This study focuses on the hygroscopic properties of submicrometer aerosol particles emitted from two small-scale district heating combustion plants (1 and 1.5 MW) burning two types of biomass fuels (moist forest residue and pellets). The hygroscopic particle diameter growth factor (Gf) was measured when taken from a dehydrated to a humidified state for particle diameters between 30–350 nm (dry size) using a Hygroscopic Tandem Differential Mobility Analyzer (H-TDMA). Particles of a certain dry size all showed similar diameter growth and the Gf at RH = 90% for 110/100 nm particles was 1.68 in the 1 MW boiler, and 1.5 in the 1.5 MW boiler. These growth factors are considerably higher in comparison to other combustion aerosol particles such as diesel exhaust, and are the result of the efficient combustion and the high concentration of alkali species in the fuel. The observed water uptake could be explained using the Zdanovski-Stokes-Robinson (ZSR) mixing rule and a chemical composition of potassium salts only, taken from ion chromatography analysis of filter and impactor samples (KCl, K2SO4, and K2CO3). Agglomerated particles collapsed and became more spherical when initially exposed to a moderately high relative humidity. When diluted with hot particle-free air, the fractal-like structures remained intact until humidified in the H-TDMA. A method to estimate the fractal dimension of the agglomerated combustion aerosol and to convert the measured mobility diameter hygroscopic growth to the more useful property volume diameter growth is presented. The fractal dimension was estimated to be ∼ 2.5.
1. INTRODUCTION
The supply of energy is one of the main issues in today's society. Due to the increasing CO2 concentrations in the atmosphere, authorities are promoting the use of renewable fuels such as biomass. In Sweden, for example, biofuels contribute to about 16% (98 TWh) of the total energy supply, increasing by ∼ 4 TWh annually (CitationSwedish Energy Administration 2003). A fraction of the increase is invested in moving grate plants between 0.5 and 10 MW used for local district heating.
While reducing the net CO2 emissions, biomass combustion emits large amounts of fine particles if no particle removal technique is utilized. There is now a general consensus that elevated concentrations of both PM10 and PM2.5 are associated with adverse health effects (CitationWHO 2003; CitationWHO 2004). Although the exact mechanisms of the health effects are not known, chemical composition, size, morphology, and solubility are likely to be important parameters.
Aerosol particles emitted from combustion of biomass can also have an impact on regional and global climate through their influence on the Earth's radiation budget and cloud microphysics. The role of atmospheric aerosols in cloud formation and lifetime along with the resulting global radiation balance currently constitute the largest source of uncertainty in our ability to predict future climate change resulting from human activities (CitationIPCC 2001).
Several studies have been carried out on particle formation and emissions from woody biomass combustion for heat and power production. These range from large-scale (25–100 MW) CFB (Circulating Fluidized Bed) boilers (e.g., CitationValmari et al. 1999), to grate combustion in 0.5–12 MW boilers (CitationPagels et al. 2003; CitationLillieblad et al. 2004) to small (10–20 kW) residential wood stoves and boilers (CitationHedberg et al. 2002; CitationJohansson et al. 2004). Emissions from other biomass fuels have also been studied, such as straw (CitationChristensen et al. 1998) and olive residues (CitationJimenez and Ballester 2004), as well as co-firing of biomass and fossil fuels. Biomass burning in the open resulting from wildfires and deforestation has also attracted attention (CitationReid et al. 2004; CitationAndreae and Merlet 2001).
During favorable combustion, ash components such as K2SO4, KCl, and in some cases K2CO3 dominate the fine particle composition. Potassium is volatilized, and contributes to the formation of particles by homogeneous nucleation and subsequent condensation as the flue gases cool down. The concentration of these ash components is mainly governed by the inorganic content of the fuel. If the combustion conditions are poor—as might be the case in forest fires and during the start-up phase in residential boilers—products of incomplete combustion, for example, Organic Carbon (OC) and Elemental Carbon (EC; i.e., soot) may dominate the chemical composition. The composition of fine particles from biomass combustion covers the full spectrum from almost pure KCl/K2SO4 particles in CFB boilers to almost pure OC/EC particles in forest fires. Although not studied in detail, the relative abundance of these two classes of particle components is expected to vary strongly over the combustion cycle. Some information is available on the variation in inorganic composition on a particle-by-particle basis from electron microscopy EDX analysis (CitationBrunner et al. 2001), but more studies are needed to better understand the mixing status.
Combustion particles may be highly agglomerated. CitationPark et al. (2004) have reported that the dynamic shape factor of diesel soot increased from 1.1 to 2.2 over the size range 50–220 nm. CitationValmari et al. (1999) suggested that fine mode particles from CFB combustion of biomass were compact and close to spherical based on Transmission Electron Microscopy (TEM) analysis.
The aim of this study was to determine the hygroscopic properties of the flue gas particles emitted from biomass fired district heating units, using a Hygroscopic Tandem Differential Mobility Analyzer (H-TDMA). Hygroscopic properties in this study relate to how the particles absorb water vapor and grow into solution droplets as a function of the relative humidity (RH). The hygroscopic properties of aerosol particles are important for several reasons. The deposition pattern and deposition probability in the respiratory system is strongly dependent on the particle size in the actual airways. In the lower respiratory tract, the equilibrium RH is about 99.5% (CitationAnselm et al. 1990). Under these conditions NaCl particles grow by a factor of five to six in diameter. Particle hygroscopic growth is also a crucial parameter for light scattering and for the selection of the sub-population of particles that act as cloud condensation nuclei. Furthermore, measurements of hygroscopic properties can provide size and time-resolved information regarding the mixing status of the aerosol, as well as proxy data on the chemical composition. These properties can be used for a better understanding of particle formation processes and to develop more efficient cleaning techniques.
Water vapor uptake of biomass combustion aerosol particles can be either due to the presence of water-soluble salts or to the presence of water-soluble organic compounds. As an example, pure KCl has a diameter growth factor of about 2 at RH = 90%. The water-soluble OC in biomass smoke consists of partly oxidized compounds originally present in the fuel. These compounds typically have lower growth factors. One abundant compound is levoglucosan—a pyrolysis product of cellulose (CitationSimoneit et al. 1999)—which has a growth factor of about 1.3 at 90% RH (CitationSvenningsson et al. 2005).
Previously, only a few measurements on hygroscopic diameter growth factors of biomass burning aerosols have been reported, some with regard to natural forest fires or clearing forest in the Amazon region (CitationVestin et al. 2004; CitationRissler et al. 2004), laboratory-scale experiments burning peat (CitationZeromskiene, 2004) and emissions to indoor air from wood stoves (CitationDua and Hopke 1996). Field studies of biomass burning smoke aerosol in the Amazon report low hygroscopic growth factors (∼ 1.1–1.3) at 90% RH (CitationVestin et al. 2004). For the laboratory-scale experiment burning peat the hygroscopic diameter growth, measured at 85% RH, was 1.04, corresponding to a growth of ∼ 1.06 at 90% RH (for 80 nm particles). For the indoor small-scale fires, measured at ∼99% RH, the growth was ∼1 for an open flame fire and 2 for particles from a smoldering fire, corresponding to a growth of ∼1–1.35 at 90% RH. The differences in results are likely due to highly variable burning conditions and combustion efficiency. To our knowledge, no measurements of hygroscopic diameter growth factors have been reported from combustion in biomass fired district heating, where the combustion conditions are favorable and water-soluble potassium salts tend to dominate the particle composition.
