Abstract
Using on-line analysis of single particles, we have observed both generation and resuspension of particles when ice crystals, cloud droplets, or dust impact an aircraft inlet. Large numbers of particles smaller than 1 μ m with a composition suggesting stainless steel were measured when flying a stainless steel inlet through cirrus clouds. Smaller numbers of metal particles were also observed when flying through dust or water clouds. A different instrument, sampling through a different inlet, found zinc particles when sampling in cirrus clouds. Laboratory experiments have verified that high-speed ice crystals can abrade stainless steel. Collision of ice crystals with the inlet wall also resuspended previously deposited particles. A notable example came when a flight through the space shuttle exhaust plume deposited large numbers of unique particles in our inlet. Some of the same types of particles were observed when the aircraft flew into an ice cloud the following day. The generation of particles by impaction of ice crystals and dust in inlets may have affected some published results about ice nuclei and metal particles in the upper troposphere. The newly generated particles cannot be distinguished from atmospheric particles by size alone.
INTRODUCTION
The accurate sampling of particles from aircraft is a difficult but important problem in studying atmospheric aerosols (CitationBaumgardner and Huebert 1993). Many properties of particles, such as their chemical composition, cannot be retrieved by remote sensing. Aircraft are the most feasible means of sampling much of the atmosphere from just above ground level to about 20 km altitude.
It is difficult to sample particles from aircraft because of the speed, which is often 100 m s−1 for turboprop aircraft and 200 m s−1 for jet aircraft. For a variety of reasons, such as transmitting particles around bends, the flow in an inlet taking air to aerosol instrumentation must be slower than the aircraft speed. The flow must either be slowed down or there must be a velocity mismatch at the entrance to the inlet. Decelerating flows are prone to turbulence and loss of particles. In some cases most of the aerosol mass is deposited on the walls of the inlet rather than transmitted to instrumentation inside the airplane (CitationHuebert et al. 1990). The other option, a velocity mismatch or anisokinetic sampling, causes a size-dependent enhancement in the number of particles compared to the ambient concentration (CitationBelyaev and Levin 1974; CitationHangal and Willeke 1990). These enhancements can be large and cannot be corrected for unless the downstream instrumentation can sort results by particle size, density, and water uptake. The flow around the aircraft itself can also alter the concentration of particles, even well outside the boundary layer (CitationKing 1984).
Most previous studies of the interaction of particles and inlet walls have emphasized losses of particles to the wall. Shattering of water droplets and ice crystals has also been mentioned (CitationGardiner and Hallett 1985; CitationWeber et al. 1998). Here we address a problem in aircraft sampling that has received less attention: false signals created when large particles such as ice crystals or dust particles impact the wall of an inlet. Our data indicate that ice crystals are capable of abrading submicron particles from a stainless steel inlet or the aircraft surface when they impact at aircraft velocities. They can also resuspend previously deposited particles.
Before turning to experimental data, it is instructive to examine some of the length scales for cloud particles entering an aircraft inlet (). All calculations in are for 120 mbar, 293 K, and an aircraft velocity of 190 m s−1. These are applicable to the WB-57 measurements discussed below. The first parameter is the stopping distance, which is defined as the distance for a particle to come to a complete halt when injected into a flow at a given velocity. The stopping distance is strictly defined for particles obeying a Stokes law drag function in which the drag force is proportional to velocity to the first power. This is appropriate for very small particle Reynolds numbers (Re < 0.1). The calculations here include a first-order correction to the drag coefficient CD = 24 (1+0.0916 Re)/Re, which is valid to Re ≈ 5 (CitationRader and Marple 1985). Nevertheless, the stopping distances in may be too short for the 100 μ m case because their Reynolds numbers exceed 5. The stopping distance becomes shorter at lower altitudes where there is more air drag but the change is less than linear in air density. For 5–100 μ m ice crystals, the stopping distance is shorter by a factor of 2.8 to 5 at sea level compared to 120 mbar, or about a factor of 8 in pressure. The stopping distance is only weakly dependent on temperature.
