The effect of double-pulse fuel split injection on the exhaust aerosol particle size distribution emitted by a state-of-the-art Heavy Duty Diesel engine was experimentally investigated. The influence of post-injection fuel quantity and dwell was evaluated at four steady-state engine conditions by analyzing the changes in the accumulation mode particle number, mean diameter and geometric standard deviation, with respect to the baseline case of single injection. Generally, rising post-injection fuel amount was found to increase accumulation mode particle number and mean diameter of the size distributions. Particle number reduction efficiency resulted dependent on the operating conditions and post-injection parameters scheme. Reductions in the particle number around 50% with respect to the baseline single-pulse injection case were observed for 1500 rpm partial load cases. For 1800 rpm 75% load conditions the reduction was lower and was achieved only when the fuel amount was below 20% of the total fuel injected. An increase in the particle number was produced when post-injection was applied at low speed and high load conditions, due to the low accumulation mode particle number emitted at this operation mode. An optimum post-injection fuel amount for particle number reduction was only seen for the 1500 rpm 75% load operation conditions. The results are indicative that further research is necessary in order to explore the existence of optimum post-injection schemes for particle number reduction at the other operating conditions studied.
Geometric standard deviation from post-injection results was higher than that corresponding to the single injection baseline cases, while an increase in the σ g value was observed for larger dwells in some operating conditions.
1. INTRODUCTION
Adverse effects of particles on human health have been reported in several studies (CitationPope 2000; CitationPetrovic et al. 2000; CitationOberdörster 2001). Recent works have been carried out in order to determine the relative toxicity of coarse, fine and ultrafine particles (CitationLi et al. 2003) by collecting highly concentrated ambient particles of various sizes to study their chemical composition and biological danger (CitationKim et al. 2001; CitationLi et al. 2002). According to these authors, the biological potency of unequal size particles is related to the location where the particles are deposited on the cells due to their sizes. In addition, these researchers consider that both particle size and chemical composition are important in terms of biological effects. CitationLi et al. (2002) showed that the size and the chemical constituents of particles seem to be related. For instance, ultrafine particles were found to contain significantly more organic carbon and PAHs than coarse or fine particles. Various researchers suggest that particle size is closely associated with lung injury since the finer the particle, the higher the inflammation produced (CitationOberdörster et al. 1994a, Citationb; CitationOberdörster and Utell 2002).
Although some investigations have reported that particle number is more related to the induction of adverse health effects than particle mass (CitationDonaldson et al. 1998; CitationSydbom et al. 2001), current legislation for particle emissions is based on a gravimetric procedure. In order to improve legislation procedures for particles emission restrictions, a new measurement system including particle number measurement is being evaluated in the European Particle Measurement Program (PMP), to replace and/or complement the existing gravimetric method in the current legislation (CitationECE-GRPE Particle Measurement Programme 2004). As a result of the findings in this program, the Swiss Agency for the Environment has already proposed a draft ordinance which stipulates that with effect from 1 January 2006, diesel passenger cars may only be placed on the Swiss market if they do not exceed the maximum number emission level of 1011 part/km (CitationSAEFL 2004). In addition, Germany's UBA (equivalent to U.S. EPA) strongly recommends the European Union to adopt much tougher Euro-5 vehicle emissions limits and create the world's first legislative limits on so-called nanoparticle particulate matter (PM) emissions.
1.1. The Diesel Aerosol
The diesel aerosol is mainly composed of highly agglomerated solid carbonaceous material and ash, volatile organic and sulphur compounds (CitationKittelson 1998). As a result of fuel pyrolysis during the combustion process, solid carbonaceous material is formed as primary soot particles of sizes from 20 to 50 nm from unburned hydrocarbons in locally fuel rich regions inside the engine cylinder (CitationHeywood 1988). The primary particles collide with each other, so that soot aggregates are formed from primary-primary particle collisions and they grow by cluster-primary particle and cluster-cluster collisions. Simultaneously, a fraction of the carbon matter is oxidized in the flame by oxidant compounds, mainly O2 and OH radicals. The resulting carbonaceous compounds from this formation-oxidation balance constitute the solid carbon agglomerates which are found in the exhaust aerosol as the carbonaceous core of the particles.
The soluble organic fraction of the particles (SOF) is formed from the gaseous hydrocarbons coming from the atomized and evaporated lube oil and fuel that have not been oxidized inside the engine. During dilution and cooling of the exhaust flow, some of the gaseous hydrocarbons and sulphates undergo gas-to-particle conversion processes, such as nucleation, to form new particles, and adsorption/condensation onto the surface of the existing carbonaceous particles. According to this, the controlling factors of all the dynamic processes related to dilution affect the final physical and chemical properties of the exhaust aerosol.
The typical particle size distribution of Diesel aerosols is trimodal and approximately log-normal in form (CitationKittelson 1998). The so-called nucleation mode is mainly made up of organic matter and sulphur compounds. It consists of volatile particles that have been formed by nucleation, and may also contain some solid carbon and metal compounds. The nucleation mode is made up of particles with diameter ranging from 5 to 50 nm and contains the largest number of particles of the exhaust aerosol (more than 90%) but a small amount of its mass (1–20%). The accumulation mode is mainly associated with carbonaceous agglomerates and the volatile matter adsorbed on their surface. The diameter of the accumulation mode particles ranges from 50 nm to 1 μ m with low number of particles in comparison with the nucleation mode but with most of the total particle mass. A third coarse mode exists consisting of particles deposited on the engine cylinder and exhaust system walls, which are later reentrained. The coarse mode contains particles larger than 1 μ m in diameter and it comprises 5% to 20% of the total particle mass.
1.2. Multiple Injection Emission Control Strategy
Extensive research is in progress to reduce gaseous and particulate emissions from Diesel engines due to health and environmental concerns. In-cylinder emission-control strategies are used to reduce the formation of pollutants during the combustion process. Matching injection pressure, injection timing and exhaust gas recirculation, are usual in-cylinder emission control strategies. However, most of the emission-control strategies applied so far reduce soot particle emissions while increasing NOx emissions and vice-versa, resulting in the known trade-off between NOx and particle emissions.
