Abstract
During an eight-day period in August of 1999, a nine-stage, micro-orifice, uniform-deposit impactor was used to determine the mass size distribution of atmospheric aerosol particles in the heavily polluted Metropolitan Area of São Paulo (MASP) in Brazil. Particle-induced X-ray emission analysis (PIXE) was used to identify up to 20 trace elements in particles collected in the five lower stages. All nine stages, as well as the after filter, were also analyzed for mass, black carbon, and various inorganic ions. A real-time carbon monitor provided organic and elemental carbon concentrations of PM 10 . For the five lower stages, the crustal material concentration was estimated by the sum of the main oxides and carbonates. In the after filter, PIXE analysis was not performed. The averaged mass balance analysis showed that black carbon was concentrated in the after filter, revealing black carbon particles to be very small in diameter. Ammonium sulfate showed simple unimodal size distribution during the day (peak diameter, 0.38 m) and bimodal size distribution at night (0.38 and 0.59 μ m). The crustal material contribution was greater in larger cutoff diameter stages. No explanation was found for a significant fraction of the fine particulate matter. The difference between the mass concentration and the sum of the component concentrations could be attributed to organic carbon being in fine mode. This could explain 38% of the difference and almost all of the missing diurnal and nocturnal mass. Based on these results and assumptions, we can conclude that the fine mode receives a greater contribution from organic and black carbon than from ammonium sulfate. The three together appear to constitute the bulk of fine particulate matter in the MASP.
INTRODUCTION
Atmospheric aerosols are comprised of a complex mixture of organic and inorganic substances and have an approximate size range of 0.01–10 μ m in diameter. These particles are of great concern because of their impact on public health. São Paulo is considered a megacity, with a population of 18 million inhabitants living on a plateau about 860 m above sea level and surrounded by hills of about 1600 m in elevation. In 1999, there were approximately 6.5 million vehicles circulating in the area. Roughly 5.5 million were cars, and the majority of these were fueled with gasohol, a mixture containing 78–80% (v/v) gasoline and 20–22% ethanol. There were also 1 million cars fueled with pure hydrated ethanol and approximately 500,000 trucks and buses that were diesel-powered. According to the Companhia de Tecnologia de Saneamento Ambiental (CETESB, São Paulo State Environmental Protection Agency) technical report for 1999 (CitationCETESB, 2000), the addition of ethanol to motor vehicle fuels reduces CO. However, the attendant increase in aldehyde emissions induces a unique photochemical smog phenomenon in urban areas. Vehicle emissions are largely responsible for the high concentrations of toxic pollutants in the local atmosphere; much more so than industrial emissions and natural sources.
Recent studies have shown that particles with a diameter less than 2.5 μ m, collectively known as fine particulate matter (FPM), are potentially harmful because they can penetrate the deepest recesses of the human lung. These fine particles may be produced by chemical transformations taking place in the atmosphere or emitted directly as particles. The majority of sulfate particles are produced by gas-to-particle conversion. Atmospheric transformation of emitted hydrocarbon gases may also result in production of organic carbon. However, the partition between primary and secondary organic aerosols remains uncertain. Various studies of inorganic FPM in the Metropolitan Area of São Paulo (MASP) have been performed (CitationMiranda et al. 2002; CitationCastanho and Artaxo 2001; CitationAndrade et al. 1994; CitationOrsini et al. 1986). Nonetheless, detailed data for organic compounds, which represent a large fraction of FPM, have yet to be collected. During the winter, when dispersion is greater, total FPM consists of 20–30% sulfates, 10–15% crustal elements (Al, Si, Ca, and Fe), 10–20% black carbon, and 40–60% organic carbon. Some questions remain unanswered. For example, the primary and secondary organic PM fractions have not been identified. In addition, although it is well known that secondary organic particles resulting from anthropogenic precursors contribute to the PM load, the importance of this contribution has not been determined.
This article presents size-distribution and size-resolved chemical mass balances for the fine portion of the atmospheric aerosol collected diurnally and nocturnally in the MASP from 3–11 August 1999. The particulate matter was collected with a nine-stage micro-orifice, uniform-deposit impactor (MOUDI) following protocols devised by CitationMarple et al. (1986). The nominal cutoff diameter (d50) of the nine stages ranged from 18 to 0.094 μ m, and the afterfilter collected those aerosols that had a d50 of less than 0.094 μ m. All stages, including the afterfilter, were analyzed for mass, black carbon, and the inorganic ions Na+, NH4 +, K+, Mg2 +, Ca+, Cl−, NO3 −, and SO4 2 −. The five lower stages were also analyzed for 20 trace elements using particle-induced X-ray emission analysis (PIXE). The total carbonaceous component (organic carbon plus elemental carbon) for PM10 was measured by an ambient carbon particulate monitor. For chemical mass balance, only the five lower stages and the afterfilter were considered.
