Ultrafine (< 100 nm) and accumulation mode (0.1–1 μm) particles were monitored in an occupied suburban house at 5-minute intervals for 37 consecutive months between November 21, 1997 and December 31, 2000. Number concentrations for 126 particle sizes from 9.8–947 nm were measured in 259,176 scans. Of 282 separate activities, 18 were chosen for detailed analysis. These included cooking with a gas stove, toasting with electric toasters and toaster ovens, burning candles and incense, and using a gas-powered clothes dryer. Activities leading to increased particle concentrations occurred 17.5% of the time, and accounted for more than half the total concentration of ultrafines and about a quarter of the total accumulation mode particles. The average duration of elevated particle concentrations ranged from 20 minutes to 3 hours. Combustion of natural gas (boiling water, gas clothes dryer) showed number peaks near 10 nm, while the electric toaster and toaster oven had peaks close to 30 nm. More complex cooking (burners plus gas oven) produced peaks in the 35–50 nm range. Burning candles and incense resulted in peaks in the 60-nm range. Finally, outdoor sources peaked at nearly 70 nm, indicating the influence of aging in shifting modes to higher diameters. The highest mean number concentrations were due to complex cooking, producing average number concentrations of 35,000–50,000 cm− 3, compared to 12,000 cm−3 outdoors and less than 3500 cm−3 indoors when no sources were observed. A strong contribution of the vented gas-powered clothes dryer was also noted (30,000 cm− 3). Volume concentrations due to these combustion events ranged from < 1 (μm/cm)3 to nearly 100 (μm/cm)3. Source strengths were calculated for three common cooking types (boiling water, deep-frying, oven baking, and broiling) and ranged from 5 × 10 12 to 4 × 10 13 particles per cooking event. The detailed concentration and size distribution data collected here may be useful for models of indoor air particle concentrations due to indoor sources and infiltration.
INTRODUCTION
Ultrafine particles are produced by combustion and atmospheric processes. A major outdoor source is gasoline and diesel vehicles (CitationWiedensohler, Wehmer, and Birmili 2002). Ultrafines, along with black carbon, decline sharply with distance from roadways, whereas PM mass does not (CitationZhu et al. 2002). Another outdoor source appears to be atmospheric nucleation reactions between sulfuric acid and water vapor (CitationJeong et al. 2004; CitationKulmala et al. 2004). Indoor sources have been identified as gas stoves (CitationAbt et al. 2000; CitationHe et al. 2004; CitationDennekamp et al. 2001; CitationLong et al. 2001; CitationWallace, Emmerich, and Howard-Reed 2004a), electric ovens (CitationDennekamp et al. 2001; CitationWallace 2000), gas clothes dryers (CitationWallace 2005a), and candles (CitationMorawska et al. 2001; CitationWallace 2000). Secondary formation of ultrafines in large quantities indoors has been observed from chemical reactions of ozone and terpenes, such as limonene and α-pinene, popular air fresheners (CitationWechsler and Shields 1999, Citation2003; CitationWainman et al. 2000).
Ultrafine deposition in human lungs has been studied extensively (CitationBrown, Zeman, and Bennett 2002), and has been shown to result in inflammation, impairment of phagocytosis, and thrombosis (CitationElder et al. 2000; CitationNemmar et al. 2002b; CitationRenwick, Donaldson, and Clouter 2001). The effect of differing ultrafine composition on toxicity has also been studied (CitationDick et al. 2003; CitationRenwick et al. 2004). Ultrafines can be transported by the blood to other body organs. For example, radiolabelled ultrafine aerosols were inhaled by humans and later found in their blood (CitationNemmar et al. 2002a, Citation2004). More recently, transport of ultrafines to the brain via the olfactory nerve has been demonstrated (CitationOberdörster et al. 2004).
Effects on human health have also been documented. For example, patients with stable coronary heart disease taking exercise tests had significant increased risk of developing ischemia following elevated ultrafine particle number concentrations two days before (CitationPekkanen et al. 2002). Personal exposure to ultrafines led to significantly worsened heart rate variability for young adults and elderly adults with impaired lung function (CitationChan et al. 2004). Elderly coronary heart disease patients showed increased cardiorespiratory symptoms with increased exposure to ultrafines (Citationde Hartog et al. 2003). Respiratory effects of ultrafines have been noted for asthmatics (CitationChalupa et al. 2004; CitationPentinnen et al. 2001; Citationvon Klot et al. 2002) and for persons suffering from chronic respiratory disease (CitationIbald-Mulli et al. 2002), the latter reporting on six European panel studies. Daily mortality in Erfurt, Germany was linked to both ultrafine and fine particles separately (CitationWichmann et al. 2000). In a five-city study of 22,000 survivors of a first cardiac event, the risk of readmission for a second event was significantly increased by increased exposure to both PM mass and ultrafine number, independently of each other (Citationvon Klot 2005). Persons living close to heavily trafficked roadways had higher risk of dying from cardiopulmonary disease (CitationHoek et al. 2002). In a separate study, exposure to traffic was significantly associated with onset of myocardial infarction one hour later (CitationPeters et al. 2004). Although the traffic-related results of the last two studies could be due to many other traffic-related pollutants, ultrafines have been shown to be a better indicator of traffic than PM mass (CitationZhu et al. 2002). These findings have been sufficiently compelling to merit a formal recommendation from the American Heart Association to carry out research specifically on the toxicity of ultrafine particles (CitationBrook et al. 2004).
Ultrafine particles have not been as extensively monitored as other particles. Also, if they have health effects, it may be due more to their total number than to their mass, which is only a small component of total particle mass. Therefore the number concentration of ultrafine particles, both indoors and outdoors, is of interest. Since the sizes of the ultrafine particles may be of crucial importance in determining infiltration into blood vessels, not only the total number but also the size distribution associated with different sources is of interest.
Data on indoor concentrations and indoor sources of ultrafines are largely lacking. However, since ultrafine particles can be produced by indoor combustion as well as outdoor combustion, and since indoor peak concentrations can be several times outdoor concentrations, it is possible that indoor sources could sometimes be important in producing health effects in susceptible individuals. Therefore additional information on the concentrations, size distributions, and indoor sources of ultrafine particles could be useful. Also, since most people spend most of their time indoors, their main exposure to particles of outdoor origin also occurs indoors. Therefore more knowledge of how the infiltration of outdoor particles is influenced by home characteristics will be useful, particularly to persons attempting to model indoor air concentrations and personal exposures to particles. For example, EPA's SHEDS-PM model attempts to relate personal activities such as cooking to the source strengths associated with those activities (CitationOlson and Burke 2006).