TABLE 1 Length scales for spherical particles with density 0.9 g cm−3 at 190 m s−1 and 120 mbar
The angular tolerance parameter in is an estimate of the maximum angle that the particle velocity vector can be from the axis of a 5 mm diameter inlet and still make the turn to follow the inlet without hitting the wall. 5 mm is chosen as illustrative of the diameter sampling inlets often used on aircraft. The pitch angle of an aircraft may vary by a few degrees in “level” flight depending on altitude and fuel load. Therefore, an inlet alignment requirement tighter than about 3° cannot be met without active alignment in flight. The final parameter in is given as a guide to how far an ice crystal will fall under the influence of gravity before it evaporates inside a heated inlet. Although the vapor pressure of water is exponential in temperature, the evaporation rate for these conditions is only roughly linear in temperature because the evaporation is limited by the heat transfer needed to supply the latent heat of evaporation.
shows that sampling ice crystals smaller than 5 or 10 μ m is feasible without the crystals hitting a wall. As the particle size approaches about 20 μ m, a fraction of the crystals will start to hit the wall due to flow misalignment. At about the same size a typical inlet will not be long enough to stop large crystals before they reach the first bend or constriction. Even if the inlet is long enough, ice crystals larger than about 25 μ m may fall to the bottom of the inlet by gravity before they can be evaporated. By 100 μ m, the length scales show that ice crystals can neither be aligned with the airflow nor brought to a stop in a reasonable size inlet. Particles larger than 50 μ m are common in cirrus clouds (e.g., CitationMcFarquhar and Heymsfield 1997). Therefore, based on calculations we expect that ice crystals will impact the walls of any forward-facing aircraft inlet when flying in a cirrus cloud.
INSTRUMENTATION
The particle analysis by laser mass spectrometry (PALMS) instrument measures the mass spectra of the ions produced when single particles are hit by a pulse from an excimer laser (CitationThomson et al. 2000). Because the measurement is performed immediately on the airplane, each particle can be associated with a specific sampling time. This is crucial to understanding data in clouds, which may have considerable fine structure. The instrument can also observe volatile species. For the data discussed below, PALMS was mounted in the nose of the high-altitude NASA WB-57 aircraft (jet, ceiling 19 km) or in a wing pod on the NOAA P3 aircraft (turboprop, ceiling 8 km).
Two inlets were used for the PALMS instrument (). In both cases air was brought to the vicinity of the instrument through a 5 cm fiberglass tube with an aerodynamic shroud. This flow was roughly at aircraft velocity. Before 2002, a forward-facing, 1 mm ID, 30 cm long glass-lined capillary brought sampled air directly into the PALMS instrument (). During 2002, the capillary was replaced with a counterflow virtual impactor (CVI) inlet (CitationOgren et al. 1985). In it, ice crystals and water droplets larger than about 5 μ m have sufficient momentum to penetrate a short region of reversed airflow. The particles are then evaporated in a heated region to yield ice residues containing the original ice nucleus with material added or removed in cloud. Our design was based on CitationLaucks and Twohy (1998) with several significant changes: a shorter frit (1.2 cm instead of 8 cm), a smaller inner diameter (4 mm instead of 6.2 mm), and a vacuum jacket to reduce thermal gradients. The tubing and frit were all stainless steel. Instead of using the entire flow, air from the CVI was sampled at 90° through an oval tube approximately 1 mm in diameter to go into an aerodynamic focusing lens (CitationSchreiner et al. 1999) that served as the interface into PALMS. The counterflow on the CVI was modulated in flight to either accept or reject aerosol particles less than about 5 μ m. The rate of particles entering PALMS dropped by a factor of over 500 when the counterflow was turned on and the airplane was not in a cloud. The few remaining particles may have been due to isolated ice crystals falling from cirrus clouds above the aircraft.
FIG. 1 Diagrams of the PALMS aircraft inlets. (a) Air was drawn into either the nose of the aircraft or wing pod through a 5 cm tube. The center of this flow was sampled either with a capillary or with a counterflow virtural impactor (CVI). (b) Detail of the CVI inlet.
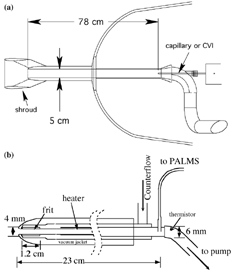
During the 2002 CRYSTAL-FACE mission, single particles were also collected using a multiple impactor sampler on the WB-57 for later electron microscope analysis (CitationSheridan et al. 1994). This sampler used a forward-facing aluminum inlet approximately 31 cm from the lower fuselage of the WB-57. Electron microscopy was done at Arizona State University using a JEOL 2000FX transmission electron microscope operated at 200 kV. Structural information was obtained using selected-area electron diffraction (SAED). Analysis of aerosol particles was done with a KEVEX energy-dispersive X-ray spectrometer attached to the microscope. The system can detect elements with atomic number greater than 4.
