Abstract
Particle-free air is required in a wide range of scientific and technical applications such as prefiltering for gas analyzers or for the artifact correction of particle mass concentration measurements. Most common processes for the removal of particles from a gas stream are filtration and electrostatic precipitation. However, both mechanism introduce changes to the thermodynamic conditions and/or the composition of the gas, which might be detrimental to the downstream measurement of gas or particle concentrations. For highly efficient separation of gas and particles with no changes to the thermodynamic conditions and substantially no changes to the gas phase, a coaxial gas particle partitioner (GPP, patent pending) has been developed and initially tested. The GPP utilizes corona charging to electrically charge the particles and a strong electric field in a separate unit to take them out of the sample flow when switched on. The vicinity of the corona wire gets continuously flushed by means of a wash flow, which is spatially separated from the sample flow, to prevent gases formed by the corona, such as ozone and oxides of nitrogen, from reaching the sample aerosol flow. Internally, the total aerosol flow is split into a sample flow and an excess gas flow. The splitting of the flow ensures that evaporation or release of particles deposited on the outer wall does not affect the sample flow but only the excess gas flow. The flow splitter is designed such that the particle number size distribution in the sample flow is identical to the ambient distribution when the GPP is switched off. When switched on, the sample flow is particle free. The initial investigations have shown that the GPP is able to separate gas and particles with a higher efficiency than another investigated common point-to-plane electrostatic precipitator (ESP). Particle losses inside the GPP are lower than in the ESP. The response time of GPP and ESP are similar.
INTRODUCTION
Gas particle separators are used in a wide range of applications, such as prefilters for gas analyzers or for zero-point control of online-particle mass concentration monitors (CitationAsbach et al. 2003; CitationPatashnick et al. 2001). Most commonly used processes are filtration, where particles are deposited on fibrous or membrane filters, and electrostatic precipitation, where particles get electrically charged due to a corona discharge, deflected, and deposited on a precipitation electrode.
Both filtration and electrostatic separation reach high separation efficiency of approximately 100% concerning the total ambient particle mass. However, both introduce changes to the gas phase and/or thermodynamic conditions. Such alterations of the aerosol may cause problems with the downstream measurement, as both gas analyzers and particle mass concentration monitors are very sensitive to changing thermodynamic conditions. Further changes to the gas compositions may negatively affect the output of a gas analyzer. In a particle mass monitor, a changed gas phase may enhance chemical reactions on the sample filter or gaseous species may recondense on the filter and therefore change the mass of the sample filter.
Filters change both the thermodynamic conditions and the gas composition, as firstly they always give rise to a pressure drop across the filter that increases with increasing filter loading, and secondly, deposited (semi-) volatile particles might evaporate from the filter (CitationFuruuchi et al. 2001; CitationRaynour et al. 1999) and mix with the aerosol's gas phase. In an electrostatic precipitator (ESP), a change of the gas composition occurs due to the corona discharge that forms mainly ozone (CitationBoelter and Davidson 1997; CitationViner et al. 1992) and nitrogen oxides (CitationBrandvold et al. 1989; CitationMartinez et al. 1996) and due to evaporation of particles deposited in the ESP. Several investigators have studied the influences on the formation of Ozone and Oxides of Nitrogen by corona discharge. CitationBrandvold et al. (1989) have found that in the investigated point-to-plane ESP the formation of Ozone (O3) was 7.2 ± 0.2 times higher and the formation of nitrous oxide (N2O) was 4.2 ± 0.2 times higher for negative compared to positive corona polarity. CitationBoelter and Davidson (1997) have studied the O3 formation in an indoor electrostatic air cleaner with a wire as corona electrode. They found the O3 concentration to be 40% lower for a wire of 100 μ m diameter instead of 200 μ m for the investigated tungsten wire. They concluded that the lower the wire diameter, the lower the ozone formation rate. Further, they found the O3 concentration to be 50% lower if silver wire is used and 30% lower if copper wire is used in place of the tungsten wire. The latter agrees well with the investigations of CitationNashimoto (1988). He found that among eight tested wire materials, silver formed the lowest amount of O3 and concluded that the ozone formation rate is dependent on the |Δ H o | (highest standard heat of formation of oxides per oxygen atom).
For the development of the gas particle partitioner (GPP), all of these conclusions were taken into account for a reduction of the formation of O3 and Oxides of Nitrogen. Further, a particle-free corona wash flow, spatially separated from the sample flow, is introduced into the device to avoid a mixing of the formed gases with the sample flow. The total aerosol flow is internally split into the sample flow and an excess gas flow, such that the sample flow is particle free when the GPP is switched on. When the GPP is switched off, the aerosol remains unchanged, i.e., the particle size distribution also remains unaltered. The latter is required when the GPP is employed in zero point control, e.g., for online particle mass concentration monitors or Nephelometers.