METHODS
This report is based on a multiyear study carried out by the author as part of an internal EPA grant. The study involved measurements of particles, black carbon, PAH, CO, air exchange, temperature, humidity, wind speed and direction, and household characteristics such as fan use and window opening activities in a single occupied home between October 1996 and March of 2001. Continuous monitoring was carried out over the entire length of the study, with about 79% completeness for the major particle monitors. During the course of the study, a number of papers have appeared on different aspects of the study. An initial paper reported on the design of the study and provided a few examples of results from some of the monitors employed (CitationWallace 2000). Indoor-outdoor black carbon as an indicator of traffic and woodburning was a focus of a second paper (CitationLaRosa, Buckley, and Wallace 2002). Two papers, one involving a second house in California, concentrated on air exchange rates as a function of window opening width, fan use, use of duct-mounted air filters, and indoor-outdoor temperature differences (CitationHoward-Reed, Wallace, and Ott 2002; CitationWallace, Howard-Reed, and Emmerich 2002). Two other papers dealt with deposition of fine and coarse particles (CitationHoward-Reed, Wallace, and Emmerich 2003) and ultrafine particles (CitationWallace, Emmerich, and Howard-Reed 2003). One paper attempted to determine the overall relative contributions of indoor and outdoor sources to observed indoor particle concentrations (CitationWallace and Howard-Reed 2002). Two papers dealt with source strengths of ultrafine particles from cooking (CitationWallace, Emmerich, and Howard-Reed 2004a) and from a gas dryer (CitationWallace 2005). One paper (Ogulei, in press) applied Positive Matrix Factorization (PMF) to source apportionment of indoor particles (including outdoor sources). One paper (CitationWallace 2005b) compared concurrent indoor-outdoor measurements of black carbon by two different methods.
The present paper is the first to deal with the entire 37-month period of ultrafine particle measurements. Because of the wide variability of indoor sources, a large number of examples of each type of source, collected over widely varying conditions such as different seasons, high and low temperatures, air exchange rates, and relative humidities, and many other conditions, is desirable to determine robust statistics. With more than 250,000 measurements of size-distributed ultrafine particles, and more than 21,000 hours of monitoring, quite good estimates of mean concentrations and the range of likely variations due to 18 different indoor sources have been obtained.
The study home has been described previously (CitationWallace and Howard-Reed 2002) and will be briefly described here. It is a three level, four-bedroom, 385-m3 end townhouse in Reston, Virginia, a suburban area 25 miles northwest of Washington, DC. The townhouse is located near the intersection of two suburban roads. A 6-lane highway is located approximately 1.5 miles north of the house.
Heating is central forced air with a gas furnace and standard furnace filter, gas hot water heater, and a vented gas dryer. Central air conditioning is also available with an outdoor compressor near the patio. The basement is partially finished, with a carpeted floor in the east portion (the recreation room) and a cement floor in the utility room containing an electric clothes washer, a gas-powered clothes dryer, and the gas furnace and hot water heater. Two adult nonsmokers lived in the house. The first floor contains a kitchen/dining area, a bathroom, and living room with fireplace (unused). The second floor contains four rooms: a master bedroom with bath, a guest bedroom, and two rooms used as offices. A plan layout of the house with the locations of the monitoring instruments is included in CitationWallace and Howard-Reed (2002).
The stove is a gas stove with four top burners rated at 2600 W (9000 BTU/h) each and an oven with an upper gas burner (2900 W; 10000 BTU/h) and a lower gas burner (5200 W; 18000 BTU/h). An electric toaster was used for making toast in the mornings. The frying pan used for frying tortillas and eggs was well used (more than 30 years old) with a blackened copper bottom but a clean unmarked metallic frying surface. A range hood vented to the outdoors was present but not used during any of the cooking episodes reported here.
The house's ventilation rate was measured continuously using the tracer decay method as described in ASTM Standard E741 with sulfur hexafluoride (SF6) as the tracer gas and a gas chromatograph with electron capture detector (GC-ECD) detection system. Every 2 h to 4 h a tracer gas (SF6) was injected into the return duct. The gas was sampled through polyethylene tubes sequentially every minute in the mixed return air duct and in 9 rooms of the house including the attic. The GC-ECD was calibrated biweekly using an 18-point calibration system to measure SF6 concentrations between 30 μ g/m3 (5 ppb) and 900 μ g/m3 (150 ppb) with an accuracy of approximately ± 2%.
From July 1999 to March 2001, the central furnace fan was kept operating about 90% of the time in order to achieve better mixing for the calculation of the air change rates. From several traverses across the return air duct, the fan was estimated to move a complete house volume of air through the furnace filter and duct system about 6 times per hour. This would cause increased particle deposition on the furnace filter and in the ducts, leading to generally cleaner air in the rest of the house. In homes where the fan is on only when the furnace or air conditioner is actually running, this extra mode of particle filtration and deposition would be in use a smaller proportion of the time.
A duct-mounted ESP filter (Carrier Model Air AAACCC-14) was installed in late 1999 and was used intermittently throughout the year 2000. Power source failures with the ESP were resolved in early April of 2000, so this had an effect only during the last 9 months of the year. Measurements of upstream and downstream particle concentrations using Climet optical scanners measuring six size fractions from 0.3 to 20 μ m (Climet Instruments, Redlands, CA) indicated that efficiencies when the filter was clean ranged from 90% for the smallest particles (0.3–0.5 μ m) to 99% for the larger particles (5–10 and 10–20 μ m). After about 3–4 weeks of constant use, efficiencies for the smallest particles declined to about 50%, but efficiencies stayed well above 90% for the larger particles. Thorough cleaning was capable of restoring the original efficiencies; such cleaning occurred six times during the 9-month period. The actual reduction of whole-house indoor particle levels was determined over a period of a month (March 2000) and ranged from 50–75% for different size fractions. Considering these factors of intermittent use and intermittent cleaning with varying efficiency between cleanings, it is likely that the ESP reduced indoor concentrations by a moderate amount during the latter part of the year.
A Scanning Mobility Particle Sizer (SMPS; Model 3077, TSI Inc., St. Paul, MN) was used throughout the 37-month sampling period. Two impactor inlets of 0.432 mm and 0.508 mm diameter were used interchangeably in the present study. The first provides a size range of about 10–450 nm; the second a range of 18–950 nm. Each range is divided into about 100 size bins evenly spaced on a logarithmic basis; the overlap leads to 126 bins between 10 nm and 950 nm. A sampling period of 5 minutes was selected for this study. Voltage settings for the two internal flow meters were checked each time the instrument was downloaded (at least every three days). The settings were required to be within 0.1% of the recommended value in the manual. A bubble flowmeter (Gillibrator 2, Sensidyne Inc., Clearwater, FL) was used to set the sample flow to within 0.3% of the recommended flow.