Conversely, it has been shown experimentally that the application of high-pressure multiple injection can reduce NOx and soot mass emissions significantly with respect to the single-pulse fuel injection case (CitationHan et al. 1996). Multiple injection or split-injection can be defined as an injection strategy in which the fuel is injected in two or more separate injection events, with similar of different fuel quantities in the different injection stages. Different types of multiple injection have been applied, including pilot injection and post-injection. In the pilot injection strategy a small fuel pulse is injected before the main injection, while in the post-injection the main injection is followed by a short injection pulse. The rest of split-injection types would include the cases in which the injection is divided in several events of similar fuel quantity.
Multiple injection has been examined in the works of CitationNehmer and Reitz (1994), CitationTow et al. (1994), CitationPierpont et al. (1995), and CitationPayri et al. (2002). CitationNehmer and Reitz (1994) reduced NOx emissions with no penalty in soot emissions by using different double-pulse split injection schemes. CitationTow et al. (1994) continued the work of Nehmer and Reitz, including different dwells between injection pulses and triple injection schemes in their investigation. They found that important particulate emissions reduction could be achieved at some engine conditions, with no penalty in the NOx emissions and fuel consumption. An important conclusion of CitationTow et al. (1994) is that the dwell between injection pulses is very important to control soot production and that an optimum dwell exists at any particular engine operating condition. CitationPierpont et al. (1995) showed that the amount of fuel injected in the first pulse affects the particulate emission in experiments where the NOx emission was held constant. CitationPayri et al. (2002) analyzed the influence of post-injection pattern on soot smoke emissions in a heavy-duty diesel engine, finding optimum values for soot reduction for certain post-injected fuel amounts at each of the operation conditions studied. According to these experimental works on the influence of split injection on soot emissions, both the amounts of fuel injected and the dwell between pulses are important for an optimum injection scheme in order to reduce emissions.
Mechanisms for soot reduction produced by split-injection were explained by CitationHan et al. (1996). According to these authors, when split injection is applied, the rich soot regions formed after the first injection pulse are not continuously replenished, as occurs in the single injection. After the first pulse, subsequent injections take place into a high temperature environment left over from combustion of previous pulses. As a consequence, the post-injected fuel burns more rapidly, soot formation rates are decreased and the net soot production is significantly reduced. Besides, secondary pulse injected sprays are expected to enhance fuel-air mixing, which tends to lean out the mixture and reduce soot formation. CitationChmela and Riediger (2000) also stated that the soot reducing effect of post-injection was partially due to the fact that the increase in the gas temperature after the main injection promotes soot oxidation in the post-injected spray.
Although a number of studies have been carried out on the effect of the main engine modifications such as injection pressure, injection timing and exhaust gas recirculation on particle size distributions (CitationBaumgard and Johnson 1996; CitationKreso et al. 1998; CitationPagán 1999; CitationBertola et al. 2001; CitationTsolakis et al. 2005; CitationDesantes et al. 2005), a lack exists in the investigation of the effect of multiple injection on exhaust particle number and size. Only a few studies have been published on the effect of pilot injection on particle number size distributions (CitationJaeger et al. 1999; CitationMohr et al. 2001), which consisted on the comparison of a pilot injection case with baseline conditions of single injection in a light-duty diesel engine.
In this present work, a comprehensive and detailed characterization study of the effect of post-injection pattern on the aerosol particle size distribution from a heavy-duty diesel engine is presented. The influence of the post-injection fuel quantity and dwell time was evaluated at four steady-state engine conditions by analyzing the changes in the accumulation mode particle number, mean diameter and geometric standard deviation, with respect to the baseline case of single injection. The results show that application of split injection strategy can reduce the accumulation mode particle number as long as the post-injected fuel quantity is adequately controlled. Varying dwell between pulses caused changes in both the particle number and mean diameter, with different trends observed for each operating condition. Higher geometric standard deviations of the size distributions were observed for the post-injection cases in comparison with the single injection baseline results, while an increase in the geometric standard deviation was found for increasing post-injection dwells in some operating conditions.
2. EXPERIMENTAL SET-UP
2.1. Engine, Fuel and Measurement Systems
The engine used in this study was a 6-cylinder state-of-the-art heavy-duty diesel engine. The engine specifications are shown in . A low sulphur #2 Diesel fuel was used for the experiments. Specifications of fuel and lubricating oil are given in and . The engine was run on an AVL-ELIN engine dynamometer type APA 4Z4/EU, and the engine data were recorded and stored with an AVL-PUMA acquisition system.
TABLE 1 Diesel Heavy-Duty engine specifications
TABLE 2 Diesel fuel specifications
TABLE 3 Lubricating oil specifications (Shell Helix-F SAE 5W-30 type)
The amount of fuel consumption was determined by a fuel gravimetric system with an AVL 733S Dynamic Fuel Meter. A hot-film anemometer system Sensyflow P Sensycon was used to measure the intake air mass flow.
2.2. Systems for Particle Emissions Measurement
A single-stage partial flow dilution system was coupled with an SMPS instrument in order to measure particle size distributions. A scheme of the experimental set-up is shown in . The measurement systems employed for particle emissions measurement are briefly described below.
Dilution Minitunnel and Particle Sizing Instrument
The dilution system used was a minitunnel AVL-SPC 472, which consists of a heated tunnel of 635 mm length and 30 mm inner diameter. The dilution ratios that can be achieved with this system range from 4 to 40 for 0.2 to 2 g/s of total dilution flow. At the specification of the dilution ratio and the total flow, a constant flow, which is the sum of the dilution air and the extracted fraction of the exhaust flow, is pumped by a pumping system.
The Scanning Mobility Particle Sizer (SMPS) is a widely used instrument for measuring the size distribution of sub-micron particles in a gas. The operating principle of the SMPS is based on the extraction of an original polydisperse aerosol in different monodisperse fractions by separating the particles according to their size by their electrical mobility inside an electrical field. The extracted monodisperse particle fractions are counted in a condensation particle counter which provides the number of particles per air volume unit for each particle size. A TSI SMPS-3936 and a CPC-3010 models were used in the present study.
