Single particle measurements were made in Baltimore, Maryland from March to December 2002 using a real-time single particle mass spectrometer, RSMS-3. Particle composition classes were identified that indicated how the aerosol composition changed with time. The results were compared with collocated instruments giving particle number concentrations and size distributions, sulfate, nitrate, organic, and elemental carbon mass concentrations and total mass. Examination of these measurements revealed several particulate matter (PM) events in which the 24 h averaged PM 2.5 mass exceeded 30 μ g/m 3 . Three of these events were studied in further detail by comparing number and mass concentrations obtained by RSMS-3 with standard methods. For all three events, the number concentrations obtained with RSMS-3 and a scanning mobility particle sizer were highly correlated (R 2 ∼ 0.7). For the event characterized by a high sulfate mass concentration, the RSMS-3 provided an accurate measure of time-dependent nitrate and carbon mass concentrations, but not for sulfate and total mass. For the two events characterized by high carbon mass concentrations (one from a transcontinental wildfire and the other from stagnation during a period of high traffic), RSMS-3 provided an accurate measure of time-dependent nitrate mass, carbon mass and total mass when the aerosol was not dominated by particles outside the size limit of RSMS-3. While the time dependencies were strongly correlated, the absolute mass or number concentrations determined by RSMS-3 were sometimes off by a constant value, which permitted the relative detection efficiencies of some particle classes to be estimated. Other factors that inhibit reconciliation of mass- and number- based concentration measurements are discussed including the difficulty of detecting ammonium sulfate by laser ablation/ionization and the varying size ranges of different particle measurement methods.
INTRODUCTION
Ambient aerosol physiochemical properties vary by source and atmospheric transformation. Continuous measurements of particulate matter and its chemical components permit the observation of transient events that filter based methods cannot capture. Real-time information about the size distribution and composition of particles in urban air allows for the investigation of source strengths, atmospheric chemical processes, and the health effects these particles induce. Toward that end, continuous monitors capable of measuring mass concentrations including total mass (PM2.5), sulfate, nitrate, and carbon (both organic and elemental) were deployed at the Baltimore Supersite. These monitors were complemented by instruments capable of measuring number concentrations including a scanning mobility particle sizer (SMPS) and a real-time single particle mass spectrometer (RSMS-3), the latter providing size-dependent chemical composition. The RSMS-3 is a single-particle, dual-polarity, time-of-flight mass spectrometer that has been deployed in several urban sites. (CitationRhoads et al. 2003; CitationLake et al. 2003; CitationPhares et al. 2003; CitationTolocka et al. 2005). In Baltimore, over 380,000 particles were analyzed by RSMS-3 between March and December 2002.
Number concentration measurements from an SMPS are not typically used for reconstructing mass concentrations (CitationBrundish et al. 2005) because of the variation of density and shape of the ambient particles (CitationMorawska et al. 1998). Only a few correlations have been made between particle counting systems and mass based measurements. CitationSioutas et al. (1999) found that SMPS measurements for monodisperse aerosols were in good agreement with gravimetric analysis for particle sizes greater than 300 nm. However, the SMPS was found to overestimate the mass concentration by a factor of ca. 1.5 for both monodisperse and polydisperse size distributions less than 300 nm. CitationMorawska et al. (1999) examined the correlation between particle mass and number for both ambient and laboratory aerosols. For high concentrations of laboratory aerosols (50–1,000 μg/m3) the correlations were quite high (R2 > 0.95, accounting for 95% of the variability). For mass concentrations less than 10 μg/m3, correlations rarely accounted for more than 60% of the variability due to changes in particle density. The SMPS has also been used in conjunction with a mass based instrument, the Aerodyne Aerosol Mass Spectometer (AMS), to determine particle morphology and density by combining the mobility and vacuum aerodynamic diameter for the same particle populations (CitationDeCarlo et al. 2004) and to characterize an aerodynamic particle inlet (CitationZhang et al. 2004).
Another single particle mass spectrometer, the ATOFMS, has been used to reconcile chemically resolved number and mass concentrations at two locations (CitationAllen et al. 2000; CitationBhave et al. 2002; CitationWenzel et al. 2003). Initially, ATOFMS detection efficiencies were calculated from size segregated mass concentration data obtained with a micro-orifice impactor (MOI), and the time-dependent ATOFMS data for particles between 0.3 and 1.8 μ m dia. were found to correlate well with total mass and individual chemical species measurements by other methods (CitationAllen et al. 2000). Subsequently, scaling factors were used to determine an underreported particle class by ATOFMS, presumably ammonium sulfate (CitationWenzel et al. 2003).
The ability of RSMS-3 to determine mass concentrations has not been fully explored. Unlike the version of the ATOFMS used in the above referenced experiments, RSMS-3 permits single particle measurements down to ca. 0.05 μ m dia., but with limited capability above 1 μ m dia. While this size range provides more complete characterization of fine and ultrafine particles, reconstructed mass concentrations with RSMS-3 may be in error if a majority of the mass resides in particles 1 μ m dia. or larger.