In this study, hygroscopic growth factor measurements are presented from two biomass fired moving grate boilers. The Zdanovski-Stokes-Robinson (ZSR) method was used to predict hygroscopic growth factors from chemical composition data partly reported previously (CitationPagels et al. 2003; CitationWierzbicka et al. 2005). A method is described that can be used to estimate the fractal dimension of agglomerated hygroscopic combustion aerosol and to convert the measured mobility hygroscopic growth factor to the more useful property volume growth factor. The study is a part of a larger national project (biofuels, health, and environment—emissions and air quality, CitationNoone 2005), which aims to study the effects on environment and human health from increasd utilization of biofuels combustion for energy production.
2. METHODS AND THEORY
2.1 Combustion System and Particle Sampling
The measurements were carried out in two biomass fired moving grate boilers used for local heat production in Sweden. The two boilers are a 1 MW boiler operating on moist forest residues (boiler 1) and a 1.5 MW boiler fired with dry pellets (boiler 2). During the measurements the two boilers were operated at 45–100% and 20–50% load, respectively.
The flue gas was sampled isokinetically downstream the multi-cyclone (the only flue gas cleaning device installed). Two different dilution systems were in use. For boiler 1 the flue gases were diluted (1:10) and cooled to room temperature using particle-free dry compressed air at ambient temperature. In this system, the flue gas sample experienced a high RH during dilution, which may to some extent simulate the dilution in and after the stack. Hygroscopic particles which go into water solution during dilution may recrystallize to a more compact shape. The dew point of the undiluted flue gas is around 60°C. The cooled gas stream was further diluted (1:10) before being sampled with the H-TDMA.
For boiler 2, a two-stage ejector based dilution system was used. The first dilution stage used heated (110°C), particle-free air. This type of dilution system is often used in biomass combustion studies and prevents condensation of water and volatile components on the particles.
The boilers and the sampling systems are described in more detail by CitationPagels et al. (2003) and CitationWierzbicka et al. (2005). All reported concentrations are normalized to 13% CO2, at 0°C and 101.3 kPa.
2.2 Hygroscopic Growth Measurements
2.2.1 Principle of the H-TDMA
The hygroscopic properties of aerosol particles, with dry sizes ranging between 20 and 350 nm, were studied with an H-TDMA. In principle the H-TDMA consists of three parts: a Differential Mobility Analyzer (DMA1) that selects a narrow, quasi-monodisperse size fraction of dry particles (RH < 10%); humidifiers conditioning the aerosol to a controlled humidified state; and a second DMA (DMA2) that measures the change in size caused by the imposed humidification. The aerosol and the sheath flows entering DMA2 were humidified separately. The DMAs used were of the Vienna type (CitationWinklmayr et al. 1991).
The H-TDMA was operated in two different modes: (1) the size-scanning mode and (2) the RH-scanning mode. Using mode 1, humidity is kept constant while the selected dry size in DMA1 is varied. This gives information on how the hygroscopic diameter growth of the aerosol changes with particle size. Mode 2, instead, keeps the selected dry size constant while scanning the RH, here between 20–90%. This can be used to evaluate the deliquescent behavior of the aerosol particles. A more detailed technical description of the H-TDMA can be found in CitationSvenningsson (1997) and CitationZhou (2001).
2.2.2 Quality Assurance and Data Processing
The H-TDMA data were evaluated and assured for quality off-line, using a number of parameters logged by the H-TDMA software. An extensive description of the quality assurance procedure is given by CitationRissler et al. (2004) and CitationSwietlicki et al. (2000). Certain criteria on the variations in concentration, RH and temperatures had to be fulfilled for data to be accepted. These criteria were used when calculating the instrumental error in .
Each spectrum of the humidified aerosol size distribution was fitted with a computer program (CitationZhou 2001) based on the theory and algorithm of “TDMAFIT” (CitationStolzenberg and McMurry 1988). This program estimates the arithmetic mean mobility diameter growth factor, Gf B (defined as the ratio between the conditioned mobility diameter d B,wet , and the dry mobility diameter, d B,dry ), the diameter growth dispersion factor (the broadening in addition to the ideal TDMA transfer function), and the number fraction of particles in each fitted hygroscopic particle group. For distributions fitted with two or more groups, the mean growth of the groups was calculated and presented as one Gf B . To present the broadening, which is related to the mixing status of the particles, an interval of the hygroscopic growth factor covering 90% of the wet distribution (fitted distribution) is given. This representation of the spectra were chosen since the fitted distributions were most often very close in growth and mainly dominated by one mode.
Hereafter we will distinguish between the mobility diameter growth factor, Gf B , and the volume diameter growth factor, Gf ve . Since wet particles are spherical, d B,wet = d ve, wet (d ve is the volume equivalent diameter) the difference between Gf B and Gf ve is introduced due to non-spherical dry particles, i.e. d B,dry ≠ d ve, dry .
Despite considerable efforts to stabilize the RH in DMA2, deviations from the nominal RH are difficult to avoid during operation. To facilitate comparisons, the growth factors of the size scans were corrected to the nominal RH of 90%. Corrections were made as described by CitationSwietlicki et al. (2000) using KCl (CitationTang 1997) as model salt. Since the accepted variation in DMA2 RH is relatively small, the dependence on the type of salt applied in this correction is negligible.
2.3 Additional Measurements
A Scanning Mobility Particle Sizer (CitationWang and Flagan 1989) was operated in parallel with the H-TDMA during the two experiments. In addition, a Dekati 13 stage multi-jet low-pressure cascade impactor (LPI) was used to collect samples for gravimetric analysis, elemental analysis (PIXE), and analysis of major ions (IC). An Electrical Low-Pressure Impactor (ELPI) (CitationMarjamäki et al. 2000) was used in the second boiler. These measurements are described in more detail by CitationPagels et al. (2003), CitationLillieblad et al. (2004) and CitationWierzbicka et al. (2005). In this article, some additional analysis is presented to identify the time and size variation of ash components like potassium salts and EC/OC. Such information is essential when interpreting the H-TDMA measurements.
2.4 Hygroscopic Growth and the ZSR Mixing Rule
The water uptake of a particle can be described using the Köhler equation (CitationKöhler 1936). It combines the Kelvin curvature effect (C k ) and Raoult's Law for water activity (a w ) as RH/100 = C k · a w . C k takes into account the increase in equilibrium water vapor pressure caused by the droplet curvature, and the second term describes the depression in water vapor pressure due to soluble material in the particles.
