A new “single box” Scanning Mobility Particle Sizer (TSI SMPS Model 3034) was deployed and operated during a period of four weeks as a part of the PMTACS-NY Winter 2004 intensive study in Queens College, New York City. The SMPS 3034 is an alternative to a conventional multi-component TSI SMPS and houses a Differential Mobility Analyzer and butanol-based Condensation Particle Counter in one cabinet. The SMPS 3034 operates at a fixed 1 l/min sample flow rate (4 l/min sheath flow rate) and measures size distributions within a 10–487 nm size range. One size scan is produced every 3 minutes. Four other measurement systems (a conventional TSI SMPS with a Nano Differential Mobility Analyzer, an Aerodynamic Particle Sizer, a stand-alone Condensation Particle Counter, and an R&P Inc. Filter Dynamic Measurement System (FDMS) TEOM mass monitor) were operated side-by-side with the SMPS 3034. It is shown that total particle number concentrations measured by the SMPS 3034 are highly correlated with those from the conventional Nano SMPS, the Condensation Particle Counter and the FDMS TEOM monitor, and that the number median diameters measured by the SMPS 3034 and the Nano SMPS agree within 3 nm.
INTRODUCTION
Ambient aerosols in the atmosphere play a number of important roles: they affect cloud formation, climate forcing, visibility, are associated with heterogeneous chemistry, and affect air quality and human health (CitationCharlson et al. 1992; CitationSeinfeld and Pandis 1998; CitationSamet 2000). Many epidemiological and toxicological studies have linked atmospheric aerosols, and especially a fraction of particulate matter consisting of particles with aerodynamic diameters below 2.5 μ m (PM2.5), to various adverse health effects (CitationPope et al. 2002; CitationKaiser 2005). Information obtained from monitoring the concentration and size distribution of atmospheric aerosols is essential in estimating the health impact of total PM2.5 and its ultrafine fraction (particles with aerodynamic diameters below 0.1 μ m (CitationGupta et al. 2004)).
The scanning mobility particle sizer (SMPS) is a multi-component instrument, which measures the size distribution of aerosols, thus providing information about the concentration of aerosol particles of various diameters in an analyzed air sample. SMPSs have been used in numerous laboratory and field studies for many years (examples of some recent field experiments where the SMPSs were used can be found in CitationShen et al. (2002), CitationStanier et al. (2004), CitationPark et al. (2004), and CitationZhu et al. (2004)). The flexibility of the SMPS, i.e., the possibility of varying the sheath and the flow rates, of selecting the scan time, and of combining different components of the system (Differential Mobility Analyzers and Condensation Particle Counters), is a feature which makes the instrument attractive to many researchers. However, SMPS preparation for transport, installation and set-up and selection of the operating parameters must be done by trained personnel. Once properly installed, the SMPS can usually operate reliably without daily maintenance. For a variety of studies (e.g., short intensive field campaigns, advanced aerosol research, or designing new instruments) a conventional SMPS is the best option for a sizing instrument. However, for many routine field measurements it can be more practical to use a particle sizer which: (1) combines basic features of the SMPS yet is convenient to transport, deploy and operate in field conditions; (2) operates with a set of fixed parameters thus producing data that are easy to compare with those from similar instruments at various locations; and (3) requires even less maintenance than the conventional SMPS. The newly developed “single box” Scanning Mobility Particle Sizer (SMPS; Model 3034, TSI Inc.) has the potential to function as such an instrument. To our knowledge, this is the first study evaluating the SMPS 3034 under field conditions.
The PM2.5 Technology Assessment and Characterization Study—New York (PMTACS-NY) is one of several U.S. Environmental Protection Agency “Supersites” intended to provide enhanced measurement data on the chemical and physical composition of PM and its associated precursors. One of the program objectives was to “test and evaluate new measurement technologies and provide technological transfer of demonstrated operationally robust technologies for network operation.” The last campaign of the PMTACS-NY was conducted from January 8, 2004 to February 6, 2004 in Queens, New York City. Evaluation of a “single box” SMPS 3034 was done during this intensive field study.
