Measurement methods for fine carbonaceous aerosol were compared under field sampling conditions in Flushing, New York during the period of January and early February 2004. In-situ 5- to 60-minute average PM 2.5 organic carbon (OC), elemental carbon (EC), and black carbon (BC) concentrations were obtained by the following methods: Sunset Laboratory field OC/EC analyzer, Rupprecht and Patashnick (R&P) series 5400 ambient carbon particulate monitor, Aerodyne aerosol mass spectrometer (AMS) for total organic matter (OM), and a two-wavelength AE-20 Aethalometer. Twenty-four hour averaged PM 2.5 filter measurements for OC and EC were also made with a Speciation Trends Network (STN) sampler. The diurnal variations in OC/EC/BC concentrations peaked during the morning and afternoon rush hours indicating the dominant influence of vehicle emissions. BC/EC slopes are found to range between 0.86 and 1.23 with reasonably high correlations (r > 0.75). Low mixing heights and absence of significant transported carbonaceous aerosol are indicated by the measurements. Strong correlations are observed between BC and thermal EC as measured by the Sunset instrument and between Sunset BC and Aethalometer BC. Reasonable correlations are observed among collocated OC/EC measurements by the various instruments.
INTRODUCTION
Particulate matter in ambient air is becoming an increasingly important topic of study in recent years as concerns about its health effects and contributions to visibility reduction, climate and catalysis of atmospheric oxidative processes rise. Since specific mechanisms related to health effects are under study, the ability to monitor various properties of the ambient aerosol is of great interest to the research community. One such property which is of interest is atmospheric particulate carbon. Carbon is one of the major components of fine particulate matter (PM2.5), and carbonaceous material accounts for a significant fraction of urban and rural aerosols (CitationShah et al. 1986). Particulate carbon may be broadly classified into three components: elemental carbon (EC), organic carbon (OC), and carbonate carbon (CC). EC and OC are predominantly emitted from combustion sources. Organic aerosol can also be formed in the atmosphere from products of gas-phase photochemical reactions (secondary organic aerosol, SOA) (CitationTurpin et al. 1991). Aerosol carbonates originate from the soil, though some may be artifacts resulting from the reaction of CO2 with filter substrate alkalinity during collection. They are generally not observed in PM2.5.
OC and EC are operationally defined by their analysis method or protocol. The Interagency Monitoring of Protected Visual Environments/Thermal Optical Reflectance (IMPROVE/ TOR) (CitationChow et al. 1993) and the National Institute of Occupational Health and Safety/Thermal Optical Transmittance (NIOSH/TOT) (CitationBirch and Cary 1996) thermal evolution protocols present different operational definitions, and hence different results (CitationChow et al. 2001).
Light absorption by particles is primarily due to the carbonaceous species in the particles. The terms “elemental carbon,” “black carbon (BC),” and “light-absorbing carbon” are often used interchangeably by researchers. However, as shown in CitationJeong et al. (2004), these quantities are not measures of the same properties of the PM2.5. Hence, these terms need to be carefully defined before monitoring their properties. These properties depend on the age and sources of the aerosol, and from the analytical methodology. When the components of the ambient carbonaceous aerosol are considered thermally different, the EC fraction is called thermal EC, and is usually determined by TOT or TOR analysis. Alternatively, when both components are considered optically different, the strongly light absorbing fraction is known as BC. BC can be measured using a variety of optical absorption methods, and the Aethalometer (CitationHansen et al. 1984) is one of the most commonly utilized, and easy-to-use techniques employed to measure real-time BC concentrations. BC is generally produced during incomplete high-temperature combustion. It is affected to a limited extent by atmospheric processing and is therefore a direct tracer for combustion emissions (CitationHansen and Novakov 1990). Both EC and BC define a comparable fraction of the carbonaceous aerosol, but have slightly different thermal, optical and chemical behavior. The various chemical and physical properties of the EC may cause changes in the measured optical BC concentrations even if the same measurement system is used (CitationJeong et al. 2004).