2.4.1 The Zdanovski-Stokes-Robinson (ZSR) Method
A relatively simple way of estimating Gf ve of mixed particles is to use the Zdanovski-Stokes-Robinson (ZSR) method (CitationStokes and Robinson 1966). The ZSR method is defined by the equation:
2.4.2 Water Activities of the Pure Salts
To calculate the hygroscopic diameter growth factor using the ZSR method, Gf ve of the pure compounds needs to be known. The main components of the biomass combustion aerosol studied were K2SO4, K2CO3, and KCl. Since no data were found for the hygroscopic growth of K2CO3, this salt was further investigated in laboratory H-TDMA measurements. KCl has been characterized by CitationTang (1997). He measured the water activity (a w ) down to the point of crystallization (a w = 0.62−1). The deliquescence point is for KCl ∼ 0.84 (CitationTang 1997), and the measured deliquescence point for K2CO3 is between 0.30–0.40 (when estimated from solubility: 0.54). For K2SO4, water activity data from CitationGuendouzi et al. (2003) were used. However, they only measured the water activity for non-supersaturated (bulk) solutions. No water activity data were found for supersaturated solutions of K2SO4. Since the solubility of K2SO4 is low (0.12 g/cm3, from Lide 1991), resulting in a high deliquescence point, a w ∼ 0.97, it is impossible to measure the deliquescent branch using our H-TDMA system. In order to estimate the hygroscopic growth below the point of deliquescence, we use the fact that the hygroscopic growth for particles consisting of non-ideal subsaturated solutions can be approximated from classical Köhler theory (CitationKöhler 1936), substituting the number of dissociating ions by a constant van't Hoff factor. As a result of the variable degree of dissociation and non-ideal interactions in the electrolyte, the van't Hoff factor varies with molality. In the interval measured by CitationGuendouzi et al. (2003) (a w = 0.972–0.9958), a van't Hoff factor of 2.05 can describe the measured water activity. This value was inserted into Köhler theory to extrapolate the hygroscopic behavior of K2SO4 down to supersaturated solutions. The errors introduced using a static van't Hoff factor are, for our purpose, small.
2.4.3 Solubility
The water solubility of the pure compounds must be taken into account when using the ZSR mixing rule. This is the case for K2SO4 and partly also for KCl. In CitationSvenningsson et al. (2005) it was assumed that the constituents of the particle go partly into solution if other salts provide the water liquid phase, an assumption also used in this study. The water accessible for the solution is assumed to be V droplet -V non−sol.frac , where V droplet is the droplet volume and V non − sol.frac is the volume of the not yet dissolved fraction of the particle. The solubilities in mixed particles were calculated from the solubility products, K sp , and take into account the common ion effect (CitationMcMurry and Fay 1998). The solubility products used were in turn calculated from the solubility of the pure salts taken from CitationLide (1991). The solubilities of the pure compounds (and the solubility products) are 1.12 g/cm3 (2129 mol3/dm9) for K2CO3, 0.344 g/cm3 (21.3 mol2/dm6) for KCl, and 0.12 g/cm3 (1.31 mol3/dm9) for K2SO4.
2.5 Non-Spherical Particles and Fractal Dimension
When dealing with freshly emitted combustion aerosols, the particles can be agglomerated. For agglomerated particles formed by coagulation, the mass-fractal dimension, Df, is often used to describe the shape of the particles. Df is a parameter linking radius (length scale) and mass of the population of particles over a certain size range, defined as
The mass of an aerosol particle can also be expressed as
The mass of the agglomerated particles is scaled by d B raised to Df (< 3) instead of 3. Note that larger particles in a population have a more open structure than smaller ones, and consequently higher dynamic shape factors.
3. RESULTS AND DISCUSSION
3.1 Time and Size-Resolved Analysis of Concentrations
Size distribution data from the two boilers have been described in more detail previously (CitationPagels et al. 2003; CitationWierzbicka et al. 2005). The total number concentration of emitted particles was 5.1 · 107 and 6.3 · 107 particles/cm3 for 50 and 100% load, respectively, in boiler 1 and 2.9 · 107 particles/cm3 for a 30% load in boiler 2. Size distributions were essentially unimodal in the measured size range (15–300 nm). The geometrical mean diameters of the fitted lognormal size distributions were 117, 90, and 77 nm. The size distributions were, in principle, stable over time, with the exception of the largest particles in boiler 2. In , the relative standard deviation (coefficient of variation) over time of the concentration in each SMPS size channel is given. Only size channels for which the variations due to counting statistics were less than 20% of the total standard deviation were included in . The relative standard deviation is significantly larger than 0.3 only for particles larger than 150 nm in boiler 2.
Figure 1 Relative standard deviation over time for each size channel, according to the SMPS measurements. Only size channels in which counting statistics contributed to less than 20% of the given variability were included.
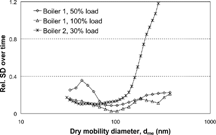
The time variations in boiler 2 were studied with higher time-resolution using the ELPI. These measurements also showed a strong increase in temporal variation with increasing particle diameter. Often there was a clear periodicity in the time variations, typically on the order of 1 min, caused by grate movements.
3.2 Size-Resolved Chemical Composition
Size-dependent information about the chemical composition is needed for comparison and interpretation of the hygroscopic properties measured on-line with the H-TDMA. Water-soluble ions detected by ion chromatography are given in as ion equivalent concentrations. The dominating ions were K+, SO4 2−, CO3 2−, and Cl− in boiler 1 and K+, SO4 2− and Cl− in boiler 2. The fraction of K2CO3 was estimated from an excess of positive ions found in the IC analyses (CitationPagels et al. 2003). More samples were analyzed with PIXE compared to IC. Also, the detection limit is lower, and thus, the variations in inorganic composition with particle size could be studied in more detail. The sulfur to chlorine mass ratio increased with particle size in boiler 1, while in boiler 2 it was essentially independent of particle diameter (). The size-dependence in the S/Cl ratio seen in boiler 1 was possibly because KCl condenses later in the combustion process compared to K2SO4, and then mostly influences the composition of the smallest particles. The reason for the difference between boiler 1 and 2 is not known. We used the detected composition from IC as input to predict the hygroscopic growth with the ZSR rule. For boiler 1 a sensitivity test of the hygroscopic growth due to the size-dependent S/Cl ratio was made. The ratios were taken from the PIXE measurements.
Figure 2 The result from IC analysis of LPI samples and filter samples (stacked filter unit, CitationPagels et al. 2003). Each bar represents an average value taken of n filters. The geometrical mean diameter of particles given in each impactor stage is given in (nm).
