Particulate emission indices (per kg fuel) have been determined by sampling the advected plumes of in-use commercial aircraft at two different airports using a novel approach. Differences are observed in the number, magnitude, and composition of the particle emissions between idle and take-off. At the first airport, Electrical Low Pressure Impactor (ELPI) data indicate that number based emission indices (EI n ) vary by an order of magnitude for take-off plumes from different aircraft. Additionally, EI n values for idle plumes are greater than take-off. At the second airport, EI n values derived from condensation particle counter (CPC) measurements span ∼ an order of magnitude (3–50 × 10 15 particles per kg fuel). The median values of the idle and take-off plumes were 1.8 × 10 16 and 7.6 × 10 15 particles per kg fuel, respectively. For take-off plumes, the magnitude of the particulate emission index is not correlated with NO x at either airport. The surface properties of the particulate emissions in take-off and idle plumes differ significantly as measured by diffusion charging (DC) and photoelectric aerosol sensor (PAS) instruments. Results indicate that take-off plumes are characterized by particles with photoelectric-active surfaces, presumably elemental carbon, whereas idle plumes are composed of non-photoelectric-active constituents and coated soot particles. Measurements of the particulate size distribution (ELPI) show evidence for two modes, one at ∼ 90 nm aerodynamic diameter and a second mode at or below the instrument cutoff ( < 30 nm).
INTRODUCTION
Particles play an important role in several atmospheric phenomena, including global climate change, local visibility, and impacts on human health. Commercial airports, where particulate emissions are generated by aircraft, ground service vehicles, private and commercial vehicles, and the power plants needed for terminal operations, are potentially important and growing sources of urban particulate matter. Characterization and quantification of direct particle emissions from various aviation-related sources has remained elusive (CitationWey 1998; CitationSchäfer et al. 2000; CitationWhitefield 2002). To address these issues, several active research programs (CitationWey 2004) are concentrating on understanding particle emissions from airports and their potential impacts on local and regional air quality (CitationIPCC 1999; CitationEEA 2004).
The modern gas turbine engine is challenged with the most diverse operating conditions at an airport. The operational conditions referred to as “ground idle” and “take-off” are extremes, each with differing demands relative to the nominal “cruise” operation at altitude. Cruise is most commonly achieved in the middle and upper troposphere. The engine design optimization criteria are weighted to produce the best overall economy and performance at cruise, where the bulk of the fuel on a given flight is expended. A significant effort over the last few decades (CitationIPCC 1999) has been spent studying subsonic and supersonic aircraft emissions at altitude to understand their impacts on upper troposphere/lower stratosphere ozone chemistry, contrail formation, and radiative properties of the atmosphere (e.g., soot absorption of incoming sunlight). In contrast, the emissions of in-use aircraft operating on the ground at airports under idle, take-off, and landing conditions differ from emissions at cruise and have been implicated in local and regional air quality issues (Yu et al. 2004). It is not clear, however that airport emissions, specifically aircraft particulate emissions, are well characterized (CitationPison et al. 2004).
At an airport, emissions from aircraft idling (at the gate and awaiting take-off), taxiing, taking-off, and landing represent a significant source of particles. The gas-phase composition of the emissions resulting from the different turbine engine operational modes can be very different (CitationSpicer et al. 1994). The major factors that control the total particulate burden the airport air-shed receives from a single aircraft are the type and mechanical history of the engines and the time spent at each engine state (related to airport schedule and the number of passengers and flights at any given airport). These factors can be modeled using the fuel-flow rate, an experimentally determined particulate emission index representative of a particular engine state, and the time spent in that state performing each activity. The LTO cycle established by the International Civil Aviation Organization (CitationICAO 1995). This organization represents a standard aircraft operational cycle for determining gaseous and particulate emission profiles. A significant fraction of published work on aircraft exhaust emissions has been focused on measurements conducted at the exit plane of engine combustors operated under well controlled cycles (e.g., PartEmis [CitationGysel et al. 2003; CitationPetzold et al. 2003]). Actual aircraft operations at an airport may vary greatly from a standard cycle depending on the pilots' techniques, flight traffic, and airport layout, for example. Furthermore, local ambient conditions may affect particle formation, coagulation, and growth down wind of the engine exit plane. Thus, it is important to characterize the particulate emission indices over all of the operational states in use at an airport to assess the full impact of airport activity on local and regional air quality.
The present study applies a new approach to aircraft particulate exhaust emission measurements at an airport, extractive sampling of wind advected plumes from in-use commercial aircraft, which represents an in situ approach to airport pollution characterization. Specifically, we report on particulate emissions measurements for in-service commercial aircraft at two major airports, John F. Kennedy airport in New York City (2001) and Logan airport in Boston (2003). Although the instrumentation packages deployed at these two airports were different, the sampling methods were similar. Exhaust plumes from individual airplanes were measured during idle, taxi, landing (or approach), take-off and engine-start conditions. The data were collected in a noninterfering fashion and with the cooperation of airport operations. All instruments were installed in a mobile laboratory located down wind of an active runway. The instruments were operated continuously, sampling aircraft plumes as they advected past the mobile laboratory. The exhaust plumes were naturally diluted by ambient air and sampled within two minutes after crossing the exit plane of the engines. The technique of rapidly sampling wind advected aircraft plumes is more completely described elsewhere (CitationHerndon et al. 2004). In addition to enabling fast characterization of emissions from aircraft fleets, this measurement technique provides critical insight into “real world” emission profiles for in-use jet engines, despite the large degree of dilution and the inability to control/measure engine states. This measurement scheme provides emission indices for aircraft exhaust that includes dilution and condensation effects under ambient conditions. This is important since an assessment of the local and regional impact of airport particulate emissions depends not only on particles that are directly emitted in the exhaust, but also on subsequent condensation of low volatility gas phase exhaust species, either to form new, nanometer sized aerosols or to coat the surfaces of existing particles, thereby changing their microphysical properties.
The findings presented here are based on measurements of approximately 100 aircraft exhaust plumes obtained during the two different measurement campaigns. During the sampling at JFK, 31 take-off plumes and 24 idle plumes were analyzed and in the Logan work, 14 take-off and 13 idle plumes as well as 16 additional taxiway acceleration and approach plumes. This work will primarily focus on measurements of intercepted plumes that correspond to aircraft under idle and take-off conditions. In particular, we report the first application of several instruments that are commonly used in the field of diesel exhaust characterization to the study of in-use aircraft emissions. Together these measurements provide an important perspective on particle number emission indices (EI), number-weighted particle size distributions, and particle composition downwind in aircraft exhaust plumes. The questions arising from this analysis will guide future studies seeking to gain a more complete understanding of the nature of particulate emissions from aircraft.