METAL PARTICLES
When measuring in cirrus clouds with the CVI inlet, the mass spectra of ice residues accessible to PALMS (about 0.15 to 3 μ m diameter) often showed Fe, Cr, Mo, and other peaks characteristic of stainless steel (). A few metal spectra were also observed that contained Na, Al, Cu, or other elements in addition to Fe and elements characteristic of stainless steel. When the CVI was in counterflow mode, metal spectra were about 90% of the particles observed in cirrus clouds over the entire range of ice crystal concentrations from 0.005 to over 20 cm−3. Most negative ion spectra showed large chlorine and oxygen peaks in addition to ions from metal oxides (). Conversely, metal particles were not observed in clouds when the glass-lined capillary inlet was used instead of the stainless steel CVI. Some particles containing barium and probably silicon were observed with the glass-lined capillary when sampling in clouds. It is not clear if these came from abrasion of the glass or from some other source.
FIG. 2 Sample mass spectra of single particles. (a) and (b) Negative and positive ion spectrum of particles using a counterflow virtual impactor in a cirrus cloud. These are inferred to be a stainless steel particle formed by collision of ice crystals with the inlet. (c) Metal particle produced in the laboratory by impact of an ice crystal on a stainless steel frit. (d) Positive ion spectrum of a particle with meteoric material. Despite the Fe, Cr, and Ni peaks in common with stainless steel the meteoric material is easily distinguished by, for example, sulfate, the large Mg peak, lack of Mo, and a specific Na/K ratio.
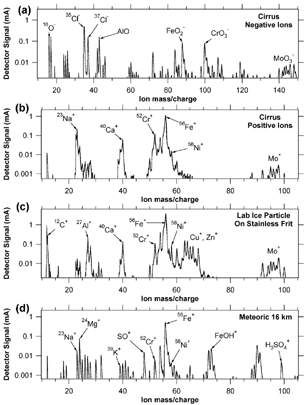
Metal spectra indistinguishable from those observed in cirrus clouds on the WB-57 were also observed when flying in clouds near 7 km altitude on the NOAA P3. Metal particles were also observed, although at a much lower rate, in low-altitude (about 100 m) marine stratus clouds off the California coast. Some of these metal particles were observed at temperatures above 0°C when the cloud droplets must have been liquid. Finally, metal particles were also observed during a portion of a flight at a few km over Arizona when a considerable number of coarse mode dust and/or fly ash particles were present.
The frequency of the metal particles was closely related to the concentration of either ice crystals or dust (). The percentage of metal spectra when sampling only ice crystals held steady at about 90%, independent of the number density of ice crystals in the clouds (). With the counterflow off, the inlet also sampled interstitial particles that are not associated with ice crystals. These diluted the metal particles, resulting in a lower fraction of metal particles in clouds. The dust particles appear to be less efficient than ice at producing metal particles. This effect could be real due to the larger size of the ice crystals, or it could be due to different inlet flow conditions at the much lower altitudes of the dust compared to the high cirrus. Aerodynamic sizing of single particles (CitationPrather et al. 1994) was added to PALMS in 2002 (CitationMurphy et al. 2004). Aerodynamic diameters of the metal and nonmetal particles are shown in . The metal particles were slightly larger on average, but it is clear that a size measurement alone could not distinguish the metal from the nonmetal particles. The transmission of particles into the PALMS vacuum system has not been calibrated for dense, possibly nonspherical particles such as metals. A change in tramsmission could be partly responsible for the dearth of metal particles larger than about 1 μ m.
FIG. 3 The rate of metal particles observed by PALMS as a function of the concentration of large particles. The cirrus cloud curves are from the NASA WB-57. The cloud concentration is defined as the particles larger than 10 μ m measured by the CAPS probe (CitationBaumgardner et al. 2002). The Arizona curve is from the NOAA P3 outside of cloud (relative humidity less than 15%) for which the coarse-mode particles are those larger than 2.5 μ m measured by a Climet 105 counter. These coarse particles were dust and fly ash from power plant plumes. There were 10461 particles sampled with counterflow on in cloud, 2838 with counterflow off in cloud, and 5479 with counterflow off out of cloud (lower left point).
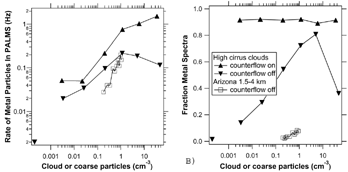
Metal particles were also found in the impactor instrument, which used a separate inlet further back on the airplane. Transmission electron microscopy showed that many single particles that collected in cirrus clouds contained zinc (). These zinc-rich particles were up to 1.5 μ m in diameter and were more than 40% of the particles in some cloud samples (CitationKojima et al. 2004). These samples were collected simultaneously with the apparent stainless steel sampled by PALMS. Interestingly, zinc was almost never detected by PALMS. We do not understand the source of the zinc. Stainless steel at PALMS is logical since the PALMS inlet is made of stainless steel, but the inlet to the impactor sampler on the WB-57 is made of aluminum with nominally no zinc present. A possible source for the zinc is from the fuselage of the aircraft ahead of the inlet. Fluid mechanics calculations indicate that ice crystals can bounce out to more than 1 m from the aircraft (Marty Ross, 2003, private communication). Because it is in the nose, PALMS would not sample particles coming off the fuselage.