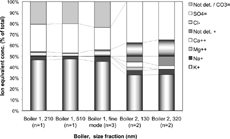
The impactor substrates were first gravimetrically analyzed and then analyzed for IC. The mass fraction determined with IC for boiler 2, decreased from 0.7 in stages 2 and 3 (77 and 133 nm) to 0.2–0.3 in stages 5 and 6 (323 and 510 nm). The detected fractions for both PIXE and IC increased to 0.50 for particles larger than 700 nm. However, neither PIXE nor IC can detect carbon. CitationWierzbicka et al. (2005) analyzed PM1 samples for organic (OC) and elemental carbon (EC) in boiler 2 during the same measurement campaign. They found that OC and EC contributed, on average, to 8 and 34% of PM1, respectively. The EC concentration varied by a factor of three in four different samples. The estimated SMPS mass concentration (assuming constant effective density) in the range 250–320 nm was highly correlated (r2 = 0.99) with the EC concentration in individual samples. The correlation was significantly lower for 150–250 nm (0.66) and 25–150 nm particles (−0.28).
From the combined results of the different measurement techniques it was inferred that EC is enriched in particles between 150 and 700 nm. This is also in line with the observed fast temporal variations in concentrations observed with ELPI and SMPS for particles larger than 150 nm. Ash components (e.g., potassium) continuously evaporate and form smaller particles for which the temporal variations were small in both boilers.
In boiler 1, temporal variations were low for particle diameters between 150 and 400 nm. The total PAH concentration (particle and gas phase) of 29 analyzed compounds (CitationLillieblad et al. 2004) was very low (2.8 μ g/m3). Therefore, we expect the OC/EC contribution in boiler 1 to be small.
3.3 Hygroscopic Properties, Boiler 1
3.3.1 RH Scan
In the 1 MW unit RH scans were performed for 120 and 200 nm particles at around 50% boiler load. The measured Gf B , is presented as a function of water activity in . The particles started to take up water at low water activities and no clear deliquescent point was seen. The absence of a deliquescent point is not surprising since aerosol particles consisting of a mixture of many compounds, such as atmospheric aerosols, often display a continuous hygroscopic growth without deliquescence steps. Since the measurements do not show any indication of fractal-like behavior of the investigated particles within the H-TMDA, d B ≈ d ve and consequently Gf B ≈ Gf ve .
Figure 4 Diameter growth factor for the RH scans performed in boiler 1 together with calculated diameter growth factor according to the ZSR mixing rule. In the model, chemical composition based on IC analysis of filter samples was used. The growth of the pure compounds is also shown.
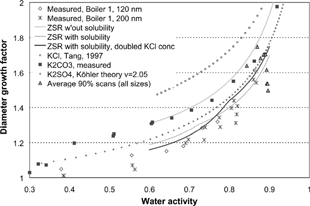
The IC analysis of the submicrometer flue gas particles showed the fine mode particles to mainly consist of KCl, K2SO4, and K2CO3. For the ZSR predictions we used a chemical composition of these compounds only, in ratios according to the IC analysis of filter samples collected in parallel with the RH scans. The composition used was in (dry) volume fractions 0.14, 0.44, and 0.42 ().
Not taking solubility into account, the ZSR model predicts a water uptake that exceeds that observed with the H-TDMA (). Comparing the slope of the experimental a w curve to that of the pure compounds, it can be seen that some of the particles constituents most likely go into solution as a w increases. Taking into account the low solubilities of K2SO4, and KCl in the ZSR prediction, the assumed mixture can reproduce the hygroscopic behavior of the flue gas particles reasonably well. Since it could be seen from the PIXE analysis that the sulfur to chloride ratio was changing as a function of size (), a calculation was also performed doubling the fraction of KCl at the cost of K2CO3. The result is then even closer to the measured hygroscopic growth. KCl here goes completely into solution at a water activity of ∼ 0.80, which can be discerned as a slight discontinuity in the calculated ZSR curve in . Only 10% of the K2SO4 is in solution at a w ∼ 0.9.
For the time periods scanning in the RH mode, the hygroscopic behavior could thus be explained with the ZSR rule using only the inorganic compounds as main components of the particles. From the RH scans, no obvious difference in Gf ve was seen between the 120 and 200 nm particles.
3.3.2 Size Scan
The hygroscopic behavior was studied as a function of dry particle diameter using the size scanning mode of the H-TDMA, at 90% RH. A rather constant growth was observed, with slightly decreasing growth factors with increasing size, shown in and . During the scans, the boiler load was either 50 or 100%. A trend with somewhat higher hygroscopic growth at lower load was also observed.
Table 1 Hygroscopic properties of particles sampled in boiler 1 and 2
Figure 5 The hygroscopic diameter growth factor, individual scans, and average values. The time series for boiler 1 is divided into high and low load. Error bars correspond to the estimated instrumental error. The larger error bars in boiler 1 are due to a larger uncertainty in RH during this measurement.
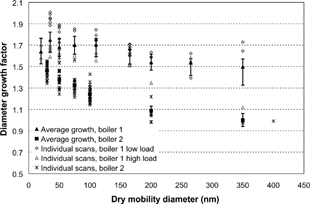
The average hygroscopic growth factors at 90% RH are also plotted in , here recalculated into water activity. Consequently, the higher activities in the figure correspond to the growth of the larger particles. The PIXE analysis showed that the sulfur to chlorine mass ratio increased with size (), indicating that the smaller particles had a slightly different chemical composition (e.g., a larger fraction of KCl). Using the ZSR method and taking limited solubility into account, an increased fraction of KCl at 90% RH would result in higher hygroscopic growth factors, which is in agreement with this observation.
Overall, we can conclude that there was no larger fraction of elemental or insoluble organic carbon present, except from possibly in the larger sized particles. This is in agreement with the results presented in section 3.2.
3.3.3 Internal Mixture and Temporal Variation
For the 1 MW boiler, the humidified size distribution mainly revealed unimodal growth. When multiple modes were present, they were close in growth. The variation of the hygroscopic growth within each scan is presented as the interval in Gf ve that covers 90% of each spectrum. For 110 nm particles the interval was ±0.12, to be compared to that of pure ammonium sulfate (similar growth factor), which is ±0.06. That means the broadening of each humidified size distribution was slightly larger than if the particles of one scan were perfectly internally mixed (i.e., all individual particles had similar hygroscopic growth). This suggests that the processes of nucleation, condensation and coagulation create an internally well-mixed aerosol, or that compounds of similar hygroscopic growth composed the particles. An example of a humidified size distribution at ∼ 90% RH of 100 nm particles is shown in . The average spread in growth factor at each dry diameter is presented together with the average growth in .
Figure 6 Example of typical humidified size distributions measured in DMA2. The size distributions were often relatively narrow but occasionally a bimodal hygroscopic growth appeared indicating a clearly external mixture. The selected dry sizes were 110 nm for boiler 1 and 100 nm for boiler 2, indicated with a bar in the figure.