EXPERIMENTAL DESIGN
An intensive aerosol sampling campaign was carried out over 9 days, from 3–11 August 1999. The sampling site was on the University of São Paulo campus (22°34′S, 46°44′W, IAG-CUASO; ), located about 10 km from downtown São Paulo. Meteorological observations were made at another USP campus (IAG-AF) located 20 km from the sampling site, in the southeastern part of the city.
FIG. 1 Metropolitan Area of São Paulo (MASP), aerosol sampling site (IAG-CUASO) and climatological station (IAG-AF).
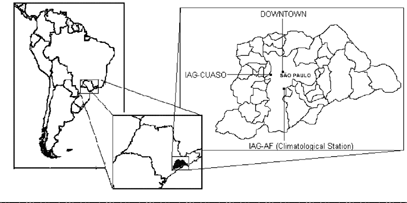
Mass concentrations of particles with a diameter less than x micrometers (PMx) were sampled using the MOUDI. The value of x was determined by the 50% d50 of the various impactor stages: 18 μ m (inlet), 9.9 μ m, 6.2 μ m, 3.1 μ m, 1.8 μ m, 1.0 μ m, 0.587 μ m, 0.384 μ m, and 0.094 μ m, respectively. Smaller particles were collected with the afterfilter. The MOUDI has rotating impaction plates and provides nearly uniform deposits over circular impaction areas. Nucleopore filters with a pore-size of 8 μ m were used for the nine stages, and a 1 μm pore-size Teflon-backed filter with a diameter of 37 mm was used at the afterfilter. During this campaign, the MOUDI operated with an integration time of 10 h during the day (from 8 a.m. to 6 p.m., diurnal samplings) and 14 h at night and early morning (from 6 p.m. to 8 a.m., nocturnal samplings) on weekdays. Over the weekend, the MOUDI integration time was 24 h, from 8 p.m. to 8 a.m. ().
TABLE 1 MOUDI sampling description and total concentrations (in μ g · m− 3) obtained by the summing of all MOUDI stages and the afterfilter
Mass concentrations were obtained gravimetrically using an electronic microscale with a sensitivity of 1 μ g. Assessments were performed in a controlled-atmosphere environment (20°C and 50% relative humidity, with fluctuations of less than ± 10% in these set points). Filters were equilibrated for 24 h prior to weighing. Electrostatic charges were controlled using radioactive Po210 sources. The concentration of black carbon was determined using a reflectance technique (CitationReid et al. 1998). On the University of São Paulo campus, the Laboratory of Analysis of Materials by Ionic Beam (LAMFI) houses the 1.7 MV tandem Pelletron accelerator described by CitationTabacniks et al. (1987). One quarter of each Nucleopore filter was submitted to PIXE analysis in order to determine elemental composition. The PIXE analysis was performed according to the technique described by CitationCampbell (1995). As many as 20 elements (Al, Si, P, S, Cl, K, Ca, Ti, V, Cr, Mn, Fe, Ni, Cu, Zn, Br, Rb, Sn, Zr, and Pb) were measured. The typical accuracy of the elemental concentration assessment is 4–8%. The five lower stages of the MOUDI were also submitted to PIXE analysis. A high-performance DIONEX-500 ion chromatograph, equipped with an ED-40 electrochemical detector, was used to determine concentrations of the inorganic ions Na+, NH4 +, K+, Mg2 +, Ca+, Cl−, NO3 −, and SO4 2 in the remaining three–quarters of each Nucleopore filter, as well as in each complete Teflon filter sample.
Organic and total carbon concentrations in the PM10 fraction were measured with a time resolution of 1 h by a direct automatic thermal-CO2 technique, performed with an ambient carbon particulate monitor (series 5400 from Rupprecht & Patashnick, Inc.) During analysis, the collected sample is first heated to 340°C and then to 750°C, in order to determine concentrations of organic and total carbon, respectively. Total elemental carbon is defined as the difference between total carbon and organic carbon.