Currently, there are very few studies which have compared and contrasted the various field measurement protocols for the different carbonaceous species. CitationBae et al. (2004) reported the results of the comparison of the semi-continuous Sunset Laboratory OC/EC Analyzer (Sunset Laboratory, Tigard, Oregon) to laboratory based OC/EC measurements of collocated 24-hour integrated filter samples. Comparison of the 24-hour averaged semi-continuous fine particle total carbon (TC), OC and EC showed good agreement with the daily integrated measurements. An intercomparison of the different measurement protocols will aid towards a more comprehensive understanding of the properties of the various carbon fractions, and a better characterization of the different operationally defined quantities. Since all of the field measurements involved higher time-resolved measurements than filter-based measurements, they could permit the observation of the dynamics of aerosol formation over the course of a day or a pollution episode. Furthermore, the determination of the OC/EC ratio from the various measurement methods can aid in investigating the extent of secondary organic aerosol formation, and in understanding the influence of meteorology, diurnal and seasonal fluctuations and the effect of local sources on OC/EC emissions.
As part of the PM2.5 Technology Assessment and Characterization Study–New York (PMTACS–NY) winter-intensive field campaign, real-time black carbon, and semi-continuous organic and elemental carbon measurements were made at an urban site in New York. This paper presents the results of the comparison of the measurements of the various instruments analyzing the OC, BC, and EC fractions of the carbonaceous aerosol. Relationships between OC, thermal EC, and BC were then explored.
EXPERIMENTAL METHODS
The study was conducted during the period of January 12, 2004 to February 5, 2004, at the New York Supersite at Queens College. This site is located at 40° 43′ 42″ N, 73° 49′ 17″ W, at an altitude of ∼ 20 m above sea level, about 14 kilometers west of Manhattan. It is close to both freeways and airports. The Long Island Expressway (I-495) and the Van Wyck Expressway (I-678), two high-traffic highways in the New York City metropolitan area, are both less than two kilometers from the site. Two international airports, John F. Kennedy and LaGuardia, are both within 12 kilometers of the sampling site. The prevailing wind direction in the region during this period was northwesterly.
Carbon fractions in PM2.5 were measured by the semi-continuous Sunset Laboratory OC/EC field instrument for OC, thermal EC and optical EC, the R&P 5400 series ambient carbon particulate monitor (Rupprecht and Patashnick, East Greenbush, New York) for OC and soot carbon (thermal EC), the aerosol mass spectrometer (AMS, Aerodyne Research Inc., Billerica, Massachusetts) for organics mass (OM), a Speciation Trends Network (STN) R&P 2300 24-hr Quartz filter sampler, for OC and EC, and the two-wavelength AE-20 Aethalometer (Magee Scientific, Berkeley, California) for BC.
Samples introduced into the semi-continuous Sunset Laboratory OC/EC field instrument and the Aethalometer were segregated by a PM2.5 Very Sharp Cut Cyclone (VSCC) (BGI Inc., Waltham, Massachusetts). The Sunset Laboratory instrument was set up to collect samples for 44 minutes and analyze the collected sample for 16 minutes every hour. In order to avoid artifacts due to gas phase organics, aerosol was passed through a carbon-impregnated filter (CIF) multichannel, parallel plate denuder (CitationEatough et al. 1993) before being sampled by the Sunset instrument. The two-wavelength Aethalometer (370 and 880 nm) acquired 5 minute averages for BC. The Aethalometer measures the transmission of light through a quartz filter tape before and after sampling (CitationHansen et al. 1984). Further details on the measurement protocol and operation of the Sunset instrument and the Aethalometer are given by CitationJeong et al. (2004).
The R&P 5400 ambient carbon particulate monitor conducted hourly measurements of total carbon (TC) including organic and soot carbon concentration levels in ambient particulate. Ambient particles were sampled through a sharp cut cyclone at 16.7 L/min and collected on an impaction surface maintained at 50°C. The impactor was then heated in air to 340°C for organic carbon (OC) and subsequently to 750°C for TC. EC is defined as the difference between TC and OC. The system allows a direct measure of carbon through the detection of CO2 concentration generated by the high temperature oxidation of carbon species volatilized from or remaining in the collected particulate matter. A complete description of this instrument is available in CitationRupprecht et al. (1995). It is pertinent to mention that the R&P 5400 monitor was located approximately 200 m from the sampling site.
Data on the total organic matter (OM) concentration was collected using an aerosol mass spectrometer (AMS) developed by Aerodyne Research Inc. (CitationJayne et al. 2000). The AMS samples aerosol through an aerodynamic lens, with an inlet cutpoint around 1 μ m, which is used to focus and transmit particles to an impaction region where thermal desorption of the particles and electron impact ionization of the vapor occurs prior to mass analysis by a quadruple mass spectrometer. Samples introduced into the AMS were segregated by a PM2.5 cyclone.