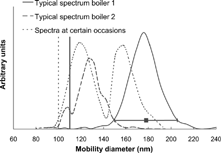
The standard deviation of Gf ve in time, presented in , indicates variations in the combustion process in time (leading to a slight variation in chemical composition), especially for the smallest and the largest particles.
3.4 Hygroscopic Properties, Boiler 2
During the measurements in boiler 2 (1.5 MW pellets boiler) the load was more stable than for boiler 1, typically around 30%. The number of scans was higher. Since the time resolution of one H-TDMA scan was ∼ 3–4 minutes, the fast events with high concentrations of EC were largely sorted out with the quality assurance procedure and no details of mixing status of single particles at these events could be determined.
3.4.1 RH Scan
For boiler 2, RH scans were made for 100, 200, and 300 nm particles. The concentrations were low and changed rapidly in the measurements for 200 and 300 nm particles. Therefore only a few spectra passed the quality criteria.
For the 100 nm particles the RH scans showed no hygroscopic growth up to ∼ 0.5 in a w . At a w around 0.5 and up to 0.78, the humidified mobility diameter decreased below the dry value (), with a minimum in Gf B at a w ∼ 0.73. For a w higher than 0.8 the hygroscopic growth factor was > 1.
Figure 7 RH scans for boiler 2, original data (Gf B ) as well as data corrected with respect to fractal structure (Gf ve ). The calculations made by the ZSR mixing rule are based on chemical composition from IC analysis of filter samples.
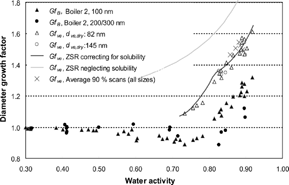
Since d B of agglomerated particles is larger than d ve , the “shrinking” behavior between a w 0.5 and 0.78 could be explained by restructuring of agglomerated particles into a more spherical shape while taking up only small amounts of water, when humidified within the H-TDMA. For agglomerate particles Gf B < Gf ve , and the approximation Gf B ≈ Gf ve would lead to a substantial underestimation of the water uptake. Since the particles tend to show a decrease in mobility diameter until a w ∼ 0.73; the particles below this water activity are not yet spherical (i.e., not liquid droplets). At higher water activities the Gf B starts to increase, indicating that the particles above this a w are likely to be liquid spherical droplets.
For 200 and 300 nm particles the number of accepted scans was low, as was the hygroscopic growth factor. However, these particles followed the same trend, with a somewhat stronger reshaping behavior (). This is consistent with larger particles of a population having a more open structure (section 2.5). The reason for the hygroscopic growth below the deliquescence point of KCl can be explained by the inverse Kelvin curvature effect or the presence of other compounds, not contributing much to the growth, but still providing a small liquid phase. Previously it has been reported that agglomerate spark-discharge carbon particles, and to a smaller extent diesel soot particles, restructure to more compact particles at RH below the deliquescent point due to the inverse Kelvin effect (CitationWeingartner et al. 1997).
The ZSR method was also applied to the boiler 2 measurements. The chemical composition used in the calculations was in volume fractions 0.70 K2SO4 and 0.30 KCl estimated from the IC analysis (chemical composition taken from the impactor stage with geometric mean aerodynamic diameter of 130 nm, in ). However, for a meaningful comparison between Gf ve calculated with the ZSR method and experimental data, the measured Gf B has to be converted to Gf ve taking the particle agglomerate structure into account. This was done using a fractal dimension, Df of 2.49 and C 3 = 0.563 (Equation Equation6). The estimation of Df and C 3 was made from the size scans as described in section 3.5.
Taking into account the limited solubility of the salts, the ZSR method can reproduce the measured growth well, as shown in . KCl is completely in solution at 0.83 a w . Due to the limited solubility of K2SO4 only 6% of K2SO4 is in solution at a w ∼ 0.9.
The RH scans performed for particles from boiler 2 clearly showed a reshaping behavior while this was not seen for particles from boiler 1. The difference can be attributed to the dilution system. For boiler 2, the primary diluting air was heated, and the RH was kept low until humidification within the H-TDMA.
3.4.2 Size Scan
Scanning DMA 1 in size at 90% RH revealed a strong decrease in Gf B from 1.46 for the 30 nm particles to 0.99 for the 350 nm particles. This decrease was observed despite the fact that the chemical composition obtained from IC analysis and the S/Cl ratio obtained from PIXE data was essentially independent of particle diameter. For particles larger than 150 nm, the decreasing growth might have been affected by the presence of EC (discussed in sections 3.1 and 3.2). For particles 100 nm and smaller the size-dependent growth is likely to be caused by the fractal-like shape of the particles. It follows from the definition of fractal dimension that larger particles have a more open structure (higher dynamic shape factors) and consequently, the observed reduction in Gf B as a function of size is explained by the increasing ratio of d B and d ve as a function of size.
3.4.3 Internal Mixture and Temporal Variation
The humidified size distribution in boiler 2 was in general narrow with a slight modal structure. On some occasions, often in connection with higher loads, a clearly distinguished modal structure was seen—generally clearer for the smaller sized particles. This means that the aerosol on these occasions was externally mixed, i.e., particles of the same size had different chemical composition (or morphology). Examples of humidified distributions for 100 nm particles at ∼ 90% RH are given in .
The temporal variation of the measured hygroscopic growth was prominent, but still smaller than for boiler 1. This may be explained by smaller load changes or the use of the more homogeneous pellets fuel in boiler 2. The variation in time is presented as standard deviation of the growth factor in .
3.5 Fractal Dimension from H-TDMA Measurements
Often when calculating the volume equivalent diameter growth factor (Gf ve ), d ve, dry is approximated by the measured d B,dry (i.e. Gf B ≈ Gf ve ). In the case of processed atmospheric aerosols, this approximation most often only introduces minor errors. However, for agglomerated particles, such as those measured here, the errors introduced are large. In order to correctly calculate Gf ve for agglomerated aerosol particles that can be described by a fractal dimension, the relation in Equation Equation5 is used, and the hygroscopic growth factor can be calculated according to:
Assuming that the chemical composition is constant over the considered size range, then for a constant a w , Gf ve is also constant over this size range. Rearranging Equation Equation6, an expression by which Df can be determined from the relative change in Gf B over dry size can be derived:
This equation is valid when the fractal dimension Df is constant over the size interval in question, and for a hygroscopic aerosol so that the particles are spherical at the a w used. Note that Df determined with Equation Equation7 does not depend on the absolute Gf ve . For a particle population with a fractal dimension lower than 3 and chemical composition constant over size, the relation between Gf B and Gf ve (i.e., the absolute Gf ve ) is determined by the primary particle size (which affects the value of C 3 in Equation Equation6).
The size scans performed during this study were made at 90% RH, corresponding to an a w ranging from 0.86 for the smallest particles, to 0.89 for the largest. Correcting the measured Gf B , to a constant a w (0.88, the average in the interval of interest) a weak dependence on assumed primary particles size is introduced. From the H-TDMA measurements, we see that the primary particle size is smaller or equal to 30 nm. In the correction to a constant water activity, a primary particle size of 30 nm was used. The sensitivity to this was investigated using a primary particles size of 10 nm. The correction to a w = 0.88 was made as described in the methods section using KCl as a model compound.
