical to almost linear (e.g., CitationDing et al. 2003). CitationAl Natsheh et al. (2003) performed the density functional theory (DFT) simulations of the optimized geometry of the gas-phase sulfuric acid and mono-, di-, and trihydrates of the sulfuric acid, and they calculated their dipole moments and total bonding energies (see ). 3, 3, 13, and 10 equilibrium structures have been identified for gas-phase sulfuric acid and mono-, di-, and trihydrates of the sulfuric acid, respectively.
TABLE 1 Dipole moment and total bonding energy (TBE) of gas-phase sulfuric acid and sulfuric acid hydrates from CitationAl Natsheh et al. (2003)
The DFT theory appears to be a relevant and precise tool for the studies of cluster formation at the molecular scale as it is able to reproduce the experimental structure of a variety of molecules and complexes with quite high precision. Recent studies for hydrogen-bonded systems show that DFT methods give accurate thermodynamics characteristics of hydrogen bonding (e.g., Arstila et al. 1998). The DFT theory we used is based on the Hohenberg-Kohn theorems (CitationHohenberg and Kohn 1964), the Kohn-Sham procedure (CitationKohn and Sham 1965), and the Levy amend (CitationLevy 1979). DFT becomes a proper tool for precise and accurate ab initio computation (CitationZacarias et al. 1999). In the present study we have employed the PW91 method, which has been proposed by CitationPerdew and Wang (1992) and includes both the exchange correction and correlation parts. Recent investigations (CitationElstner et al. 2001; CitationTsuzuki and Lüthi 2001; CitationPatey and Dessent 2002; Citationvan Mourik and Gdanitz 2002) have shown that the PW91 method gives a reliable representation of the nonbonded molecular interaction and the calculation of the structural data and energies.
As may be seen from Table 1, the ab initio calculations show very wide variations in the structures and electrical properties within each group. Such a big difference in the electrical properties (up to ∼ 1.34, 1.58, 2.60, and 2.14 times in the dipole moments of gas-phase sulfuric acid, mono-, di-, and trihydrate, respectively) is not surprising. Based on their own measurements of the structure and electrical dipole moment, CitationKuczkowski et al. (1981) have predicted the existence of different modifications of gas-phase sulfuric acid that lead to the variations in the hydrates' structures and properties. Despite the wide variations of the dipole moments, the total bonding energies of the structures are pretty close within each group that is believed to indicate quite close probabilities of the formation for different structures.
UPTAKE COEFFICIENTS
The uptake of sulfuric acid by charged ultrafine particles/ clusters can be calculated as the Brownian coagulation kernel (K mp ) (CitationFuchs 1964) between polar molecules/molecular clusters and charged clusters/particles in the free molecular regime,
RESULTS AND DISCUSSION
To illustrate the consequences of the variations in the structure and electrical properties of free and hydrated sulfuric acid, and to study the hydration effect, we compared the uptake coefficients calculated using EquationEquations (1) and (2). presents the upper and lower limits of the ratio between the uptake coefficients calculated using the maximum and minimum values of the dipole moments from (EF upper , EF lower ) and those (EF ref ) calculated using the reference measured value of the dipole moment of sulfuric acid (2.73 D; Kuczkowski et al. 1981) for (a) free sulfuric acid molecules, (b) monohydrates, (c) dihydrates, and (d) trihydrates. In order to estimate the overall effect, we included in the calculations performed using average value of the dipole moment for each group assuming the probability of the formation of different configurations to be equal (EF average ).
FIG. 1 The upper and lower limits of the ratio between the uptake coefficients calculated using the maximum and minimum values of the dipole moments from (EF upper , EF lower ) and those (EF ref ) calculated using the reference measured value of the dipole moment of sulfuric acid (2.73 D; Kuczkowski et al. 1981) for (a) free sulfuric acid molecules, (b) monohydrates, (c) dihydrates, and (d) trihydrates.
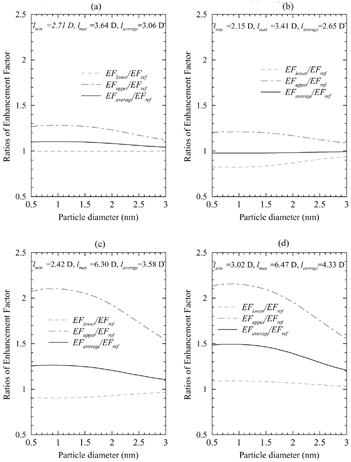
As may be seen from , the difference in the dipole moments leads to the significant variations in the uptake coefficients (up to ∼ 1.3, 1.5, 2.3, and 2.0 times for free sulfuric acid and mono-, di-, and trihydrate, respectively). The presence of a new configuration of the gas-phase sulfuric acid (CitationNatsheh et al. 2003) suggests that the average enhancement in the uptake coefficient may be higher than that calculated using the only measured dipole moment reported in the literature. Both dihydrates and trihydrates appear to have much higher enhancement factor than that of free sulfuric acid molecules and monohydrates. In general, the hydration is likely to enhance the uptake rate; however, this conclusion needs to be justified through a more rigorous study concerning the probability of the formation for different structures.
