Abstract
A prototype variable supersaturation condensation particle sizer (VSCoPS) capable of measuring particle size distributions from 5 to 30 nm has been developed. This system design is adapted from existing condensation particle counter (CPC) technology with three significant differences: (1) the working fluid is a perfluorinated organic compound that is nonreactive toward, and not an effective solvent for, most laboratory or ambient particle compositions; (2) the vapor pressure of the working fluid is controlled by dilution of saturated air with vapor-free air at the same temperature; and (3) the optical block and condenser are located below the saturator, so that fluid condensed on the condenser walls does not flow back toward the saturator. By using fast-response flow controllers to vary the ratio of saturator and dilution air while keeping total flows and temperatures constant, the vapor saturation ratio in the condenser can be controlled with time constants of ∼ 1 s. The nucleation threshold diameter is changed by stepping through small increments in saturation ratio. The particle size distribution can be recovered by inverting the measured concentration using the known instrument response for each saturation ratio. Further development of the system may allow size distribution measurements to smaller particle diameters and scan times of < 30 s at total particle concentrations as low as ∼ 100 cm− 3 .
INTRODUCTION
Nanoparticle size distribution measurements are used for environmental and process-control monitoring, combustion and health-related studies, and nanotechnology research applications. Current technologies for such measurements include impaction with electrical sensing (CitationKeskinen et al. 1992; CitationMaricq et al. 2000) and various forms of differential electrical mobility spectrometry (CitationKnutson and Whitby 1975; CitationWang and Flagan 1990). These techniques are accurate and well studied, but can lack sensitivity at low particle concentrations, thus requiring relatively long integration times, and are relatively expensive. Other techniques, including pulse-height analysis for sizing droplets produced by condensation particle counters (CPCs) and using temperature control to vary the nucleation threshold diameters of CPCs, have been attempted. The pulse-height analysis technique has been useful in studying some cases of particle nucleation and growth (CitationSaros et al. 1996; CitationMarti et al. 1996), but is limited in its size range and has displayed composition-dependent size biases (CitationBall et al. 1999; CitationHanson et al. 2002; CitationO'Dowd et al. 2004). Controlling CPC saturation ratios by varying temperatures of the condenser and/or saturator has shown promise, but has not been actively pursued due in part to slow thermal response which would limit size distribution measurement to tens of minutes with current CPCs (CitationWiedensohler et al. 1994; CitationMcDermott et al. 1991).
We have developed a prototype instrument that uses flow control to vary the saturation ratio in the condenser of a CPC. Initial data show that this technique can determine the size distribution of laboratory-generated particles over the range from 5 to 30 nm with a sizing accuracy similar to the SMPS system but with slightly lower size resolution response to nearly-monodisperse aerosols. In this paper we describe the technique and the instrument's response during initial testing.
METHOD
Design
The VSCoPS instrument () is a continuous-flow CPC that sheaths an aerosol stream with a vapor-laden flow. Critical dimensions and operating parameters are given in and , and are similar to those reported by CitationMcDermott et al. (1991). There are three significant differences between the VSCoPS and typical sheathing CPCs: the working fluid, the mechanism for control of saturation ratio in the condenser, and the location of the condenser and optical block below the saturator. The working fluid of the VSCoPS instrument is a perfluorinated organic compound, perfluorotributylamine, (FluorinertTM FC-43, 3M Performance Materials, St. Paul, MN, USA). Fluorinert is unreactive toward most inorganic and organic compounds, with the exception of other perfluorinated species. It is not an effective solvent and is immiscible with water. As a result of these properties, Fluorinert FC-43 is not expected to show a sensitivity in Kelvin diameter due to reactivity or solubility effects. In testing a different CPC operating with Fluorinert FC-43, CitationBrock et al. (2000) found no detectable change in sizing sensitivity toward nearly spherical particles composed of silver, ammonium sulfate, or sulfuric acid. Direct substitution of FluorinertTM FC-40 and FC-43 for butanol has been attempted with some commercial CPCs, but performance of these instruments has not been satisfactory at tropospheric pressures and is not recommended without changes to geometry and/or operating flows and temperatures (CitationHermann et al. 2005).
FIG. 1 Schematic diagram of the VSCoPS. Three flows—aerosol, dilution, and saturator—are brought to identical temperatures of 49.0°C. The dilution and saturator flows are mixed, then used to sheath the aerosol flow prior to the condenser, which is chilled to 11.0°C. The vapor concentration is controlled by varying the ratio of saturator to dilution flows. Aerosol flow is the difference between the exhaust flow and the saturator and dilution flows. MFC = mass flow controller.