The estimation of fractal dimension from H-TDMA data was made using least squares regression analysis, fitting Df to get a constant Gf ve as a function of size. The fit was performed in the range 30–100 nm since constant chemical composition over size was restricted to apply only to particles smaller than 150 nm (section 3.2). The fractal dimension was estimated to be 2.49 (2.54 not including the 100 nm particles). Using a primary particle size of 10 nm decreased the fractal dimension by 0.02.
As discussed, the fitted fractal dimension only weakly depends on the absolute Gf ve . However, to determine Gf ve we have to assume a primary particle size. The lower limit of Gf ve is set by the growth of the 30 nm particles. Assuming spherical 30 nm particles, C 3 was fitted to 0.563 (0.592 using 30–75 nm). Inserting C 3 and Df in Equation Equation6, Gf ve was calculated and is shown together with Gf B in . It is worth noting that if the 30 nm particles also had an agglomerated structure, the real water uptake would be underestimated and our estimate of Gf ve would be a lower limit. The estimated Df and C 3 result in dynamic shape factors (CitationHinds 1999) of 1.42, 1.65, and 1.84 (mobility diameters) for 100, 200, and 300 nm particles, respectively. In it can be seen that when the larger particles (> 150 nm) were corrected to the same fractal dimension as particles < 150 nm in diameter, these larger particles had a somewhat lower Gf ve than expected if the chemical composition was constant over size; not as low, however, as for an aerosol dominated by soot. This is either because the intermittent events with high soot concentration are sorted out by the quality assurance procedure (restrictions in the variation in concentration during one scan) or due to a different fractal behavior of these particles.
Figure 8 Size scans, boiler 2, showing both Gf B and Gf ve , calculated taking the fractal-like shape into account (Df =2.49, C 3 = 0.563). The solid line shows the theoretical Gf ve of a compound having a constant chemical composition over size.
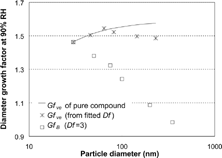
The estimated Df and C 3 were also applied to the RH scans, to recalculate the hygroscopic growth. The recalculated growth was used in the comparison with the result from the ZSR method. The agreement was good when using the recalculated growth (see previous section and ) indicating that the primary particle size may be close to 30 nm.
Recently De CitationCarlo et al. (2004) distinguished between two different values of the fractal dimension in a single aerosol population, depending on the number of primary particles in the agglomerate. The fractal dimension was 2.46 for fewer primary particles than 60 and 1.79 for larger agglomerates. For diesel soot with a primary particle size of 33 nm, the break point between the two regions was around 165 nm. The value for agglomerates of less than 60 primary particles is in agreement with our estimation for 30–100 nm particles (2.49). We also found a lower fractal dimension for larger particles (2.37) in the size range 100–300 nm.
CitationPagels (2005) varied the temperature in a similar ejector sampling system in a larger boiler operated with moist forest residue. It was found that dried particle samples decreased their mobility diameter on average 20% when the particles experienced high RH during sampling. Larger particles shrank more than smaller ones. The fractal dimension of dry sampled particles was estimated to be 2.5–2.6 (and that of humid sampled particles 3.0–3.1) with the SMPS-ELPI distribution fitting method. In that study the elemental carbon concentration was negligible. A partial restructuring process occurred at temperatures above 400°C in experiments incorporating a thermodesorber.
In previous electron microscopy analyses (CitationValmari et al. 1999) it was concluded that particles from CFB combustion are spherical or compact. This was explained by transformation through condensation in preference to coagulation. The agglomerated structure found in in-situ measurements suggests that coagulation is a strong transformation process and that the nucleation rate and the initial number concentrations are higher in the moving grate boiler than what would be the case for an aerosol mainly formed by condensation. In-situ measurements performed on airborne particles avoid uncertainties caused by sample storage or exposure to the electron beam in an electron microscope. CitationKrämer et al. (2000) used an H-TDMA to show that NaCl particles generated from the gas phase in a laminar flow reactor were highly agglomerated and that even at an RH significantly below the deliquescent point, particle compaction occurred (NaCl has a vapor pressure similar to KCl). Dynamic shape factors assessed from mobility changes and hygroscopic growth models were in the order of 4–5.
4. SUMMARY AND CONCLUSIONS
The flue gas particles emitted from two biomass combustion units had, in contrast to other combustion aerosols such as diesel exhaust particles, relatively high hygroscopic growth factors. This is explained by the particle composition dominated by potassium salts (KCl, K2SO4, and K2CO3). The average volume equivalent diameter growth factor at RH = 90%, for 110/100 nm particles, was 1.68 in boiler 1 and ∼ 1.5 (uncorrected growth 1.24) in boiler 2. For both boilers the particles were internally well-mixed, except on some occasions in boiler 2, when a second more hygroscopic mode appeared. The temporal variation in growth factor for the 110/100 nm particles was 0.10 (1 std. dev.) and 0.07 for boilers 1 and 2, respectively.
The volume equivalent diameter growth factor could be reproduced surprisingly well by the modeled growth using the ZSR method, where the chemical composition was assumed on the basis of IC and only inorganic ions were considered. The consistency between measured and modeled hygroscopic growth was found for both boilers.
Fast temporal variations in the size spectrometer (both ELPI and SMPS) measurements identified as EC enriched particles were observed for particles larger than 150 nm in the 1.5 MW boiler. These observations were consistent with filter measurements of EC/OC published previously (CitationWierzbicka et al. 2005) and size-resolved recovered mass fractions from ion chromatography. The mixing status at these occasions is not known since events with fast variations were sorted out in the H-TMDA quality procedure.
In boiler 2 the measured Gf B decreased strongly with increasing particle size, while the chemical composition was largely independent for particle sizes in the range 30–100 nm. Furthermore, 100 nm particles decreased their mobility diameter by around 10% at RH∼ 75% before growing to solution droplets. These results were attributed to particles changing the morphology from agglomerated structures to droplets when humidified, leading to an underestimation of the water uptake if assuming Gf B = Gf ve . A method was developed to estimate and correct for the fractal dimension of hygroscopic agglomerated particles. From our H-TDMA data, the fractal dimension was estimated to ∼ 2.5, which is somewhat higher than the value of 2.35 for diesel engines (CitationPark et al. 2004), indicating more compact particles. No indication of agglomerate particles shape was seen for boiler 1. This was attributed to a different sampling system used, where the particles experienced high RH already during sampling.