The SMPS was placed in the basement recreation room with its intake about 0.5 m from an outside wall. Outdoor samples were collected by attaching tubing (0.75 m in length) to the intake. No tubing was used for indoor sampling.
During the first year (November 21, 1997 throughout 1998), only the smaller inlet was used; the selected size range was 10.6–400 nm. For the next two years, the two inlets were used interchangeably, alternating every 4–6 weeks. Six size categories based on the differing size ranges of the nozzles were selected for summary purposes: 10–18 nm, 18–50 nm, 50–100 nm, 100–200 nm, 200–450 nm, and 450–950 nm. The first category could only be measured by the smaller inlet, and the last only by the larger one; the four categories in between could be measured by either inlet. In the final statistics, this leads to some discontinuities at the 18 nm and 450 nm breakpoints due to the different time periods that each inlet was used.
The two residents were employed and spent the working hours of most weekdays away from home at their jobs. Breakfasts and dinners were nearly always at home. Breakfast normally included boiling water in a teakettle for tea or coffee, and toasting bread, English muffins, or bagels in an electric toaster or electric toaster oven. Dinners were normally prepared on the gas stove and usually involved both the gas burners and the gas oven. On many days, the type of food and cooking method was recorded.
The times when indoor sources were observed were entered into the electronic database. Periods of elevated concentrations after the source ceased operating were ascribed to that source. For indoor sources, it was normally very easy to detect their beginning (to within 5 minutes, the length of the SMPS cycle time). Sharp increases on the order of minutes were ascribed to indoor air sources; longer trends on the order of hours or days (corresponding to meteorological synoptic periods) were ascribed to outdoor air sources. However, the end of the indoor-source peak was more difficult to determine, since the background concentration may have shifted while the source was on. Fortunately, since these final concentrations are near background, their influence on the total contribution of the peak is small.
The time series of particle concentrations for different types of cooking was studied by identifying each episode and ordering concentrations by the time since the cooking began.
RESULTS
Over the 37-month sampling period, 259,176 five-minute averages (the equivalent of 900 full days) were obtained, a 79% completion rate. Elevated concentrations due to indoor sources were observed about 17.5% of the time (). For the ultrafine particles, average number concentrations with sources active were > 20,000 cm− 3 compared to 2,500 cm− 3 when sources were not active (). Indoor sources were less effective at elevating particle numbers in the accumulation mode (0.1–1 μ m); number concentrations were increased by a factor of about 3.3. Over the full 3-year sampling period, identified indoor sources accounted for 55% of the total ultrafine particles, and 27% of the accumulation mode particles (). These estimates may be slight underestimates, since small increases due to minor indoor sources would not be identified by the algorithm described above.
FIG. 1 Indoor number concentrations while indoor sources are active compared to concentrations due to outdoor particle infiltration. Error bars on means are standard errors.
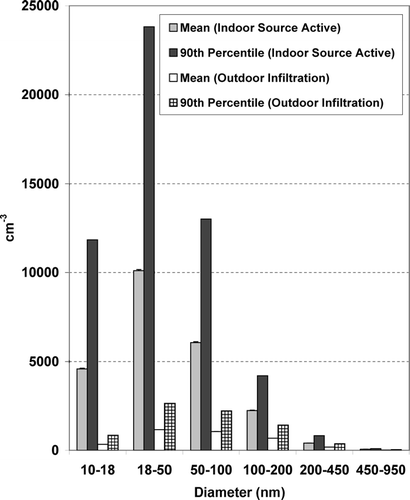
TABLE 1 Number concentrations (cm− 3) for six size ranges
TABLE 2 Indoor and outdoor contributions to particle number concentrations (cm− 3)
A total of 282 different activities affecting number concentrations were recorded. From these, 18 activities were selected for further analysis. These included different types of cooking using a gas stove, electric toaster, and electric toaster oven; other indoor combustion sources, including a gas-powered clothes dryer and a candle; and open windows (considered as a source of outdoor particles). The number of times each activity occurred and the average combined duration both of the activity itself and the subsequent elevation of particle concentrations are shown in . Also shown in are the modal diameters for both the number concentration and the volume (mass) concentration.
TABLE 3 Number and durationFootnote a of 18 selected activities, with modal diameters for both particle number and volume
Four activities had peak concentrations close to and perhaps below the smallest diameter measured (about 10 nm): tea, tea and toast, breakfast, and gas clothes dryer. In all four of these cases, the bulk of the particles were supplied by nearly pure natural gas burning. For the two that also employed a toaster or toaster oven, a secondary peak near 30 nm was noted. A peak at this diameter due to electric toaster ovens was previously noted by CitationWallace (2000), CitationDennekamp et al. (2001), CitationHe et al. (2004), and CitationWallace, Emmerich, and Howard-Reed (2004). Somewhat more complex cooking, such as using the gas burners to cook dinner, stir-fry vegetables, and fry eggs, had modes at to 36–40 nm. Cooking involving the gas oven (broiling fish, baking potatoes) produced modes at somewhat higher diameters (45–46 nm). Burning of a Citronella candle also produced a peak at this diameter. Deep-frying tortillas followed by baking them in the oven resulted in a mode at 64 nm, as did burning incense. Finally, outdoor particles, and also those infiltrating indoors (No Indoor Sources) had modes at 69 nm, the highest modal diameter observed, perhaps due to coagulation of aged particles. The volume modal diameters were much greater than the number modes, ranging from 46 nm for making tea to 638 nm for burning a candle ().
The activity durations supplied in can be combined with the average number concentrations () and volume concentrations () to calculate the excess exposure due to each type of activity. The values in and (except those for the outdoor concentrations) are the differences in concentrations after subtracting the daily average indoor background concentrations (“No indoor sources”). Note that the values for the outdoor concentrations are based on a relatively small number of measurements made at varying times throughout the 3-year monitoring period, and do not represent a true average over that time of outdoor concentrations.
TABLE 4 Size-resolved particle number (cm)− 3 produced by 18 activities
TABLE 5 Size-resolved particle volume (μm/cm)3due to 18 activities
In , the units for volume ((μ m/cm)3) can be multiplied by the density of the aerosol to arrive at a mass. Since combustion particles may have a density close to 1 g/cm3, the volume units translate directly into units of μ g/m3. With this assumption, it can be seen that several of the types of cooking could lead to increased mass concentrations averaging 10–50 μ g/m3 for the duration of the cooking activity followed by the period of elevated concentrations.