Quartz filters were collected every third day during the period of sampling as part of the Speciation Trends Network (STN) PM2.5 chemical speciation monitoring program (CitationEPA 1999). An additional 12 days of 24-hour integrated samples were collected by the Atmospheric Sciences Research Center (ASRC), Albany, New York during the intensive period. All the filters were analyzed at Research Triangle Park (RTP), North Carolina for OC and EC using the STN version of the NIOSH OC/EC protocol.
RESULTS AND DISCUSSION
Data Summary
OC/EC values for the R&P 5400 instrument were lost from January 16 to January 23 due to its unexpected malfunction. The average mass concentrations of the various fractions of the carbonaceous aerosol between January 12, 2004 and February 5, 2004 for each instrument are shown in . The values were calculated based on the hourly averages of each measurement, except for the STN and ASRC filters which were 24-hour integrated samples. The OC concentrations measured by the Sunset OC/EC instrument were found to be consistently higher than those measured by the R&P 5400 and the STN and ASRC filters. A linear regression between the OC measured by the Sunset instrument and the PM2.5 mass concentrations measured at this site yielded an intercept of 1.14 μ g/m3. The presence of an intercept given that the EC concentrations were in good agreement among the different methods suggests a positive artifact (CitationTolocka et al. 2001; CitationKim et al. 2005). It is possible given the wide range in diffusion coefficients that some gas phase organic species can penetrate the denuder and adsorb on the quartz fiber filter. The intercept value was used as the measure of the positive artifact, and the Sunset OC data were corrected to address this issue.
TABLE 1 Average hourly concentrations of OC, thermal EC, BC, and OM
BC Comparison
shows the diurnal variations of the BC and UV-absorbing organic material (UV-BC) concentrations, averaged over the sampling period, measured by the Aethalometer at wavelengths of 880 and 370 nm respectively. The UV wavelength measurement serves to identify the presence of certain organic compounds such as polycyclic aromatic hydrocarbons (PAHs) that strongly absorb UV wavelengths. It is seen that the hourly average BC levels peak around 0700 to 0900 local time and again around 1700 local time with the concentrations beginning to drop at around 2100 hours. This pattern is consistent with observed rush hour traffic emission patterns on the nearby expressways. Wood combustion emissions from nearby residential establishments may also have contributed to the observed peaks. The small peak observed in the early morning hours around 0300 hours could be attributed to the snow plows that operated close to the sampling site at this time during the period of sampling. The peaks and valleys of the BC and UV-BC values are seen to track each other very closely and this is also observed by a comparison of the corresponding BC and UV-BC 5 minute data. The absence of any considerable enhancement in the UV absorbing signal seems to indicate that there were minimal quantities of non-black, UV-absorbing aromatic organic material associated with the carbonaceous aerosol.
FIG. 1 Diurnal variations of hourly BC and UV-BC concentrations from the two-wavelength AE-20 Aethalometer for (a) average concentrations and (b) median concentrations.
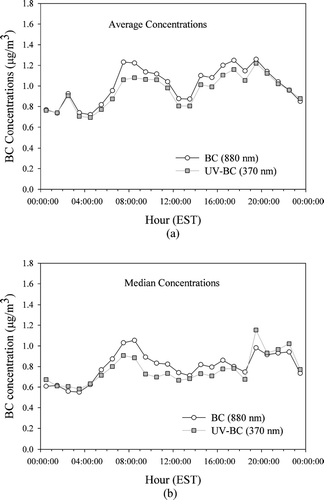
The morning peak pattern (0700 to 0900 hours) is observed for the hourly median BC concentrations, albeit ∼0.2 μg/m3 lower than the corresponding average concentrations, as seen in . It is seen, however, that the evening peak is diluted, probably by the presence of a few large concentration spurts observed by the Aethalometer. It is also observed that the nightly drop is not as prominent in the hourly median BC concentrations.