To extract a diluted sample for the particle size measurement, the SMPS was connected downstream of the dilution system. The sampling point where the sample probe of the SMPS is connected is considered to be an influencing factor on particle size measurements, since the mixing conditions between dilution air and exhaust sample determine particle dynamics and, as a result, they affect particle size measurements (CitationSuresh and Johnson 2001). The filter holder piece after the dilution tunnel, which is normally used for the gravimetric analysis, was chosen as a location to connect the 6 mm inner diameter, perforated type tube sample probe to ensure an uniform mixture of the dilution air and the extracted sample flow. The SMPS system was positioned so that the required sampling line was as short as possible (1.2 m) to reduce sampling tube losses. The flexible sampling line was the same type of material as the connections of the SMPS instrument, i.e. silicone conduct with an inner diameter of 10 mm.
3. TEST MATRIX AND MEASUREMENT METHODOLOGY
The experimental plan was designed to study independently the effect of post-injection parameters at different steady-state engine operating conditions. The experiments consisted on the variation of the fuel amount distribution between the main and the post-injection pulses at constant dwell, as well as the variation of the dwell time between pulses at constant fuel distribution settings. The tests were performed at several operating conditions in order to analyze whether a different effect is produced by the post-injection schemes on the particle size distributions at different engine operating conditions. Four engine steady-state operation modes defined by the European Stationary Cycle for Heavy-Duty Diesel engines certification (CitationDirective 1999) were selected for the experimental study. The engine operation modes denoted as A100, B50, B75, and C75, whose speed and load specifications are displayed in , were selected for the experimental work. Post-injection settings of the experiments performed are also included in .
TABLE 4 Experimental plan for the parametric study
After the engine was started and warmed, a stabilization time of 15 minutes was considered prior to each measurement. After this stabilization time, three consecutive measurements with a 2-minute interval between them were carried out with the SMPS instrument.
A standard methodology to perform particle size measurements under laboratory conditions has not been established yet. This is due to the existence of many factors of the sampling and dilution systems that affect the final measurements (CitationAbdul-Khalek et al. 1999; CitationMathis 2002), and a standard instrument and calibration procedure for the measurement of particle size distribution have not yet been selected.
The measurement methodology described in a previous work (CitationDesantes et al. 2004) was applied in this study. Such measurement procedure was developed in order to reduce the sensitivity of particle size measurements to dilution, sampling and measurement factors at typical laboratory conditions. The measurement procedure specifications for the performance of experiments in this study are shown in . Particles losses in the sampling system and the SMPS system were not considered here. The dilution ratio used in this study was 20, which is a very low value compared with real atmospheric dilution conditions. CitationKittelson et al. (1998b) reported that a dilution ratio of 1000 is typical for an atmospheric dilution process of the exhaust flow. However, such a dilution ratio cannot be applied for many laboratory studies due to the high requirements of the dilution ratio control. Some investigators claim that low values of dilution ratio can cause artefact formation of nuclei mode particles, however, CitationKittelson et al. (1998b) and CitationVogt et al. (2003) have measured nuclei mode particles in real road atmospheric conditions. Whether the aerosols of these real road measurements consist of the same particle composition as laboratory experiments requires considerable investigation that is presently ongoing. The contribution of the present investigation is to report conditions that were used here and how those conditions influenced exhaust particle results for a given engine, the different engine operating conditions and sampling system tested.
TABLE 5 Operation thresholds and procedures applied in the measurement methodology (CitationDesantes et al. 2004)
5. ANALYSIS METHODOLOGY AND RESULTS
Influence of post-injection fuel quantity and dwell is studied in this work by analyzing the effect on the particle number size distribution characteristics. However, it should be noted that the objective of the work is to explore the effect of post-injection pattern on the particle size distributions and not to carry out an optimizing work in order to reduce particle number emission.
Up to this time, high variability has been found in the measurement of the nucleation mode because nucleation process is highly nonlinear and very sensitive to sampling conditions (CRC E-43 project, 2002). Although in some works, changes in the nucleation mode have been linked to modifications in engine parameters (CitationPagán 1999; CitationDesantes et al. 2005), the variations observed in this study could not be attributed to changes in the parameters evaluated. Hence, nucleation mode data were not used for the interpretation of results, but only those corresponding to the accumulation mode. In order to obtain accurate values of accumulation mode particle number and mean diameter, the accumulation mode was extracted from the particle number distributions by fitting the experimental data to the theoretical equation for normalized lognormal distributions (CitationSeinfeld and Pandis 1998):
In the discussion that follows, the results are described separately for the different steady-state engine conditions used in the experimental study. In , , , particle size distributions corresponding to each operating conditions are shown; the influence on the accumulation mode total particle number and mean diameter are represented in and , respectively.
FIG. 2 Influence of post-injection fuel quantity (A, B, and C plots) at constant dwell and influence of post-injection dwell (D, E, and F plots) at constant fuel quantity on the particle size distributions. Engine operation mode A100 (1200 rpm, 100% load).
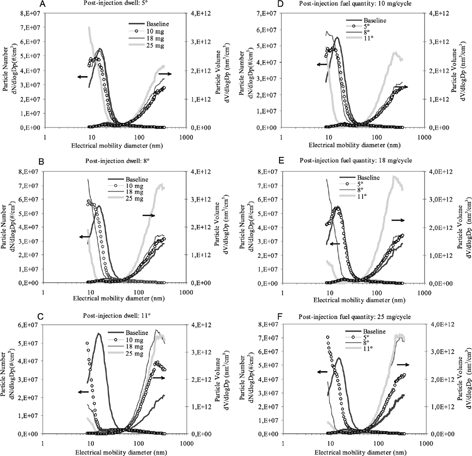
FIG. 3 Influence of post-injection fuel quantity (A, B, and C plots) at constant dwell and influence of post-injection dwell (D, E, and F plots) at constant fuel quantity on the particle size distributions. Engine operation mode B50 (1500 rpm, 50% load).