Six particulate matter (PM) events, defined here as time periods when the 24 hour averaged PM2.5 exceeded 30 μ g/m3, were observed in 2002 at the Baltimore Supersite (CitationPark et al. 2005). During these events, a single chemical component, either sulfate or carbon was found to dominate the particulate mass. In addition, the ambient number concentration and RSMS-3 particle hit rate were correspondingly high. The high mass and number concentrations, coupled with the singular nature of the aerosol, provide a unique opportunity to investigate correlations between the RSMS-3, a particle number-based instrument, and other mass- and number-based measurements. Factors that inhibit the reconciliation of number- and mass based measurements are discussed.
EXPERIMENTAL
RSMS-3 measurements at the Baltimore Supersite have been previously discussed (CitationLake et al. 2003, Citation2004; CitationTolocka et al. 2004a, Citation2004b, Citation2005). In brief, particles were aerodynamically selected and passed through a series of differentially pumped stages into the mass spectrometer. Individual particles were ablated and ionized in-flight using an excimer laser at 193 nm. Positive and negative ion spectra were collected from 0 to +/−250 m/z for each particle. The positive ion spectra were classified using Art2-a (CitationCarpenter et al. 1991; CitationPhares et al. 2001). Over 99% of the 380,000 particles analyzed could be described by 10 major chemical composition classes (CitationTolocka et al. 2005). The most prevalent composition class, representing almost 40% of the particles in Baltimore aerosol over the eight-month period, corresponded to internally mixed particles containing organic and possibly elemental carbon, ammonium nitrate and ammonium sulfate (OCANS). A carbon class accounted for approximately 30% of the particles in Baltimore aerosol, and an ammonium nitrate class accounted for approximately 10% of the particles. A potassium/potassium-sodium class (including particles from a wildfire event that will be discussed below) accounted for another 10% or the particles. The remaining 10% of particles were split among several transition and/or heavy metal classes. Because of the difficulty in detecting sulfate in positive ion spectra, no distinct sulfate class was identified by Art 2-a analysis other than OCANS. Instead, particulate sulfate was monitored through the negative ion signature of particles in the various classes described above. Details concerning sulfate detection are given in CitationLake et al. (2004).
Continuous mass measurements were made with an R&P Model 1400a Tapered Element Oscillating Microbalance (TEOM). Continuous monitors of elemental and organic carbon (R&P Model 5400 East Greenbush, NY), sulfate (R&P Model 8400S, East Greenbush, NY), and nitrate (R&P Model 8400N, East Greenbush, NY) were also employed (CitationHarrison et al. 2004; CitationPark et al. 2005). A Scanning Mobility Particle Sizer (SMPS) (Electrostatic Classifier Model 3080 and CPC Model 3025A; TSI Inc., St. Paul, MN) provided the number concentration and particle size distribution for mobility diameters between 10 and 440 nm as a function of time.
For detection efficiency measurements of laboratory aerosols, polydisperse aerosols of desired composition were generated with a TSI Constant Output Atomizer (Model 3076, TSI Inc., St. Paul, MN). The aerosol output line was split to the RSMS-3 and SMPS. Conductive tubing (1/4″ o.d.) was used to transport the generated aerosol to RSMS-3 and to the SMPS. The SMPS was used to measure the particle size distribution leaving the atomizer. During the analysis of each solution, the distribution was measured at least three times to ensure output consistency. Ethanol or 50/50 ethanol-water solutions containing ammonium nitrate, ammonium sulfate and oleic acid were atomized. A 1:1 oleic acid and ammonium sulfate solution and a separate 1:1:1 oleic acid, ammonium sulfate, and ammonium nitrate solution were analyzed.
RESULTS AND DISCUSSION
Events Description
Six events, designated as “A” through “F” by (CitationPark et al. 2005), were observed in which the 24 h averaged PM2.5 mass exceeded 30 μ g/m3. Three of these events were studied in more detail to investigate the specific challenges of reconciling RSMS-3 measurements with standard methods. Event “D” began on August 11 and continued to August 14. About 80% of the PM mass was attributable to ammonium sulfate, while 15% was due to organic carbon. The aerosol is believed to have been transported from the Ohio River Valley (CitationPark et al. 2005). High sulfate mass concentrations were observed in Events A, B, and E. Because of their similarity with Event D, they are not discussed here. Event C, characterized by a high carbon mass concentration, was associated with long range transport of aerosol from Canadian wildfires (CitationSapkota et al. 2005) between the 5th and 8th of July. Event F occurred between the 20th and the 21st of November and was characterized by a high carbon mass concentration owing to stagnant air during high traffic conditions (CitationPark et al. 2005).