A variety of aerosol sizing instruments were deployed and operated by the Atmospheric Sciences Research Center, U-Albany and the NYS Department of Environmental Conservation alongside the SMPS 3034. Among them were a conventional TSI Model 3936 Scanning Mobility Particle Sizer with a Model 3085 Nano Differential Mobility Analyzer and a Model 3025 Condensation Particle Counter (Nano SMPS), an Aerodynamic Particle Sizer (TSI Model 3321, APS) and a stand-alone Condensation Particle Counter (TSI Model 3022, CPC 3022). A PM2.5 Filter Dynamic Measurement System monitor based on a Tapered Element Oscillating Microbalance mass monitor (R&P Model 8500, FDMS TEOM) was operated at the site as well.
The objective of this article is to present data obtained using the SMPS 3034, to compare the data with those from the Nano SMPS and the FDMS TEOM, to evaluate our experience of operating the SMPS 3034 in field environment and to suggest some improvements for instrument design and operation.
SMPS 3034 DESCRIPTION
The SMPS system measures the number size distribution of ultrafine particles using an electrical mobility separation technique (CitationWang and Flagan 1989). The two main components of the conventional SMPS (TSI Model 3936) are an Electrostatic Classifier (ESC) which includes a Differential Mobility Analyzer (DMA), and a Condensation Particle Counter (CPC) (). In the ESC polydisperse particles are separated according to their electrical mobility. This is achieved by passing a charged aerosol sample through a DMA, which consists of two concentric metal cylinders. While the outer cylinder is grounded, a controlled negative voltage is applied to the inner cylinder. Two laminar flows, one containing a charged sample aerosol and the other containing particle-free sheath air, flow through an annular space between the two cylinders. At each specific voltage, only particles with a specific electrical mobility (which is proportional to the number of charges on the particles but inversely proportional to the particle diameter) are capable of passing through the DMA into a butanol-based CPC where the particles are counted. To obtain a full size distribution of an aerosol, the voltage applied to the inner cylinder of the DMA is exponentially scanned. A NanoDMA is used for sizing smaller size aerosol particles (2.5–150 nm), while a Long DMA can be used for sizing larger particles (10–1000 nm).
FIG. 1 Schematics of a conventional Scanning Mobility Particle Sizer with a Long DMA, TSI SMPS Model 3936 (courtesy of TSI, Inc.). The ESC is located on the left from the CPC and a computer.
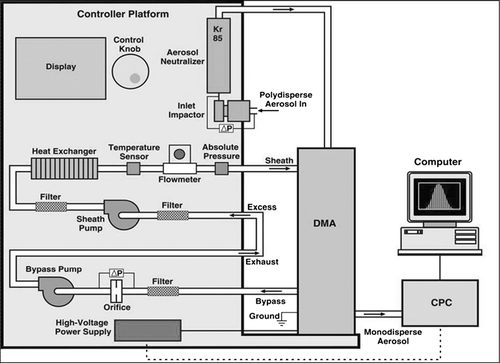
The SMPS 3034 is designed using the same principles as the conventional SMPS (). One of the major differences is that the components of the SMPS 3034 (ESC with a DMA and CPC) are integrated into a single box, which makes instrument transport and set-up much more convenient, especially during field campaigns. Routine maintenance of the SMPS 3034 is easier due to replacement of the impactor at the ESC inlet by a 800-nm mini-cyclone (resulting in less frequent, faster and easier inlet cleanings), and implementation of a patented water-removal technology from the butanol reservoir in the integrated CPC (resulting in much less frequent draining and refilling of butanol). The SMPS 3034 DMA is approximately 1/4 shorter than a conventional Long DMA, thus combining higher sensitivity towards smaller particles and a wide range of diameters in a scan, 10–470 nm (although not as wide as those from the Long DMA). Fewer particle losses and a faster instrument response of the SMPS 3034 are ensured by direct connection of the DMA outlet to the CPC inlet (which leads to shortening of the aerosol flow path), as well as by a higher sample flow rate (1 l/min). In addition, this higher sample flow rate results in good counting statistics even at low particle concentrations. The saturator and condenser temperatures of the CPC in the SMPS 3034 are controlled separately, and the temperature differential is 25°C in contrast to 17°C and 27°C for the CPC models 3010 and 3025, respectively. As a result the SMPS 3034 CPC can detect small particles with a higher efficiency than the CPC 3010. It has a lower efficiency than the CPC 3025 because of the lower temperature difference and different internal design. The sheath-to-sample flow ratio for the SMPS 3034 is fixed at 4:1, while the conventional SMPS is typically operated at the sheath-to-sample flow ratio of 10:1. The lower flow ratio results in a lower size resolution (CitationCollins et al. 2004). Other differences in design and operation between the conventional SMPS and the SMPS 3034 are an automated correction for temperature and pressure leading to more accurate mean free path and viscosity values, and a maximum resolution of 32 channels/decade (SMPS 3034) versus 64 channels/decade (conventional SMPS).