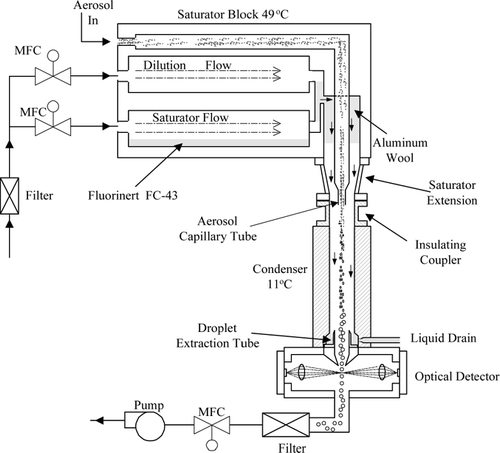
TABLE 1 Critical mechanical dimensions of the VSCoPS
TABLE 2 Operating parameters of the VSCoPS
The nucleation of particles and their growth to detectable droplet sizes within the CPC is governed largely by the peak supersaturation the particles encounter in the condenser. The peak supersaturation is a function of the thermodynamic properties of the vapor (including its mass diffusivity in air, which has not been reported by the manufacturer), the sheath and aerosol flowrates, the temperatures of the saturator and condenser, the condenser geometry, and the vapor concentration. Peak supersaturations occur along the axis of the condenser, where the aerosol flow is introduced and constrained by the surrounding sheathing flow. In the VSCoPS system, all parameters are kept constant with the exception of the vapor concentration. The vapor concentration in the sheathing flow is controlled by diluting the warmed saturator flow, which contains FC-43 vapor at saturation conditions at 49°C, with a vapor-free, particle-free flow warmed to the same temperature (). The flows are well mixed by passing through a volume within the warmed saturator block packed with aluminum wool. The mixed, diluted saturator flow is used to sheath the aerosol sample flow prior to entry in the condenser. This sheathing flow rate is kept constant, but its vapor concentration is varied by controlling the ratio of dilution to saturator air. Mass flow controllers with time responses of < 1 s and accuracy and precision better than 1% of full scale (Model C180HAXF, Celerity Group Inc., Yorba Linda, CA, USA) are used to control the vapor pressure. Total flow through the system is governed by a flow controller downstream of the optical sensor; aerosol flow is the difference between this total flow and the saturator and dilution flows, and is accurate to within 9%.
In a typical CPC, fluid condensed on the walls of the condenser flows by gravity back into the saturator block. In the VSCoPS, such wetted walls prior to the condenser would result in an uncontrolled introduction of vapor into the sheathing flow as the fluid warmed to the temperature of the saturator block. To avoid this, the VSCoPS was constructed with the saturator above the condenser, and a fluid trap and drain built into the walls of the condenser just upstream of the optical droplet detector. There have been no cases of fouling of the detector optics due to liquid contamination during operation of the VSCoPS.
Calibration
At fixed conditions of temperature and total and sample flow, the particle detection efficiency of the VSCoPS varies as a function of particle diameter and the ratio of saturator to dilution flow. To determine the detection efficiency as a function of particle diameter, or response curve, for a specific flow ratio, a laboratory calibration system composed of a particle generator and particle detector was used. Particles were produced by ion-assisted nucleation from trace low-vapor-pressure hydrocarbons present as contaminants in an N2 gas cylinder (Brock and Lovejoy manuscript in preparation). A nearly monodisperse aerosol was selected using a nano-DMA (Model 3085, TSI Inc., St. Paul, MN, USA) operating with sheath and sample flows of 10 and 1 volumetric liters per minute (lpm). This aerosol flow was diluted with filtered room air and split to the VSCoPS, a TSI 3025A ultrafine CPC operating in high flow mode, and, for comparison studies, a scanning differential mobility particle sizer (Model 3936N25, TSI Inc., St. Paul, MN, USA) using a TSI 3786 water CPC as the detector. Tubing lengths were sized to equalize diffusion losses among the detectors assuming laminar pipe flow.
The detection efficiency of the VSCoPS was determined relative to the TSI 3025A CPC, which has high counting efficiency for particles larger than ∼ 3.5 nm (CitationStolzenburg and McMurry 1991). The VSCoPS response at a single setting of saturator/dilution flow ratio shows sharp response characteristics when that ratio is large (). Note that, since the calibration aerosol is not perfectly monodisperse, a CPC with an infinitely sharp nucleation threshold diameter would appear to have a linearly sloping response function extending over ± 5–6% of the 50% efficiency diameter. For small saturator/dilution flow rates (i.e., low supersaturations), the response curve is less sharp as a function of particle diameter, the total counting efficiency is lower, and the repeatability is poorer (). The low detection efficiency at low supersaturations is likely due to the supersaturation region in the condenser becoming smaller than the sheathed aerosol flow, to depletion of vapor by nucleated droplets, and/or to kinetic effects such as inadequate nucleation or droplet growth times.