EXPERIMENTAL DESCRIPTION
This work is based on a technique that positions the instrumentation aboard a mobile platform downwind from operational aircraft (CitationHerndon et al. 2004). The Aerodyne Mobile Laboratory (CitationKolb et al. 2004) utilizes a common sampling inlet for all gaseous and particulate instruments. The inlet is located on the front left side of the vehicle, ∼ 2.4 m above the road. Ambient air is drawn through the inlet at 20 lpm and isokinetically split inside the mobile laboratory to provide individual flows to the various instruments. This paper focuses on the particulate measurements made during two different studies of aircraft emissions that were conducted two years apart. Neither airport experienced a disruption to normal operating conditions as a result of this work.
All of the particle instruments described here have been utilized for diesel soot characterization studies. Here we report the first application of some of these particle instruments to aircraft emissions measurements. In addition to the particulate instrumentation during both studies, a Licor model 6262 near infrared absorption instrument was used to measure the carbon dioxide concentrations. NO and NO2 were measured at JFK Airport using Tunable Infrared Laser Differential Absorption Spectroscopy (TILDAS) and the results have been published (CitationHerndon et al. 2004). At Boston Logan Airport, NO2 was measured by TILDAS, and total NOy was measured using a chemiluminescence instrument (CitationDunlea 2005). These gaseous measurements are used here for their insight into aircraft engine operational state, however a detailed discussion of these results will be given elsewhere (CitationHerndon et al. 2005).
The first study was carried out at the John F. Kennedy airport in New York as part of the PM 2.5 Technology Assessment and Characterization Study, PMTACS-NY (CitationDemerjian 2000, Citation2001, Citation2002, Citation2003, Citation2004) in August 2001. A detailed discussion of the gas phase emissions, wind speed, sampling geometry and diluted, advected plume analysis for the John F. Kennedy airport study has been given previously (CitationHerndon et al. 2004). The particle data collected during this study were obtained with an electrical low-pressure impactor (ELPI). The second study presented in this paper was performed at Logan International Airport in Boston during May 2003. The Logan study was accomplished with the cooperation of the airport staff. In a similar manner as that described for the previous JFK study, the mobile laboratory was positioned within 200 m of an active taxiway and runway. The mobile laboratory was moved several times to sample different activities according to the prevailing wind. Several different types of plumes were sampled, including approach (landing) and engine start-up in addition to idle, taxi, and take-off. Similar to the JFK study, these measurements spanned about three hours. The particle instrumentation used during the Logan study consisted of a condensation particle counter (CPC), a diffusion charging (DC) active surface monitor, and a photoelectric aerosol sensor (PAS). Brief descriptions of the various particle measurement methods are provided below.
JFK 2001—Electrical Low Pressure Impactor
Particle size distributions were measured using an Electrical Low Pressure Impactor (ELPI, Dekati, Ltd., Finland). The ELPI measures particle aerodynamic diameters using twelve stages, characterized by a midpoint diameter, over the size range 30–10,000 nm (CitationKeskinen et al. 1992). The aerodynamic diameter is related to geometric (and mobility) diameters through a particle's material density and dynamic shape factor (CitationDeCarlo et al. 2004; CitationSlowik et al. 2004). Through signal integration, the ELPI effectively operates as a particle counter with a minimum aerodynamic cut diameter of ∼ 30 nm. Particles entering the ELPI are charged using a unipolar diode type corona charger and size discriminated using a series of Berner low-pressure impactors designed with sequentially smaller cut diameters. Ions and particles smaller than approximately 20 nm are electrically trapped prior to entering the impactor stages (CitationMarjamäki et al. 2000). The quantity of charge transferred to the impactors is measured at a 1-Hz sampling frequency by electrometers connected to the individual stages. The aerodynamic size of the particles is determined from the impactor stage cut diameter and the number concentration is determined from an empirical charging efficiency function. The true response time of the ELPI is a function of the particle transit time through the impactor series and the response of the electrometer to changing currents (CitationMaricq et al. 2000). A typical response time of 1–20 s makes the ELPI effective at capturing real-time changes in particle size distributions and the ELPI has been previously used for the measurement of ultrafine particle emissions from diesel mobile sources such as transit buses (CitationLanni et al. 2001; CitationChatterjee et al. 2002; CitationLanni et al. 2003). CitationMaricq et al. (2000) explicitly address several complicating issues concerning ELPI size distribution measurements of combustion exhaust (CitationMaricq et al. 2000). For example, the smallest particles, with the highest electrical mobility, must pass through all of the impactor stages and can readily be lost in the larger particle stages through diffusion and electrostatic forces. The measured size distributions are empirically corrected for these small particle losses, though rapid changes in signal complicates the correction due to the several second residence time for air passing through the cascade impactor series. Improvements in the ELPI design, including an additional impactor stage to probe particle sizes ∼ 20 nm have been made by Dekati Ltd. subsequent to these measurements and could prove useful in future studies of aircraft aerosol.
Logan 2003—Diffusion Charger
A measure of the aerosol active surface area or Fuchs surface area (i.e., surface accessible by direct diffusion) was obtained using an EcoChem Diffusion Charger (DC) model 2000CE (CitationSiegmann et al. 1999). The DC employs a unipolar corona charger to charge sampled particles and measures the total current subsequently carried by the particles to an electrometer. This instrument provides a measure of the surface area of the emission particles, but it is not the same as Brunauer-Emmet-Teller (BET) measurements (CitationMatter et al. 1999). Ion attachment depends on particle size and is relatively independent of particle composition (CitationHinds 1999). Factory calibrations were used to convert the DC signal to a surface area measurement. The limit of detection for this instrument is 1 mm2 m− 3, and the maximum meaningful value is 5000 mm2 m− 3. Due to nonlinearities in the analog output for smaller concentrations, values less than ∼ 30 mm2 m−3 are of uncertain quality. Typical plumes encountered in the Logan work contained CO2 concentrations ∼ 25 ppm larger than ambient concentrations and DC signal levels ∼ 10 times greater than the non-plume condition signal levels, which were typically 10 mm2 m−3. The total systematic uncertainty is estimated to be 20% based on a comparison of four sets of factory calibrated instruments. The signal variance during the non-plume conditions when taken together with the typical excess CO2 and DC measurements within plumes define a minimum detectable EI of 0.1 m2 kg−1 CO2 at a signal-to-noise ratio of 2.