FIG. 2 Schematics of a “single box” Scanning Mobility Particle Sizer, TSI SMPS Model 3034 (courtesy of TSI, Inc.).
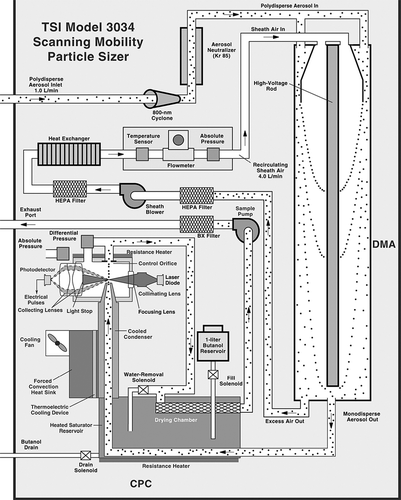
An additional convenience for an operator of the SMPS 3034 is that operational parameters (scan time, sheath and sample flow rates) are fixed (see ). On the other hand, these preset parameters make the SMPS 3034 less flexible and more suitable for routine measurement rather than for research purposes.
TABLE 1 Operation parameters and data completeness for the particle sizing and counting instruments
OPERATION OF PARTICLE SIZING AND COUNTING INSTRUMENTS, DATA COLLECTION, AND PROCESSING
The sampling site was located on the campus of Queens College, Queens, New York City (parking field #6–40°44′ N latitude, 73°49′ W longitude, and approximately 25 m above sea level). There are two busy highways within 1 kilometer of the site: the Long Island Expressway (I-495) and the Van Wyck Expressway (I-678). Particle sizing and counting instruments were housed in two adjacent rooms inside an assembly of several permanent trailers, and sampled outside air from 01/09 to 02/05.
The SMPS 3034 and the CPC 3022 sampled through a flow splitter (TSI Model 3708) connected to a port in the wall of a main inlet tube (stainless steel, 3″ OD, flow speed 1.1 m/sec). Another port of the main inlet tube was used for the APS sampling. Inlet height was approximately 6.5 meters above the ground. The second main inlet tube was identical in design to the first one, with one of its ports used for sampling by the Nano SMPS. Both inlet tubes were located within a few meters of each other. Quality assurance and maintenance procedures included daily operational parameter checks, dynamic filter background checks with a HEPA filter at the inlet (normally once a week, and daily on several occasions), weekly inlet flow audits, cyclone/impactor cleaning (as necessary) and replacing activated charcoal in a scrubber at the CPC exhaust port used to absorb butanol vapors. Size calibrations of the SMPSs were performed prior to sending instruments to the field. Operational parameters for the SMPS 3034, Nano SMPS, APS, and the CPC 3022 are listed in . The SMPS 3034 data were saved every 3 minutes, the Nano SMPS data—every 2.5 minutes, the CPC 3022 and the APS data—every 5 minutes. All instruments sampled almost uninterrupted for four weeks. also reports the percentage of data capture which is calculated as the ratio of valid data points over the maximum possible data points. Invalid points include data missed because of scheduled maintenance or removed during data processing (when it was clear that an instrument was malfunctioning).