RSMS-3 Number and Mass Concentrations
Particle number concentrations were calculated from the rate of single particle data collection with RSMS-3:
Size-dependent detection efficiencies for RSMS-3 averaged over all ambient particle composition classes in Baltimore aerosol are shown in (CitationLake et al. 2003). Not surprisingly, the detection efficiency decreases with decreasing particle size. Also shown in are detection efficiencies for laboratory generated particles having related compositions—ammonium nitrate, oleic acid (representative of an organic particle), and mixtures of oleic acid with ammonium sulfate and/or ammonium nitrate. As expected from previous work with an earlier version of RSMS (CitationKane and Johnston 2000), ammonium nitrate particles give higher detection efficiencies than the ambient particle average because the ablation efficiency is inherently large and the hygroscopic character of these particles promotes a nearly spherical morphology. The difference between the ammonium nitrate and ambient particle detection efficiencies is relatively low at small particle sizes because ambient particles in this size range are composed primarily of chemical species that are easily detected (ammonium nitrate, elemental carbon, potassium). As expected, particles composed of oleic acid with or without ammonium sulfate/nitrate are much smaller than ambient particles at small particle sizes as these chemical compositions give lower ablation efficiencies. As the particle size increases, internally mixed OCANS (organic carbon, ammonium nitrate and sulfate) particles become the dominant composition type in ambient aerosol. Therefore, it is not surprising that as the particle size increases, the ammonium nitrate detection efficiency increases more rapidly than that for ambient particles. What is surprising is that the detection efficiencies of oleic acid particles with or without ammonium sulfate and nitrate eventually become greater than those for ambient particles as the particle size increases. The large detection efficiencies for the laboratory particle compositions most likely are the result of the liquid oleic acid component that maintains a spherical particle morphology. In contrast, ambient OCANS particles are more likely to contain solid organic matter that combined with solid ammonium sulfate and/or nitrate do not produce a spherical morphology. The high detection efficiencies of laboratory-generated oleic acid—ammonium sulfate and/or nitrate particles suggests that ambient OCANS particles are also efficiently detected.
FIG. 1 Detection efficiency versus vacuum aerodynamic diameter (Dva) for ambient Baltimore aerosol (CitationLake et al. 2003) and four laboratory generated aerosols (ammonium nitrate; oleic acid; oleic acid and ammonium sulfate; and oleic acid, ammonium nitrate, and ammonium sulfate).
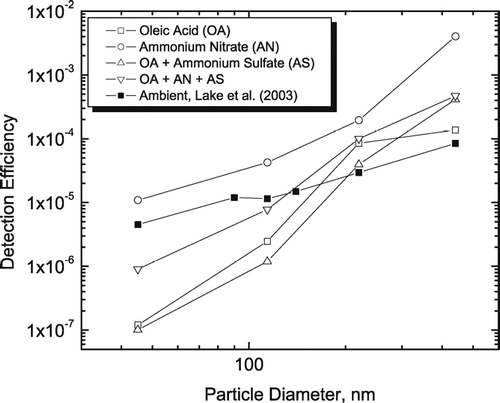
Within the constraint of particle-to-particle variations of the detection efficiency, the direct comparison of RSMS-3 number concentration measurements with those taken by the SMPS requires conversion to mobility diameter:
The mass concentration, (dM/dlog D p ), of the entire aerosol or an individual particle class can be determined from the appropriate RSMS-3 number concentration:
Sulfate PM Event
As discussed above, the ability of RSMS-3 to detect ammonium sulfate is determined by the mixing state of the aerosol. This ability is explored for Event “D,” a three day period in August 2002 in which both sulfate mass and PM2.5 were quite high. shows how number and mass concentrations determined by RSMS-3 compare to standard methodology. compares the total particle number concentrations obtained with RSMS-3 and SMPS. The two measurements are highly correlated (R2 = 0.77) suggesting that the great majority of ambient particles during this event were efficiently detected by RSMS-3. compares the total mass concentrations obtained with RSMS-3 and TEOM during the same time period. While the mass concentrations are of the same order of magnitude, the correlation is poor (R2 = 0.26). A similarly poor correlation is obtained when the TEOM measurements are compared with mass concentrations determined from the SMPS size distribution (data not shown).
FIG. 2 Number and mass concentration measurements during Sulfate PM Event “D.” (A) RSMS-3 vs. SMPS. (B) RSMS-3 vs. TEOM.
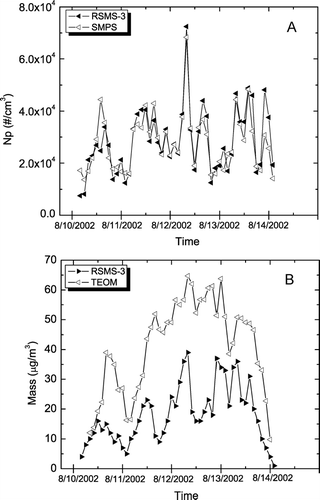
The combination of a high correlation with number concentration and a low correlation with mass concentration suggests that the discrepancy is associated with a small number of large diameter particles that are not detected with RSMS-3 or SMPS. shows the size distributions during episode D at 0700 EST on 13 August. Here, the size bins from the SMPS were co-added to account for the differences in the size distributions of each size bin (CitationPhares et al. 2002). As shown in the figure, the difference may arise from the upper size ranges for particle detection −2.5 μ m for TEOM versus 0.4 μ m for SMPS and ca. 0.6 μ m for RSMS-3. Alternatively, it is clear that the RSMS-3 did not detect some particles between 0.4 and 0.6 μ m dia. that were nominally “pure” ammonium sulfate.