Comparisons between collocated Aethalometer BC and EC measurements from the Sunset, R&P and STN & ASRC filter EC are reported in . Slopes ranging from 0.86 ± 0.09 and 0.86 ± 0.66 (BC versus Sunset EC and BC versus STN and ASRC filter EC respectively) to 1.23 ± 0.15 (BC versus R&P EC) are observed with reasonably high correlations (r > 0.75). A significantly higher degree of scatter is observed in the plot of BC versus R&P EC () compared to the plot of BC versus Sunset EC (). This might be attributed to the fact that the R&P 5400 EC is measured and defined differently than the Sunset EC. The lower values from the R&P instrument may result from its lower capture efficiency since it uses a collection surface that is heated to 50°C and has an impaction cutpoint at 0.18 μ m. In addition, the R&P 5400 was located approximately 200 m from the Aethalometer and Sunset inlets and on the other side of a parking lot. Pairwise comparisons as well as the observed slope indicate that the Aethalometer BC levels are lower than filter EC concentrations which is consistent with earlier studies (CitationWatson and Chow 2002; CitationLiousse et al. 1993). It is to be noted that in the case of the STN and ASRC measurement, the three outliers were selected based on unacceptable reconstructed mass values observed in these cases. To reconcile the difference between the measured Aethalometer BC and EC measurements, the former's internal conversion factor 16.6 m2g−1, that is recommended by the manufacturer, and translates the measured light absorption to BC values, may need to be adjusted. Similar results have been found in several prior studies (CitationJeong et al. 2004). It has been suggested that the Aethalometer requires calibration based on the sampling location (CitationLavanchy et al. 1999) and the physical and chemical properties of the aerosol (CitationHorvath 1993; CitationPetzold et al. 1997).
Sunset Laboratory Semi-Continuous Carbon Comparison
Ambient organic and elemental carbon concentrations were measured on an hourly basis using the in situ Sunset Laboratory carbon analyzer for the period of the study. Average concentrations of the particulate organic and elemental carbon and ozone, an indicator of the intensity of atmospheric photochemical activity, are plotted in as a function of the time of day. The ozone data was obtained from standard photometric ozone monitors maintained by the New York State Department of Environmental Conservation (NYSDEC) at the site. Each data point represents the midpoint of the sampling period. The EC shows a distinct maximum at about 0800 hours local time, that corresponds to the morning rush hour. It is seen that from this time into the afternoon, the EC concentrations dropped whereas the concentrations of the OC and ozone rose. Ozone reached its maxima in the early afternoon between 1300 and 1400 hours local time. During this time, the ratio of organic to elemental carbon also reached its maximum. These observations strongly suggest the presence of secondary organic aerosol (SOA) in the OC measured during the afternoon peak. The contribution of SOA to the total measured organic carbon is small, as expected, considering the reduced photochemical activity indicated by the low ozone concentrations even at their maxima. The EC concentrations again exhibit a secondary maximum at about 1700 hours local time, corresponding to the evening rush hour in the nearby expressways. The diurnal profiles of OC concentrations resembled profiles of EC concentrations in the early morning and late evening periods. This result suggests a strong influence of primary OC on total OC measured during these times, since EC is a marker for primary combustion-generated OC (CitationLim and Turpin 2002). These observations point strongly to the fact that vehicular traffic was the major variable in the measurements.
FIG. 3 (a) Average hourly Sunset thermal EC, Sunset OC and ozone concentrations in Flushing, New York during January 12–February 5, 2004, and (b) Average hourly Sunset thermal EC, Sunset optical EC and Aethalometer BC concentrations during the same period.
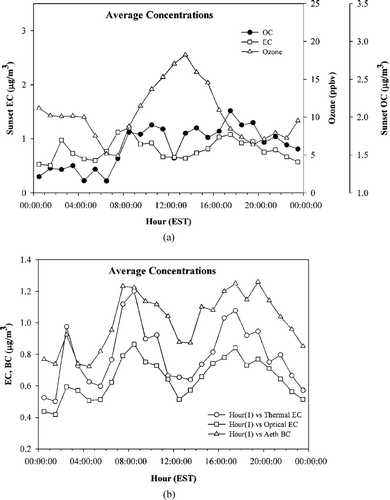
A comparison of the averaged thermal and optical EC measured by the Sunset Laboratory instrument and the BC measured by the Aethalometer is shown in as a function of the time of day. The diurnal variations are pronounced, and again appear to be strongly related to vehicular traffic. It was observed in CitationJeong et al. (2004) that there was a lack of an afternoon peak in OC/EC, primarily because the boundary layer mixing heights were high enough to dilute out the rush hour peak. In our study, the absence of the dilution of the afternoon peaks seems to suggest low mixing heights or that there might not have been an effect of the mixing height on ambient carbon concentrations over the course of the day. Also, there might not have been significant sources of transported carbonaceous aerosol because of the presence of northwesterly winds for much of the period. For northwesterly flow, there are no cities with a population over one million people within 500 km of New York City. Also, the high wind speeds encountered during the sampling period provide high dilution and low residence time in source areas. It is observed that the Sunset Laboratory instrument also registered a peak in elemental carbon at around 0300 hours local time that corroborates the results of the BC comparisons.