FIG. 4 Aerodynamic size distribution of particles measured in cirrus clouds with the counterflow active so that only ice crystals could enter the CVI inlet. The nonmetal particles are primarily residues of ice crystals. The lower size limit for detection by PALMS for these flights was about 0.12 μ m optical diameter with decreasing efficiency below about 0.2 μ m.
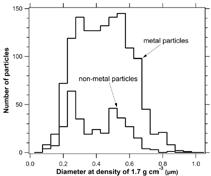
FIG. 5 Transmission electron microscope image of particles collected from clouds with the impactor sampler on the WB-57. Angular-to-irregularly-shaped particles (indicated by arrowheads) contain Zn and minor C and O.
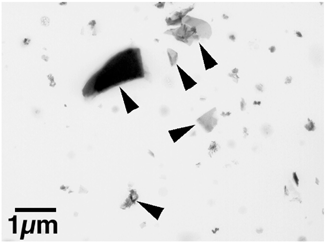
Laboratory experiments were performed in order to test the ability of ice crystals to remove material from metal surfaces. Submicron “seed” aerosols were produced by flowing 1.0 liter per minute (lpm) dry nitrogen through a fritted bubbler containing 20 wt% NaCl dissolved in distilled water. This flow was combined with 2.0 lpm nitrogen saturated by passing it through a second fritted bubbler containing only distilled water. The combined flow was passed into a 2.5 l cylindrical mixing volume surrounded by dry ice (−78°C). It is assumed that all particles froze because this is below the homogenous freezing point of dilute salt solutions (CitationKoop et al. 2000). No attempt was made to determine ice crystal size. The ice crystals were accelerated toward a target when the flow was expanded from 840 to 80 mbar through a 1 mm capillary.
Three types of target material were alternately placed in front of the flow 5 mm from the capillary exit: porous stainless steel identical to the CVI frit material, IC-grade stainless steel tubing, and gold. The actual CVI was not used because we had no way in the lab of placing it in a suitable flow that was seeded with ice. After the target the flow was heated to 25°C so that any remaining ice crystals were melted and evaporated. Then the PALMS instrument was used to determine the composition of any particles that were present. Both the frit and the tube produced stainless steel particles smaller than 1 μ m with mass spectra similar to those observed during flight in ice clouds (see ). The porous frit target produced more metal particles than the tubing relative to NaCl particles which, in this case, were a measure of the number of ice crystals. The gold target produced the lowest frequency of metal particles, and was spectroscopically unambiguous due to the presence of a peak at positive mass 197 (Au+). Two control experiments were also performed. First, ice crystals were produced but no target was held at the capillary exit. Second, targets were held at the capillary exit but in the absence of dry ice around the mixing volume (i.e., submicron aqueous aerosols, not ice crystals, were formed). Metal particles were not observed in either control case.
RESUSPENSION OF PARTICLES
Resuspension of previously deposited particles from the glass-lined capillary inlet was observed when flying in cirrus clouds. A clear example is shown in . A flight through the space shuttle plume provided a source of unique particles consisting of alumina from the aluminum perchlorate solid rocket fuel along with various additives and impurities (CitationCziczo et al. 2002). No such particles were observed during approximately the first hour of operation on the return flight to Houston from Cape Canaveral the next day, but then nearly identical mass spectra were observed when the WB-57 flew into a high cloud. Trajectories do not go back to the launch site when this particle was observed. Another example of resuspension was observed during our 1999 WB-57 flights. For those flights our inlet was contaminated with tin-carbon particles after drawing air into the instrument from a nearby soldering iron during lab tests. There were no cloud probes on the WB-57 for these flights, but tin-containing particles only appeared when the air was saturated with respect to ice, strongly suggesting that ice crystals were responsible for knocking the tin particles from the inlet wall.
FIG. 6 Mass spectra of particles in the Space Shuttle exhaust plume and in a cloud the next day. The latter particle was probably deposited in the inlet during the plume encounter and dislodged by an ice crystal.