Logan 2003—Photoelectric Aerosol Sampler
The photoelectric aerosol sensor (EcoChem PAS 2000 CE) provides a measure of the chemical surface properties of sampled particles (CitationMatter et al. 1999). Particles are irradiated with UV light from an excimer lamp. Specific chemical compounds, such as polycyclic aromatic hydrocarbons (PAH), absorb the light and emit an electron. The positively charged particles are collected on a filter and the total aerosol charge is monitored by an electrometer. The PAS has been shown to provide a measure of the surface-bound PAH concentrations for PAH-containing particles 1.0 μ m and smaller (CitationBurtscher et al. 1993; CitationHart et al. 1993; CitationWilson et al. 1994). Recent work indicates that the PAS also responds to elemental carbon (CitationBaltensperger et al. 2001; CitationBurthscher 2005) on the surface of soot particles that do not contain surface-bound PAHs. While it is likely that the measured PAS signal in this work is due to elemental carbon response, we have made no attempt to explicitly calibrate or quantify the PAS measurements aside from applying the manufacturer's built-in calibration. The built-in calibration is widely used due to the unavailability of a PM stream with a known PM-bound PAH quantity. Furthermore, PAH measured by PAS (total PM-bound PAHs) is different from PAH measured through off-line chemical analysis, which provides additional PAH speciation. Prior studies, however have evaluated the built-in calibration with NIST diesel PM standard reference materials with 42 certified PAH values and found good correlation and satisfactory values with the built-in calibration (CitationTang et al. 2001; CitationLanni et al. 2003).
Analogous to the diffusion charger instrument, the systematic error is estimated to be 20%. The ratios of the PAS/DC signals, unaffected by the applied calibration and conversion to EI, are presented and discussed in the context of characterizing the surface properties of aircraft combustion particles. The ratios provide a unique surface-sensitive combination of a chemically dependent signal (PAS) and a chemically independent signal (DC). The PAS instrument has been used previously in studies of motor vehicle exhaust, specifically in conjunction with co-located DC and CPC instrumentation (CitationMatter et al. 1999; CitationBukowiecki et al. 2002), and exposure to PAHs (CitationLevy et al. 2001; CitationLevy et al. 2002; CitationMarr et al. 2004). With the factory calibration, the limit of detection for this instrument is 1 ng m−3, and the maximum meaningful value is 5000 ng m−3. Analogous to the issues associated with the diffusion charger, values less than ∼ 30 ng m−3 are of uncertain quality due to nonlinearities in the analog output for smaller concentrations.
Logan 2003—CPC
The condensation particle counter (CPC) used for these measurements was a TSI, Inc. model 3022A. The CPC optically counts particles with physical diameters in the range from 7 nm to 2.5 um. The upper size limit is defined by the size cut of cyclone separator on the main inlet system in the mobile laboratory. Smaller size particles are “grown” to larger, optically detectable size by condensation of butanol vapor. The counting efficiency of the 7 nm size particles is rated at 50% efficiency and reaches 100% efficiency for particles of ∼ 20 nm. The model 3022A CPC uses both single-count and photometric detection modes and provides accurate measurements in concentrations as high as 107 particles cm−3. The large dynamic range is important for measurements of combustion plumes. The CPC was operated in the high flow mode (1.5 LPM) to maximize response time. The instrument was sampled and data logged at 1 Hz (monitoring the available analog output), but has a measured response of ∼ 2 for a 50% response to a step change in aerosol concentration.
Analysis Methods
The metric used to compare aviation related emissions, is an emission index (EI). It typically is expressed in units of mass of emissions per mass of fuel. In the specific case of particle number measurements, the number-based emission index (EIn) is calculated with units of particle number per mass of fuel (CitationFahey 1995; CitationAnderson et al. 1998b). The particle emission index is determined from the measured em ission ratio, defined as the ratio of the emitted exhaust component concentration (e.g., particle number concentration or the active surface area concentration) to the emitted CO2 concentration, and an assumed CO2 EI. The EI either particle number or particulate mass (per kg fuel) for a given exhaust component concentration, X, is calculated as
Two different methods were used to extract emission indices from the time series measurements of the diluted plumes presented in this work. In the case of the ELPI data obtained during the JFK study, the ELPI software was used to create size distributions and integrated number concentrations as a function of time. Time periods of interest and engine operational states that were identified during previous analysis of gas-phase NOx emissions (CitationHerndon et al. 2004) were used again in this analysis. For each of the plume periods (15–35), an average background size-distribution was determined from periods just before and after each plume event. The size distributions measured by the ELPI during the plume event were background subtracted and divided by the excess CO2 concentration. These distributions were then averaged for each plume event into a representative CO2-normalized size distribution. Particle number emission indices were then obtained for each plume by integrating these normalized size distributions.
For the Logan measurements, number-based emission ratios were obtained from a direct correlation of the 1 s CPC and CO2 measurements. Since the instrument response time constants for the DC and PAS instruments were ∼ 10 s, somewhat longer than the CO2 instrument, an alternative approach was adopted for the analysis of these data. In this case, background signal levels were determined by choosing periods prior to and after the plume event and the area between the event signal and this background level was calculated for each instrument.
Plume events were classified by engine condition using wind speed, direction, and distance to the taxiway or runway and the visually identified operational state of the aircraft. The mobile laboratory was moved twice at Logan airport in order to place it downwind from aircraft operating in the various states. Simultaneous measurements of NOx emission ratios confirm the plume assignments and engine operation states. The median NOx emission indices at Logan airport for the various visually identified activities were; idle: 2.0, taxiway acceleration: 10, approach: 13, take-off: 19, each in units of g of NOx (as NO2) per kg fuel.
RESULTS AND DISCUSSION
Particle Size Distribution Measurements
The ELPI-based size distributions during a 20-min period at JFK for taxi and take-off plumes are shown in the left and right panels of , respectively. The spectra shown in this figure have been background subtracted and normalized by the excess CO2 concentration measured in the plume. These size distributions are a subset of the total number of aircraft idle and take-off plumes measured during the JFK study and were chosen due to the unchanging, well-characterized background aerosol that exists before and after each of these plumes. The mobile laboratory was stationary during this testing period relative to the target taxiway and runway, providing nearly constant evolution periods between exhaust emission and sampling for idle and take-off conditions, respectively. Each spectrum is colored according to the measured NOx EI. All of the taxi plumes exhibit a NOx EI less than approximately 10 g kg−1, whereas the take-off plumes typically exhibit larger NOx EIs.
FIG. 1 ELPI size distributions for taxi and take-off plumes. The figure depicts background subtracted, CO2 normalized, average size distributions for individual taxi and take-off plume encounters from 7 aircraft during a 20-min period. These size distributions are representative of all size distribution measurements taken at JFK airport. Each distribution is shaded by the simultaneously determined NOx emission ratio. The panel on the left shows the taxi plumes, while the right panel shows the take-off plumes. These size distributions show a prevalent mode ∼ 90 nm. Some of the aircraft also show evidence for a second mode at smaller sizes (< 30 nm).