Since the solubility of K2SO4, a salt often formed in biomass combustion, is limited, it is important to take the low solubility into account when extrapolating the hygroscopic growth from water vapor subsaturations to supersaturations relevant for cloud processes or the humidity in our lungs. Estimating the number of soluble ions from the hygroscopic growth at 90% RH would lead to a significant underestimation of the hygroscopic growth, since at 90% RH only ∼ 60% (boiler 1) and 35% (boiler 2) of the particle was in solution even though the soluble volume fraction was close to 1. This is, however, most likely only a problem when dealing with an aerosol constituted of only a few compounds and of low solubility.
The environmental importance of the observation with high hygroscopic growth of the flue gas particles is mainly related to the atmospheric residence time of the emitted particles. Hygroscopic particles are more easily incorporated in cloud and raindroplets, leading to a higher probability of wet deposition and thus a shorter residence time in the atmosphere, compared to a hydrophobic particle of the same dry size. From a human health aspect, the deposition pattern of the particles in the humid environment of the human respiratory system (RH close to 99.5%) is strongly affected by the hygroscopic properties of the inhaled aerosol particles. The growth in diameter inside the lungs for 100 nm particles from both boilers would be ∼ 4.5 leading to an increase in volume of 90 times (assuming ideal solutions). For the number size distribution, a reduction in total respiratory deposition of a factor of 2–3 is obtained for the aerosols considered in this paper according to the ICRP model, by assuming RH = 99.5% throughout the respiratory tract.
Acknowledgments
The financial support from the Swedish Energy Agency under contract nr 21848-1 is gratefully acknowledged.
REFERENCES
- Andreae , M. O. and Merlet , P. 2001 . Emissions of Trace Gases and Aerosols from Biomass Burning . Global Biogeochem. Cycles , 15 : 955 – 966 . [CSA]
- Anselm , A. , Heibel , T. , Gebhart , J. and Ferron , G. 1990 . In-vivo Studies of Growth-Factors of Sodium-Chloride Particles in the Human Respiratory-Tract . J. Aerosol Sci. , 21 : S427 – S430 . [CSA] [CROSSREF]
- Brunner , T. , Obernberger , I. , Jöller , M. , Arich , A. and Pölt , P. 2001 . “ Behavior of Ash Forming Compounds in Biomass Furnaces—Measurement and Analysis of Aerosols Formed during Fixed-bed Biomass Combustion ” . In Aerosols from Biomass Combustion Edited by: Nussbaumer , T. 75 Verenum, , Switzerland
- Christensen , K. A. , Stenholm , M. and Livbjerg , H. 1998 . The Formation of Submicron Aerosol Particles, HCl and SO2 in Straw-fired Boilers . J. Aerosol Sci. , 29 : 421 – 444 . [CSA] [CROSSREF]
- DeCarlo , P. F. , Slowik , J. G. , Worsnop , D. R. , Davidovits , P. and Jimenez , J. L. 2004 . Particle Morphology and Density Characterization by Combined Mobility and Aerodynamic Diameter Measurements. Part 1: Theory . Aerosol Sci. Technol. , 38 : 1185 – 1205 . [CSA] [CROSSREF]
- Dua , S. K. and Hopke , P. K. 1996 . Hygroscopic Growth of Assorted Indoor Aerosols . Aerosol Sci. Technol. , 24 : 151 – 160 . [CSA]
- Guendouzi , M. E. L. , Mounir , A. and Dinane , A. 2003 . Water Activity, Osmotic and Activity Coefficients of Aqueous Solutions of Li2SO4, Na2SO4, K2SO4, (NH4)2SO4, MgSO4, MnSO4, NiSO4, CuSO4 and ZnSO4 at T = 198.15 K . J. Chem. Thermodynamics , 35 : 209 – 220 . [CSA] [CROSSREF]
- Hedberg , E. , Kristensson , A. , Ohlsson , M. , Johansson , C. , Johansson , P.-Å. , Swietlicki , E. , Vesely , V. , Wideqvist , U. and Westerholm , R. 2002 . Chemical and Physical Characterization of Emissions from Birch Wood Combustion in a Woodstove . Atmos. Environ. , 36 : 4823 – 4837 . [CSA] [CROSSREF]
- Hinds , W. C. 1999 . Aerosol Technology: Properties, Behaviour and Measurement of Airborne Particles , 2nd ed. , New York : John Wiley & Sons, Inc. .
- IPCC (Intergovernmental Panel on Climate Change) . 2001 . Third Assessment Report, Scientific Basis
- Jimenez , S. and Ballester , J. 2004 . Formation and Emission of Submicron Particles in Pulverized Olive Residue (orujillo) Combustion . Aerosol Sci. Technol. , 38 : 707 – 723 . [CSA] [CROSSREF]
- Johansson , L. S. , Leckner , B. , Gustavsson , L. , Cooper , D. , Tullin , C. and Potter , A. 2004 . Emission Characteristics of Modern and Old-Type Residential Boilers Fired with Wood Logs and Wood Pellets . Atmos. Environ. , 38 : 4183 – 4195 . [CSA] [CROSSREF]
- Krämer , L. , Pöschl , U. and Niessner , R. 2000 . Microstructural Rearrangement of Sodium Chloride Condensation Aerosol Particles on Interaction with Water Vapor . J. Aerosol Sci. , 31 : 673 – 685 . [CSA] [CROSSREF]
- Köhler , H. 1936 . The Nucleus in and the Growth of Hygroscopic Droplets . Trans. Faraday. Soc. , 32 : 1152 – 1161 . [CSA] [CROSSREF]
- Lide , D. R. E. 1991 . Handbook of Chemistry and Physics , 72nd edition , Boston : CRC Press .
- Lillieblad , L. , Szpila , A. , Strand , M. , Pagels , J. , Rupar-Gadd , K. , Gudmundsson , A. , Swietlicki , E. , Bohgard , M. and Sanati , M. 2004 . Boiler Operation Influence on the Emissions of Submicrometer-Sized Particles and Polycyclic Aromatic Hydrocarbons from Biomass-Fired Grate Boilers . Energy & Fuels , 18 : 410 – 417 . [CSA] [CROSSREF]
- Marjamäki , M. , Keskinen , J. , Chen , D. R. and Pui , D. Y. H. 2000 . Performance Evaluation of the Electrical Low-Pressure Impactor, (ELPI) . J. Aerosol Sci. , 31 : 249 – 261 . [CSA] [CROSSREF]
- McMurry , J. and Fay , R. C. 1998 . Chemistry , 2nd ed. , London : Prentice-Hall International (UK) .