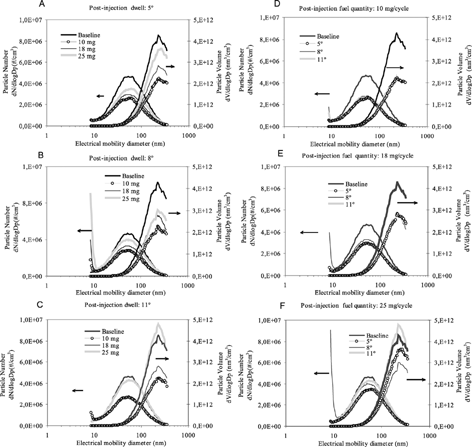
FIG. 4 Influence of post-injection fuel quantity (A, B, and C plots) at constant dwell and influence of post-injection dwell (D, E, and F plots) at constant fuel quantity on the particle size distributions. Engine operation mode B75 (1500 rpm, 75% load).
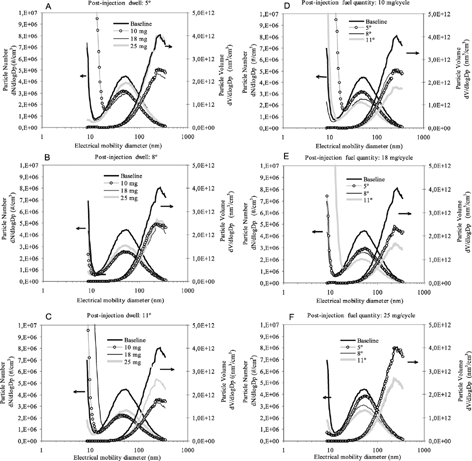
FIG. 5 Influence of post-injection fuel quantity (A, B, and C plots) at constant dwell and influence of post-injection dwell (D, E, and F plots) at constant fuel quantity on the particle size distributions. Engine operation mode C75 (1800 rpm, 75% load).
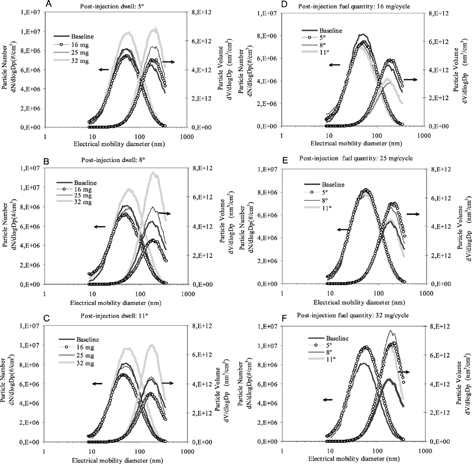
FIG. 6 Effect of post-injection fuel quantity and dwell on accumulation mode particle number at different operating conditions.
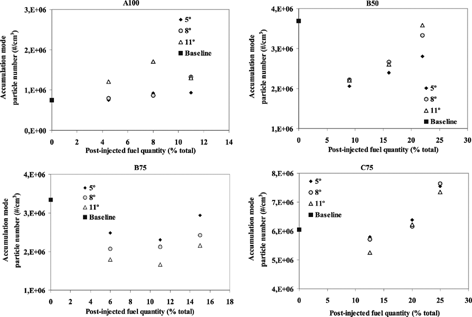
FIG. 7 Effect of post-injection fuel quantity and dwell on the accumulation mode particle mean diameter at different operating conditions.
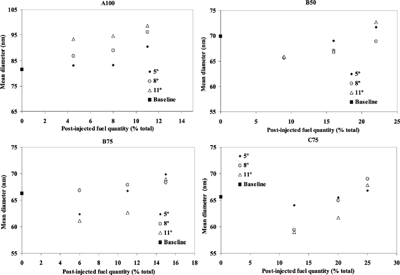
A100 Operating Conditions
Particle number size distributions for the A100 operating conditions are presented in , where A, B and C plots show the influence of post-injection fuel quantity at constant dwell and D, E and F plots present the influence of split injection dwell at constant fuel quantities.
The aerosol from these operating conditions consists of a large nucleation mode and a very small accumulation mode. Little carbonaceous aerosol is observed due to the high exhaust temperatures and low speed conditions (i.e., long residence times), that strongly favour soot oxidation. As shows, the accumulation mode particle number concentration is so low that analysis of the influence can only be done in a volume basis. From plots A, B and C in , an increase in the accumulation mode particle volume is observed as the split injection fuel quantity is increased at constant post-injection dwell. For all the cases, the particle volume baseline, which corresponds to single-injection conditions, is below the distributions obtained from the application of post-injection. Furthermore, the difference between the baseline case and the other experiments seems to increase with increasing dwell. The influence of post-injection dwell on the particle number and volume size distribution for the A100 case can be seen in plots D, E and F in . The 11° case shows the highest volume concentrations while the shortest dwell shows the lowest concentration at any fuel amount. The intermediate 8° dwell results were close to the 5° or the 11° distributions depending on the fuel amount used.
The effect of both injection dwell and fuel quantity (as a percentage of the total fuel amount) on the accumulation mode particle number, compared with the baseline case, is shown in the A100 plot in . As can be seen from this plot, the particle number is increased as the fuel quantity is raised for the 5° and 8° dwells, while at 11° a rise is produced when the fuel quantity is increased from 4.5% to 8% of total amount, followed by a reduction to a similar 4.5% fuel amount particle number level when 11% fuel quantity is applied. As mentioned above, the difference between the baseline conditions and the post-injection results is higher, the higher the dwell applied. Baseline particle number is similar to values found for the 5° and 8° cases with 4.5% and 8% fuel amounts. The experimental data corresponding to 11° dwell 8% fuel quantity is too high when compared with the trend followed by the other dwell series. By analyzing in detail the particle size distributions of the A100 case it was seen that for this experiment the accumulation mode was higher than for the other post-injected fuel amounts at 11° dwell. The low amount of accumulation mode particles at these operation conditions makes difficult to obtain precise measurements, since the eventual reentrainment of soot deposits or the presence of volatiles can contribute more significantly to overestimate the particle number in the accumulation mode. This may explain the unexpected high accumulation mode particle number measured at the 11° dwell 8% fuel quantity case.
Regarding the influence of dwell, the highest amount of particles is measured for the highest dwell, except for the 25 mg fuel quantity, in which the highest particle number corresponds to the 8° and 11° cases, both with a similar particle number.