and show the correlations between the hourly averaged Sunset Laboratory optical EC and the Sunset Laboratory thermal EC and Aethalometer BC, respectively. The number of pair-wise valid samples for the comparisons were 506 and 539, respectively. As presented in , the optical EC was strongly correlated with the thermal EC values with a high correlation (r = 0.93), and a slope of 0.58 ± 0.05. The optical EC was found to be even more strongly correlated with the Aethalometer BC values (r = 0.96), as shown in , with a slope of 0.62 ± 0.05. The fact that the Sunset Laboratory optical EC measures light absorbance using a 680 nm laser, whereas the Aethalometer measures light absorption at 880 nm might account in part for the higher BC values as compared to the optical EC values.
Comparison Among Filter-Based and Semi-Continuous Carbon Measurements
For the available 24-hour sample pairs, it is found that the R&P 5400 reported 0.25 μ g m−3 more OC and 0.29 μg m−3 more TC than the STN and ASRC averages (). Pair-wise comparisons between the two measurements, shown in , indicate reasonable correlations of the R&P 5400 with the STN and ASRC of 0.76 for OC, 0.88 for EC, and 0.91 for TC. The coefficient of divergence (COD) values (CitationWongphatarakul et al. 1998) between contemporaneous R&P 5400 and the STN and ASRC measurements were calculated to ascertain the similarity in their measurement performance. It was observed that the R&P 5400 tracked TC closely (COD = 0.094), but did not compare as well with the 24-hour OC (COD = 0.171) or EC (COD = 0.213). Reasons for the differences between the filter measurements and the R&P 5400 could include different OC versus EC split temperatures, different combustion atmospheres, and higher maximum temperature reached during filter analysis.
TABLE 2 Collocated comparisons among semi-continuous and filter based carbon measurements
Collocated comparisons of the R&P 5400 measurements with the Sunset Laboratory analyzer measurements, indicate a moderate correlation of 0.66 for OC, and reasonable correlations of 0.78 for EC and 0.77 for TC. A COD of 0.275 for TC, 0.3 for EC, and 0.32 for OC indicate a moderate similarity between the R&P 5400 and Sunset samplers. The Sunset instrument was also found to correlate well with the 24-hour filter samples with a high correlation of 0.97 for EC, 0.82 for OC, and 0.88 for TC. The average values () show the Sunset reporting only about 3% less OC and 5% more TC than the 24-hour samples. However, the COD between the Sunset and the 24 hour samples for TC (0.172), OC (0.196), and EC (0.188) indicate a greater spread between the measurement methods.
The Aerosol Mass Spectrometer (AMS) is a very useful tool in understanding the composition and processes of volatilizable particulate organics in the atmosphere. Ten-minute averaged values of total organic mass (OM) were obtained from this instrument during the course of the study. Average OM/OC ratios () were found to be 2.89 for the Sunset, 2.43 for the R&P 5400, and 2.79 for the 24-hour integrated STN and ASRC filter samples. shows that the correlations of the AMS OM with the three other measurements ranged from 0.57 to 0.87, with slopes ranging from 1.24 to 1.61. This value is in the range of the OM/OC ratio of 1.4 employed in the IMPROVE protocols to determine species contributions to fine particle mass.
OC/EC Ratios and Implications
Estimation of the contribution of secondary organic aerosol (SOA) to ambient particulate OC is essential to the development of effective control strategies for particulate matter. SOA formation has been estimated using time-resolved OC and EC data (CitationTurpin and Huntzicker 1995; CitationStrader et al. 1999). CitationLim and Turpin (2002) suggest the use of ambient OC/EC ratios to estimate SOA formation. In our study, as seen in , the regression of all hourly OC on EC data suggests 1.3 as a reasonable upper limit to represent the primary OC/EC ratio based on the observed slopes with the Sunset (slope: 1.29 ± 0.20, r2 = 0.58) and the R&P (slope: 1.05 ± 0.21, r2 = 0.22) measurements. The low OC/EC ratio compared to 2.1 suggested by Lim and Turpin could be because of reduced photochemical activity during winter months compounded with the high urban emission sources. It is apparent that the primary OC/EC ratio is dependent on location and meteorology. It is also observed that the 1-h average OC and EC measurements are moderate to poorly correlated, which is consistent with the presence of SOA, since EC is a primary OC tracer.