DESIGN OF THE GPP
The GPP is a cylindrical and coaxial arrangement that is separated into four zones: aerosol inlet, charging zone, fractionation zone, and aerosol outlet. illustrates the general design along with the flow rates and the rough dimensions for the employment in a monitor for the real particle mass concentrations (CitationAsbach et al. 2003; CitationPatashnick et al. 2001). The total flow is internally split into a sample flow and an excess flow. The flow splitter is designed such that no change of the particle size distribution in the aerosol occurs when the GPP is switched off (), but all particles either reach the excess gas flow or get deposited on the outer wall when it is active ().
The aperture angle of the aerosol inlet cone is 30° in accordance with the VDI guideline for similarly designed plane filter devices (VDI 1993). This ensures that no turbulences occur, as the entire GPP is designed for laminar flow conditions. In the charging zone, the inner electrode consists of a stainless steel grid that has the same electric potential as the remaining inner electrode in the fractionation zone. A 25 μm corona wire is situated in the centre of the grid electrode. By applying a high voltage to the wire (corona voltage), air molecules in the vicinity of the wire get ionized and deflected towards the grid electrode. Since a voltage of same polarity as the corona voltage is applied to the grid electrode while the outer wall of the GPP is grounded, the ions penetrate through the grid into the charging zone between the grid and the outer wall. Here the particles get charged due to both diffusion and field charging (CitationHinds 1982). To avoid that gases formed by the corona—mainly ozone (CitationBoelter and Davidson 1997; CitationViner et al. 1992) and nitrogen oxides (CitationBrandvold et al. 1989; CitationMartinez et al. 1996), enter the charging zone and mix with the aerosol's gas phase, the corona region inbetween the grid gets continuously flushed by means of an additional particle-free wash flow. The flow rate of the wash flow is chosen to have the same velocity as the aerosol flow to minimize mixing of the two flows. The wash flow transports the gases formed by the corona out of the GPP. To assure an efficient charging of the particles, an n
i
· t
r
product of ion concentration n
i
and residence time in the ion environment t
r
of 107
has been assumed to be sufficient for highly efficient charging (CitationHinds 1982). Since the ion concentration cannot be determined in advance, an average concentration of n
i
= 107
has been assumed for the design of the charging zone. In that assumed case, a particle residence time of t
r
= 1 s is sufficient for efficient charging. The residence time of a particle in the charging zone may be calculated by means of the velocity distribution for a flow in an annulus, as given in EquationEquation (1) (CitationBird 1960):
In addition to the wash flow, several measures were taken to avoid an effect of the charging process on the gas phase. Firstly, positive polarity has been chosen for the corona, since several investigators (e.g., CitationBrandvold et al. 1989; CitationMartinez and Brandvold 1996) have shown that positive corona produces a lower amount of ozone and nitrogen oxides. Secondly, a 25 μ m silver wire has been chosen for the corona wire. CitationNashimoto (1988) has found that among eight tested wire materials, silver formed the lowest amount of ozone (approximately 70% less than gold), whereas the formation of N2O is just slightly higher (+10%). CitationBoelter and Davidson (1997) have shown for tungsten wires that, at the same efficiency, a thinner wire produces a lower amount of ozone. A wire diameter of 25 μm seems to be the lowest manageable diameter. Thirdly, due to the design of the flow splitter, gases formed by evaporation of deposited particles from the outer wall may only affect the excess gas flow but not the sample flow.
For the design of the fractionation zone, the trajectory of a “worst case particle” has been calculated. The definition of the “worst case particle” is (1) lowest possible electrical mobility for PM10 particles (due to the impactor characteristic, the PM10 fraction includes particles of up to approximately 13 μm), and (2) particle initially (t = 0) situated directly at the surface of the inner electrode (r = r i ) at the transition from charging zone to fractionation zone (see ). The lowest mobility was estimated by means of the charge distribution of particles, charged by a corona discharge (CitationHinds 1982) to be Z p,min = 7.3 · 10−8m2 Vs (for d p = 0.3 μm, n = 15 elementary charges). The trajectory of that particle was then calculated by the superposition of the radial motion r(t) and the longitudinal position z(t) · r(t) can be derived from the radial velocity v r (r(t)) of the regarded particle:
Integration of u(r(t)) (EquationEquation (8) in EquationEquation (5)) gives the longitudinal position z(t):
FIRST RESULTS AND DISCUSSION
Ion Concentration
Prior to the design of the GPP, assumptions were made for the ion concentration in the charging zone. A product of ion concentration n
i
and residence time t
r
of n
i
· t
r
= 107
was assumed to be sufficient for a highly efficient charging of the particles. To determine the ion concentration n
i
(r) in the charging zone, the ion current I
i
has to be known. CitationBüscher et al. (1994) have found the expression for the ion concentration for a very similarly designed particle charger to be
To measure the ion current produced by the corona discharge, an aluminium foil was installed on the inside of the outer electrode and electrically insulated from the electrode. The ion current was measured by means of a highly sensitive ampere meter connected to the aluminium foil via a shielded wire. The average measured ion current was 3.72 μ A for a deflection voltage of U i = 2 kV and a corona voltage of U cor = 10 kV, where the corona voltage is the potential difference between the corona potential of 12 kV and the grid potential of 2 kV. For the determination of the electric field strength, space charge effects have to be taken into account, since they strongly interfere with the electric field distribution. The electric field strength can be expressed as (CitationBüscher et al. 1994)
Separation Efficiency
As a next step, the total number concentration of particles in ambient air was measured upstream and downstream of the GPP in both active and inactive mode in order to draw conclusions about the general functionality of the GPP concerning gas particle separation. Ambient air was chosen as sample aerosol, as the GPP will mainly be applied for separating atmospheric particles from ambient air. The total number concentration was measured, as it is a very sensitive measure to judge the separation efficiency. However, measurements with monodisperse fine and coarse particles have recently been carried out. Since the main objective of this study is the development of the GPP, the results will be published separately in the near future.