For kinetic calculations of cluster growth and nucleation, we need absolute values of the uptake coefficients for the sulfuric acid molecules/hydrates and ions/charged particles. shows the comparison of the uptake coefficients calculated using EquationEquations (1) and (2) with and without enhancement factors (EF) as a function of the particle diameter for (a) free sulfuric acid molecules, (b) monohydrates, (c) dihydrates, and (d) trihydrates at the ambient gas temperature of 300 K using the same input data set as that for . As may be seen from , absolute values of uptake coefficients for charged particles/ clusters are much bigger than those for the neutrals, which clearly shows that the charged particles/clusters do have a great growth advantage over the neutral ones. The uptake coefficients for the gas-phase sulfuric acid, monohydrates, dihydrates, and trihydrates interacting with molecular ions (diameter ∼ 0.5 nm) are in the range of 3.2–4.1× 10− 9 cm3/s, 2.8–3.7× 10− 9 cm3/s, 2.8–6.1× 10− 9 cm3/s, and 3.3–6.5 × 10− 9 cm3/s, respectively. Since the enhanced uptake of sulfuric acid molecules by charged clusters is critical to the ion-mediated nucleation of particles in the atmosphere (CitationYu and Turco 2000), it is necessary to evaluate how the variations in the structure and hydration of sulfuric acid affect the ion-mediated nucleation rates.
FIG. 2 The comparison of the uptake coefficients calculated using EquationEquation (2) with and without enhancement factors (EF) as a function of the particle diameter for (a) free sulfuric acid molecules, (b) monohydrates, (c) dihydrates, and (d) trihydrates at the ambient gas temperature of 300 K using the same input data set as that for .
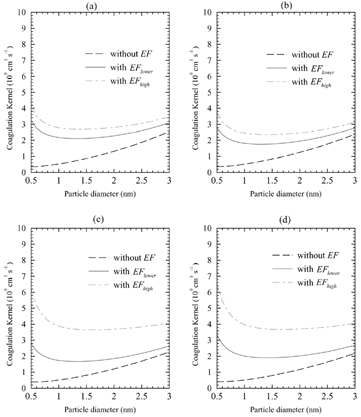
SUMMARY
In this article, the effect of the variations of the structure of gas-phase sulfuric acid and mono-, di-, and trihydrates of the sulfuric acid on the uptake of the sulfuric acid by charged ultrafine particles/clusters is investigated. The charged clusters/ultrafine particles have a great growth advantage over the neutral ones due to the dipole–charge interaction. We find that the different structures of gas-phase sulfuric acid and its hydrates lead to very big (up to ∼ 230%) variations in the uptake coefficients due to the large difference in the dipole moments. The hydration of the sulfuric acid is likely to increase the uptake coefficients; however, this effect has to be quantified through a more rigorous study concerning the probability of formation of different structures.
Acknowledgments
This work was supported by the NSF under grant ATM 0104966. KVM thanks the Danish Natural Science Research Council, the Danish Technical Research Council, Danish Center for Scientific Computing and the EU-network: MOLPROP for support.
REFERENCES
- Al Natsheh , A. , Nadykto , A. B. , Mikkelsen , K. V. , Yu , F. and Ruuskanen , J. 2003 . Dipole Moments of Sulfuric Acid and Sulfuric Acid Hydrates in Gas Phase: A DFT Investigation . Phys. Chem. Chem. Phys. , Submitted
- Arstilla , H. , Laasonen , K. and Laaksonen , A. 1998 . Ab initio study of gas-phase sulphuric acid hydrates containing 1 to 3 water molecules . J. Chem. Phys. , 108 : 1031 – 1039 .
- Clarke , A. D. , Davis , D. , Kapustin , V. N. , Eisele , F. , Chen , G. , Paluch , I. , Lenschow , D. , Bandy , A. R. , Thornton , D. , Moore , K. , Mauldin , L. , Tanner , D. , Litchy , M. , Carroll , M. A. , Collins , J. and Albercook , G. 1998 . Particle Nucleation in the Tropical Boundary Layer and its Coupling to Marine Sulfur Sources . Science , 282 : 89 – 92 .