CONCLUSIONS
The diurnal patterns in average OC/EC concentrations indicate the strong influence of local sources on the concentrations and this is corroborated by the diurnal trends in BC concentrations observed by the Aethalometer. Effects of transported aerosol and mixing heights are observed to be minimal. Hourly averaged optical EC, thermal EC, and Aethalometer BC are observed to be highly correlated (r > 0.90). BC values are found to be consistently different from the measured EC values, suggesting the need to calibrate the Aethalometer attenuation coefficient based on the sampling location and physical and chemical properties of the aerosol. The presence of SOA in the measured OC is observed, and it is suggested that the estimation of SOA based on OC/EC ratios is dependent on sampling location, season and local meteorology, and that a single ratio cannot be used universally to estimate SOA in measured OC.
REFERENCES
- Bae , M. -S. , Schauer , J. J. , DeMinter , J. T. , Turner , J. R. , Smith , D. and Gary , R. A. 2004 . Validation of a Semi-Continuous Instrument for Elemental and Organic Carbon Using a Thermal-Optical Method . Atmos. Environ. , 38 : 2885 – 2893 . [CSA]
- Birch , M. E. and Gary , R. A. 1996 . Elemental Carbon-Based Method for Monitoring Occupational Exposures to Particulate Diesel Exhaust . Aerosol Sci. Technol. , 25 : 221 – 241 . [CSA]
- Chow , J. C. , Watson , J. G. , Pritchett , L. C. , Pierson , W. R. , Frazier , C. A. and Purcell , R. G. 1993 . The DRI Thermal/Optical Reflectance Carbon Analysis System: Description, Evaluation and Applications in U.S Air Quality Studies . Atmos. Environ. , 27A : 1185 – 1201 . [CSA]
- Chow , J. C. , Watson , J. G. , Crow , D. , Lowenthal , D. H. and Merrifield , T. 2001 . Comparison of IMPROVE and NIOSH Carbon Measurements . Aerosol Sci. Technol. , 34 : 23 – 34 . [CSA]
- Eatough , D. J. , Wadsworth , A. , Eatough , D. A. , Crawford , J. W. , Hansen , L. D. and Lewis , E. A. 1993 . A Multiple System, Multi-Channel Diffusion Denuder Sampler for the Determination of Fine Particulate Organic Material in the Atmosphere . Atmos. Environ. , 27A : 1213 – 1219 . [CSA]
- Environmental Protection Agency . 1999 . EPA Strategic Plan , Research Triangle Park, North Carolina : Office of Air Quality Planning and Standards . Development of the Particulate Matter (PM2.5) Quality System for the Chemical Speciation Monitoring Trends Sites, http://www.epa.gov/ttn/amtic/files/ambient/pm25/spec/stratel.pdf
- Hansen , A. D. A. , Rosen , H. and Novakov , T. 1984 . The Aethalometer-an Instrument for the Realtime Measurement of Optical Absorption by Aerosol Particles . Sci. Total Environ. , 36 : 191 – 196 . [CROSSREF] [CSA]
- Hansen , A. D. A. and Novakov , T. 1990 . Real-Time Measurement of Aerosol Black Carbon During the Carbonaceous Species Methods Comparison Study . Aerosol Sci. Technol. , 12 : 194 – 199 . [CSA]
- Horvath , H. 1993 . Comparison of Measurements of Aerosol Optical Absorption by Filter Collection and a Transmissometric Method . Atmos. Environ. , 27 : 319 – 325 . [CSA]
- Jayne , J. T. , Leard , D. C. , Zhang , X. , Davidovits , P. , Smith , K. A. , Kolb , C. E. and Worsnop , D. R. 2000 . Development of an Aerosol Mass Spectrometer for Size and Composition Analysis of Submicron Particles . Aerosol Sci. Technol. , 33 : 49 – 70 . [CROSSREF] [CSA]