FIG. 3 Number concentrations compared from the SMPS to the RSMS-3 plotted versus mobility diameter (Dm).
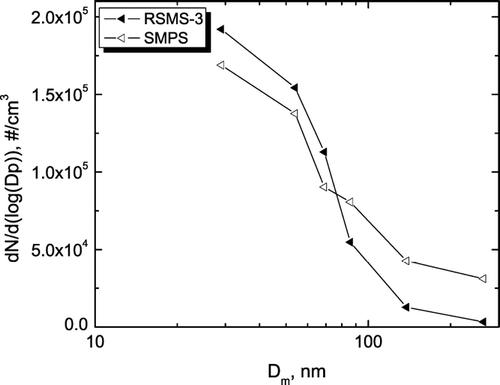
Correlations among mass concentrations from RSMS-3 particle classes and several continuous monitors are shown in . The OCANS class encompasses internally mixed particles containing secondary aerosol components including organic carbon, ammonium sulfate and ammonium nitrate. One of these components, ammonium nitrate is also found in a separate particle class, although the mass concentration of the ammonium nitrate class is almost an order of magnitude smaller than that of the OCANS class during this PM event. compares the mass concentration of the OCANS and nitrate classes to the sum of the corresponding continuous monitors—sulfate, nitrate and organic carbon. Note that the sum of these monitors is obtained by multiplying the organic carbon value by 1.4. While there exists some debate about the absolute magnitude of this multiplier (CitationTurpin et al. 2000), it appears to reconcile the speciation monitors with the TEOM data. The correlation between the RSMS-3 classes and the sum of the three continuous monitors is adequate (R2 = 0.55) and the combined mass of the OCANS and nitrate classes is consistently smaller than the sum of the continuous monitors by a factor of ca. 2.5. The nature of this discrepancy revealed in , , . In , the sulfate mass determined by RSMS-3 and a continuous monitor are compared. The RSMS-3 values were obtained from the total mass of all particles showing indicator ions for sulfate in either the positive or negative ion spectra. If anything, these values would be expected to give an overestimate of the sulfate mass since the particles must contain other chemical species for efficient detection (and indeed other species are observed in the mass spectra). Nonetheless, the sulfate mass determined in this manner grossly underestimates the values obtained from the continuous monitor by a factor of 8 and the correlation is poor (R2 < 0.1). As with the TEOM correlation in , RSMS-3 is missing a significant portion of the mass, because it exists in particles larger than 0.8 μm dia. and/or it exists in nominally “pure” ammonium sulfate particles that are not detected.
FIG. 4 Mass concentrations from RSMS-3 and continuous monitors. (A) RSMS-3: sum of OCANS and nitrate classes; continuous monitors: sum of sulfate, nitrate and organic carbon (×1.4). (B) RSMS-3: sum of particles exhibiting sulfate in negative ion spectra; sulfate monitor. (C) RSMS-3: ammonium nitrate class; nitrate monitor. (D) RSMS-3: carbon class; elemental carbon monitor.
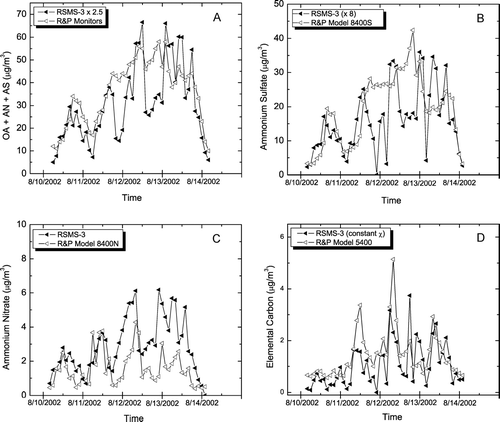
While sulfate detection is clearly a problem, other chemical species are not. compares the nitrate mass determined from the ammonium nitrate particle class detected by RSMS-3 with the continuous monitor. Although nitrate is a minor component of ambient aerosol during this time period, the correlation is good (R2 = 0.65). It should be noted, however, that the nitrate mass determined from the ammonium nitrate class is consistently higher than the nitrate monitor by about a factor of 2. The good correlation indicates that most of the ammonium nitrate in the aerosol is encompassed by the ammonium nitrate class. Although OCANS particles do show signature ions for ammonium nitrate along with other chemical species, the absolute amount of ammonium nitrate in these particles is relatively low. Other components in these particles, primarily organic carbon and ammonium sulfate, are less sensitively detected and therefore are likely to constitute a much larger portion of the particle mass. The overestimate of nitrate mass based on the ammonium nitrate particle class most likely arises from the high detection efficiency of these particles () and the presence of other chemical components in the particles. These other components are less sensitively detected by laser ablation and give much weaker ion signals in the mass spectra. The factor of 2 difference between RSMS-3 and continuous nitrate mass measurements suggests that the detection efficiencies (η in Equation [Equation1]) for the ambient ammonium nitrate particle class are 2 times greater than the composition-averaged ambient particle detection efficiencies in . The magnitude of this difference is consistent with the laboratory particle measurements in .