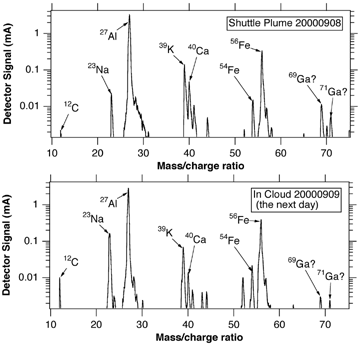
Previously deposited particles were not observed during flights with a clean capillary inlet during 1998. The particles may not have been sufficiently unique to recognize. Both the space shuttle and a soldering iron produce solid particles that are probably more easily resuspended than typical atmospheric particles that are liquid or at least coated with a liquid. So far, there has been no clear evidence of resuspension in the CVI inlet. The capillary inlet may be especially susceptible to resuspension because of the very high shear between a near-sonic flow at the center and the wall only 0.5 mm away. There also may have been fewer unique particles to trace resuspension in the CVI inlet.
DISCUSSION
We have three pieces of evidence that ice crystals can produce submicron metal fragments when they impact a stainless steel inlet. First, lab experiments duplicate this phenomenon. Second, there is a close association between stainless particles and the density of cirrus cloud. This association is newly observed because of the real-time response of PALMS. The occurrence of zinc in the MACS samples is also strongly correlated with cloud density (CitationKojima et al. 2004). Finally, the similarity of the stainless steel particles produced by encounters with cirrus clouds and the dust over Arizona is important since there is little doubt that dust particles could sandblast stainless steel.
The interaction of ice crystals with the stainless steel surface may not be determined by the bulk properties of stainless steel. First, the particles abraded from the stainless steel surface are considerably smaller than the grain size of the steel, which is roughly 40 μ m for a 316 grade stainless with ASTM 5 to 8 grain size (CitationLyman 1961). Second, stainless steels are covered with an oxide coating, and this may affect the mechanical properties at these small scales. Finally, the interaction of micrometer-sized particles with surfaces involves very high stress rates so that the dynamic yield properties of the materials involved must be taken into account (CitationJohn 1995).
The energetics of the collision of an ice crystal with the inlet at speeds of 100 to 200 m s−1 put some bounds on what can happen in the collision. There is insufficient kinetic energy to supply the latent heat required to totally vaporize the ice crystal. Aircraft velocities are well above the thresholds for both bounce and permanent deformation of micron-sized particles (CitationDahneke 1995; however, none of those data are specifically for ice). Studies using flat-plate impactors have demonstrated that ice crystals readily bounce off ungreased plates. In fact, this property has been used to separate ice crystals and water droplets (CitationTenberken-Potzsch et al. 2000). Graupel and aggregated ice crystals larger than 100 μ m can shatter when they impact a surface at 100 m s−1 (CitationGardiner and Hallett 1985). CitationSugi et al. (1998) studied the impact of 250 mg ice projectiles with velocities up to 300 m s−1 onto ice surfaces at normal incidence. Below 100 m s−1, almost none of the energy goes into vaporization, whereas between 150 and 180 m s−1 18–26% of the kinetic energy is partitioned into vaporization. Impacts at aircraft velocity have enough energy to produce shattering in the ice substrate. However, their projectiles have over 106 times the mass of a 50 μ m ice crystal, so it is not clear that the collision dynamics are the same.
The cause of the high chlorine content of the stainless steel particles () is uncertain. However, chlorine is a main cause of corrosion of 316 grade stainless steel in the atmosphere, especially in marine environments like those encountered in the CRYSTAL-FACE flights in 2002 based in Key West, Florida. It is therefore possible that chlorine was present on the surface of the stainless steel.
CitationHuebert et al. (1990) reported recovery of large amounts of coarse-mode aerosol by washing an aircraft inlet after a flight. This shows that the material available to be resuspended by collision of cloud droplets in the inlet is probably biased towards the coarse mode.
PREVIOUS STUDIES
It is likely that metal particles formed by ice crystals abrading aircraft inlets have affected previously published data on the composition of the residues of atmospheric ice crystals.CitationPodzimek et al. (1994) used a forward-pointing inlet on the NCAR Sabreliner to sample ice crystals onto a coated impactor. Although their inlet is not fully described, they sampled ice crystals up to 100 μ m that most likely bounced off the inlet walls. Their results include a high frequency of mineral dust and fly ash, along with frequent Ba and Cl and a few submicron iron particles that were attributed to a possible extraterrestrial origin. The high frequency of mineral dust is consistent with later investigations. The high Ba and Cl contents are unusual and may reflect the occasional use of BaCl2 on their impactors for chemical detection of SO4 2−. In light of our work, we question whether the submicron Fe particles were indeed extraterrestrial or if the high nickel content of stainless steel was mistaken for extraterrestrial material. Their article does not present enough information on other elements, such as Mg, that would allow a more definitive conclusion about the extraterrestrial or inlet origin of their submicron Fe particles.