- Jeong , C. H. , Hopke , P. K. , Kim , E. and Lee , D. W. 2004 . The Comparison Between Thermal-Optical Transmittance Elemental Carbon and Aethalometer Black Carbon Measured at Multiple Monitoring Sites . Atmos. Environ. , 38 : 5193 – 5204 . [CROSSREF] [CSA]
- Kim , E. , Hopke , P. K. and Qin , Y. 2005 . Estimation of Organic Carbon Blank Values and Error Structures of the Speciation Trends Network Data for Source Apportionment . J. Air Waste Manage. Assoc. , 55 : 1190 – 1199 . [CSA]
- Lavanchy , V. M. H. , Gaggeler , H. W. , Nyeki , S. and Baltensperger , U. 1999 . Elemental Carbon (EC) and Black Carbon (BC) Measurements with a Thermal Method and an Aethalometer at the High-Alpine Research Station Jungfraujoch . Atmos. Environ. , 33 : 2759 – 2769 . [CROSSREF] [CSA]
- Lim , H. -J. and Turpin , B. J. 2002 . Origins of Primary and Secondary Organic Aerosol in Atlanta: Results of Time-Resolved Measurements During the Atlanta Supersite Experiment . Environ. Sci. Technol. , 36 : 4489 – 4496 . [INFOTRIEVE] [CROSSREF] [CSA]
- Liousse , C. , Cachier , H. and Jennings , S. G. 1993 . Optical and Thermal Measurements of Black Carbon Aerosol Content in Different Environments: Variation of the Specific Attenuation Cross-Section, Sigma (σ) . Atmos. Environ. , 27A : 1203 – 1211 . [CSA]
- Petzold , A. , Kopp , C. and Niessner , R. 1997 . The Dependence of the Specific Attenuation Cross-Section on Black Carbon Mass Fraction and Particle Size . Atmos. Environ. , 31 : 661 – 672 . [CROSSREF] [CSA]
- Rupprecht , E. G. , Patashnick , H. , Beeson , D. E. , Green , R. E. and Meyer , M. B. 1995 . “ A New Automated Monitor for the Measurement of Particulate Carbon in the Atmosphere ” . In Paniculate Matter: Health & Regulatory Issues , Edited by: Cooper , J. A. and Grant , L. D. Pittsburgh, PA : Air & Waste Management Assoc. .
- Shah , J. J. , Johnson , R. L. , Heyerdahl , E. K. and Huntzicker , J. J. 1986 . Carbonaceous Aerosol at Urban and Rural Sites in the United States . J. Air Pollut. Control Assoc. , 36 : 254 – 257 . [CSA]
- Strader , R. L. , Lurmann , F. and Pandis , S. N. 1999 . Evaluation of Secondary Organic Aerosol Formation in Winter . Atmos Environ. , 33 : 4849 – 4863 . [CROSSREF] [CSA]
- Tolocka , M. P. , Solomon , P. A. , Mitchell , W. , Norris , G. A. , Gemmill , D. B. , Wiener , R. W. , Vanderpool , R. W. , Homolya , J. B. and Rice , J. 2001 . East Versus West in the US: Chemical Characteristics of PM2.5 During the Winter of 1999 . Aerosol Sci. Tech. , 34 : 88 – 96 . [CROSSREF] [CSA]
- Turpin , B. J. , Huntzicker , J. J. , Larson , S. M. and Cass , G. R. 1991 . Los Angeles Summer Midday Particulate Carbon: Primary and Secondary Aerosol . Environ. Sci. Technol. , 25 : 1788 – 1793 . [CROSSREF] [CSA]
- Turpin , B. J. and Huntzicker , J. J. 1995 . Identification of Secondary Organic Aerosol Episodes and Quantitation of Primary and Secondary Organic Aerosol Concentrations During SCAQS . Atmos. Environ. , 29 : 3527 – 3544 . [CROSSREF] [CSA]
- Watson , J. G. and Chow , J. C. 2002 . Comparison and Evaluation of In Situ and Filter Carbon Measurements at the Fresno Supersite . J. Geophys. Res. , 107 : 3-1 – 3-15 . ICC[CSA]
- Wongphatarakul , V. , Friedlander , S. K. and Pinto , J. P. 1998 . A comparative Study of PM2.5 Ambient Aerosol Chemical Databases . Environ. Sci. Technol. , 32 : 3926 – 3934 . [CROSSREF] [CSA]