RESULTS AND DISCUSSION
Meteorological Conditions
Meteorological observations made at the Institute of Astronomy, Geophysics and Atmospheric Sciences climatological station for this period are shown in . On 1 August 1999, a cold front passed through the state of São Paulo, and the intensive campaign began under postfront conditions.
shows that, during the first week of August, minimum temperatures were between 10 and 12°C, and maximum temperatures rose slowly, attaining the highest value of 28.3°C on 7 August. Minimum relative humidity was approximately 50% on the first days, when greater amounts of cloudiness were also registered (), and decreased on the 5th and 7th. The slight rise in temperature and drop in relative humidity during the night, usually around midnight, were attributed to the formation of dew, which releases heat as the vapor condenses, warming and drying the atmosphere. Average wind velocity during the first week was 2 m.s− 1, with prevailing winds from the ENE/NE ().
A sharp drop in pressure on the 7th (Saturday; ) and simultaneous observations of cirrus clouds from 1 p.m. signaled the arrival of a new cold front. Wind gusts of 8 m.s− 1 at 3 p.m. were also registered. There was no precipitation, although the cold front increased cloud cover. Maximum temperature on the 8th (Sunday; ) was less than 15°C.
The second week began with cloud cover caused by SE winds, which carried humidity from the ocean. From the 10th to the 13th a high-pressure system dominated the MASP. This system inhibited cloud formation () and was also responsible for long periods of light or no winds (), maximum temperatures reached almost 29°C, and relative humidity was as low as 20% (). In terms of air pollution dispersion, these characteristics constituted an extremely unfavorable period, which affected the MOUDI data.
Size Distribution of Mass and Black Carbon
shows the sampling description and the total concentration obtained by summing all MOUDI stages, including the afterfilter. As described in the meteorological conditions section, the effects of the various meteorological systems on mass concentration can be seen clearly. The first and second weeks were dominated by a high-pressure system. However, wind speeds were higher in the first week than in the second week, inducing higher aerosol concentrations in the second week. The effects of the cold front and subsequent clear cold air mass are depicted in the lowest concentration obtained by MOUDI 09.
In the MASP, PM10 concentrations consistently exceed the 24 h National Ambient Air Quality Standard (NAAQS) for PM10 of 150 μ g m− 3 during the last 20 years at several monitoring stations around the urban area. Episodes are more frequent during the winter season, mainly in August, when subsidence and thermal inversion layers provide unfavorable conditions for air pollution dispersion. Within this 9-day sampling period, 5 of the 13 monitoring stations registered PM10 concentrations above the PM10 NAAQS on 11 and 12 August. This is in good agreement with high concentrations measured by MOUDIs 13 to 15.
FPM concentrations were obtained by the summing of stages having a d50 of less than 2.5 μ m, and coarse particulate matter (CPM) concentrations were obtained by the summing of stages having a d50 between 2.5 and 10 μ m. Temporal variations are shown in . shows black carbon concentrations in these two modes.
FIG. 5 FPM and CPM calculated by the summing of the mass concentration (above) and black carbon concentration (below) in stages with a d50 of less than 2.5 μ m (considered FPM) and in those with a d50 between 2.5 and 10 μ m (CPM) for nocturnal (N), diurnal (D), and 24 h sampling.
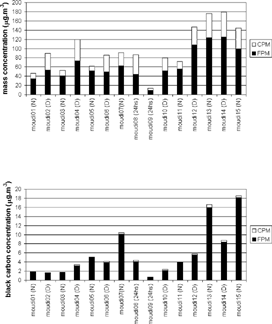
It can be seen that, during the first week (samples MOUDI1 to MOUDI7), CPM and FPM concentrations, as well as the CPM to FPM ratio (CPM/FPM), were higher during the day than at night. Higher concentrations of CPM during the daytime may be attributable to greater thermal turbulence that resuspends more soil dust. During calm nights, higher relative humidity and dew formation helped to prevent resuspension of soil dust, decreasing CPM concentrations. There were also local forcings. Traffic was more intense during the day than at night. This could explain the higher daytime concentrations of FPM associated with photochemical production of secondary aerosols. The weekend sampling (MOUDIs 8 and 9) showed that FPM and CPM contributed almost equally to PM10 concentration.