FIG. 2 (a) Counting efficiency of the VSCoPS at a high ratio of saturator to dilution air (triangles) and a low ratio of saturator to dilution air (squares). Filled and open symbols show two calibrations 10 days apart. The error bars on the open symbols show the total error due to counting statistics and flow uncertainties. (b) Counting efficiency curves manually fit to calibration data for 25 different values of saturator to dilution flow ratio. These response curves are used in the solution of Equation (Equation1).
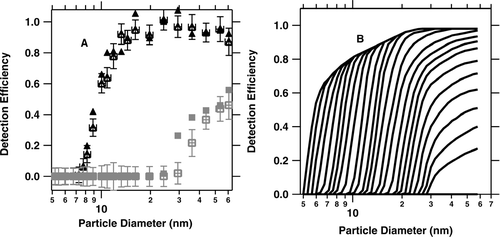
To use the VSCoPS as a sizing instrument, a set of response functions at predetermined values of vapor supersaturation must be determined. Lacking adequate thermodynamic data, we have not numerically simulated the distribution of vapor supersaturation in the condenser to determine the vapor concentration conditions to nucleate a specific size. Instead, we have empirically determined the response of the instrument to a range of saturator/dilution flow ratios for a fixed sample and total flow, with the goal to measure approximately 30 different response functions per decade of particle diameter (). The uncertainty in determining these counting efficiency curves is governed by flow uncertainties and counting statistics ().
Operation
To measure a size distribution, the VSCoPS flow ratios were stepped through each of the flow ratios that produced the response curves in , dwelling on each flow setting for four seconds. Particle count rates were measured at 0.5 s intervals. The response time of the VSCoPS to large step changes in flow ratio was ∼ 1 s with a time lag due to transit in the condenser and optics of ∼ 2 s. After adjusting for the time lag and allowing a 2 s settling time, 2 s of counts were recorded and the concentration determined from the known sample flowrate. The saturator/dilution flow ratios were stepped from high to low, then returned to a high value to repeat the cycle.
The size distribution is determined by solving the Fredholm integral equation,
PERFORMANCE
Four different, nearly monodisperse test aerosols were produced from the nano-particle source and nano-DMA, and were then measured by the VSCoPS and SMPS (). Single, 120 s scans of the VSCoPS resulted in recovered size distributions with peak diameters that agreed with the test aerosol diameter within experimental uncertainty (7%). The integrated number concentration of the VSCoPS size distribution agreed within combined experimental uncertainty (∼ 15%) with that from the CPC in three of the four cases. The test aerosol had a full-width-at-half-max (FWHM) of 5 to 6% of the peak diameter, not including diffusional broadening. The size distributions measured by the VSCoPS were broader, with FWHM ranging from 15 to 22% of the peak diameter, and were negatively skewed. This broadening and skewing effect is evident for the nearly monodisperse test aerosol, but is not likely to significantly bias measurements of moderately polydisperse aerosol size distributions.
FUTURE DEVELOPMENT
Initial testing of the variable condensation CPC technique demonstrates that the diameter of nucleation (Kelvin diameter) can be controlled from 5 to 30 nm with sufficient precision to allow size distributions to be determined over time scales of less than two minutes. The time response of the system is ∼ 1 second to changes in flows, suggesting that scans of particle size distributions could be accomplished in < 30 s if concentrations are sufficient to permit adequate counting statistics. Operation in a true scanning, rather than stepping, mode might allow optimization of the response time and the opportunity for filtering and deconvolution in the frequency domain. Potential dynamic effects on droplet nucleation and growth in such a changing supersaturation environment might complicate recovery of the size distribution, however.
Diffusional losses for particles with D p < 10 nm were significant due to the low aerosol sample flowrate and its long path through the saturator block. Shortening this path and/or increasing the aerosol flowrate would substantially reduce these losses while still warming the aerosol flow to the saturator temperature. The lower size limit of the instrument might be reduced by operating with a larger temperature difference between the saturator and condenser. The detection efficiency and controllability at sizes larger than 20 nm might be improved by operating with a higher vapor concentration (higher saturator and condenser temperatures), or by lengthening the condenser to increase the time available for nucleation and growth at low supersaturations. The indication by CitationHermann et al. (2005) of the presence of composition-dependent nucleation diameters in an FC-43 CPC (qualitatively similar to the VSCoPS threshold control issues at low supersaturations, ) should also be thoroughly investigated.
DISCLAIMER
The use of trade, firm, or corporation names in this publication is for the information and convenience of the reader. Such use does not imply an official endorsement or approval by the University of Colorado, the United States Department of Commerce, or the National Oceanic and Atmospheric Administration of any product or service to the exclusion of others that may be suitable.