In the work of CitationPayri et al. (2002) a similar study as this one was performed by analyzing the influence of the post-injection pattern on the soot smoke emission from a heavy-duty diesel engine. A comparison of the trends observed by Payri et al. can be done with the results of accumulation mode particle number in the present study. These authors found that at the operating conditions in which a low smoke emission was measured, the results of post-injection were close to or above those of the baseline conditions, as occurs in our experimental results for the A100 case, for particle number. In the referred work they also found an optimum for soot smoke reduction for 10% of post-injected fuel mass. The reduction achieved in the referred work was the lowest with respect to other operating conditions in which a higher level of soot was emitted. The results of Payri et al. along with our observations for the A100 case are indicative that post-injection is not so efficient at operating conditions in which a low particle amount is emitted. More precisely, in our results, the injection schemes studied do not provide a particle number reduction with respect to the baseline case. However, further exploration with post-injection fuel percents below 10% and short dwells (5 and 8°) would clarify if a reduction can be achieved by using post-injection and if a minimum in the particle number exists at certain post-injection quantity.
shows the influence of post-injection scheme on the mean diameter of the distributions, compared with the data corresponding to the baseline case. For the A100 operating conditions the accumulation mode mean diameter for any post-injection scheme is higher than for the baseline data. This difference is enhanced by the use of larger dwells and higher fuel quantities.
B50 Operating Conditions
presents the influence of post-injection on particle number size distributions for the B50 case. Plots A, B and C indicate that higher accumulation mode is produced as the fuel amount is increased at constant dwell. By analyzing plots D, E, and F, the influence of split injection dwell at constant fuel quantity can be determined. A slight change in the particle size distributions is obtained when the injection dwell is changed for the 10 and 18 mg/cycle post-injected fuel amounts, while for the 25 mg quantity a higher accumulation mode particle number is produced as the dwell is increased. In the B50 plot in , the influence of both post-injected fuel quantity percent and dwell on the total particle number is shown. The accumulation mode particle number concentration rises as the post-injection fuel quantity is increased. This effect is enhanced by the use of the highest dwells. A reduction in the particle number was achieved at the different post-injection patterns studied in comparison with the baseline case. Although the results indicate that low post-injected fuel amount along with short dwells reduces particle number, an optimum injection scheme for particle number reduction is not evident for the post-injection settings studied. Other authors have found optimum schemes for smoke reduction at post-injected fuel amounts below 10% (CitationPayri et al. 2002), which is the minimum percent used here. Optimum values of dwells for smoke level reduction were also found in the work of CitationTow et al. (1994) and CitationPierpont et al. (1995). This suggests again that exploration of fuel amount and dwells below those used in this study could reveal the existence of an optimum scheme for accumulation mode particle number reduction at these operating conditions.
In the B50 plot in a larger mean diameter for the post-injection results is observed as the fuel amount is increased, but a defined trend cannot be identified for the dwells. With regards to the baseline conditions, the 9% and 16% fuel quantity cases exhibit mean diameter values below the baseline one, while the mean diameter is larger than the baseline data when 22% fuel amount is post-injected at 5° and 11° dwells. It is observed in these results that higher mean sizes are associated with increasing particle number, as reported in a previous work (CitationDesantes et al. 2005).
At present, it is not clear if small particles are more dangerous than large ones, although some suggestions have been made that the small particles can have higher biological potency. In case these hypotheses are real it should be taken into account that although post-injection strategy can reduce particle number with respect to the baseline conditions there are cases in which this is also accompanied by a reduction in the mean particle size ( and ).
B75 Operating Conditions
The particle number distributions for the B75 operating conditions are shown in . It can be observed in the A, B, and C plots, that at any constant dwell, a rise is provoked in the accumulation mode when the post-injected fuel amount is increased from 10 or 18 mg/cycle to 25 mg/cycle, with similar size distributions at 10 and 18 mg/cycle fuel quantities. In plots D, E, and F a higher accumulation mode is observed, the shorter the dwell.
Results of mode B75 in show that the particle number presents a minimum for a post-injection fuel quantity around 11%. All the cases of post-injection produced an accumulation mode particle number below the baseline case value, with lower particle number, the longer the split injection dwell applied. Differences between the B50 and B75 engine conditions results in can be explained by considering that the minimum post-injected fuel amount tested for the B75 case (6%) is a value low enough to observe the optimum fuel amount for particle reduction, which was not the case for the B50 conditions.
shows a rise in the mean diameter for any dwell as the fuel post-injected amount is increased, collapsing to a single value at around 70 nm for the highest quantity tested. The baseline mean diameter for this operation mode is below the 15% fuel quantity results and similar to 5 and 8° cases at 11% fuel amounts. It is also seen that the maximum size at 6 and 11% fuel amounts is produced for the intermediate dwell considered.
C75 Operating Conditions
The results of the C75 operating conditions are presented in . In plots A, B, and C the trend that increasing post-injected fuel amount produces a rise in the accumulation mode is seen again. A shift to higher sizes is also observed as fuel amount augments. Plots D, E, and F show that particle size distributions change slightly with varying dwell, except for the 16 mg case, in which the 5° dwell presents the highest particle number. The baseline conditions size distribution is very similar to the 25 mg/cycle (i.e., 20%) post-injected fuel at any dwell. The results indicate that fuel amounts above 25 mg/cycle produce higher particle number than the baseline conditions, while when below 25 mg/cycle the particle size distributions obtained are lower than in the baseline case.
Results for the C75 case presented in confirm that the accumulation mode particle number increases as the fuel amount is raised. The baseline case shows similar result as the 20% post-injected fuel conditions, with higher particle concentrations than the 12.5% fuel quantity case and lower value than the 25% fuel amount case, as observed above. An optimum injection scheme for particle reduction is not seen for this case, but further explorations of fuel amounts below 12.5% and dwells above 11° might show the existence of an optimum setting for fuel amount and dwell.
Studying the effect on the mean diameter in indicates an increase in the mean size as the fuel quantity is raised, with no clear trend shown by changing the dwell. With respect to the baseline case, its mean diameter value is below that of the 25% fuel case, close to the 5° and 8° dwells at 20% post-injected fuel amount and above the 20% fuel 11° dwell case and all the 12.5% fuel amount cases.