The number concentration size distributions of 16 activities are shown in , , , , , . In each figure, the observed size distribution for infiltrated outdoor particles (“No Indoor Source”) is provided as a reference.
FIG. 2 Size distributions of outdoor particles measured outdoors and indoors. “No indoor sources” refers to indoor measurements showing no influence of indoor sources. “Open windows” refers to indoor measurements made with windows open. “Unknown outdoor source” refers to indoor measurements showing slow increases typical of slow outdoor changes in concentration, with no apparent indoor source. “Outdoors” refers to measurements made outdoors. Discontinuities at 18 nm and 440 nm in this and following figures are due to different ranges associated with the two nozzle assemblies.
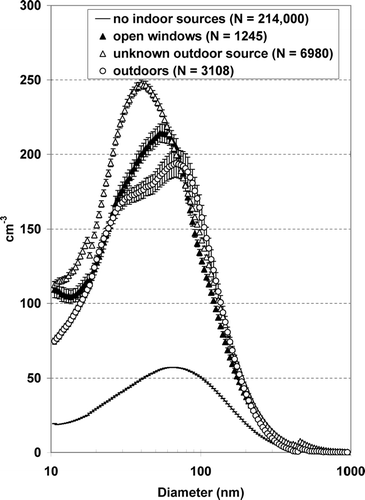
FIG. 3 Size distributions of particles created by the gas burner only (boiling water for tea) and the gas burner and electric toaster or toaster oven.
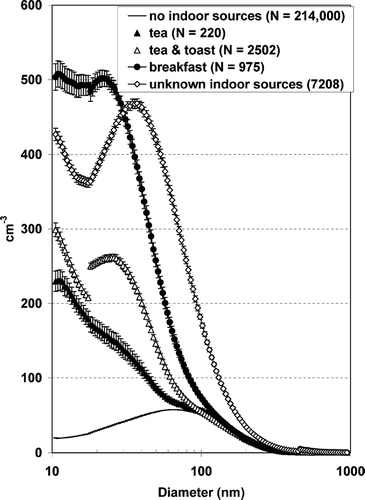
No indoor sources were apparent for 82.5% of the 3-year period. At these times, indoor concentrations are presumably almost entirely due to infiltration of outdoor particles, assuming no low-level continuous indoor particle sources (such as pilot lights) are active. No appliances in this home employed pilot lights. This subset of outdoor-influenced data was investigated for year-to-year variation, seasonal (monthly) variation, day-of-the-week dependence, and diurnal variation.
Year-to-year variation showed no particular trend for any of the six size classes (). 1999 was usually but not always the year with the highest mean number concentrations, ranging from about 10–30% higher than the lowest year (1998).
TABLE 6 Variation of infiltrated outdoor particle number concentration (cm− 3) by size category and by year
Seasonal variation was not marked nor was it consistent across size categories. Ultrafine particles had 13–35% higher concentrations in winter and in spring compared to summer and fall, but the accumulation mode showed the opposite behavior ().
TABLE 7 Variation of infiltrated outdoor particle number concentration (cm− 3) by size category and by month
The smallest particles (10–18 nm) were significantly higher on weekdays than on weekends, perhaps due to increased traffic on weekdays (). Sundays were also significantly lower than Saturdays. The larger ultrafine particles showed little if any dependence on day of the week.
TABLE 8 Variation of infiltrated outdoor particle number concentration (cm− 3) by size category and by day of week
Diurnal variation of the infiltrated outdoor particles was most marked for the smallest particles (10–18 nm), which declined during the night and then increased by a factor of 5 between 5 and 8 AM (). They then stayed nearly constant for the remainder of the daylight hours. The larger ultrafines also showed strong declines during the night and increases during the day. However, particles > 200 nm in diameter showed little diurnal variation.
FIG. 8 Diurnal variation, no indoor sources. The increase observed at dinnertime (6–8 PM) may be partly due to difficulty in detecting minor peaks due to cooking.
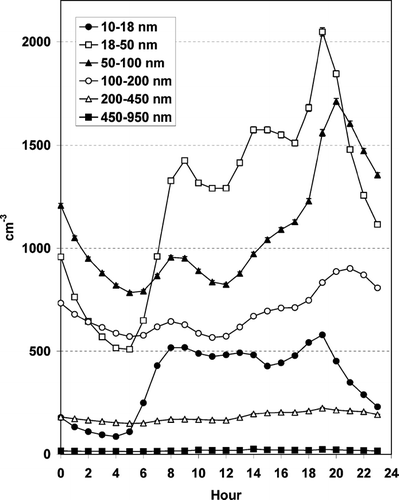
The diurnal variation of the total indoor particles is compared to the infiltrated outdoor particles in . The concentrations when indoor sources were active ranged from 6–10 times the concentrations at times with no indoor sources, with the greatest difference occurring at the dinner hours.
FIG. 9 Diurnal variation, total particles (< 950 nm). When indoor sources are active, the mean particle concentration indoors is 6–10 times that due to infiltration of outdoor particles, with the main contribution coming during dinnertime cooking.
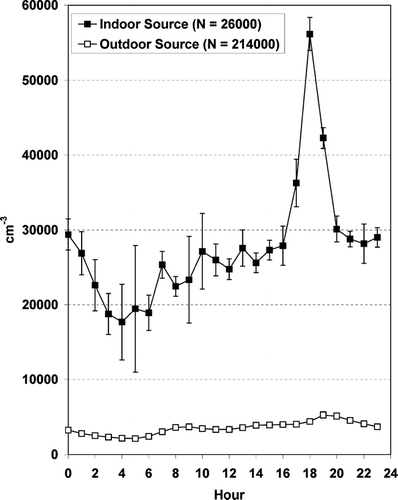
The time series of particle concentrations was studied for a few of the 18 sources. For example, a total of 245 breakfasts of tea and toast were recorded. These consisted of boiling water on a gas burner for about 5–10 minutes and toasting bread in a toaster oven for a few minutes. Since the main effect was shown by ultrafine particles, only the three size categories < 100 nm were considered (). The smallest category (10–18 nm) reached a peak 10 minutes after the start, the next smallest (18–50 nm) 15 minutes after the start, and the next category (50–100 nm) 20 minutes after the start. This could be due to coagulation of the smaller particles as they age.