The second week began with a pattern very similar to that of the first week (MOUDIs 10 and 11); however, the CPM/FPM decreased in subsequent samplings. The FPM increase was probably caused by a higher photochemical reaction rate (higher maximum temperatures, lower relative humidities, and fewer clouds than in the previous week), and the lower CPM was probably due to lower winds.
shows that typically more than 90% of the black carbon was concentrated in the FPM. Nocturnal values were higher than previous diurnal values. Diesel and vehicle emissions are the main source of black carbon in São Paulo. Emissions are higher during the morning (8–10 a.m.) and early evening (6–8 p.m.) traffic hours. However, concentration values represent emissions plus the planetary boundary layer (PBL) evolution effect. Solar heating supplies energy for the development of a thermally unstable mixing boundary layer that reaches its maximum height during the afternoon. As solar heating ceases, a stable nocturnal boundary layer develops and is limited by a low-temperature inversion. Diurnal emissions were homogeneously distributed in a higher boundary layer, resulting in lower concentrations, whereas nocturnal emissions were trapped in a lower stable layer, resulting in higher concentrations.
Since the weekend samplings were very different from the other samplings, they were not included in subsequent analyses. The lowest concentration measured on Sunday (MOUDI09) was mainly caused by the entrance of a clean air mass rather than lower emissions on weekends.
The average concentrations of mass and black carbon as a function of particle size can be seen in . Diurnal total mass concentrations were approximately 1.3 times higher than nocturnal concentrations. Nighttime total black-carbon concentrations were nearly double diurnal concentrations. In mass concentration, fine particles were present in 65% of the aerosol collected during daytime, increasing to 72% at night. More than 90% of black-carbon particles found were in the fine mode and therefore concentrated in the after filter, 62% during the day and 76% at night.
TABLE 2 Daytime and nighttime mass and black-carbon average concentrations (in μ g · m− 3) as a function of particle size
The size distribution for mass concentration and black-carbon concentration are shown in , respectively, averaged for daytime periods (MOUDIs 2, 4, 6, 10, 12, and 14) and for nighttime periods (MOUDIs 1, 3, 5, 7, 11, 13, and 15). shows that there were three modes of mass size distribution: nucleation mode (d50 0.09 μ m), accumulation mode (d50 = 0.38 μ m), and coarse mode (d50 = 6.2 μ m). At night, the nucleation mode was more pronounced, whereas the other two modes were more prominent during the day. In , the black-carbon nucleation mode is shown for both periods and is obviously more pronounced at night. The accumulation mode appeared as a secondary peak of approximately 0.59 μ m. This indicated that black carbon is primarily emitted as minute particles.
Size Distribution of Inorganic Ions
Some species like Ca, K, and S (SO4 2 −) were analyzed by both PIXE and IC. The highest correlation coefficient (r2) was obtained between sulfate measured by IC and S by PIXE (0.77). Even supposing that all S measured by PIXE were sulfate (that is, sulfate would be estimated by the sulfur concentration obtained by PIXE analysis assuming a conversion factor of 3) there would be S excess. This could be an indication of nonionic sulfur compounds in the urban aerosol and therefore not measured by IC. However, K (r2 = 0.51) and Ca (r2 = 0.41) measured by IC had higher concentrations than those measured by PIXE. These differences may be due to analytical and experimental problems. Since concentrations measured at the After Filter are relevant for a fine particulate matter study, IC analysis was chosen whenever available.
The average concentrations of the inorganic cations and anions as a function of particle size obtained by IC can be seen in , respectively. The results for day and night samplings are distinguished. The two tables also contain average total-ion concentrations. The dominant ions were, as expected, ammonium, sulfate, and nitrate. Unexpectedly, calcium ion was also dominant. Total concentrations of these ions were all comparable. However, particle size distributions differed between diurnal and nocturnal samplings. Sodium, potassium, magnesium, and particularly chloride had total concentrations up to 2.6 times higher in nighttime than in daytime samplings.