- Noone , B. 2005 . Biobränsle Hälsa Miljö , http://www.itm.su.se/bhm/rapporter/slutrapport_sammanlattning_prd.pdf [CSA]
- Pagels , J. , Strand , M. , Rissler , J. , Szpila , A. , Gudmundsson , A. , Bohgard , M. , Lillieblad , L. , Sanati , M. and Swietlicki , E. 2003 . Characteristics of Aerosol Particles Formed During Grate Combustion of Moist Forest Residue . J. Aerosol Sci. , 34 : 1043 – 1059 . [CSA] [CROSSREF]
- Pagels , J. 2005 . Fine and Ultrafine Particles from Combustion—Investigations with In-Situ Techniques , Lund, , Sweden : Lund University, Div. of Ergonomics and Aerosol Technology . PhD thesis
- Park , K. , Kittelson , D. B. and McMurry , P. H. 2004 . Structural Properties of Diesel Exhaust Particles Measured by Transmission Electron Microscopy (TEM): Relationships to Particle Mass and Mobility . Aerosol Sci. Technol. , 38 : 881 – 889 . [CSA] [CROSSREF]
- Reid , J. S. , Koppmann , R. , Eck , T. F. and Eleuterio , D. P. 2004 . A Review of Biomass Burning Emissions, Part II: Intensive Physical Properties Biomass Burning Particles . Atmos. Chem. Phys. Discussions , 4 : 5135 – 5200 . [CSA]
- Rissler , J. , Swietlicki , E. , Zhou , J. , Roberts , G. , Andreae , M. O. , Gatti , L. V. and Artaxo , P. 2004 . Physical Properties of the Sub-Micrometer Aerosol over the Amazon Rain Forest During the Wet-to-Dry Season Transition—Comparison of Modeled and Measured CCN Concentrations . Atmos. Chem. Phys. , 4 : 2119 – 2143 . [CSA]
- Schmidt-Ott , A. , Baltensperger , U. , Gäggeler , H. W. and Jost , D. T. 1990 . Scaling Behaviour of Physical Parameters Describing Agglomerates . J. Aerosol Sci. , 21 : 711 – 717 . [CSA] [CROSSREF]
- Simoneit , B. R. T. , Schauer , J. J. , Nolte , C. G. , Oros , D. R. , Elias , V. O. , Fraser , M. P. , Rogge , W. F. and Cass , G. R. 1999 . Levoglucosan, a Tracer for Cellulose in Biomass Burning and Atmospheric Particles . Atmos. Environ. , 33 : 173 – 182 . [CSA] [CROSSREF]
- Stokes , R. H. and Robinson , R. A. 1966 . Interactions in Aqueous Nonelectrolyte Solutions: I. Solute Solvent Equilibria . J. Phys. Chem. , 70 : 2126 – 2130 . [CSA]
- Stolzenberg , M. R. and McMurry , P. H. 1988 . TDMAFIT User's Manual , Minneapolis, MN, , USA : PTL Publications No. 653, Particle Technology Laboratory, Department of Mechanical Eng., University of Minnesota .
- Swedish Energy Administration . 2003 . Energy in Sweden, Facts and Figures, 2004 , Available online at: www.stem.se [CSA]
- Svenningsson , B. 1997 . Hygroscopic Growth of Atmospheric Aerosol Particles and its Relation to Nucleation Scavenging in Clouds , Lund, , Sweden : Lund University, Dept. of Nuclear Physics . PhD thesis, ISBN: 91-628-2764-2
- Svenningsson , B. , Rissler , J. , Swietlicki , E. , Mircea , M. , Bilde , M. , Facchini , M. C. , Decesari , S. , Fuzzi , S. , Zhou , J. , Mønster , J. and Rosenørn , T. 2005 . Hygroscopic Growth and Critical Supersaturations for Mixed Aerosol Particles of Inorganic and Organic Compounds of Atmospheric Relevance . Atmos. Chem. Phys. Discuss. , 5 : 1 – 45 . [CSA]
- Swietlicki , E. , Zhou , J. , Covert , D. S. , Hämeri , K. , Busch , B. , Väkevä , M. , Dusek , U. , Berg , O. H. , Wiedensohler , A. , Aalto , P. P. , Mäkelä , J. , Martinsson , B. G. , Papaspiropoulos , G. , Mentes , B. , Frank , G. and Stratmann , F. 2000 . Hygroscopic Properties of Aerosol Particles in the Northeastern Atlantic During ACE-2 . Tellus , 52B : 202 – 227 . [CSA]
- Tang , I. N. 1997 . Thermodynamic and Optical Properties of Mixed-Salt Aerosols of Atmospheric Importance . J. Geophys. Res. , 102 : 1883 – 1893 . [CSA] [CROSSREF]
- Valmari , T. , Lind , T. M. , Kauppinen , E. I. , Sfiris , G. , Nilsson , K. and Maenhaut , W. 1999 . Field Study on Ash Behavior During Circulating Fluidized-Bed Combustion of Biomass. 2. Ash Deposition and Alkali Vapor Deposition . Energy and Fuels , 13 : 390 – 395 . [CSA] [CROSSREF]
- Vestin , A. , Swietlicki , E. , Rissler , J. , Zhou , J. and Frank , G. 2004 . CCN Closure Study for Amazonian Dry Season Biomass Burning Aerosol . Proc. EAC 2004. , [CSA]
- Wang , S. C. and Flagan , R. C. 1989 . Scanning Electrical Mobility Spectrometer . J. Aerosol Sci. , 20 : 1485 – 1488 . [CSA] [CROSSREF]
- Weingartner , E. , Burtscher , H. and Baltensperger , U. 1997 . Hygroscopic Properties of Carbon and Diesel Soot Particles . Atmos. Environ. , 31 : 15 [CSA]
- Winklmayr , W. , Reischl , G. P. , Lindner , A. O. and Berner , A. 1991 . A New Electromobility Spectrometer for the Measurement of Aerosol Size Distributions in the Size Range from 1 to 1000 nm . J. Aerosol Sci. , 22 : 289 – 296 . [CSA] [CROSSREF]
- WHO . 2003 . Report on a WHO Working Group. Health Aspects of Air Pollution with Particulate Matter, Ozone and Nitrogen Dioxide , Copenhagen : WHO Regional Office for Europe . Document EUR/03/5042688
- WHO . 2004 . Report on a WHO Working Group. Meta-Analysis of Time-Series Studies and Panel Studies of Particulate Matter (PM) and Ozone (O3). , Copenhagen : WHO Regional Office for Europe . Document EUR/04/5042688
- Wierzbicka , A. , Lillieblad , L. , Pagels , J. , Strand , M. , Gudmundsson , A. , Gharibi , A. , Swietlicki , E. , Sanati , M. and Bohgard , M. 2005 . Particle Emissions from District Heating Units Operating on Three Commonly Used Biofuels . Atmos. Environ. , 39 : 139 – 150 . [CSA] [CROSSREF]
- Zeromskiene , K. 2004 . Physical characterization of laboratory-produced biomass burning aerosol particles , Leipzig, , Germany : Leibniz-Institute for Tropospheric Research . PhD thesis, (no ISBN available)
- Zhou , J. 2001 . Hygroscopic Properties of Atmospheric Aerosol Particles in Various Environments. , Lund, , Sweden : Lund University, Dept. of Nuclear physics . PhD thesis, ISBN: 91-7874-120-3