FIG. 10 Ultrafine particle concentration time course due to boiling water for tea and toasting bread (N = 245 events). Error bars are standard errors.
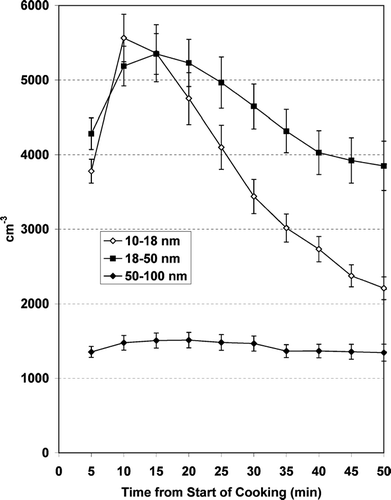
There were 64 dinners consisting of a potato baked in a 500°F oven for 45 minutes followed by broiling fish in the oven for about 8 minutes. The smallest particles (10–18 nm) reached a peak an average of 15 minutes after cooking began, with the largest particles (50–100 nm) reaching a peak 75 minutes after cooking began (). On average, elevated concentrations were observed for 120 minutes after cooking began, with 20 of the episodes lasting 160 minutes or longer.
FIG. 11 Particle concentrations during and after baking a potato in a gas oven for 45 minutes and broiling fish in the oven for 8 minutes. (N = 66 events).
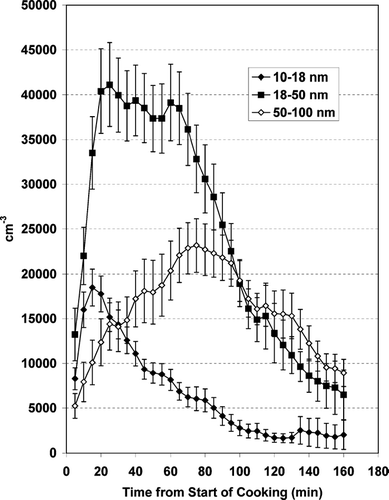
Another common dinner included deep-frying tortillas (74 instances). In this recipe, tortillas are deep-fried in peanut oil on one gas burner while beans are steamed in a double boiler on another gas burner. The tortillas, beans, and Monterey Jack cheese are then baked in the oven at 450°F for about 8 minutes. The smallest size category reached its peak 10 minutes after cooking started, but the next three categories (from 18–200 nm) reached their peaks about 25 minutes after the start ().
FIG. 12 Particle concentrations during and after deep-frying tortillas in peanut oil and then baking them with cheese and beans in an oven for 8 minutes. (N = 74 events).
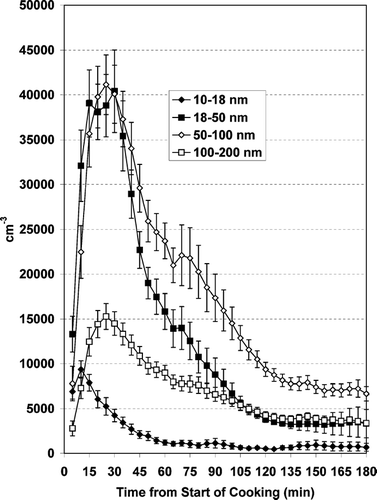
A rough estimate of the size-resolved source strengths of these three common meals may be made by assuming that the peak number and volume concentrations measured by the basement SMPS represent a whole-house average of all the particles released in the cooking event. Since there is a time lag between the creation of the particles and their dispersion throughout the house, and since many of them are forced to go through the ductwork where they have an increased probability of deposition, while others have the possibility of exfiltrating, the resulting source strengths will be slight underestimates. provides the size-resolved average peak number and volume concentrations for each of the three meal types. Using the house volume of 385 cubic meters, the number of particles released per cooking event can be calculated for each of the size categories selected. The total numbers range between 5 × 1012 for the simple tea and toast meal (gas burners plus electric toaster oven) to nearly 4 × 1013 for the more complicated deep-frying and baking of tortillas.
TABLE 9 Size-resolved peak number and volume concentrations and estimated source strengths for common cooking events
Differences between different types of cooking in the size distribution are clear in this table, since more than 80% of the particles released by the tea and toast breakfast are less than 50 nm in diameter, whereas almost 80% of the particles released by the tortilla dinner are between 18 and 100 nm in diameter. The time since the start of cooking at which the peak number and volume concentrations occurred varied considerably depending on the cooking type. For the simple tea and toast breakfast, the peak concentrations occurred 10 minutes after the start of cooking. For the deep-fried tortilla dinners, the peak occurred 25 minutes after the start. For the broiled fish dinner, which began with a 45-minute period of baking a potato, the peak number concentration occurred about 15 minutes after the start, but the peak mass concentration occurred 60 minutes after the start. Finally, assuming spherical particles with a density of 1 g/cm3, the total mass aerosolized per cooking event is calculated. The mass released varies from about 1 mg for the tea and toast breakfast to about 20 mg for the tortilla dinner.
DISCUSSION
Short-term studies including residential indoor ultrafine particle measurements have been carried out by several investigators. CitationAbt et al. (2000) studied 3 homes for 6–12 days each, with 17 selected cooking events providing mean peak volume concentrations of particles between 20 and 500 nm ranging between 29 and 57 (μ m/cm)3. CitationLong et al. (2001) studied 9 homes for 6-12 days each, finding mean peak volume concentrations for ultrafine particles (20–100 nm) of 2.2 to 18.2 (μ m/cm)3. CitationDennekamp et al. (2001) carried out 11 cooking experiments in a laboratory finding peak ultrafine (10–100 nm) particle number concentrations ranging from 11,000 to 590,000 particles/cm3, about 5 times our observed range for three cooking types of 13,000 to 100,000 particles/cm3. Since the volume reported for the laboratory was 70 m3, about 5 times less than our volume, the results match well. CitationHe et al. (2004) studied 15 homes for 48 h at a time while carrying out cooking experiments under high and low ventilation conditions, finding a range of peak submicrometer number concentrations for cooking events between 16,000 and 180,000 particles/cm3, slightly larger than our observed range. CitationHe et al. (2004) did not provide the volumes of their homes, but it may be that the homes (all in Brisbane, Australia) were smaller than the three-story townhouse in this study, which would lead to the slightly higher peak concentrations observed. CitationHe et al. (2004) also developed estimates of the emission rate ranging between 0.2–4 × 1012 particles/min. The number of minutes per cooking event was not provided, but assuming active cooking lasting from 10–20 minutes on average, these values are very comparable to our calculated emission rates of 5 × 1012 up to 4 × 1013 particles per cooking event. Finally, in an earlier publication from the present study, 24 cooking events with high concentrations and well-shaped decay curves, including concurrent air exchange rate measurements, were analyzed more precisely, taking into account losses due to deposition during the lag time required to reach the peak, for their source strengths (CitationWallace, Emmerich, and Howard-Reed 2003). A value of 3 × 1012 ultrafine particles (10–100 nm) per minute was obtained, in good agreement with the upper-range results of CitationHe et al. (2004).