This research was supported through the Innovative Research Program of the Cooperative Institute for Research in Environmental Sciences (CIRES) at the University of Colorado. We thank Darin Toohey, Shelly Miller, and David Henderson for their contributions to an earlier prototype of this instrument.
Notes
1Taper 25ˆ from 1.9 cm to 0.64 cm.
1At 1013 hPa and 0°C.
2Sum of saturator and dilution flows.
REFERENCES
- Ball , S. M. , Hanson , D. R. , Eisele , F. L. and McMurry , P. H. 1999 . Laboratory Studies of Particle Nucleation: Initial Results for H2SO4, H2O, and NH3 Vapors . J. Geophys. Res. , 104 : 23709 – 23718 . [CSA]
- Brock , C. A. , Schröder , F. , Kärcher , B. , Petzold , A. , Busen , R. and Fiebig , M. 2000 . Ultrafine Particle Size Distributions Measured in Aircraft Exhaust Plumes . J. Geophys. Res. , 105 ( 26 ) : 555 – 22 . 567.[CSA]
- Hanson , D. R. , Eisele , F. L. , Ball , S. M. and McMurry , P. H. 2002 . Sizing Small Sulfuric Acid Particles with an Ultrafine Condensation Nucleus Counter . Aerosol Sci. Technol. , 36 : 554 – 559 . doi:10.1080/02786820252883793.[CROSSREF] [CSA]
- Hermann , M. , Adler , S. , Caldow , R. , Stratmann , F. and Wiedensohler , A. 2005 . Pressure-Dependent Efficiency of a Condensation Particle Counter Operated with FC-43 as Working Fluid . J. Aerosol Sci , 36 : 1322 – 1337 . [CROSSREF] [CSA]
- Keskinen , J. , Pietarinen , K. and Lehtimäki , M. 1992 . Electrical Low Pressure Impactor . J. Aerosol Sci. , 23 : 353 – 360 . [CROSSREF] [CSA]
- Knutson , E. O. and Whitby , K. T. 1975 . Aerosol Classification by Electric Mobility: Apparatus Theory and Applications . J. Aerosol Sci. , 6 : 443 [CROSSREF] [CSA]
- Maricq , M. M. , Podsiadlik , D. H. and Chase , R. E. 2000 . Size Distributions of Motor Vehicle Exhaust PM: A Comparison Between ELPI and SMPS Measurements . Aerosol Sci. Technol. , 33 : 239 – 260 . doi:10.1080/027868200416231.[CROSSREF] [CSA]
- Markowski , G. R. 1987 . Improving Twomey's Algorithm for Inversion of Aerosol Measurement Data . Aerosol Sci. Technol. , 7 : 127 – 141 . [CSA]
- Marti , J. , Weber , R. , Saros , M. and McMurry , P. H. 1996 . Modification of the TSI 3025 Condensation Particle Counter for Pulse Height Analysis . Aerosol Sci. Technol. , 25 : 214 – 218 . [CSA]
- McDermott , W. T. , Ockovic , R. C. and Stolzenburg , M. R. 1991 . Counting Efficiency of an Improved 30 Angstrom Condensation Nucleus Counter . Aerosol Sci. Technol. , 14 : 278 – 287 . [CSA]
- O'Dowd , C. D. , Aalto , P. P. , Yoon , Y. J. and Hämeri , K. 2004 . The Use of the Pulse Height Analyser Ultrafine Condensation Particle Counter (PHA-UCPC) Technique Applied to Sizing of Nucleation Mode Particles of Differing Chemical Composition . J. Aerosol Sci. , 35 : 205 – 216 . [CROSSREF] [CSA]
- Saros , M. T. , Weber , R. J. , Marti , J. J. and McMurry , P. H. 1996 . Ultrafine Aerosol Measurement Using a Condensation Nucleus Counter with Pulse Height Analysis . Aerosol Sci. Technol. , 25 : 200 – 213 . [CSA]
- Stolzenburg , M. R. and McMurry , P. H. 1991 . An Ultrafine Aerosol Condensation Nucleus Counter . Aerosol Sci. Technol. , 14 : 48 – 65 . [CSA]
- Wang , S. C. and Flagan , R. C. 1990 . Scanning Electrical Mobility Spectrometer . Aerosol Sci. Technol. , 13 : 230 – 240 . [CSA]
- Wiedensohler , A. , Aalto , P. , Covert , D. , Heintzenberg , J. and McMurry , P. H. 1994 . Intercomparison of Four Methods to Determine Size Distributions of Low-Concentration (∼ 100 cm− 3), Ultrafine Aerosols (3 < D p < 10 nm) with Illustrative Data from the Arctic . Aerosol Sci. Technol. , 21 : 95 – 109 . [CSA]