Finally, the elemental carbon mass is compared in for the continuous monitor and the carbon particle class detected by RSMS-3. Although elemental carbon is a minor component of the ambient aerosol during this time period, the correlation is reasonably high (R2 = 0.6) and the absolute values of the mass agree well. The agreement among the absolute mass values suggests that the detection efficiencies for the carbon particle class are approximately equal to the composition-averaged detection efficiencies in . It should be noted that a constant shape factor, χ, was used to calculate these mass concentrations. When a variable shape factor (CitationSlowik et al. 2004) was used for fresh combustion emissions, the amount of elemental carbon in these particles is was overestimated by a factor of 8 using RSMS-3, clearly a not realistic result.
Several key observations can be drawn from the sulfate PM event. This event represents a worst case situation for RSMS-3 analysis as ammonium sulfate is poorly detected and a considerable amount of the aerosol mass may exist beyond the upper size limit of the instrument. Nonetheless, RSMS-3 provides an accurate measure of time-dependent particle number concentrations. In addition, the time-dependent nitrate and elemental carbon mass concentrations can be determined even though these species represent minor components (ca. 10% each) of the total aerosol mass.
Carbon PM Event #1 (Stagnation During Heavy Traffic)
Event “F” occurred in November 2002 and was characterized by a high carbon mass loading as stagnant air and high traffic contributed to an elevated PM2.5. shows how number and mass concentrations determined by RSMS-3 compare to standard methods during this time period. As with the sulfate event, number concentration measurements obtained with RSMS-3 and SMPS are highly correlated (R2 = 0.68) as shown in . The smaller values obtained with RSMS-3 on the last day of the event are due to the underestimation of the number concentrations using the RSMS-3. During the latter part of the event the SMPS consistently measured number concentrations throughout the size range a factor of 1.5 higher than the RSMS-3. On this day, a significant number of particles were observed with the SMPS below the lower size limit of RSMS-3 (30 nm dia.). The RSMS-3 mass concentration correlates well with the TEOM data, R2 = 0.74 as shown in . In particular, a large spike in mass concentration was recorded by both methods around mid-day on 20 November. Note that the SMPS number concentration data and the TEOM mass concentration data for the event were also associated (R2 = 0.62), indicating that most of the particle mass was found in the submicron size range.
FIG. 5 Number and mass concentration measurements during Carbon PM Event “F” (stagnation). (A) RSMS-3 versus SMPS. (B) RSMS versus TEOM.
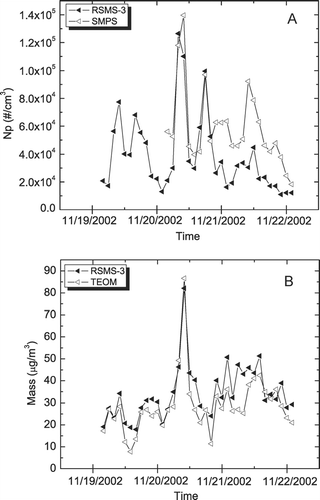
Correlations between RSMS-3 and continuous monitor speciation data are shown in . compares the sum of the organic carbon, ammonium nitrate and ammonium sulfate continuous monitors (as described above for the sulfate event) with the combined mass concentration of the OCANS and ammonium nitrate classes observed with RSMS-3. Here the correlation is quite high (R2 = 0.81) indicating that RSMS-3 efficiently detects most particles containing these components and that the detection efficiencies of the OCANS particles are approximately equal to the composition-averaged detection efficiencies in . As with the sulfate event, and show that the nitrate particle class and carbon particle class mass concentrations correlate well with the nitrate (R2 = 0.75) and elemental carbon (R2 = 0.72) continuous monitors. Unlike for the previous event, no offset of the nitrate concentration is observed here as the majority of the nitrate mass is in the OCANS particle class rather than the ammonium nitrate particle class. Ammonium sulfate determined from RSMS-3 data (procedure described in the sulfate event section) shows a poor correlation with the sulfate monitor (R2 < 0.1), again highlighting the difficulty in extracting sulfate mass concentration data from laser ablation mass spectra. While sulfate speciation is not possible, the high correlations in and show that the contribution of ammonium sulfate to total mass can be accounted for when the sulfate exists in internally mixed particles (i.e., OCANS) that do not exceed the upper size limit of RSMS-3.