In the present study, sufficient time (> 3 years of continuous monitoring) was allotted to provide adequate number statistics for a variety of sources. However, this is also the most obvious limitation of the study: it deals with only a single home. A second limitation was the necessity of leaving the central HVAC fan on most of the time to ensure adequate mixing of the tracer gas—this is probably not the case for many homes with central heating and air conditioning. One result is a more even distribution of particle concentrations in this home than in those with only intermittent use of the central fan. Also, for this home, the movement of air through the duct system reduced particle concentrations in the house by about 20% due to deposition in the duct (CitationWallace, Emmerich, and Howard-Reed 2004b). In addition, kitchen concentrations in this home would be lowered, and concentrations in the remainder of the home increased, due to the mixing produced by the constantly running central fan. A third limitation is lack of use of the stove exhaust fan, resulting in probable higher concentrations than would otherwise have occurred. A countervailing effect was the intermittent use of a duct-mounted electrostatic precipitator, which reduced whole-house concentrations by an estimated 50% when the ESP was running at high efficiency.
Since all concentrations were measured on a different floor from the kitchen, they represent whole-house averages rather than the concentrations experienced by the cook. The personal exposures of the cook may be several times the whole-house concentrations, as has been shown for larger particles measured by an optical scattering device (CitationWallace 2000).
In this house with two working non-smoking adults, the major indoor source of particles was cooking. Indoor sources accounted for the majority of ultrafine particles (< 0.1 μ m) whereas outdoor sources contributed the majority of the accumulation mode particles (0.1–1 μ m). When the indoor sources were active (17.5% of the time) the total number concentrations produced were 6–10 times the number due to outdoor infiltration.
The particle size distributions produced by gas stove burners, and also by the gas clothes dryer, peaked very close to the lower limit of the SMPS at about 10 nm. This suggests that the total number of particles due to these sources is considerably greater than the amount observed in this study. More recent differential mobility analyzers can measure smaller (3 nm) particles and could be used to measure the total number produced by these two sources more accurately. The size distributions from more complex forms of cooking and from candles and incense burning have peaks at higher diameters (about 35–70 nm) and thus the total number of particles observed for these sources is more likely to be an accurate reflection of the true total.
This study and others suggest that indoor sources can produce ultrafine particle concentrations that are much higher than outdoor peak concentrations, and that indoor sources often provide a substantial fraction of total exposure to ultrafines. A common indoor source is the gas stove, although electrical appliances also appear to produce some ultrafines. The question arises as to whether ultrafines from combustion of natural gas may be of different toxicity than ultrafines from common outdoor sources such as vehicles. One recent study found that most of the mutagenicity of Los Angeles air could be traced to gas appliance emissions rather than automobiles and diesel vehicles (CitationHannigan, Busby, and Cass 2005). Therefore there is some evidence suggesting that ultrafine particles from indoor sources may have important health effects. Further research into their toxicological properties and extent of human exposure seems warranted.
Acknowledgments
Cynthia Howard-Reed, then an EPA post-doctoral fellow, now at the National Institute of Standards and Technology (NIST), supplied valuable engineering capability in keeping the equipment running at high efficiency, and also programmed the reduction of the data from multiple sources to a single database. Andrew Persily of NIST provided the one-of-a-kind GC-ECD for air exchange measurements, and Steve Emmerich and other NIST employees and summer students made regular multipoint calibrations of the instrument for two years. This paper has benefited from the perceptive comments of three peer reviewers.
Notes
a Duration refers to the sum of the time the combustion or other activity was occurring and the subsequent time of elevated concentrations.
REFERENCES
- Abt , E. , Suh , H. H. , Catalano , P. and Koutrakis , P. 2000 . Relative Contribution of Outdoor and Indoor Particle Sources to Indoor Concentrations . Environ. Sci. Tech. , 34 : 3579 – 3587 . [CSA]
- Brook , R. D. , Franklin , B. , Cascio , W. , Hong , Y. , Howard , G. , Lipsett , M. , Luepker , R. , Mittleman , M. , Samet , J. , Smith , S. C. Jr. and Tager , I. 2004 . Air Pollution and Cardiovascular Disease: A Statement for Healthcare Professionals from the Expert Panel on Population and Prevention Science of the American Heart Association . Circulation , 109 : 2655 – 2671 . [PUBMED] [INFOTRIEVE] [CROSSREF] [CSA]
- Brown , J. S. , Zeman , K. L. and Bennett , W. D. 2002 . Ultrafine Particle Deposition and Clearance in the Healthy and Obstructed Lung . Amer. J. Resp. Crit. Care Med. , 166 ( 9 ) : 1240 – 1247 . [CROSSREF] [CSA]