- Ding , C. G. , Taskila , T. , Laasonen , K. and Laaksonen , A. 2003 . Reliable Potential for Small Sulfuric Acid–Water Clusters . Chem. Phys. , 287 ( 1–2 ) : 7 – 19 .
- Elstner , M. , Hobza , P. , Frauenheim , T. , Suhai , S. and Kaxiras , E. 2001 . Hydrogen Bonding and Stacking Interactions of Nucleic Acid Base Pairs: A Density-Functional-Theory Based Treatment . J. Chem. Phys. , 114 : 5149
- Fuchs , N. A. 1964 . Mechanics of Aerosols , Ann Arbor, MI : Ann Arbor Press .
- Hohenberg , P. and Kohn , W. 1964 . Inhomogeneous Electron Gas . Phys. Rev. B , 136 : 864 – 871 .
- Jaecker-Voirol , A. and Mirabel , P. 1989 . Heteromolecular Nucleation in the Sulfuric Acid-Water System . Atmos. Environ. , 23 : 2053 – 2057 .
- Kohn , W. and Sham , L. J. 1965 . Quantum Density Oscillations in an Inhomogeneous Electron Gas . Phys. Rev. A , 140 : 1133 – 1138 .
- Kuczkowski , R. L. , Suenram , R. D. and Loves , F. J. 1981 . Microwave spectrum, structure, and dipole moment of sulfuric acid . J. Am. Chem. Soc. , 103 : 2561 – 2566 .
- Levy , M. 1979 . Universal Varation Functionals of Electron Densities, First Order Density Matrices, and Natural Spin Orbitals and Solution of the v-Representability Problem . Proc. Natl. Acad. Sci. USA , 76 : 6062 – 6065 .
- Loeb , L. B. 1961 . Fundamentals of Electricity and Magnetism Dover, New York
- Nadykto , A. B. and Yu , F. 2003 . Uptake of Neutral Polar Vapor Molecules by Charged Clusters/Particles: Enhancement Due to Dipole–Charge Interaction . J. Geophy. Res. , 108 ( D23 ) : 4717
- Noppel , M. 1998 . Binary Nucleation of Water-Sulfuric Acid System: Reexamination of Classical Hydrates Interaction Model . J. Chem. Phys. , 109 ( 20 ) : 9052 – 9056 .
- Patey , M. D. and Dessent , C. E. H. 2002 . A PW91 Density Functional Study of Conformational Choice in 2-Phenylethanol, n-Butylbenzene, and Their Cations: Problems for Density Functional Theory? . J. Phys. Chem. A , 106 : 4623
- Perdew , J. P. and Wang , Y. 1992 . Pair-Distribution Function and its Coupling-Constant Average for the Spin-Polarized Electron Gas . Phys. Rev. B , 46 : 6671
- Tsuzuki , S. and Lüthi , H. P. 2001 . Interaction Energies of van der Waals and Hydrogen Bonded Systems Calculated Using Density Functional Theory: Assessing the PW91 Model . J. Chem. Phys. , 114 : 3949
- van Mourik , T. and Gdanitz , R. J. 2002 . A Critical Note on Density Functional Theory Studies on Rare-Gas Dimmers . J. Chem. Phys. , 116 : 9620
- Weber , R. J. , Marti , J. J. , McMurray , P. H. , Eisele , F. L. , Tanner , D. J. and Jefferson , A. 1996 . Measured Atmospheric New Particle Formation Rates: Implications for Nucleation Mechanisms . Chem. Eng. Comm. , 151 : 53 – 62 .
- Yu , F. 2002 . Altitude Variations of Cosmic Ray Induced Production of Aerosols: Implications for Global Cloudiness and Climate . J. Geophy. Res. , 107 ( A7 ) : 1118
- Yu , F. 2003 . Nucleation Rate of Particles in the Lower Atmosphere: Estimated Time Needed to Reach Pseudo-Steady State and Sensitivity to H2SO4 Gas Concentration . Geophys. Res. Lett. , 30 : 1526 – 1529 .
- Yu , F. and Turco , R. P. 2000 . Ultrafine Aerosol Formation Via Ion-Mediated Nucleation . Geophys. Res. Lett. , 27 : 883 – 886 .
- Zacarias , A. G. , Castro , M. , Tour , J. M. and Seminario , J. M. 1999 . Lowest Energy States of Small Pd Clusters Using Density Functional Theory and Standard ab Initio Methods. A Route to Understanding Metallic Nanoprobes . J. Phys. Chem. A , 103 : 7692 – 7700 .