TABLE 3 Daytime and nighttime mass concentrations (in μ g · m− 3) of inorganic cations as a function of particle size
TABLE 4 Daytime and nighttime mass concentrations (in μ g · m− 3) of inorganic anions as a function of particle size
The most significant difference observed in the ammonium size distribution was in the coarse mode, in which diurnal concentration was 5 times higher than nocturnal concentration. For particles 10 μ m in diameter, almost 9 times more ammonium was measured during the day than at night. In contrast, particles less than 1 μ m in diameter presented the greatest difference in the 0.384 μ m stage, in which the diurnal value was 1.2 times higher than the nocturnal value. Nocturnal sulfate concentrations for particles with a diameter of less than 1 μ m were higher than diurnal concentrations. However, for larger particles, nocturnal concentrations were lower than diurnal concentrations. This analysis suggests that, during the day, the higher ratios of small sulfate particles resulted from photochemical oxidation of SO2, whereas at night the process of aqueous oxidation of SO2 resulted in the predominance of larger particles. As can be seen by the size distributions shown in , respectively, ammonium and sulfate were typically correlated. Most ammonium and sulfate ions were found in the fine-particle size range. During the day, 90% of each ion was in the fine mode. At night, this fraction increased to 97%. During the day, the size distribution of both ions was bimodal, with peaks at 0.38 μ m and 6.2 μ m. At night, however, there was a sharp increase in 0.59 μ m-stage sulfate concentration, and the diurnal fine mode peak broadened. On average, the field experiment period was characterized by an ammonium-rich atmosphere, and all the sulfate was neutralized by ammonium.
Diurnal nitrate concentrations were higher than nocturnal concentrations. The greatest difference was found in the 0.09 μ m stage, in which diurnal concentration was 3 times higher than nocturnal concentration. The nitrate size distribution depicted in shows the nucleation mode (with a peak at the afterfilter), the coagulation mode (at 0.38 μ m), and the coarse mode (with a peak at 3.1 μ m). For particles with a diameter greater than 0.59 μ m, concentrations increased in parallel with increasing d50. During the day, 55% of nitrate ions were in the fine mode, whereas at night this decreased to 47%.
Total nocturnal sodium concentrations were about 1.7 times higher than diurnal concentrations. The greatest differences were found in the two lower stages (d50 of 0.09 and 0.38 μ m), being, respectively, 11 and 13 times higher than diurnal concentrations. reveals that the sodium fine mode had two peaks (afterfilter and 1 μ m) and that its coarse mode presented a peak at 6.2 μ m. During the day, 82% of sodium ions were in the fine mode, whereas at night this decreased to 72%.
Total nocturnal concentrations of chloride and potassium were, respectively, 2.6 and nearly 2.0 times higher than diurnal concentrations. The similarities in potassium and chloride size distributions seen in suggest an association between the two, thereby supporting the findings of CitationMiranda et al. (2002). Chloride and potassium particulate matter may originate from biomass- or refuse-burning processes.
Diurnal calcium and magnesium concentrations were found to be mainly in the coarse mode (73 and 56%, respectively), indicating their crustal origin. However, in nocturnal samplings, their participation in the fine mode increased. For calcium, the greatest difference was found in the 0.38 μ m stage and, for magnesium, the greatest difference was found in the afterfilter. Nocturnal concentrations in these stages were 10 times higher than diurnal concentrations.
The sum of the mass of cations and anions was calculated in equivalents. The results for diurnal and nocturnal samples are summarized in . Deviation between cation and anion equivalents is expressed in percentage of cation excess. Positive values indicate cation excess, negative values denote cation deficiency.
TABLE 5 Daytime and nighttime concentrations (in nequ · m− 3) of cations and anions as a function of particle size
In both diurnal and nocturnal samplings, high cation excess was observed. The cation excess was probably due to the lack of anions uninvolved in the ion balance. Those anions, such as hydroxide and carbonates (in the coarse mode), as well as, organic acids (in the fine mode) specifically, were not determined.
Size-Resolved Mass Balance
Size-resolved mass balance analysis was performed for the five lower MOUDI stages and the after filter. presents the size-resolved mass balance in terms of percentage.
FIG. 13 Daytime (above) and nighttime (below) averaged size-resolved mass balance for the five lower stages of the MOUDI and the afterfilter.
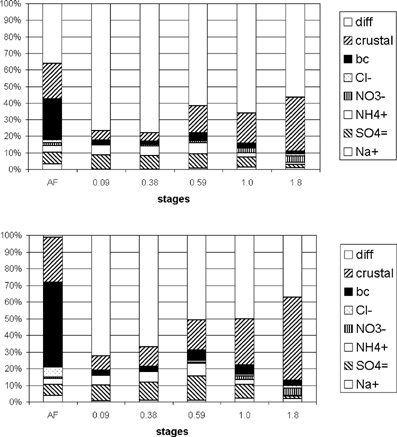
Crustal material concentrations were estimated by summing the main oxides and carbonates Al2O3, SiO2, CaCO3, K2CO3, Fe2O3, and ZnO, calculated as PIXE values Al * 1.89, Si * 2.14, Ti * 1.67, Fe * 1.43, and Zn * 1.25 (), and as ion chromatography values Ca * 2.5 and K * 3.31. Since PIXE analysis was performed only for the five lower stages, the afterfilter mass balance was calculated by considering only black-carbon concentrations and ion concentrations obtained through ion chromatography.