- Chalupa , D. C. , Morrow , P. E. , Oberdörster , G. , Utell , M. J. and Frampton , M. W. 2004 . Ultrafine Particle Deposition in Subjects with Asthma . Environ. Health Persp. , 112 ( 8 ) : 879 – 882 . [CSA]
- Chan , C.-C. , Chuang , K.-J. , Shiao , G.-M. and Lin , L.-Y. 2004 . Personal Exposure to Submicrometer Particles and Heart Rate Variability in Human Subjects . Environ. Health Persp. , 112 ( 10 ) : 1063 – 1067 . [CSA]
- de Hartog , J. J. , Hoek , G. , Peters , A. , Timonen , K. L. , Ibald-Mulli , B. and Brunekreef , B. 2003 . Effects of Fine and Ultrafine Particles on Cardiorespiratory Symptoms in Elderly Subjects with Coronary Heart Disease . Amer. J. Epidemiol. , 157 : 613 – 623 . [CROSSREF] [CSA]
- Dennekamp , M. , Howarth , S. , Dick , C. A. , Cherrie , J. H. W. , Donaldson , K. and Seaton , A. 2001 . Ultrafine Particles and Nitrogen Oxides Generated by Gas and Electric Cooking . Occup. Environ. Med. , 58 : 511 – 516 . [PUBMED] [INFOTRIEVE] [CROSSREF] [CSA]
- Dick , C. A. , Brown , D. M. , Donaldson , K. and Stone , V. 2003 . The Role of Free Radicals in the Toxic and Inflammatory Effects of Four Different Ultrafine Particle Types . Inhal. Toxicol. , 15 ( 1 ) : 39 – 52 . [PUBMED] [INFOTRIEVE] [CROSSREF] [CSA]
- Elder , A. C. , Gelein , R. , Finkelstein , J. N. , Cox , C. and Oberdörster , G. 2000 . Pulmonary Inflammatory Response to Inhaled Ultrafine Particles Is Modified by Age, Ozone Exposure, and Bacterial Toxin . Inhal. Toxicol. , 12 ( Suppl 4 ) : 227 – 246 . [PUBMED] [INFOTRIEVE] [CROSSREF] [CSA]
- Hannigan , M. P. , Busby , W. F. and Cass , G. R. 2005 . Source Contributions to the Mutagenicity of Urban Particulate Pollution . J. Air Waste Manage. Assoc. , 55 : 399 – 410 . [CSA]
- He , C. , Morawska , L. , Hitchins , J. and Gilbert , D. 2004 . Contribution from Indoor Sources to Particle Number and Mass Concentrations in Residential Houses . Atmos. Environ. , 38 : 3405 – 3415 . [CROSSREF] [CSA]
- Hoek , G. , Brunekreef , B. , Goldbohm , S. , Fischer , P. and Van Den Brandt , P. A. 2002 . Association between Mortality and Indicators of Traffic-Related Air Pollution in the Netherlands: A Cohort Study . Lancet , 360 : 1203 – 1209 . [PUBMED] [INFOTRIEVE] [CROSSREF] [CSA]
- Howard-Reed , C. , Wallace , L. A. and Emmerich , S. J. 2003 . Effect of Ventilation Systems and Air Filters on Decay Rates of Particles Produced by Indoor Sources in an Occupied Townhouse . Atmos. Environ. , 37 ( 38 ) : 5295 – 5306 . [CROSSREF] [CSA]
- Howard-Reed , C. H. , Wallace , L. A. and Ott , W. R. 2002 . The Effect of Opening Windows on Air Change Rates in Two Homes . J. Air Waste Manage. Assoc. , 52 : 147 – 159 . [CSA]
- Ibald-Mulli , A. , Wichmann , H. E. , Kreyling , W. and Peters , A. 2002 . Epidemiological Evidence on Health Effects of Ultrafine Particles . J. Aerosol Med. , 15 ( 2 ) : 189 – 201 . [PUBMED] [INFOTRIEVE] [CROSSREF] [CSA]
- Jeong , C.-H. , Hopke , P. K. , Chalupa , D. and Utell , M. 2004 . Characteristics of Nucleation and Growth Events of Ultrafine Particles Measured in Rochester, NY . Environ. Sci. Tech. , 38 ( 7 ) : 1933 – 1940 . [CROSSREF] [CSA]
- Kulmala , M. , Vehkamäki , H. , Pegäjä , T. , Dal Maso , M. , Lauri , A. , Kerminen , V.-M. , Birmili , W. and McMurry , P. H. 2004 . Formation and Growth Rates of Ultrafine Atmospheric Particles: A Review of Observations . J. Aerosol Sci. , 35 : 143 – 176 . [CROSSREF] [CSA]
- LaRosa , L. E. , Buckley , T. J. and Wallace , L. A. 2002 . Real-Time Indoor and Outdoor Measurements of Black Carbon in an Occupied House: An Examination of Sources . J. Air Waste Manage. Assoc. , 52 : 174 – 185 . [CSA]
- Long , C. M. , Suh , H. H. , Catalano , P. and Koutrakis , P. 2001 . Using Time- and Size-Resolved Particulate Data to Quantify Indoor Penetration and Deposition Behavior . Environ. Sci. Tech. , 35 : 2089 – 2099 . [CROSSREF] [CSA]
- Morawska , L. , He , C. , Hitchins , J. , Gilbert , D. and Parappukkaran , S. 2001 . The Relationship between Indoor and Outdoor Airborne Particles in the Residential Environment . Atmos. Environ. , 35 : 3463 – 3473 . [CROSSREF] [CSA]
- Nemmar , A. , Hoet , P. H. , Vanquickenborne , B. , Dinsdale , D. , Thomeer , M. , Hoylaerts , M. F. , Vanbilloen , H. , Mortelmans , L. and Nernery , B. 2002a . Passage of Inhaled Particles into the Blood Circulation in Humans . Circulation , 105 : 411 – 414 . [PUBMED] [INFOTRIEVE] [CROSSREF] [CSA]
- Nemmar , A. , Hoet , P. H. M. , Dinsdale , D. , Vermylen , J. , Hoylaerts , M. F. and Nemery , B. 2002b . Diesel Exhaust Particles in Lung Acutely Enhance Experimental Peripheral Thrombosis . Circulation , 107 : 1202 – 1208 . [CROSSREF] [CSA]
- Nemmar , A. , Hoylaerts , M. F. , Hoet , P. H. and Nernery , B. 2004 . Possible Mechanisms of the Cardiovascular Effects of Inhaled Particles: Systemic Translocation and Prothrombotic Effects . Toxicol. Lett. , 149 : 243 – 253 . [PUBMED] [INFOTRIEVE] [CROSSREF] [CSA]
- Oberdörster , G. , Sharp , Z. , Atudorei , V. , Elder , A. , Gelein , R. , Kreyling , W. and Cox , C. 2004 . Translocation of Inhaled Ultrafine Particles to the Brain . Inhal. Toxicol. , 16 : 437 – 445 . [CROSSREF] [CSA]
- Ogulei , D. , Hopke , P. and Wallace , L. in press . Analysis of Indoor Particle Size Distributions using Positive Matrix Factorization. Accepted by . Indoor Air. , [CSA]