FIG. 6 RSMS-3: sum of OCANS and nitrate classes; continuous monitors: sum of sulfate, nitrate, and organic carbon (×1.4). (B) RSMS-3: sum of particles exhibiting sulfate in negative ion spectra; sulfate monitor. (C) RSMS-3: ammonium nitrate class; nitrate monitor. (D) RSMS-3: carbon class; elemental carbon monitor.
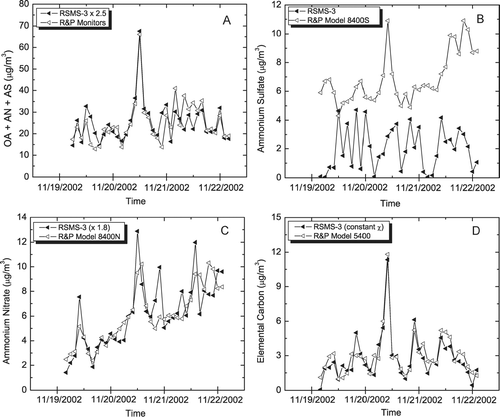
Carbon PM Event #2 (Wildfire)
Event “C” presents a special case because of the unique composition of the aerosol. While OCANS was normally the largest particle class in both number and mass concentration in Baltimore aerosol, during this time period the potassium and potassium-OCANS (K-OCANS) classes were larger owing to the impact of wildfire aerosol. Over the eight-month period, particles containing potassium were responsible for 10% of the total aerosol mass, which corresponded to ca. 1 μ g/m3. During the wildfire PM event, the potassium and K-OCANS classes together exceeded 50 μg/m3 and represented 90% of the total mass. It should be noted that the K-OCANS class was not observed any other time during the eight-month study. This particle class most likely corresponds to wildfire aerosol transformed by the addition of secondary components during long range transport.
shows how the total number and mass concentrations obtained from RSMS-3 compare to standard methods. Both are reasonably well correlated, R2 = 0.71 and 0.65 respectively for number () and mass () concentrations. The RSMS-3 number concentration is about 50% larger than the SMPS number concentration, which most likely reflects higher detection efficiencies of potassium particles relative to the composition-averaged values from that were used in the calculation. It is interesting to note that during this time period, mass concentrations derived from the SMPS measurements did not correlate at all with the TEOM (R2 < 0.1), despite the reasonably high correlation of the RSMS-3 data with both SMPS and TEOM measurements. The better correlation of RSMS-3 with the TEOM is a consequence of the greater size range of RSMS-3 relative to the SMPS coupled with the fact that most of the aerosol mass was associated with particles larger than the upper size limit of the SMPS. Nonetheless, shows that RSMS-3 under-reports the total mass during the peak of the event on 6 July, presumably because much of the aerosol mass exists in particles larger than the upper size limit of RSMS-3. As with the other events, the correlation between the sulfate mass determined by RSMS-3 and the continuous monitor was poor (R2 = 0.36). Interestingly, the carbon class did not track well with the elemental carbon mass (R2 = 0.35), perhaps because such a large amount of elemental carbon resides in the potassium and K-OCANS classes. We suspect that the carbon class, which contains mostly small particles that show only carbon cluster ions in the mass spectra (CitationTolocka et al. 2005), is indicative of local emissions only. No distinct nitrate class was observed during this time period, although nitrate was evident in mixed composition particles.
CONCLUSIONS
For all three PM events, particle number concentrations obtained with RSMS-3 and SMPS correlated well, R2 ∼ 0.7. In contrast, mass concentrations obtained with RSMS-3 and TEOM did not always correlate well. When sulfate was a minor component of the aerosol (Events “C” and “F”), the discrepancy was small and appeared to arise from the smaller upper particle size limit of RSMS-3 (0.8 μm dia.) relative to the TEOM. When sulfate was the major component of the aerosol, RSMS-3 could not be used to obtain the total mass concentration owing to inefficient detection of particles composed primarily of ammonium sulfate in combination with a limited ability to characterize particles larger than 0.8 μ m dia. Nonetheless, even during periods of exceptionally high sulfate mass concentration, the nitrate and carbon particle classes identified by RSMS-3 gave mass concentrations that correlated well with the nitrate and elemental carbon continuous monitors. These correlations were obtained with a simple procedure based on Art2-a particle classification and particle detection efficiencies averaged over the entire ambient aerosol. In some situations, a constant offset between RSMS-3 and the continuous monitors was observed which permitted the relative detection efficiencies of different particle composition classes to be estimated. Based on these offsets, it appears that the detection efficiencies of ammonium nitrate particles are two times larger, potassium-organic particles are 1.5 times larger, OCANS and elemental carbon particles are approximately equal to, and ammonium sulfate particles are much less than the composition-averaged detection efficiencies reported here and previously.