TABLE 6 Oxide concentrations estimated as a factor multiplied by PIXE-analyzed elemental concentration
The elemental concentration size distribution of Si, Al, Ti, and Fe shows that these elements were typically found in the CPM, since concentration increased with diameter. The concentrations of these elements in the lower stage (d50 = 0.094 μ m) were very low. Therefore, their contribution to the afterfilter mass balance can be disregarded. The elemental concentrations of Ti, Al, and Fe were very similar in both diurnal and nocturnal samplings, presenting higher concentrations in diurnal samples than in nocturnal samples. The zinc size distribution had a peak at a d50 of 1 μ m with approximately equal diurnal and nocturnal total concentrations. The fine mode zinc was probably generated by industrial combustion sources such as the burning of refuse and oil. The crustal material, estimated in the same manner as the oxides of the elements listed above, presented unimodal size distribution, with, as expected, a peak in the 1.8 μ m stage (the largest analyzed). Adding the estimated calcium carbonates to the crustal material modified this size distribution, with a slight increase in the diurnal 0.094 μ m stage.
The fraction of unresolved material concentration was calculated as the difference between the gravimetric concentration and the sum of inorganic ions (sulfate, ammonium, nitrate, and chloride), crustal material, and black carbon. The difference was greater during the day than at night. With the exception of the after filter, the difference was greater for smaller particles than for larger ones. According to CitationCastanho and Artaxo (2001), 40% of the FPM in winter consists of organic carbon. A carbon monitor was used to measure PM10 organic carbon, but its size distribution was not determined. Organic carbon aerosol is emitted directly in vehicle exhaust and is also formed by photochemical reactions in the atmosphere. Assuming that all the organic particles were in the fine mode, the organic carbon fraction of the total FPM mass would be 25% during the day and 43% at night. The remaining difference would decrease from 65% to 40% for diurnal samplings, and the organic portion would explain all the difference at night.
Temporal evolution of various compounds (inorganic ions, crustal material, and black carbon) in the fine particulate mode is shown in . All organic carbon was assumed to be in the fine mode. During the first week, nocturnal sulfate concentrations in the fine mode were slightly higher than diurnal concentrations, suggesting that there may be oxidation of SO2 in aqueous phase at night, when relative humidities are higher. From MOUDI1 to MOUDI6, diurnal organic carbon concentrations were higher than nocturnal concentrations, indicating photochemical reactions producing secondary organic carbon. During the second week, however, sulfate concentrations decreased with time, from 8.7 μ g.m− 3 at MOUDI10 to 1.4 μ g.m− 3 at MOUDI15. As shown in , minimum relative humidities were as low as 20%. This may have caused less aqueous oxidation of SO2 to sulfate. Secondary organic carbon production was higher than during the first week, and peak values observed at nighttime integration period may be due to very stable atmospheric conditions. Unresolved matter was high for MOUDIs 2, 12, and 14, all of them sampled during daytime; it was negative for MOUDIs 9 and 15.
FIG. 14 Temporal evolution of FPM compounds (inorganic ions, crustal material, and black carbon) calculated by the summing of the concentration in stages with d50 less than 2.5 μ m and total organic carbon measured by the carbon monitor.
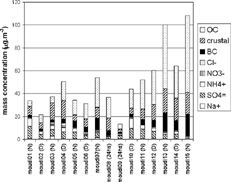
The campus is surrounded by important highways with intensive vehicle emissions. Automobile traffic and soil dust resuspension are the main air pollution sources in the Metropolitan Area of São Paulo; their contribution is so large that although there are some stationary sources near the campus (a small cement plant at northwest and a garbage incinerator at northeast) their influence could not be noticed in this analysis of 15 samples. According to a three-month study (CitationCastanho and Artaxo 2001), industrial emissions contributed with only 5% of PM2.5 during the winter season.