In order to compare the effect of post-injection pattern on the size distributions on a same scale basis, the total particle number and mean diameter percentage variation for post-injection experiments with respect to the baseline case are plotted in and . In , case A100 exhibits a significant particle number increase ranging from 5 to 125% when post-injection is applied. Significant reductions in the particle number are achieved with post-injection in the medium speed part load cases, with maximum percentage variations of the order of 44% for the B50 case and 50% for the B75 case. For the C75 operating conditions, a reduction was only achieved when the post-injected fuel was 12.5% of the total fuel amount, with a maximum particle reduction of 13% at 11° dwell. The reduction of particle number at this case is not as high as for the 1500 rpm conditions, probably due to the short residence times at high speed conditions, which makes soot oxidation for the post-injected spray less favorable.
FIG. 8 Effect of post-injection fuel quantity and dwell on the accumulation mode particulate number percentage variation at different operating conditions.
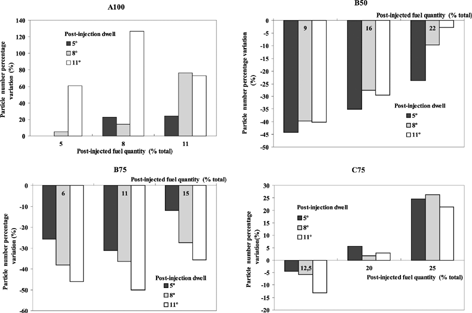
FIG. 9 Effect of post-injection fuel quantity and dwell on the mean diameter percentage variation at different operating conditions.
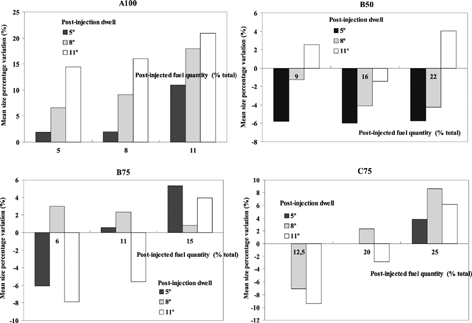
shows the percentage variations of mean diameter with respect to the baseline case. The highest changes correspond to the A100 mode in which increments in the mean size with respect to the baseline case are produced when post-injection is used. Mean diameter values in the A100 increase from 2 to 21%, with an effect enhanced by both larger dwells and higher fuel amounts. For the other three cases, variations with respect to the baseline conditions are not so clear, and particle size increases or reductions are always lower than 10%, with trends depending very much on the particular injection strategy. In general terms variations are more important for the cases of higher loads.
Geometric standard deviations (σ g ) were compared for the B50, B75, and C75 cases, whose values are presented in . For the A100 case, geometric deviation data are not available since the low accumulation mode at these conditions makes their calculation impossible. The geometric standard deviation is a parameter defining the width of normalized particle size distributions. The geometric standard deviation of exhaust particle size distributions is almost constant (∼ 1.8) and independent of engine type, operating conditions and fuel properties (CitationHarris and Maricq 2001). However, a more complete evaluation of the distribution width can be done if the values of σ g are observed with detail. Geometric standard deviations in show an increase from 2.08 to ∼ 2.16 for the B50 and B75 cases, as the post-injection dwell is increased, while the changes in the fuel amount did not cause a defined trend. The increase in the geometric deviation with the dwell was not observed for the C75 case either. For all the cases analyzed the standard deviations of the size distributions obtained from post-injection results were higher than the baseline case value, which means that wider particle size distributions are obtained when post-injection is applied.
TABLE 6 Geometric standard deviations of particle size distributions for post-injection and baseline tests. Operating conditions B50, B75 and C75
4. PARTICLE SIZE DISTRIBUTION VARIABILITY ANALYSIS
Three consecutive measurements were carried out for obtaining an average particle size distribution for each experiment. The variability found between consecutive measurements was below 5% at each experiment, which means that measurements show an acceptable repeatability. However, because of the similarities in the particle size distributions measured in this study a question arises as to whether the differences observed are mostly a result of the changes in engine conditions or if a large part of the observed differences may be due to measurement variability. In order to clarify this issue a repeatability study was carried out in order to evaluate the reproducibility and repeatability of measurements performed with the SMPS. Measurements of the particle size distribution at 1100 rpm and 50% load operating conditions with only main injection were carried out with intervals of 10–15 min. during one hour in different days. The results of variability in the total particle number and mean diameter within the same day are shown in , where the coefficient of variation within each day is indicated. Furthermore, shows the average particle size distributions obtained for the three different days, along with the theoretical fit for the measurements. This representation and data in indicates that an acceptable repeatability is achieved by using the experimental methodology of this work, with an average variability value of 7% in the particle number measurement.
FIG. 10 Measurement repeatability of particle size distributions at three different days. 1100 rpm, 50% load.
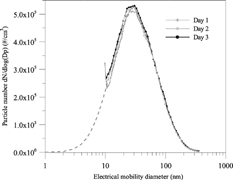
TABLE 7 Coefficient of variation for particle size distribution measurements within 3 different days
To determine if the changes observed in the particle size distributions measured in this study are actually due to engine modifications, coefficients of variability were calculated for each group of three experiments for the independent evaluation of post-injected fuel mass and dwell at each operating condition. The calculated coefficients of variability, calculated on accumulation mode particle number basis, are listed in the . From these results it is seen that the change caused by the increase in the post-injected fuel mass is higher than the measurement variability, whose average value is 7%, which means that the change in the results due to changes in this parameter studied is significant. In addition, the consistency of the results with respect to the effect of the fuel post-injected mass for all the operating conditions is an evidence of the data repeatability.
TABLE 8 Coefficients of variation for particle number calculated between measurements from the study of the influence of post-injection dwell and fuel mass quantity
On the other side, the coefficient of variation for the case of the injection dwell shows values above that corresponding to the measurement variability for the A100 and B75 operating conditions, while the B50 and C75 cases present coefficients within the measurement variability range. For the last two cases it is not easy to determine if the changes observed are mostly a product of variability or if they are mostly due to post-injection dwell modifications. Thus, further analysis is necessary to determine the influence of post-injection dwell at these operating conditions.
5. SUMMARY AND CONCLUSIONS
The influence of post-injection fuel quantity and dwell on the particle size distributions from a heavy-duty diesel engine was investigated at four engine steady-state conditions. As a general trend, when post-injection is applied, the rise in the post-injected fuel amount was found to increase accumulation mode particle number and mean diameter of the size distributions. Slight increase approximately 5–7% in the mean diameter values was produced with increasing fuel amount for B50, B75 and C75 operating conditions. Injection dwell was also found to affect particle size distributions.