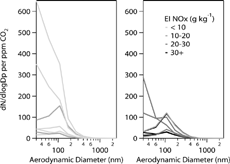
All size distributions, except for three plume events, show a distinct mode near 100 nm. The average geometric mean diameter (GMD) for this group is 90 +/− 6 nm. Three events, two idle/taxi events and one take-off event, also show clear evidence for a second smaller mode that peaks below the 30 nm cutoff of the ELPI instrument. The average GMD for the second group is 61 +/− 9 nm. These observations suggest that aircraft particulate emissions contain at least a single ∼ 90 nm mode and potentially a second smaller (< 30 nm) mode. An inventory of all of the size distribution data suggests that the sub-30 nm mode is more prevalent in idle plumes relative to the take off plumes. The smaller mode is at or near the ELPI size cutoff, where some variability in the measurements may be expected. The apparent variability in the results from the 30 nm impactor stage is greater than previous ELPI measurements (CitationLanni et al. 2001; CitationChatterjee et al. 2002; CitationLanni et al. 2003), supporting the presence of a small particle mode.
The size distributions measured using the ELPI in this work have been dramatically diluted by ambient air and aged ∼ 90 seconds. The measurements of the size distribution of particulate emissions from aviation engines in the literature follow two different approaches, neither of which is completely analogous to this measurement strategy. The first are conducted at altitude, while the engine is operating under representative cruise conditions. The second are conducted on the ground using direct extractive sampling within several meters of the engine exit plane. Despite the differences in sampling and engine state, it is useful to compare these size distributions to other reported measurements.
In situ measurements of number distributions measured in flight 40–100 meters behind the target aircraft (CitationSchröder et al. 2000) indicate the presence of two modes. The larger mode has a mode size between 20–30 nm and consists mainly of non-volatile components (soot). The smaller mode is less than 10 nm, is greater in number than the soot mode and it comprised of principally volatile components.
CitationLilenfeld and colleagues (1995) provide two size distributions for aircraft engines measured 2 m behind the engine exit plane on the ground. The GE-404 engine shown in and the Pratt PT6-42 engine shown in of the Lilenfeld paper indicate lognormal size distributions with mode diameters of 50 and 35 nm, respectively CitationPetzold and colleagues (2003) measured a GMD of ∼ 40 nm during a ground test of an aircraft engine combustor. CitationSchmid and colleagues (2004) measured size resolved number emission indices as a function of power setting on an “old” engine technology and observed an increase in the GMD with increasing power. The CitationSchmid and colleagues (2004) measurements conducted at the engine exit plane observed a single soot mode between 55 and 90 nm (58% to 98% of rated thrust, respectively). CitationAnderson and colleagues (2005) measured bimodal size particle distributions in the exhaust of a Rolls Royce RB211 during ground-based tests, with a high number count mode at ∼ 22 nm and a minor mode at 60 nm.
FIG. 2 Photoelectric Aerosol Sampler (PAS) vs. Diffusion Charging (DC). The emission indices for each instrument for each sampled plume at Logan Airport (Boston) is plotted against one another. The factory calibration for each of the PAS and DC instruments has been used to calculate the EI units. The points have been colored according the measured NOx emission ratio and are denoted by markers indicative of the plume origin. The video record was used in conjunction with plume arrival times and wind speed to make these tentative assignments. There are two modes apparent in this data, one that coarsely follows the one-to-one reference line, and another that exhibits DC signal, but has little measured PAS signal. This suggests that the character of the particulate surface at high power or take-off is very different from that of the low power (idle and taxiway accelerations) aerosol. See text for additional details.
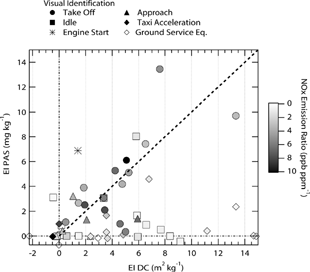
FIG. 3 Histogram of estimated EIn for take-off plumes. The take-off plumes measured at JFK have been binned into the depicted histogram (top). Note that due to the size dependence of the ELPI transmission, these are assumed to be low. The lower panel of this figure shows the time series of the integrated ELPI signal along with the CO2 and NOx data. The selected time shows two distinct take-off plumes (Jet #1 and #2) with essentially equivalent emission indices for NOx, but very different EIn.
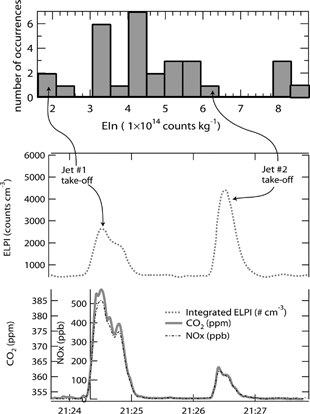
A majority of the literature results provide evidence for a bimodal nature to the size distributions. This is in agreement with the subset of the ELPI size distributions shown in , which exhibited two modes. The larger mode in this work, ∼ 90 nm—aerodynamic diameter, agrees well with the larger mode measured by Anderson et al. and Lilenfeld et al. 60 and 80 nm—mobility diameter, respectively. All of the ground-based measurements of this mode are larger than the observed 20–30 nm soot mode at altitude. Generally the literature values of mode diameters are smaller when measured at altitude compared to ground based measurements.
Two key differences between all of these measurements are differences in engine operational state and in evolution timescales at their respective ambient conditions. In this study, the exhaust plumes were sampled downwind of the aircraft engines. Generally this resulted in 1–2 minutes of dilution and atmospheric processing. Particle coagulation and gas-to-particle condensation with time could play a role in reducing the smaller mode (relative to the larger mode) at our downwind measurement point. For example, not all of the ELPI size distributions contained the smaller mode. There are two possible reasons for these observations: (1) the small mode is coming to the downwind sampling point intact but is below the size cutoff of the instrument (30 nm); (2) the smaller mode is attenuated via particle coagulation or condensational growth during advection.
Particle Surface Composition
shows the emission index for the DC and PAS instruments for each plume detected at the Boston Logan International Airport as a correlation plot. The data have been converted into emission indices for the active surface area (DC) and for the concentration of surface-bound photoionizable species (PAS), using the factory default calibrations (CitationSiegmann et al. 1999). The zero lines and the one-to-one line are included for reference. The simultaneously determined plume NOx emission strength colors each of these data points and the symbol indicates their source. The interpretation of the individual instrument signals, PAS and DC, is uncertain, however the significant result, the ratio of the two signals, is unchanged by subsequent conversion to units of EI via the concomitant CO2 plume strength.