The time series of 3-minute number concentrations and number median diameter measured by the SMPS 3034 during the entire study period are shown in . A week-long data gap (01/14–01/20) occurred due to an SMPS 3034 malfunction. Data from this period have been discarded resulting in 75% data capture. The likely reason is that an induced leak in the system resulted in high background (up to 10,000 particles/cm3 at times) signal. It has been noticed that the high background readings were associated with improper installation of a mini-cyclone at the inlet of the SMPS 3034. In contrast, the data capture for the other TSI instruments used in this study ranged from 93 to 100%.
FIG. 3 Time series of 3-minute aerosol number concentrations and number median diameters from SMPS 3034.
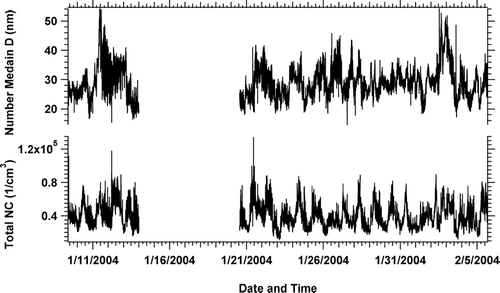
It should be noted that artificially high particle number concentrations (30 particles/cm3 or higher, while the concentration in the adjacent bins is lower than 10 particles/cm3) were periodically recorded in one or two diameter bins by the SMPS 3034. These higher than normal concentrations are random, noticeable only at higher diameters (264–469 nm), rarely present in two consecutive 3-minute samples, and could be caused by presence of 471–800 nm particles carrying a multiple charge. While they have almost no impact on the average number size distributions, their effect on mass size distributions can be quite significant. Therefore these higher mass concentration data were removed from the data set by setting a threshold mass concentration values for each of the last nine size bins (264–469 nm) and running those nine mass concentration data sub-sets first through a 5-point median filter and then through a 3-point median filter. As a result, data points which were higher than a predetermined threshold value were replaced by a median of 5 or 3 consecutive points. Artifacts not removed by the median filters were replaced by those from an adjacent size bin, or from the same size bin but collected 15 minutes before the sample in question.
It should also be noted that when reviewing the original time series of total particle number concentrations measured by the Nano SMPS, occasional unusually high values were observed. These concentrations had values of 800,000 particles/cm3 or higher in a single bin, which exceeded the number concentration in adjacent size bins by factor of 20 or more. The concentration “spikes” are random, not correlated with either the second Nano SMPS, or with the CPC 3022 measurements, and are present in only one or two size bins. Spikes with the same characteristics (randomly occurring in the Nano SMPS data and not correlated to the CPC 3022 measurements) were also observed previously during the summer 2001 PMTACS-NY field intensive, indicating that they are not specific to the winter 2004 data. These spikes were therefore considered artifacts and the corresponding samples were removed from the Nano SMPS data set. The total number of samples containing the artifacts was 28 during the whole campaign (0.2% of all Nano SMPS data). Number concentration data from particle sizing and counting instruments were averaged over 30 minutes and 1 hour for data comparisons and analysis. Averaging intervals that had less than 75% valid values were removed from the data set.
DATA ANALYSIS, RESULTS, AND DISCUSSION
Observed 3-minute total number concentrations as measured by the SMPS 3034 ranged from 1.3 × 104 particles/cm3 to 1.3 × 105 particles/cm3, while the number median diameter ranged from 15 nm to 54 nm (). No extended “clean air” periods, that is, periods with low particle number concentrations, were detected during the campaign. Average diurnal patterns of the total number concentration and the number median diameter are presented in as boxplots with the means, medians, and 5%, 25%, 75%, and 95% percentiles of the data shown for every hour of the day. The number concentration diurnal pattern shows an extended high concentration period from 6 A.M. to noon (with a clear maximum at 7 A.M.–9 A.M.) and a minimum (midnight–2 A.M.), which are consistent with traffic patterns and perhaps residential heating in the urban New York City area. As the particle number concentration starts to increase around 4–5 A.M., the particle median diameter decreases, which can be attributed to fresh particle emissions and, after a sunrise, to new particle formation. As the day progresses (and particles age), the particle diameter increases while the particle concentration stays high. This phenomenon lasts until approximately noon, and may be similarly correlated with traffic patterns.