- Olson , D. A. and Burke , J. M. 2006 . Distributions of PM2.5 Source Strengths for Cooking from the Research Triangle Park Particulate Matter Panel Study (2005) . Environ. Sci. Tech. , 40 ( 1 ) : 163 – 169 . [CSA]
- Pekkanen , J. , Peters , A. , Hoek , G. , Tittanen , P. , Brunekreef , B. , De Hartog , J. , Heinrich , J. U. , Ibald-Mulli , A. , Kreyling , W. G. , Lanki , T. , Timonen , K. L. and Vanninen , E. 2002 . Particulate Air Pollution and Risk of ST-Segment Depression During Repeated Submaximal Exercise Tests Among Subjects With Coronary Heart Disease . Circulation , 106 : 933 – 944 . [PUBMED] [INFOTRIEVE] [CROSSREF] [CSA]
- Penttinen , P. , Timonen , K. L. , Tiittanen , P. , Mirme , A. , Ruuskanen , J. and Pekkanen , J. 2001 . Ultrafine Particles in Urban Air and Respiratory Health among Adult Asthmatics . European Respiratory J. , 17 ( 3 ) : 428 – 435 . [CROSSREF] [CSA]
- Peters , A. , Von Klot , S. , Heire , M. , Trentinaglia , I. , Hormann , A. , Wichmann , H. E. and Lowel , H. 2004 . Exposure to Traffic and the Onset of Myocardial Infarction . New England J. Med. , 351 : 1721 – 1730 . [CROSSREF] [CSA]
- Renwick , L. C. , Donaldson , K. and Clouter , A. 2001 . Impairment of Alveolar Macrophage Phagocytosis by Ultrafine Particles . Toxicol. Applied Pharmacol. , 172 ( 2 ) : 119 – 127 . [CROSSREF] [CSA]
- Renwick , L. C. , Brown , D. , Clouter , A. and Donaldson , K. 2004 . Increased Inflammation and Altered Macrophage Chemotactic Responses Caused by Two Ultrafine Particle Types . Occup. Environ. Med. , 61 ( 5 ) : 442 – 447 . [PUBMED] [INFOTRIEVE] [CROSSREF] [CSA]
- von Klot , S. , Wolke , G. , Tuch , T. , Heinrich , J. , Dockery , D. W. , Schwartz , J. , Kreyling , W. G. , Wichmann , H. E. and Peters , A. 2002 . Increased Asthma Medication use in Association with Ambient Fine and Ultrafine Particles . European Respiratory Journal , 20 ( 3 ) : 691 – 702 . [PUBMED] [INFOTRIEVE] [CROSSREF] [CSA]
- von Klot , S. , Peters , A. , Aalto , P. , Bellander , T. , Berglind , N. , D'Ippoliti , D. , Elosua , R. , Hörmann , A. , Kulmala , M. , Lanki , T. , Löwel , H. , Pekkanen , J. , Picciotto , S. , Sunyer , J. , Forastiere , F. and Health Effects of Particles on Susceptible Subpopulations (HEAPSS) Study Group . 2005 . Ambient Air Pollution Is Associated with Increased Risk of Hospital Cardiac Readmissions of Myocardial Infarction Survivors in Five European Cities . Circulation , 112 : 3073 – 3079 . [PUBMED] [INFOTRIEVE] [CROSSREF] [CSA]
- Wainman , T. , Zhang , J. , Weschler , C. J. and Lioy , P. J. 2000 . Ozone and Limonene in Indoor Air: A Source of Submicron Particle Exposure . Environ. Health Persp. , 108 : 1139 – 1145 . [CSA]
- Wallace , L. A. 2000 . Real-Time Monitoring of Particles, PAH, and CO in An Occupied Townhouse . Applied Occup. Environ. Hygiene , 15 ( 1 ) : 39 – 47 . [CROSSREF] [CSA]
- Wallace , L. A. and Howard-Reed , C. H. 2002 . Continuous Monitoring of Ultrafine, Fine, and Coarse Particles in a Residence for 18 Months in 1999–2000 . J. Air Waste Manage. Assoc. , 52 ( 7 ) : 828 – 844 . [CSA]
- Wallace , L. A. , Howard-Reed , C. H. and Emmerich , S. J. 2002 . Continuous Measurements of Air Change Rates in An Occupied House for One Year: the Effect of Temperature, Wind, Fans, and Windows . J. Expo. Anal. Environ. Epidemiol. , 12 : 296 – 306 . [PUBMED] [INFOTRIEVE] [CROSSREF] [CSA]
- Wallace , L. A. , Emmerich , S. J. and Howard-Reed , C. 2004a . Source Strengths of Ultrafine and Fine Particles due to Cooking With a Gas Stove . Environ. Sci. Tech. , 38 ( 8 ) : 2304 – 2311 . [CROSSREF] [CSA]
- Wallace , L. A. , Emmerich , S. J. and Howard-Reed , C. 2004b . Effect of Central Fans and In-Duct Filters on Deposition Rates of Ultrafine and Fine Particles in an Occupied Townhouse . Atmos. Environ. , 38 ( 4 ) : 405 – 413 . [CROSSREF] [CSA]
- Wallace , L. A. 2005a . Ultrafine Particles from a Vented Gas Clothes Dryer . Atmos. Environ. , 39 : 5777 – 5786 . [CROSSREF] [CSA]
- Wallace , L. A. 2005b . Real-Time Measurements of Black Carbon Indoors and Outdoors: A Comparison of the Photoelectric Aerosol Sensor and the Aethalometer . Aerosol Sci. Technol. , 39 : 1015 – 1025 . [CROSSREF] [CSA]
- Weschler , C. J. and Shields , H. C. 1999 . Indoor Ozone/Terpene Reactions as a Source of Indoor Particles . Atmos. Environ. , 33 : 2301 – 2312 . [CROSSREF] [CSA]
- Weschler , C. J. and Shields , H. C. 2003 . Experiments Probing the Influence of Air Exchange Rates on Secondary Organic Aerosols Derived from Indoor Air Chemistry . Atmos. Environ. , 37 : 5621 – 5631 . [CROSSREF] [CSA]
- Wichmann , H. E. , Spix , C. , Tuch , T. , Wolke , G. , Peters , A. , Heinrich , J. , Kreyling , W. G. and Heyder , J. 2000 . Daily Mortality and Fine and Ultrafine Particles in Erfurt, Germany Part I: Role of Particle Number and Particle Mass . Health Effects Inst. , 98 : 5 – 86 . [CSA]
- Wiedensohler , A. , Wehner , B. and Birmili , W. 2002 . Aerosol Number Concentrations and Size Distributions at Mountain-Rural, Urban-Influenced Rural, and Urban-Background Sites in Germany . J. Aerosol Med. , 15 ( 2 ) : 237 – 243 . [PUBMED] [INFOTRIEVE] [CROSSREF] [CSA]
- Zhu , Y. , Hinds , W. C. , Kim , S. and Sioutas , C. 2002 . Concentration and Size Distribution of Ultrafine Particles Near a Major Highway . J. Air Waste Manage. Assoc. , 52 ( 9 ) : 1032 – 1042 . [CSA]