Although the research described in this article has been funded by the United States Environmental Protection Agency through grant number R82806301, it has not been subjected to the Agency's required peer and policy review and therefore does not necessarily reflect the views of the Agency and no official endorsement should be inferred.
REFERENCES
- Allen , J. O. , Fergenson , D. P. , Gard , E. Hughes , L. S. 2000 . Particle Detection Effiicencies of Aerosol Time of Flight Mass Spectrometers under Ambient Sampling Conditions . Environ. Sci. Technol. , 34 ( 1 ) : 211 – 217 . [CSA]
- Bhave , P. V. , Kleeman , M. J. , Allen , J. O. , Hughes , L. S. , Prather , K. A. and Cass , G. R. 2002 . Evaluation of an Air Quality Model for the Size and Composition of Source-Oriented Particle Classes . Environ. Sci. Technol. , 36 : 2154 – 2163 . [INFOTRIEVE] [CROSSREF] [CSA]
- Brundish , K. D. , Miller , M. N. , Wilson , C. W. , Jefferies , M. , Hilton , M. and Johnson , M. P. 2005 . Measurement of Smoke Particle Size and Distribution Within a Gas Turbine Combustor . Trans. ASME , 127 : 286 – 294 . [CSA]
- Carpenter , G. A. , Grossberg , S. and Rosen , D. B. 1991 . ART 2-A—An Adaptive Resonance Algorithm for Rapid Category Learning and Recognition . Neural Networks , 4 : 493 – 504 . [CROSSREF] [CSA]
- DeCarlo , P. F. , Slowik , J. G. , Worsnop , D. R. , Davidovits , P. and Jimenez , J. L. 2004 . Particle Morphology and Density Characterization by Combined Mobility and Aerodynamic Diameter Measurements. Part 1. Theory . Aerosol Sci. Technol. , 38 : 1185 – 1205 . [CROSSREF] [CSA]
- Gross , D. S. , Galli , M. E. , Silva , P. J. and Prather , K. A. 2000 . Relative Sensitivity Factors for Alkali Metal and Ammonium Cations in Single-Particle Aerosol Time-of-Flight Mass Spectra . Anal. Chem. , 72 : 416 – 422 . [INFOTRIEVE] [CROSSREF] [CSA]
- Harrison , D. , Park , S. S. , Ondov , J. M. , Buckley , T. , Kim , S. R. and Jayanty , R. K. M. 2004 . Highly-Time Resolved Particulate Nitrate Measurements at the Baltimore Supersite . Atmos. Environ. , 38 : 5321 – 5332 . [CROSSREF] [CSA]
- Jimenez , J. L. , Jayne , J. T. , Shi , Q. , Kolb , C. E. , Worsnop , D. R. , Yourshaw , I. , Seinfeld , J. H. , Flagan , R. C. , Zhang , X. F. , Smith , K. , Morris , J. W. and Davidovits , P. 2003 . Ambient Aerosol Sampling Using the Aerodyne Aerosol Mass Spectrometer . J. Geophy. Res. , 108 ( D7 ) : 8425 [CROSSREF] [CSA]
- Kane , D. B. and Johnston , M. V. 2000 . Size and Composition Biases on the Detection of Individual Ultrafine Particles by Aerosol Mass Spectrometry . Environ. Sci. Technol. , 34 : 4887 – 4893 . [CROSSREF] [CSA]
- Kane , D. B. and Johnston , M. V. 2001 . Nanoparticle Detection by Aerosol Mass Spectrometry . Aerosol Sci. Technol. , 34 : 520 – 527 . [CSA]
- Kane , D. B. , Wang , J. J. , Frost , K. and Johnston , M. V. 2002 . Detection of Negative Ions from Individual Ultrafine Particles . Anal. Chem. , 74 : 2092 – 2096 . [INFOTRIEVE] [CROSSREF] [CSA]
- Lake , D. L. , Tolocka , M. P. , Wexler , A. S. and Johnston , M. V. 2003 . Mass Spectrometry of Individual Particles Between 50 and 750 nm in Diameter at the Baltimore Supersite . Environ. Sci. Technol. , 37 ( 15 ) : 3268 – 3274 . [INFOTRIEVE] [CROSSREF] [CSA]
- Lake , D. L. , Tolocka , M. P. , Wexler , A. S. and Johnston , M. V. 2004 . The Character of Single Particle Sulfate in Baltimore . Atmos. Environ. , 38 ( 31 ) : 5311 – 5320 . [CROSSREF] [CSA]
- Morawska , L. , Johnson , G. , Ristovski , Z. D. and Agranovski , V. 1999 . Relation Between Particle Mass and Number for Submicrometer Airborne Particles . Atmos. Environ. , 33 : 1983 – 1990 . [CROSSREF] [CSA]