A reduction in the particle number in comparison with the baseline case of single injection was observed when post-injection was applied for the B50 and B75 cases at any injection scheme. For the C75 case the reduction was obtained when the fuel amount was below 20% of the total fuel amount. Reduction efficiency resulted dependent on the post-injection parameters scheme and operating conditions. The maximum reductions observed were around 50%, for the 1500 rpm cases studied. Conversely, for the case of low accumulation mode particle number from the low speed high load conditions (A100) an increase in the particle number was produced when post-injection was applied at any injection scheme. Although accumulation mode cannot be seen with detail in the A100 operating conditions, the increase observed in the particle volume can be interpreted as an increase in the particle number, because particle volume distribution is calculated from particle number, by considering the particle volume at each particle size.
An optimum fuel quantity for particle number reduction was only observed for the B75 case. The results are indicative that further exploration by using post-injected fuel amounts below 10% of total fuel and a wider study of dwells, are necessary in order to find whether an optimum post-injection setting for particle reduction exists at the other operating conditions studied.
Geometric standard deviations from post-injection results were higher than that corresponding to the single injection baseline case, while an increase in the geometric standard deviation values was found for larger dwells in some operating conditions.
Acknowledgments
This work was done within the frame of the project PARTSIZE (NNE5-2001-00108), financed by the European Commission and the Spanish Government (DPI2002-11471-E).
Notes
*For the C75 operation mode, the fuel mass quantities employed are 18, 25, and 32 mg/cycle.
REFERENCES
- Abdul-Khalek , I. , Kittelson , D. B. and Brear , F. 1999 . The Influence of Dilution Conditions on Diesel Exhaust Particle Size Distribution Measurements . SAE Techn. paper , 1999-01-114[CSA]
- Baumgard , K. J. and Johnson , J. H. 1996 . The Effect of Fuel and Engine Design on Diesel Exhaust Particle Size Distributions . SAE Techn. paper 960131 , [CSA]
- Bertola , A. , Schubiger , R. , Kasper , A. , Matter , U. , Forss , A. M. , Mohr , M. , Boulouchos , K. and Lutz , T. 2001 . Characterization of Diesel Particulate Emissions in Heavy Duty DI Diesel Engines with Common Rail Fuel Injection. Influence of Injection Parameters and Fuel Composition . SAE Techn. paper , 2001-01-3573[CSA]
- Chmela , F. and Riediger , H. 2000 . Analysis Methods for the Effects of Injection Rate Control in Direct Injection Diesel Engines . THIESEL 2000 Conference on Thermo-and Fluid Dynamic Processes in Diesel Engines. Conference Proceedings . September 13–15 2000 , Valencia. ISBN 84–7721-910–9, 333–344
- CRC E-43-Diesel Aerosol Sampling Methodology . Final Report , Minneapolis : University of Minnesota. Department of Mechanical Engineering . http://www.crcao.com/reports/recentstudies00–02/UMN%20Final%20E43%20Report.pdf
- Desantes , J. M. , Bermúdez , V. , Pastor , J. V. and Fuentes , E. 2004 . Methodology for Measuring Exhaust Aerosol Size Distributions by Means of a Scanning Mobility Particle Sizer . Meas. Sci. Technol. , 15 : 2083 – 2098 . [CROSSREF] [CSA]
- Desantes , J. M. , Bermúdez , V. , García , J. M. and Fuentes , E. 2005 . Effect of Current Engine Strategies on the Exhaust Aerosol Particle Size Distribution from a Heavy-Duty Diesel Engine . J. Aerosol Sci. , 36 : 1251 – 1276 . [CROSSREF] [CSA]
- 2005 . Directive 1999/96/EC of the European Parliament and the Council of December 13, 1999 . Official J. European Communities , L 44 : 1 – 155 . [CSA]
- Donaldson , K. , Li , X. Y. and Mac Nee , W. 1998 . Ultrafine (nanometer) Particle Mediated Lung Injury . J. Aerosol Sci. , 29 : 553 – 560 . [CROSSREF] [CSA]
- ECE-GRPE Particle Measurement Programme . 2004 . Proposal for A Draft Amendment To The 05 Series of Amendments to Regulation No. 83 (Emissions of M1 and N1 categories of vehicles). Informal document No. GRPE-48–11 (48th GRPE, 1–4 June 2004, agenda item 2) . http://www.akpf.org/pub/2004_grpe4811.pdf [CSA]
- Han , Z. , Uludogan , A , Hampson , G. J. and Reitz , R. 1996 . Mechanism of Soot and NOx Emission Reduction Using Multiple-Injection in a Diesel Engine . SAE Tech. paper 960633 , [CSA]
- Harris , S. and Maricq , M. 2001 . Signature Size Distribution for Diesel and Gasoline Engine Exhaust Particle Matter . J. Aerosol Sci. , 32 : 749 – 764 . [CROSSREF] [CSA]
- Heywood , J. B. 1988 . Internal Combustion Engine Fundamentals. , McGraw-Hill International Editions .