The residues from evaporation of ice crystals from cirrus clouds and aircraft contrails were studied using a CVI inlet on the Falcon research aircraft. About 75% of the residues from cirrus cloud samples contained Fe (CitationHeintzenberg et al. 1996). These were classified as mineral particles, and many also included other elements such as Al or Si that support a crustal source. But up to 50% of the particles contained Fe and no other elements supporting the mineral designation. Such Fe particles without Al or Si were common only in clouds. CitationPetzold et al. (1998) found that about 1% of the ice residue particles in contrails and 0.13% of those in cirrus clouds were stainless steel. An additional, unspecified, percentage were metals mixed with carbon. The stainless steel particles were larger (> 1.5 μ m) than other residues, so they contributed about 50% of the residual mass in contrails and up to 10% in cirrus clouds.
Another CVI inlet was flown on the DC8 aircraft by CitationTwohy and Gandrud (1998). They found that 12–25% of the ice residues from contrails were metals, usually either Fe/Cr or Ti. An additional 3–5% consisted of metals mixed with other species. Furthermore, particles containing only Fe were classified as mineral. Together, from 15% to possibly over 30% of the ice crystal residues had a high metal content. A typical size for the metal particles was 0.4 μ m. The Fe/Cr composition and the typical size indicate that many of these metal particles could be consistent with our results for metal particles generated in the inlet.
A mass budget for metallic particles in the upper troposphere can be used to estimate the plausibility of these estimates of high stainless steel content for ice residues. Of the published results, only those of CitationPetzold et al. (1998) are complete enough to estimate the mass concentration of metallic particles. They estimate an ambient concentration of about 2.4*10−5 cm−3 coarse stainless steel particles that serve as nuclei in cirrus clouds. Using a particle lifetime in the upper troposphere of 2 weeks, a source of over 20,000 tons per year would be required for the Northern Hemisphere. Mechanical wear from aircraft cannot be the source of so much stainless steel in the upper troposphere: this amount corresponds to on the order of a ton per plane per year. Nor could this much stainless steel come from the Earth's surface. First, it would not be plausible that stainless steel would be preferentially lifted into the upper troposphere compared to ordinary steel, aluminum, or other common metals. Second, the required flux would represent a fraction of a percent of the global production of stainless steel not only turned into fine particles but also lifted into the free troposphere. Even if the metal particles are smaller, as observed in our data and the data of CitationTwohy and Gandrud (1998), the mass budget implies that most of the observed stainless steel must have been an artifact. Cirrus clouds often have 0.01 to 1 cm−3 of ice crystals. For stainless steel to supply even 1–10% of these requires an implausible global flux.
One advantage for PALMS, not available to previous investigations, is the real-time measurement. This is important for correlating observations of metal particles with the cloud properties. As an example, during one flight segment off the coast of California about 1300 positive mass spectra were acquired in just over 15 min. Three of these were stainless steel, and correlation of the times at which they had been acquired with relative humidity data showed that all three had been acquired during a few seconds of flight through wisps of clouds. A 15 min impactor or filter sample during the same time interval might well have been considered as out of cloud and the connection of the stainless steel particles with the cloud might not have been suspected.
Although they also contain large amounts of Fe with some Ni and Cr, meteoric residues are easily distinguished from stainless steel by PALMS (). Our results on meteoric material and other elements in stratospheric and upper tropospheric aerosols have excluded data in obvious clouds and were therefore not significantly affected by this inlet contamination (CitationMurphy et al. 1998). We believe that, despite the high proportion of metal particles, the remaining particles are representative of ice residues (CitationCziczo et al. 2004). However, any real metal particles in cloudy regions of the atmosphere cannot currently be analyzed in the presence of so much contamination from the inlet.
Besides the studies of chemical composition mentioned above, the generation of metal particles in inlets may or may not affect other studies of cirrus or mixed phase clouds. One key question requiring additional study is if the metal particles are formed on a one-for-one basis with the loss of ice crystals to the walls. If not, counts of ice nuclei could be affected. Whether studies of ice crystal concentrations in clouds are affected depends on the type of inlet or lack thereof. High ice number concentrations have been measured by, for example, particle measuring systems (PMS) probes in the free stream (CitationRangno and Hobbs 1991). Such measurements should not be affected by contamination unless the generation of particles from nearby aircraft surfaces is extraordinarily efficient.