The PAS and DC techniques, when taken together, provide information about the surface composition of the measured exhaust particles. In particular, the ratio of PAS to DC is a characteristic property of different types of combustion particles (CitationMatter et al. 1999). In previous studies, the PAS/DC signal ratio has been found to be 1–2 in roadway air influenced by fresh vehicle exhaust and 0.4–0.6 in urban locations away from roadways (CitationMatter et al. 1999; CitationMarr et al. 2004). When the ratio of PAS/DC is lower than ∼ 0.05 there are two possibilities regarding the implied composition (CitationBukowiecki et al. 2002): (1) the aerosol is composed of numerous particles not containing EC or PAHs (i.e., non-sooty particles), or (2) the particles contain EC/PAHs but are coated by organic/inorganic species that do not exhibit a large photoelectric effect, thereby rendering the core EC/PAHs undetectable by photoionization. This phenomenon is well summarized in Figure 10 of the CitationBukowiecki et al. (2002) paper.
The relationship in between the response of the PAS and DC instruments clearly indicates two different categories of plume events. The majority of the take-off plumes lie along the one-to-one line indicating that the PAS/DC ratio for the take off plumes is around 1. This ratio, which is similar to that observed for fresh vehicle exhaust plumes, indicates that the take-off plume particles contain significant amounts of uncoated EC surface area. In contrast, the majority of the idle plumes lie along the zero PAS line (x-axis). This suggests that the surface composition of the minutes old idle plumes is very different from that of the minutes old take-off plumes. In particular, the low ratio suggests that under idle conditions the exhaust particle surface area is dominated by condensable organic/inorganic species rather than by EC. Gas-phase sulfuric acid is the primary condensable inorganic species. Ultrafine aerosol growth mechanisms find that something besides gas-phase sulfuric acid controls particle growth when the engine is burning low-sulfur fuel (CitationKärcher et al. 1998). Since the gas-phase hydrocarbon content in idle plumes will generally be more than ten times greater than that in take-off plumes (CitationSpicer et al. 1994), it is likely that organic condensable species play an important role in the idle plume particles.
Particle Number Emission Indices
The results from the two campaigns for the analyzed take-off and idle plumes are tabulated in . The listed results for EIn at JFK were measured using the ELPI while those for the Logan work were measured using the CPC. The DC and PAS instruments were not present in the JFK work.
TABLE 1 Summary of emission index results for JFK and Logan
EIn JFK-ELPI Measurements
ELPI particle number emission indices were obtained by integrating the CO2-normalized size distributions for idle and take-off events. These EIn span a wide range of values, consistent with which indicates that the ∼ 100 nm and < 30 nm size emission modes vary greatly in number count for both types of events. The average ELPI measured idle emission index (2 ± 3 × 1014) is greater than the take-off emission index (1 ± 0.7 × 1014) in magnitude and variance.
The size dependence and magnitude of number-based aerosol emission indices vary greatly with atmospheric composition and plume age (CitationAnderson et al. 1998b; CitationSchröder et al. 1998). During the JFK measurements, both the take-off and idle plumes had nearly equivalent ages and were measured under similar conditions. The dilution ratios were typically very similar as well. The ambient humidity and temperature also did not appreciably change during the timescale of this work. Thus, observed variability in the idle and take-off EIn is likely related to engine condition and state and/or fuel composition rather than environmental factors. It is also consistent with the Logan CPC results that are discussed below.
provides an example of the type of variability observed between take-off plumes. The top panel shows a histogram of the ELPI-based number emission indices determined for take-off plumes. The time series for the CO2, gas-phase NOx, and integrated ELPI signal for two of these take-off plumes with very different EIn are shown in the lower panels of . The two plumes have nearly equivalent NOx emissions, as indicated by the similar ratios of NOx to CO2; however, they exhibit a strikingly difference in the particle emission indices. The aircraft in question were each powered by modern turbine engines and no intervening taxiing or other ground service equipment were observed in the video record of these activities. This variability could arise from numerous sources, including differences in engine technologies, engine maintenance history, or fuel sulfur content. At this point no systematic effort has been made to correlate the variability in EIn with these aircraft specific parameters.
EIn Logan-CPC Measurements
At Logan airport, in addition to the PAS/DC aerosol surface area instruments, a condensation particle counter (CPC) was used to measure total particulate number concentrations. The PAS/DC ratio is plotted against the CPC based EIn for take-off and idle conditions in . Though only the take-off and idle plumes are shown for clarity, the median values of EIn for all plume categories are shown in a lower section of .
FIG. 4 Ratio of photoionization to diffusion charging as a function of EIn. The y-axis in this figure is the ratio of two surface area measurements; Photoionization (PAS) over Diffusion Charging Active Surface (DC) Area. This ratio is plotted against EIn. The symbol key shows the visual identification of the plume origin (state of the aircraft in question). The shading scale shows the estimate of the degree of dilution with the lighter gray tones indicative of more dilution prior to sampling. The points in the lower panel have nothing to do with PAS/DC ratios, but rather represent the median values of EIn for each of the engine activity categories.
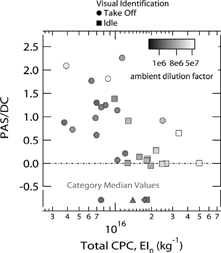
In principle, some of the observed plume to plume variability in this EIn could be due to differences in plume age (CitationAnderson et al. 1998b; CitationSchröder et al. 1998). To first order, the degree of dilution could be used as a proxy for the age of the plume prior to sampling. The dilution factor was estimated for each plume using the peak CO2 concentration and reasonable estimates of CO2 concentrations in the combustor exhaust and turbine cooling flows. This figure shows there may be a slight bias toward lower EIn for the most diluted plumes, however no conclusive trend is present.
The CPC EIn depicted in were measured for different aircraft with differing engine types under take-off and taxi conditions. The magnitude and variance of the EIn in are similar to those observed previously (CitationAnderson et al. 1998a). Even though the plumes studied in this work are for idle and take off, of the CitationAnderson et al. (1998a) paper shows the in-situ cruise total CPC EIn ranging from (0.8–500) × 1015 particles kg−1 fuel for particles > 4 nm in diameter. Their study reports results for various engines at cruise, and with differing ambient conditions where the non-volatile component of these emissions were between 1–50% of the total condensation nuclei. The reported variations of individual aircraft EIn measurements were less than the variability observed between different airframe/engine combinations.