A condensation particle counter (CPC; TSI, Model CPC 3022) was used to measure the particle number concentrations. For the determination of the losses in the GPP, a three-way valve was installed and the air was either being transported through the GPP or through a bypass. In , the ambient particle number concentration is plotted against the number concentration downstream of the inactive GPP. It can be seen that less than 1.5% of the total ambient particle number is getting lost in the GPP.
FIG. 5 Ambient particle number concentration versus particle number concentration downstream of inactive GPP.
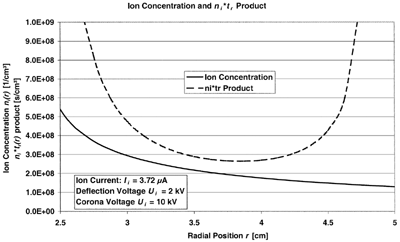
For derivation of the separation efficiency, a PC was used to download the data from the CPC and to switch the power supplies on and off every 60 s. Altogether 98 measurements were carried out for each voltage setting. The separation efficiency η was then calculated for each single measurement by means of EquationEquation (8):
Gas Phase
The ozone and nitrogen dioxide concentrations in the sample flow were recently measured downstream of the GPP with active corona. Different wire materials, wire diameters, and positive and negative polarity of the voltages were used to investigate the effect on the gas composition of the sample flow. Considering a 100 μ m gold wire used along with negative polarity and without wash flow as the worst case, the measures for a minimization of the influence of the corona on the sample flow resulted in a reduction of the additional ozone concentration of approximately 98.5% and in approximately 89% lower nitrogen dioxide concentrations. However, a detailed description of the gas phase measurements will be published along with the abovementioned results for monodisperse particles in a separate paper in the near future.
COMPARISON TO A COMMON ESP
The performance of the GPP has been compared to that of a common point-to-plane ESP. The ESP is equipped with a potentiometer by which the corona current is adjusted. The electronics of the ESP are designed such that a constant corona current is maintained for a fixed potentiometer setting. The precipitation efficiency of the ESP was investigated with a CPC (TSI, Model 3022). The total number concentration of particles in ambient air and downstream of the ESP were measured alternatively with the same setup as for the GPP, and the precipitation efficiency was calculated. The investigations showed that at a corona current of approximately 60 nA the ESP reaches a maximum efficiency of 92.3% with a standard deviation of 0.8%. Compared to the maximum separation efficiency of the GPP of 96%, it can be concluded that the GPP takes particles out of a gas with a higher efficiency than the investigated common ESP. Furthermore the particle losses have been studied. Approximately 5% of the total particle number in ambient air get lost inside the ESP compared to < 1.5% in the GPP.
Maintenance intervals are much longer for the GPP due to two reasons. Firstly, inside the GPP only one part of the particles gets deposited on the outer wall, whereas a large fraction of particles is carried out of the GPP with the excess gas flow. In the ESP, all particles get deposited on the outer wall that has direct contact to the sample flow. Hence the required cleaning intervals of the GPP are longer. Secondly, the corona wire does not come in contact with particles. Therefore, no residue may build up on the corona electrode as it did on the corona tip in the ESP during the measurements. This residue needs to be wiped away frequently.
For practicability and maintenance reasons, it is suggested that gold or tungsten wire be installed in the GPP instead of silver wire. During the investigations, corona wires made from silver broke frequently (25 μ m wires lasted for approximately 48 h), while gold and tungsten wires lasted for more than three months. Even though there is a higher production of ozone when gold or tungsten wire is used, we expect that most gases formed by the corona are washed out by the wash flow and do not affect the sample's gas phase.