CONCLUSIONS
The impact of ice crystals, coarse-mode dust particles, and possibly even water droplets in a stainless steel inlet at 120–200 m s−1 generated submicron stainless steel particles from the walls of the inlet. Zn-rich particles were collected by an impactor sampler further back on the aircraft. Ice crystals can also resuspend solid particles previously deposited to the walls of an inlet from highly concentrated particle sources. These sources of contamination must be accounted for when measuring the composition of the residues of cirrus or contrail ice crystals. Previous experiments have also measured metal particles, albeit at frequencies less than we have (1–30% of the particles as opposed to our 90% with PALMS and lower but still high rates with the TEM). The reason for the difference in rates is not clear, although it may be due to design details such as surface finish or the high sensitivity of PALMS to metals. Even the lower percentages, however, are inconsistent with a mass budget for stainless steel in the upper troposphere.
There are no known design modifications to prevent large ice crystals from impacting the walls of a forward-facing aircraft inlet. A different inlet material, such as gold, would be more readily differentiated from the background atmosphere than steel. Gold also produced fewer particles in the laboratory, perhaps because the surface of gold is the metal rather than an oxide. In the long run, the resuspension of previously deposited particles may be the more difficult problem because some of the particles on the wall of an inlet will have come from atmospheric particles and hence will be indistinguishable as real or artifact. Frequent cleaning of the inner surfaces of inlets may be helpful.
Acknowledgments
The CAPS probe data used for were provided by Darrel Baumgardner and Bruce Gandrud. The coarse-mode concentrations in were provided by Chuck Brock. Financial support for the ASU portion of this work was provided through grant NAG5-11552 from the NASA Radiation Sciences Program.
REFERENCES
- Baumgardner , D. and Huebert , B. 1993 . The Airborne Aerosol Inlet Workshop: Meeting Report . J. Aerosol Sci. , 24 : 835 – 846 .
- Baumgardner , D. , Jonsson , H. , Dawson , W. , O'Connor , D. and Newton , R. 2002 . The Cloud, Aerosol and Precipitation Spectrometer (CAPS): A New Instrument for Cloud Investigations . Atmos. Res. , 59–60 : 251 – 264 .
- Belyaev , S. P. and Levin , L. M. 1974 . Techniques for Collection of Representative Aerosol Samples . Aerosol Sci. , 4 : 325 – 338 .
- Cziczo , D. J. , Murphy , D. M. , Thomson , D. S. and Ross , M. N. 2002 . Composition of Individual Particles in the Wakes of an Athena II Rocket and the Space Shuttle . Geophys. Res. Lett. , 29 ( 21 ) : 2037 DOI: 1029/2002GL015991
- Cziczo , D. J. , Murphy , D. M. , Hudson , P. K. and Thomson , D. S. 2004 . Single Particle Measurements of the Chemical Composition of Cirrus Ice Residue During CRYSTAL-FACE . J. Geophys. Res. , 109 : D4 DOI: 10.1029/2003JD004032
- Dahneke , B. 1995 . Particle Bounce or Capture-Search for an Adequate Theory: I. Convervation-of-Energy Model for a Simple Collision Process . Aerosol Sci. Technol. , 23 : 25 – 39 .
- Gardiner , B. A. and Hallett , J. 1985 . Degradation of in-Cloud Forward Scattering Spectrometer Probe Measurements in the Presence of Ice Particles . J. Atmos. Oceanic Technol. , 2 : 171 – 180 .
- Hangal , S. and Willeke , K. 1990 . Overall Efficiency of Tubular Inlets Sampling at 0–90 Degrees from Horizontal Aerosol Flows . Atmos. Environ. , 24A : 2379 – 2386 .
- Heintzenberg , J. , Okada , K. and Ström , J. 1996 . On the Composition of Non-Volatile Material in Upper Tropospheric Aerosols and Cirrus . Atmos. Res. , 41 : 81 – 88 .
- Huebert , B. J. , Lee , G. and Warren , W. L. 1990 . Airborne Aerosol Inlet Passing Efficiency Measurement . J. Geophys. Res. , 95 : 16369 – 16381 .
- John , W. 1995 . Particle-Surface Interactions: Charge Transfer, Energy Loss, Resuspension, and Deagglomeration . Aerosol Sci. Technol. , 23 : 2 – 24 .
- King , W. D. 1984 . Air Flow and Particle Trajectories Around Aircraft Fuselages. I: Theory . J. Atmos. Oceanic Technol. , 1 : 5 – 21 .
- Kojima , T. , Buseck , P. R. , Wilson , J. C. and Reeves , J. M. 2004 . Aerosol Particles from Tropical Convective Systems: 1. Cloud Tops and Cirrus Anvils . J. Geophys. Res. , in review
- Koop , T. , Kapilashrami , A. , Molina , L. T. and Molina , M. J. 2000 . Phase Transitions of Sea-Salt/Water Mixtures at Low Temperatures: Implications for Ozone Chemistry in the Polar Marine Boundary Layer . J. Geophys. Res. , 104 : 26393 – 26402 .