- Morawska , L. , Thomas , S. , Bofinger , N. , Wainwright , D. and Neale , D. 1998 . Comprehensive Characterization of Aerosols in A Subtropical Urban Atmosphere: Particle Size Distribution and Correlation with Gaseous Pollutants . Atmos. Environ. , 32 ( 14/15 ) : 2467 – 2478 . [CROSSREF] [CSA]
- Park , S. S. , Kleissl , J. , Harrison , D. , Nair , N. P. , Kumar , V. and Ondov , J. M. 2005 . Investigation of PM2.5 Episodes Using Semi-Continuous Instruments at the Baltimore Supersite at Ponca St . J. Geophys. Res. , submitted[CSA]
- Phares , D. J. , Rhoads , K. P. , Johnston , M. V. and Wexler , A. S. 2003 . Size-Resolved Ultrafine Particle Composition Analysis Part 2: Houston . J. Geophys. Res. , 108 ( D7 ) : 8420 [CROSSREF] [CSA]
- Phares , D. J. , Rhoads , K. P. and Wexler , A. S. 2002 . Performance of a Single-Ultrafine-Particle Mass Spectrometer . Aerosol Sci. Technol. , 36 ( 5 ) : 583 – 592 . [CROSSREF] [CSA]
- Phares , D. J. , Rhoads , K. P. , Wexler , A. S. , Kane , D. B. and Johnston , M. V. 2001 . Application of the ART-2a Algorithm to Laser Ablation Aerosol Mass Spectrometry of Particle Standards . Anal. Chem. , 73 : 2338 – 2344 . [INFOTRIEVE] [CROSSREF] [CSA]
- Rhoads , K. P. , Phares , D. J. , Wexler , A. S. and Johnston , M. V. 2003 . Size-Resolved Ultrafine Particle Composition Analysis Part 1: Atlanta . J. Geophys. Res. , 108 ( D7 ) : 848 [CROSSREF] [CSA]
- Sapkota , A. , Symons , J. M. , Kleissl , J. , Wang , L. , Parlange , M. B. , Ondov , J. M. and Buckley , T. J. 2005 . Impact of the 2002 Canadian Forest Fires on PM Air Quality in Baltimore City . Environ. Sci. Technol. , 39 ( 1 ) : 24 – 32 . [INFOTRIEVE] [CSA]
- Sioutas , C. , Abt , E. , Wolfson , J. M. and Koutrakis , P. 1999 . Evaluation of the Measurement Performance of the Scanning Mobility Particle Sizer and Aerodynamic Particle Sizer . Aerosol Sci. Technol. , 30 : 84 – 92 . [CROSSREF] [CSA]
- Slowik , J. G. , Stainken , K. , Davidovits , P. , Williams , L. R. , Jayne , J. T. , Kolb , C. E. , Worsnop , D. R. , Rudich , Y. , DeCarlo , P. F. and Jimenez , J. L. 2004 . Particle Morphology and Density Characterization by Combined Mobility and Aerodynamic Diameter Measurements. Part 2: Application to Combustion Generated Soot Aerosols as a Function of Fuel Equivalence Ratio . Aerosol Sci. Technol. , 38 ( 12 ) : 1206 – 1222 . [CROSSREF] [CSA]
- Tolocka , M. P. , Lake , D. L. , Johnston , M. V. and Wexler , A. S. 2005 . Size-Resolved Fine and Ultrafine Particle Composition in Baltimore, MD . J. Geophys. Res. , 110 ( D07S04 ) doi:10.1029/2004JD004573[CSA]
- Tolocka , M. P. , Lake , D. L. , Wexler , A. S. and Johnston , M. V. 2004a . Number Concentrations of Fine and Ultrafine Particles Containing Metals . Atmos. Environ. , 38 : 3263 – 3273 . [CROSSREF] [CSA]
- Tolocka , M. P. , Lake , D. L. , Wexler , A. S. and Johnston , M. V. 2004b . Ultrafine Nitrate Particle Events in Baltimore Observed by Real-Time Single Particle Mass Spectrometry . Atmos. Environ. , 38 : 3215 – 3223 . [CROSSREF] [CSA]
- Turpin , B. J. , Saxena , P. and Andrews , E. 2000 . Measuring and Simulating Particulate Organics in the Atmosphere: Problems and Prospects . Atmos. Environ. , 34 : 2983 – 3013 . [CROSSREF] [CSA]
- Wenzel , R. J. , Liu , D. Y. , Edgerton , E. S. and Prather , K. A. 2003 . Aerosol Time-of-Flight Mass Spectrometry During the Atlanta Supersite Experiment: 2. Scaling Procedures . J. Geophys. Res. , 108 ( D7 ) : 8427 doi:10.1029/2001JD001563[CROSSREF] [CSA]
- Zhang , X. , Smith , K. A. , Worsnop , D. R. , Jimenez , J. L. , Jayne , J. T. , Kolb , C. E. , Morris , J. and Davidovits , P. 2004 . Numerical Characterization of Particle Beam Collimation: Part II Intergrated Aerodynamic Lens Nozzle System . Aerosol Sci. Technol. , 38 : 619 – 638 . [CROSSREF] [CSA]