The specified lower limit for particle detection in the ELPI (at JFK) is estimated to be ∼ 30 nm while that for the CPC (at Logan) is 7 nm. The equivalent aerodynamic diameter of the CPC is estimated to be 9 nm (CitationHinds 1999; CitationKhlystov et al. 2004). Though the potential for different processing of the emitted aerosol exists between the two sampling sites, the results indicate that turbine engine exhaust aerosol at idle is characterized by the presence of a significant small size mode. The EIn measured by the ELPI, (1–10) × 10 14 particles kg−1 fuel, appear to be 2 orders of magnitude lower than the EIn measured by the CPC, (1–5) × 1016 particles kg−1 fuel. In light of the size cutoffs for the two instruments, and assuming similar processing of the aerosol prior to sampling, this result suggests the presence of a large number of particles that are smaller than the ELPIs cutoff size (< 30 nm) and are larger than the reported ∼ 7–9 nm cut diameter of the CPC. Furthermore, the good agreement between the ELPI EIn (1–10) × 1014 particles kg−1 fuel and the observed nonvolatile (> 20 nm) EIn (1–20)× 1014 particles kg−1 fuel indicates that the ∼ 90 nm mode observed by ELPI, and characterized by a positive PAS/DC ratio, may be dominated by nonvolatile elemental carbon components.
For the JFK and Logan EIn results, the variance with the sampled pool of engine states and the overall comparison between engine states are qualitatively similar. In both cases, the take-off and taxi plume EIn's are observed to cover an order of magnitude and are not well correlated with the emitted NOx. This indicates that the total particle emissions are not controlled by the same engine conditions that control the gas phase NOx chemistry. Furthermore, the median value for idle EIn(1.8 × 1016) is greater than that of take-off EIn (7.6 × 1015). This observation is present in the ELPI measurements as well, though the absolute magnitudes differ.
shows an inverse relationship between the PAS/DC ratio and the CPC EIn for the take-off plumes measured at Logan Airport. This suggests that changes in particle surface composition are correlated with observed EIn variability for take-off plumes. Specifically, the take-off plumes with a PAS/DC ratio of ∼ 1 exhibit a median EIn of ∼ 8 × 1015 particles kg−1 fuel, whereas the idle plumes with a near zero PAS/DC ratio have a median EIn of ∼ 2 × 1016 particles kg−1 fuel. These results do not appear absolute, however, as several take-off plumes exhibit a lower PAS/DC ratio and a higher EIn. The take-off plumes appear to follow a general trend in which larger EIn values correlate with smaller PAS/DC ratios. This trend could be explained by the following two observations: (1) ELPI size distributions and ELPI/CPC EIn comparisons indicate that the larger EIn values are associated with increased production of the number of sub-30 nm exhaust particles; (2) Previous studies (CitationAnderson et al. 1998b; CitationKärcher et al. 1998; CitationSchröder et al. 1998) have shown that aircraft exhaust particles smaller than 30 nm are primarily composed of volatile organic/inorganic species. The variability in idle EIn values does not appear to be as clearly correlated with PAS/DC ratios.
CONCLUSIONS
This work describes the measurement of particulate emissions, (EIn and surface properties) by the analysis of data from wind advected plumes at an airport. The first point demonstrated in this work is that the take-off and idle particulate emissions, whether measured with the ELPI or with the CPC appear to be significantly variable from one engine to the next. Statements about the genuine distributions of EIn among the fleet of operational aircraft would require many more samples under different atmospheric conditions. The range of EIn for the take-off plumes measured by the CPC spans almost an order of magnitude. This is presumably due to a weighting of EIn toward the aerosol number counts below 30 nm. This indicates the presence of a mode at ∼ 90 nm aerodynamic diameter as well as a second mode (< 30 nm). Future work will be required to elucidate the source of the observed variability in EIn. There is no evidence that the cause of the width of the distribution is due to changing atmospheric processing or measurement noise.
The second finding is that EIn appears to be greater at idle, relative to take-off. Both of the distributions resulting from the ELPI and CPC measurements show a shift in the median and average values to greater numbers at idle. For both the ELPI and CPC data at two different airports, the median value of EIn for idle is approximately twice as large as take-off. The third important finding in this work involves the surface composition of the aerosol between the take-off and idle modes of operation. The ratio of PAS to DC surface area for the take-off plumes indicates that the one-minute old aerosol contains surface bound PAH or other PAS active material. The most likely explanation is that the PAS instrument is responding to the EC in the take-off plume. In the case of the idle plumes, the aerosol is comprised of various organic (non-PAS active), inorganic components and black carbon coated with condensable material.
These measurements demonstrate that the technique of monitoring advected plumes from in-use commercial aircraft to a suite of sampling instruments can be used to give quantitative insight into the magnitude and composition of aviation related aerosol emissions. These results show that the engine state does not lead to as consistent a trend in EIn as is observed in NOx. This work also suggests that in the context of airport activity, the majority of the particles in “aged” plumes may be formed by the condensation of low-volatility gas-phase species as the plume cools and dilutes. Future work will be required to associate the variability of EIn with other factors.
Acknowledgments
These measurements would not have been possible without cooperation from The Port Authority of NY & NJ, specifically LaGuardia and John F. Kennedy International Airport Operations Staff, “99,” “97,” and “93.” The authors gratefully acknowledge the assistance of Flavio Leo, Aviation Planning Manager and Vincent Cardillo, Airport Operations Manager at The Massachusetts Port Authority. We would like to thank Gregg Fleming of Volpe National Transportation Systems Center for helping set up the Logan measurements and providing assistance in the analysis of the JFK results. We would also like to thank Shida Tang of the New York State Department of Environmental Conservation for assistance in the analysis of the ELPI data and helpful discussions. This work was funded in part by the EPA Supersite Programs: PMTACS-NY and CEPEX, the MCMA field measurement program within the Integrated Program on Urban, Regional, and Global Air Pollution at Mass. Institute of Technology and the NOAA Global Climate Change program.
Notes
a EI NOx is expressed as g NO2 per kg fuel.
b EIn measured by two instruments with different sample size ranges: ELPI at JFK (30–2500 nm) and CPC at Logan (7–2500 nm).
c Units for DC and PAS instruments are provided using factory calibration.
d Fishers skewness coefficient.
REFERENCES
- Anderson , B. E. , Blake , D. R. and McEachern , M. 2003 . “ Hydrocarbon Emissions from a Modern Commercial Airliner, ” . In NASA Technical Memorandum, Chemistry and Dynamics Branch, Atmospheric Sciences Competency , NASA Langley Research Center . Appendix B
- Anderson , B. E. 2005 . “ Overview of Results from the NASA Experiment to Characterize Aircraft Volatile Aerosol and Trace Species Emissions (EXCAVATE) ” . In NASA Technical Memorandum. coauthors
- Anderson , B. E. , Cofer , W. R. , Bagwell , D. R. , Barrick , J. D. , Hudgins , C. H. and Brunke , K. E. 1998a . Airborne Observations of Aircraft Aerosol Emissions I: Total Nonvolatile Particle Emission Indices . Geophys Res. Lett. , 25(10) : 1689 – 1692 .