- Laucks , M. L. and Twohy , C. H. 1998 . Size-Dependent Collection Efficiency of an Airborne Counterflow Virtual Impactor . Aerosol Sci. Technol. , 28 : 40 – 61 .
- Lyman , T. , ed. 1961 . Metals Handbook, Vol. 1 , 8th ed. , American Society for Metals .
- McFarquhar , G. M. and Heymsfield , A. J. 1997 . Parameterization of Tropical Cirrus Ice Dize Distributions and Implications for Radiative Transfer: Results from CEPEX . J. Atmos. Sci. , 54 : 2187 – 2200 .
- Murphy , D. M. , Thomson , D. S. and Mahoney , M. J. 1998 . In situ Measurements of Organics, Meteoritic Material, Mercury, and Other Elements in Aerosols at 5 to 19 Kilometers . Science , 282 : 1664 – 1669 .
- Murphy , D. M. , Cziczo , D. J. , Hudson , P. K. , Schein , M. E. and Thomson , D. S. 2004 . Particle Density Inferred from Simultaneous Optical and Aerodynamic Diameters Sorted by Composition . J. Aerosol Sci. , 25 : 135 – 139 .
- Ogren , J. A. , Heintzenberg , J. and Charlson , R. J. 1985 . In situ Sampling of Clouds with a Droplet to Aerosol Converter . Geophys. Res. Lett. , 12 : 121 – 124 .
- Petzold , A. , Ström , J. , Ohlsson , S. and Schröder , F. P. 1998 . Elemental Composition and Morphology of Ice Crystal Residues in Cirrus Clouds and Contrails . Atmos. Res. , 49 : 21 – 34 .
- Podzimek , J. , Hagen , D. E. and Robb , E. 1994 . Large Aerosol Particles in Cirrus Type Clouds . Atmos. Res. , 38 : 263 – 282 .
- Prather , K. A. , Nordmeyer , T. and Salt , K. 1994 . Real-Time Characterization of Individual Aerosol Particles Using Time-of-Flight Mass Spectrometry . Anal. Chem. , 66 : 1403 – 1407 .
- Rader , J. and Marple , V. A. 1985 . Effect of Ultra-Stokesian Drag and Particle Interception on Impaction Characteristics . Aerosol Sci. Technol. , 4 : 414 – 156 .
- Rangno , A. L. and Hobbs , P. V. 1991 . Ice Particle Concentrations and Precipitation Development in Small Polar Maritime Cumuliform Clouds . Q. J. Royal Met. Soc. , 117 : 207 – 241 .
- Schreiner , J. , Schild , U. , Voigt , C. and Mauersberger , K. 1999 . Focusing of Aerosols into a Particle Beam at Pressures from 10 to 150 Torr . Aerosol Sci. Technol. , 31 : 373 – 382 .
- Sheridan , P. J. , Brock , C. A. and Wilson , J. C. 1994 . Aerosol-Particles in the Upper Troposphere and Lower Stratosphere—Elemental Composition and Morphology of Individual Particles in Northern Midlatitudes . Geophys. Res. Lett. , 21 : 2587 – 2590 .
- Sugi , N. , Arakawa , M. , Kouchi , A. and Maerno , N. 1998 . In-situ Mass Spectrometric Observation of Impact Vaporization of Water-Ice at Low Temperatures . Geophys. Res. Lett. , 25 : 837 – 840 .
- Tenberken-Potzsch , B. , Schwikowski , M. and Gaggeler , H. W. 2000 . A Method to Sample and Separate Ice Crystals and Supercooled Cloud Droplets in Mixed Phased Clouds for Subsequent Chemical Analysis . Atmos. Environ. , 34 : 3629 – 3633 .
- Twohy , C. H. and Gandrud , B. W. 1998 . Electron Microscope Analysis of Residual Particles from Aircraft Contrails . Geophys. Res. Lett. , 25 : 1359 – 1362 .
- Thomson , D. S. , Schein , M. E. and Murphy , D. M. 2000 . Particle Analysis by Laser Mass Spectrometry WB-57F Instrument Overview . Aerosol Sci. Technol. , 33 : 153 – 169 .
- Weber , R. J. , Clarke , A. D. , Litchy , M. , Li , J. , Kok , G. , Schillawski , R. D. and McMurry , P. H. 1998 . Spurious Aerosol Measurements when Sampling from Aircraft in the Vicinity of Clouds . J. Geophys. Res. , 103 : 28337 – 28346 .