- Jaeger , L. , Boulouchos , K. and Mohr , M. 1999 . Analysis of Factors Influencing Particulate Matter Emissions of a Compression-Ignition Direct-Injection Engine . SAE Tech. paper , 1999-01-3492[CSA]
- Kim , S. , Jaques , P. , Chang , M. and Sioutas , C. 2001 . Versatile Aerosol Concentration Enrichment Systems (VACES) for Simultaneous In Vitro and In Vivo Evaluation of Toxic Effects of Ultrafine, Fine and Coarse Ambient Particles. Part II: Field Evaluation . J. Aerosol Sci. , 11 : 1299 – 1314 . [CROSSREF] [CSA]
- Kittelson , D. B. 1998 . Engines and Nanoparticles: A Review . J. Aerosol Sci. , 29 : 575 – 588 . [CROSSREF] [CSA]
- Kittelson , D. B. , Watts , W. F. and Arnold , M. 1998b . Review of Diesel Particulate Sampling Methods. , Univ. Minnesota Dep. Mech. Eng. . http://www.crc90.com/reports/recentstudies00–02/UMN%20Final%20E-43%“20Report.pdf
- Kittelson , D. , Johnson , J. , Watts , W. , Wei , Q. , Drayton , M. , Paulsen , D. and Bukowiecki , N. 2000 . Diesel Aerosol Sampling in the Atmosphere . SAE Techn. paper , 2000-01-2212[CSA]
- Kreso , A. M. , Johnson , J. H. , Gratz , L. D. , Bagley , S. T. and Leddy , D. G. 1998 . A Study of the Effects of Exhaust Gas Recirculation on Heavy-Duty Diesel Engine Emissions . SAE Techn. paper , : 981422 [CSA]
- Li , N. , Kim , S. , Wang , M. , Froines , J. , Sioutas , C. and Nel , A. 2002 . Use of a Stratified Oxidative Stress Model to Study the Biological Effects of Ambient Concentrated and Diesel Exhaust Particulate Matter . Inhal Toxicol. , 14 : 459 – 486 . [PUBMED] [INFOTRIEVE] [CROSSREF] [CSA]
- Li , N. , Hao , M. , Phalen , R. F. , Hinds , W. C. and Nel , A. E. 2003 . Particulate Air Pollutants and Asthma. A Paradigm for the Role of Oxidative Stress in PM-Induced Adverse Health Effects . Clinical Inmunol. , 109 : 250 – 265 . [CROSSREF] [CSA]
- Mathis , U. Influencing Parameters of Nanoparticles Formation From Diesel Exhaust . Proceedings of the 6th International ETH-Conference on Nanoparticle Measurements . August 19–21 2002 .
- Mohr , M. , Jaeger , L. W. and Boulouchos , K. 2001 . The Influence of Engine Parameters on Particulate Emissions . Mtz Worldwide , 9 : 25 – 28 . [CSA]
- Nehmer , D. A. and Reitz , R. D. 1994 . Measurement of the Effect of Rate Shaped and Split Injections on Diesel Engine Soot and NOx Emissions . SAE Techn. pape , : 940668 [CSA]
- Oberdörster , G. , Ferin , J. and Lehnert , B. E. 1994a . Correlation between Particle Size and In Vivo Particle Persistence and Lung Injury . Environ. Health Persp. , 102 : S5 [CSA]
- Oberdörster , G. , Gelein , R. M. , Ferin , J. and Weiss , B. 1994b . Association of Particulate Air Pollution and Acute Mortality: Involvement of Ultrafine Particles? . Inhal. Toxicol. , 7 : 111 – 124 . [CSA]
- Oberdörster , G. 2001 . Pulmonary Effects of Inhaled Ultrafine Particles . Int. Arch. Occup. Environ. Health , 74 : 1 – 8 . [CROSSREF] [CSA]
- Oberdörster , G. and Utell , M. J. 2002 . Ultrafine Particles in the Urban Air: To the Respiratory Track and Beyond? . Environ. Health Persp. , 110 : A440 – A441 . [CSA]
- Pagán , J. 1999 . Study of Particle Size Distributions Emitted by a Diesel Engine . SAE Techn. paper , 1999-01-1141[CSA]
- Payri , F. , Benajes , J. , Pastor , J. V. and Molina , S. 2002 . Influence of the Post-Injection Pattern on Performance, soot and NOx emissions in a HD Diesel Engine . SAE Techn. paper , 2002-01-0502[CSA]
- Petrovic , S. , Urch , B. , Brook , J. , Datema , J. , Purdham , J. , Liu , L. , Lukic , Z. , Zimmerman , B. , Tofler , G. , Downar , E. , Corey , P. , Tarlo , S. , Broder , I. , Dales , R. and Silverman , F. 2000 . Cardiorespiratory Effects of Concentrated Ambient PM2.5: A Pilot Study Using Controlled Human Exposures . Inhal. Toxicol. , 12 : 173 – 188 . [CROSSREF] [CSA]
- Pierpont , D. A. and Reitz , R. D. 1995 . Reducing Particulate and NOx Emissions by Using Multiple Injections and EGR in a DI Diesel Engine (1995) . SAE Tech. paper , : 950217 [CSA]
- Pope , C. A. 2000 . Review: Epidemiological Basis for Particulate Air Pollution Health Standards . Aerosol Sci. Technol. , 32 : 4 – 14 . [CROSSREF] [CSA]
- SAEFL-Swiss Agency for the Environment, Forests and Landscape. Ordinance on the Determination of the Particle Number Emission Level of Passenger Cars with Compression Ignition Engines. 1 March 2004 http://europa.eu.int/comm/enterprise/tbt/tbt_repository/CHE39_EN_1_1.DOC
- Seinfeld , J. H. and Pandis , S. N. 1998 . Atmospheric Chemistry and Physics , New York : Wiley .
- Suresh , A. and Johnson , J. H. 2001 . A Study of the Dilution Effects on Particle Size Measurement from a Heavy-Duty Diesel Engine with EGR . SAE Technical paper , 2001-01-0220[CSA]
- Sydbom , A. , Blomberg , A. , Parnia , S. , Stenfors , N. , Sandstrom , T. and Dahlen , S. E. 2001 . Health Effects of Diesel Exhaust Emissions . Eur. Resp. J. , 17 : 733 – 746 . [CROSSREF] [CSA]
- Tow , T. C. , Pierpont , D. A. and Reitz , R. D. 1994 . Reducing Particulate and NOx Emissions by Using Multiple Injections in a Heavy Duty Diesel engine . SAE Tech. paper , : 940897 [CSA]
- Tsolakis , A. , Hernández , J. J. , Megaritis , A. and Crampton , M. 2005 . Dual Fuel Diesel Engine Operation Using H2. Effect on Particulate Emissions . Energy & Fuels , 19 : 418 – 425 . [CROSSREF] [CSA]
- Vogt , R. , Scheer , V. , Casati , R. and Benter , T. 2003 . On-Road Measurements of Particle Emission in the Exhaust Plume of a Diesel Passenger Car . Environ. Sci. Technol. , 37 : 4070 – 4076 . [PUBMED] [INFOTRIEVE] [CROSSREF] [CSA]