- Anderson , B. E. , Cofer , W. R. , Barrick , J. D. , Bagwell , D. R. and Hudgins , C. H. 1998b . Airborne Observations of Aircrat Aerosol Emissions II: Factors Controlling Volatile Particle Production . Geophys Res. Lett. , 25 : 1693 – 1696 . [CROSSREF] [CSA]
- Baltensperger , U. , Weingartner , E. , Burthscher , H. and Keskinen , J. 2001 . “ Dynamic Mass and Surface Area Measurements ” . In Aerosol Measurement. , Edited by: Baron , P. A. and Willeke , K. 387 – 418 . New York : Wiley .
- Bukowiecki , N. , Kittleson , D. B. , Watts , W. F. , Burtscher , H. , Weingartner , E. and Baltensperger , U. 2002 . Real-Time Characterization of Ultrafine and Accumulation Mode Particles in Ambient Combustion Aerosols . Aerosol Sci. , 33 : 1139 – 1154 . [CROSSREF]
- Burthscher , H. 2005 . Physical Characterization of Particulate Emissions from Diesel Engines: a review . J. Aerosol Science. , 36 : 896 – 932 . [CROSSREF]
- Burtscher , H. and Siegmann , H. C. 1993 . Photoemission for in Situ Analysis of Particulate Combustion Emissions . Water, Air, and Soil Pollution , 68 : 125 – 136 . [CROSSREF] [CSA]
- Chatterjee , S. , Conway , R. , Lanni , T. , Tang , S. , Frank , B. P. , Rosenblatt , D. , Bush , C. , Lowell , D. , Evans , J. , McLean , R. and Levy , S. 2002 . Society of Automotive Engineers , 2002-01-0430[CSA]
- DeCarlo , P. F. , Slowik , J. G. , Worsnop , D. R. , Davidovits , P. and Jimenez , J. L. 2004 . Particle Morphology and Density Characterization by Combined Mobility and Aerodynamic Diameter Measurements. Part 1: Theory . Aerosol Sci. Technol. , 38 : 1185 – 1205 . [CROSSREF]
- Demerjian , K. L. 2000–2004 . PMTACS-NY Overview . http://www.asrc.cestm. albany.edu/pmtacsny/
- Dunlea , E. J. , Herndon , S. C. , Nelson , D. D. , Volkamer , R. M. , San Martini , F. , Zahniser , M. S. , Shorter , J. H. , Wormhoudt , J. C. , Lamb , B. K. , Allwine , E. J. , Gaffney , J. S. , Marley , N. A. , Grutter , M. , Kolb , C. E. , Molina , L. T. and Molina , M. J. 2005 . “ Evaluation of Nitrogen Dioxide Chemiluminescence Monitors in a Polluted Urban Environment ” . In preparation for ACP
- E. E. A. 2004 . “ EMEP/CORINAIR Emissions Inventory Guidebook ” . In EEA Group 08 http://reports.eea.eu.int/EMEPCORINAIR4/en/page002.html
- Fahey , D. W. , Keim , E. R. Boering , K. A. 1995 . Emission Measurements of the Concorde Supersonic Aircraft in the Lower Stratosphere . Science , 270 : 70 – 74 .
- Gysel , M. , Nyeki , S. Weingartner , E. 2003 . Properties of Jet Engine Combustion Particles During the PartEmis Experiment: Hygroscopicity at Subsaturated Conditions . Geophys Res. Lett. , 30 ( 11 ) : 1566 [CROSSREF]
- Hart , K. M. , McDow , S. R. , Giger , W. , Steiner , D. and Burthscher , H. 1993 . The Correlation Between In-Situ, Real-Time Aerosol Photoemission Intensity and Particulate Polycyclic Aromatic Hydrocarbon Concentration in Combustion Aerosols . Water, Air, and Soil Pollution , 68 : 75 – 90 . [CROSSREF] [CSA]
- Herndon , S. C. , Rogers , T. , Dunlea , E. J. , Miake-Lye , R. C. and Knighton , B. 2005 . “ Using Proton Transfer Reaction Mass Spectrometry (PTRMS) to Measure Emission Indices from in-use Commercial Aircraft ” . In Submitted to ES&T.
- Herndon , S. C. , Shorter , J. H. , Zahniser , M. S. , Nelson , D. D. J. , Jayne , J. T. , Brown , R. C. , Miake-Lye , R. C. , Waitz , I. A. , Silva , P. , Lanni , T. , Demerjian , K. L. and Kolb , C. E. 2004 . NO and NO2 Emissions Ratios Measured from in use Commercial Aircraft during Taxi and Take-Off . Environ. Sci. Technol. , 38 : 6078 – 6084 . [PUBMED] [INFOTRIEVE] [CROSSREF]
- Hinds , W. C. 1999 . Aerosol Technology: Properties, Behavior, and Measurements of Airborne Particles. , New York : John Wiley & Sons .
- ICAO . 1995 . Engine Exhaust Databank Montreal
- 1999 . “ Aviation and the Global Atmosphere, Intergovernmental Panel on Global Change ” . In Aviation and the Global Atmosphere. , Cambridge University Press . IPCC, Ed.