CONCLUSIONS
The particulate matter collected with a MOUDI impactor in the MASP was analyzed. Higher daytime CPM/FPM ratio was attributed to soil dust resuspension by both thermal turbulence and vehicle traffic. This process was inhibited at nighttime because of higher humidity and more stable atmosphere. Higher daytime concentrations of FPM were also observed and were probably generated by photochemical production of secondary aerosols. Black-carbon particles were concentrated in the afterfilter, indicating that emissions of particles with diameters less than 0.09 μ m were dominant. Nocturnal concentrations were higher than diurnal concentrations, resulting from a combined effect of vehicle emission and PBL evolution. As expected, the dominant ions were ammonium, sulfate, and nitrate. Calcium appearing as a dominant ion was an unexpected finding. The highest concentration of both ammonium and sulfate were found in the 0.38 μ m stage. Nitrate had higher concentrations in the coarse mode, peaking at 3.1 μ m. Sodium, chloride, and potassium had higher FPM concentrations, whereas calcium and magnesium had higher CPM concentrations. The high cation excess observed was probably due to the lack of hydroxide, carbonates, and organic acids, which were not evaluated in this experiment. Crustal material had higher concentrations at higher d50s. The main crustal elements were aluminum, silicium, titanium, and iron. For the five lower stages and for the afterfilter, the fraction of unresolved material in the size mass balance was substantial. If we assume that all the organic carbon determined for PM10 by the carbon monitor was in the fine mode, it would explain 38% of the unresolved diurnal mass and almost all the nocturnal difference. Based upon these results, it can be assumed that the fine mode receives a greater contribution from carbonaceous material (organic carbon + black carbon) than from ammonium sulfate. Nonetheless, our results show that the combination of organic carbon, black carbon, and ammonium sulfate constitutes the greater part of the fine particulate matter in the atmosphere over the city of São Paulo.
Acknowledgments
We thank the LAMFI staff for assistance during PIXE analysis, the GEPA staff for assistance during gravimetric and reflectance analysis, Rosana Astolfo for ion chromatograph analysis, and the IAG-AF staff for meteorological data. This work was financed by FAPESP (Fundação de Amparo à Pesquisa do Estado de São Paulo) through grant number 99/08211-1 and by the Meteorology and Air Pollution in the São Paulo Metropolitan Area Thematic Project.
REFERENCES
- Andrade , M. F. , Orsini , C. and Maenhaut , W. 1994 . Relation Between Aerosol Sources and Meteorological Parameters for Inhalable Atmospheric Particles in São Paulo City, Brazil . Atmos. Environ. , 28 : 2307 – 2315 .
- Campbell , J. L. 1995 . “ Instrumentation, Fundamentals, and Quantification ” . In Particle Induced X-ray Emission Spectrometry , Edited by: Johansson , S. E. , Campbell , J. L. and Malmqvist , K. G. pp. 19 – 99 . New York : Wiley .
- Castanho , A. D. A. and Artaxo , P. 2001 . Wintertime and Summertime São Paulo Aerosol Source Apportionment Study . Atmos. Environ. , 35 : 4889 – 4902 .
- CETESB . 2000 . Relatório de qualidade do ar de 1999 Report CETESB, São Paulo, in Portuguese. Available at
- Marple , V. A. , Kenneth , L. R. and Steven , M. B. 1986 . A Micro-orifice Uniform Deposit Impactor (MOUDI): Description, Calibration and Use . J. Aerosol Sci. , 17 : 489 – 494 .
- Miranda , R. M. , Andrade , M. F. , Worobiec , A. and Van Grieken , R. 2002 . Characterisation of Aerosol Particles in the São Paulo Metropolitan Area . Atmos. Environ. , 36 : 345 – 352 .
- Orsini , C. , Tabacniks , M. , Artaxo , P. , Andrade , M. F. and Kerr , A. S. 1986 . Characteristics of Fine and Coarse Particles of Natural and Urban Aerosols of Brazil . Atmos. Environ. , 20 : 2259 – 2269 .
- Reid , J. S. , Hobbs , P. V. , Liousse , C. , Martins , J. V. , Weiss , R. E. and Eck , T. F. 1998 . Comparison of Techniques for Measuring Short-Wave Absorption and Black Carbon Content of Aerosol from Biomass Burning in Brazil . J. Geophys. Res. , 103 : 32031 – 32040 .
- Tabacniks , M. , Osrini , C. and Artaxo , P. 1987 . PIXE Analysis for Air Pollution Source Apportionment in Urban Areas of Brazil . Nuclear Inst. Methods Phys. Res. , B22 : 315 – 318 .