TABLE 1 Comparison of GPP and ESP
Compared to the investigated ESP the GPP has several advantages:
• | Higher separation efficiency | ||||
• | Lower particle losses | ||||
• | Due to its design, substantially no changes of the aerosol's gas phase | ||||
• | Longer maintenance intervals (when gold or tungsten wire is used) |
SUMMARY
A gas particle partitioner (GPP, patent pending) for highly efficient separation of gas and particles with substantially no effect on the gas phase has been developed and initially tested. The GPP is designed such that the aerosol's thermodynamic conditions remain unaltered. Several measures were taken to leave the gas composition of the aerosol substantially unaffected, i.e., a corona wire of only 25 μ m diameter was chosen along with positive corona polarity. Initially, silver was chosen as corona wire material, as silver is supposed to produce the lowest amount of ozone. In the course of the experiments, it has been found that silver wire broke very frequently (on average every 48 h), whereas gold and tungsten wires lasted for several months. Therefore we suggest the use of gold or tungsten wire in the GPP as we expect most gases formed by the corona to be in the wash flow that does not mix with the sample flow. When the GPP is switched off, the aerosol penetrates through the device without any changes. This is required for several applications, such as the zero point control in online monitors for ambient particle mass concentrations. In the latter case, alternatively the GPP is switched on and off. When switched off, the particle mass concentrations are determined conventionally, whereas when the GPP is switched on, the net sum of all artifacts that bias the measurement is being determined.
The investigations have shown that the GPP is able to separate the gas and particulate phase efficiently and with a short response time. The separation efficiency is approximately 96% with the remaining 4% particles penetrating through the GPP assumed to be uncharged nanoparticles with only negligible effect on the downstream process. Particle losses inside the GPP are low (< 1.5% by number). The design of the GPP is such that thermodynamic conditions and gas composition of the sample aerosol remain substantially unaltered.
Further investigations concerning the gas phase, sizedependent, and mass-related separation efficiency were recently carried out and will be published in the near future.
Acknowledgments
The authors wish to thank Rupprecht & Patashnick Co., Inc., 25 Corporate Circle, Albany, NY 12203, USA for supporting this work.
REFERENCES
- Asbach , C. , Kuhlbusch , T. A. J. and Fissan , H. 2003 . “ Online Monitor for Measurement of Real Airborne Particle Mass Concentrations ” . In Proceedings of QA/QC in the Field of Emission and Air Pollution Control Conference, Prague , European Commission Joint Research Centre . (accepted for publication)
- Bird , B. 1960 . Transport Phenomena , New York : John Wiley & Sons .
- Boelter , K. J. and Davidson , J. H. 1997 . Ozone Generation by Indoor, Electrostatic Air Cleaners . Aerosol Sci. Technol. , 27 : 689 – 708 .
- Brandvold , D. K. , Martinez , P. and Dogruel , D. 1989 . Polarity Dependence of N2O Formation from Corona Discharge . Atmos. Environ. , 23 : 1881 – 1883 .
- Büscher , P. , Schmidt-Ott , A. and Wiedensohler , A. 1994 . Performance of a Unipolar “Square-Wave” Diffusion Charger with Variable nt-Product . J. Aerosol Sci. , 25 : 651 – 663 .
- Furuuchi , M. , Fissan , H. and Horodecki , J. 2001 . Evaporation Behavior of Volatile Particles on Fibrous Filter Flushed with Particle Free Air . Powder Technol. , 118 : 171 – 179 .
- Hinds , W. 1982 . Aerosol Technology , New York : John Wiley & Sons .
- Martinez , P. and Brandvold , D. K. 1996 . Laboratory and Field Measurements of NOx Produced from Corona Discharge . Atmos. Environ. , 30 : 4177 – 4182 .
- Nashimoto , K. 1988 . The Effect of Electrode Materials on O3 and NOx Emissions by Corona Discharging . J. Imaging Sci. , 32 : 205 – 210 .
- Patashnick , H. , Rupprecht , G. , Ambs , J. L. and Meyer , M. B. 2001 . Development of a Reference Standard for Particulate Matter Mass in Ambient Air . Aerosol Sci. Technol. , 34 : 42 – 45 .
- Raynour , P. C. and Leith , D. 1999 . Evaporation of Accumulated Multicomponent Liquids from Fibrous Filters . Ann. Occup. Hyg. , 43 : 181 – 192 .
- Verein Deutscher Ingenieure (VDI) . 1993 . Measurement of Particulate Matter: Manual Dust Measurement in Flowing Gases Gravimetric Determination of Dust Load Plane Filter Devices Guideline VDI 2066, Part 7
- Viner , A. S. , Lawless , P. A. , Ensor , D. S. and Sparks , L. E. 1992 . Ozone Generation in DC-Energized Electrostatic Precipitators . IEEE Trans. Ind. Applicat. , 28 : 504 – 512 .