- Kärcher , B. , Yu , F. , Schröder , F. P. and Turco , R. P. 1998 . Ultrafine Aerosol Particles in Aircraft Plumes: Analysis of Growth Mechanisms . Geophys Res. Lett. , 25 ( 15 ) : 2793 – 2796 . [CROSSREF] [CSA]
- Keskinen , J. , Pietarinen , K. and Lehtimäki , M. 1992 . Electrical Low Pressure Impactor . J. Aerosol Science , 23 : 353 – 360 . [CROSSREF]
- Khlystov , A. , Stanier , C. and Pandis , S. N. 2004 . An Algorithm for Combining Electrical Mobility and Aerodynamic Size Distributions Data When Measuring Ambient Aerosol . Aerosol Sci. Technol. , 38 : 229 – 238 . [CROSSREF]
- Kolb , C. E. , Herndon , S. C. McManus , J. B. 2004 . Mobile Laboratory with Rapid Response Instruments for Real-time Measurements of Urban and Regional Trace Gas and Particulate Distributions and Emission Source Characteristics . Environ. Sci. Technol. , 38 : 5694 – 5703 . [PUBMED] [INFOTRIEVE] [CROSSREF] [CSA]
- Lanni , T. , Chatterjee , S. , Conway , R. , Windawi , H. , Rosenblatt , D. , Bush , C. , Lowell , D. , Evans , J. , McLean , R. and Levy , S. 2001 . “ Performance and Durability Evaluation of Continuously Regenerating Particulate Filters on Diesel Powered Urban Buses at NY City Transit ” . In Society of Automotive Engineers 2001-01-0511
- Lanni , T. , Frank , B. P. , Tang , S. , Rosenblatt , D. and Lowell , D. 2003 . “ Performance and Emissions Evaluation of Compressed Natural Gas and Clean Diesel Buses at New York City's Metropolitan Transit Authority ” . In Society of Automotive Engineers 2003-01-0300
- Levy , J. I. , Dumyahn , T. and Spengler , J. D. 2002 . Particulate Matter and Polycyclic Aromatic Hydrocarbon Concentrations in Indoor and Outdoor Microenvironments in Boston, Massachusetts . J Exposure Analysis and Environ. Epidemiol. , 12 : 104 – 114 . [CROSSREF] [CSA]
- Levy , J. I. , Houseman , E. A. , Spengler , J. D. , Loh , P. and Ryan , L. 2001 . Fine Particulate Matter and Polycyclic Aromatic Hydrocarbon Concentration Patterns in Roxbury, Massachusetts: A Community-Based GIS analysis . Environ. Health Perspectives , 109 ( 4 ) : 341 – 347 . [CSA]
- Lilenfeld , H. , Whitefield , P. D. and Hagen , D. 1995 . “ Soot Emissions from Jet Aircraft ” . In American Institude of Aeronautics and Astronautics AIAA-95-0110
- Maricq , M. M. , Podsiadlik , D. H. and Chase , R. E. 2000 . Size Distributions of Motor Vehicle Exhaust PM: A Comparison Between ELPI and SMPS Measurements . Aerosol Sci. Technol. , 33 : 239 – 260 . [CROSSREF]
- Marjamäki , M. , Keskinen , J. , Chen , D.-R. and Pui , D. Y. H. 2000 . Performance Evaluation of the Electrical Low-Pressure Impactor (ELPI) . J. Aerosol Sci. , 31 ( 2 ) : 249 – 261 . [CROSSREF] [CSA]
- Marr , L. C. , Grogan , L. A. Worhrnschimmel , H. 2004 . Vehicle Traffic as a Source of Particulate Polycyclic Aromatic Hydrocarbon Exposure in Mexico City . Environ. Sci. Technol. , 38 ( 9 ) : 2584 – 2592 . [PUBMED] [INFOTRIEVE] [CROSSREF] [CSA]
- Matter , U. , Siegmann , H. C. and Burthscher , H. 1999 . Dynamic Field Measurements of Submicron Particles from Diesel Engines . Environ. Sci. Technol. , 33 : 1946 – 1952 . [CROSSREF] [CSA]
- Petzold , A. , Stein , C. Nyeki , S. 2003 . Properties of Jet Engine Combustion Particles During the PartEmis Experiment: Microphysics and Chemistry . Geophys Res. Lett. , : 30
- Pison , I. and Menut , L . 2004 . Quantification of the Impact of Aircraft Traffic Emissions on Tropospheric Ozone Over Paris Area . Atmos. Environ. , 38 : 971 – 983 . [CROSSREF]
- Schäfer , K. , Heland , J. Lister , C. W. W. D. H. 2000 . Nonintrusive Optical Measurements of Aircraft Engine Exhaust Emissions and Comparison with Standard Intrusive Techniques . Appl. Optics , 39 : 441 – 455 .
- Schmid , O. , Hagen , D. E. Whitefield , P. D. 2004 . Methodology for Particle Characterization in the Exhaust Flows of Gas Turbine Engines . Aerosol Sci. Technol. , 38 : 1108 – 1122 . [CROSSREF]
- Schröder , F. P. , Kärcher , B. , Petzold , A. , Baumann , R. , Busen , R. , Hoell , C. and Schumann , U. 1998 . Ultrafine Aerosol Particles in Arcraft Plumes: In situ Observations . Geophys Res. Lett. , 25 ( 15 ) : 2789 – 2792 . [CROSSREF] [CSA]
- Schröder , F. P. Brock , C. A. 2000 . In Situ Studies on Volatile Jet Exhaust Particle Emissions: Impact of Fuel Sulfur Content and Environmental Conditions on Nuclei Mode Aerosols . J. Geophys. Res. , 105 : 19941 – 19954 . [CROSSREF] [CSA]
- Schulte , P. , Schlager , H. , Ziereis , H. , Schumann , U. , Baughcum , S. L. and Deidewig , F. 1997 . NOx Emission Indices of Subsonic Long-Range Jeft Aircraft at Cruise Altitude: In Situ Measurements and Predictions . J. Geophys. Res. , 102 : 21431 – 21442 . [CROSSREF] [CSA]
- Siegmann , K. , Scherrer , L. and Siegmann , H. C. 1999 . Physical and Chemical Properties of Airborne Nanoscale Particles and How to Measure the Impact on Human Health . J Molecular Structure: THEOCHEM , 458 : 191 – 201 . [CROSSREF]
- Slowik , J. G. , Stainken , K. Davidovits , P. 2004 . Particle Morphology and Density Characterization by Combined Mobility and Aerodynamic Diameter Measurements. Part 2: Application to Combustion-Generated Soot Aerosols as a Function of Fuel Equivalence Ratio . Aerosol Sci. Technol. , 38 : 1206 – 1222 . [CROSSREF]
- Spicer , C. W. , Holdren , M. W. , Riggin , R. M. and Lyon , T. F. 1994 . Chemical Composition and Photochemical Reactivity of Exhaust from Aircraft Turbine Engines . Ann. Geophysicae , 12 : 944 – 955 . [CSA]
- Tang , S. , Johnson , R. , Lanni , T. and Webster , W. 2001 . “ Monitoring of PM-Bound Polycyclic Aromatic Hydrocarbons from Diesel Vehicles by Photoelectricl Aerosol Sensor (PAS) ” . In Society of Automotive Engineers 2001-01-3578
- Wey , C. C. 2004 . Overiew of the Aircraft Particle Emissions Experiment (APEX) Program , Clevland, OH : Aircraft Particle Emissions Workshop .
- Wey , C. C. , Wey , C. Dicki , D. J. 1998 . Engine Gaseous, Aerosol Precursor and Particulate at Simulated Flight Altitude Conditions , National Aeronautics and Space Administration .
- Whitefield , P. D. , Hagen , D. E. Wormhoudt , J. C. 2002 . NASA/QinetiQ Collaborative Program—Final Report , National Aeronautics and Space Administration .
- Wilson , N. K. , Barbour , R. K. , Chuang , J. C. and Mukund , R. 1994 . Evaluation of a Real-Time Monitor for Fine Particle-Bound PAH in air . Polycyclic Aromatic Compounds , 5 : 167 – 174 .
- Yu , K. N. , Cheung , Y. P. , Chueng , T. and Henry , R. C. 2004 . Identifying the Impact of Large Urban Airports on Local Air Quality by Nonparametric Regression . Atmos. Environ. , 38 : 4501 – 4507 . [CROSSREF]