FIG. 4 Average diurnal patterns for number concentrations (a) and number median diameters (b) as measured by the SMPS 3034. The legends are as follows: boxes—25% percentiles, the medians and the 75% percentiles; whiskers—5% and 95% percentiles, crosses—the means.
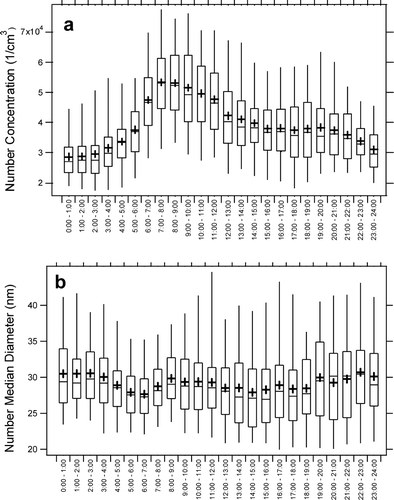
Average number concentration size distributions measured on weekdays (Monday–Friday), weekends (Saturday–Sunday) and Sundays alone are shown in . Presented size distributions were obtained by averaging the 6 A.M.–6 P.M. data from the whole campaign. A clear shift to larger median diameter during weekends (30 nm versus 23 nm during weekdays) is likely due to a decrease of campus activity, resulting in significant reduction in local mobile source emissions. It is consistent with findings of CitationZhu et al. (2002), Citation(2004), who have shown that increased number concentrations of ultrafine particles associated with mobile source emissions are detectable only in a close proximity to the sources (within 300 m or less). A slight (4–5%) decrease in the total particle number concentration on Sundays is consistent with reduction of numbers of cars in the campus parking field #6. High particle number concentrations can be explained by the proximity of the site to major New York City highways, which are busy on weekends as well as on weekdays.
FIG. 5 Average weekday and weekend number size distributions as measure by the SMPS 3034. Only 6 A.M.–6 P.M. data are used for averaging.
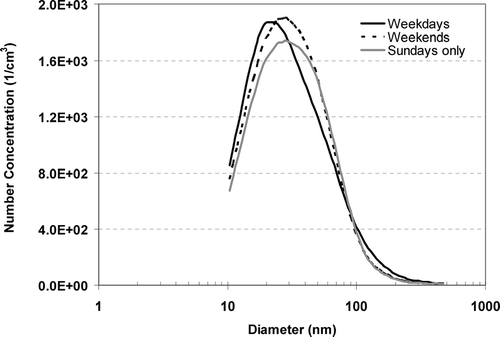
The time series of hourly total particle number concentrations measured by the SMPS 3034, Nano SMPS and the CPC 3022 are shown in . Both SMPSs track the CPC 3022 quite well, however, CPC-measured number concentrations are higher than the total number concentration measured by the SMPS 3034 by approximately a factor of two. Adding the number concentration from the Nano SMPS size bins 7 through 9.7 nm to the total SMPS 3034 number concentration leads only to a small increase in the slope of the correlation scatter plot from 0.47 to 0.49. The discrepancy between the total number concentrations measured by the SMPSs and the CPC 3022 can be largely attributed to particle losses in the inlet and neutralizer of the electrostatic classifiers in the SMPSs, in addition to the failure to adequately measure the smallest particles by the SMPS systems. These losses increase with decreasing particle size and decreasing the sample flow (sample flows were 1 l/min in the SMPS 3034 and 0.6 l/min in the Nano SMPS vs. 1.5 l/min in the CPC 3022).
FIG. 6 The time series of hourly aerosol number concentrations from the SMPS 3034, the SMPS 3936 with the Nano DMA and the CPC 3022.
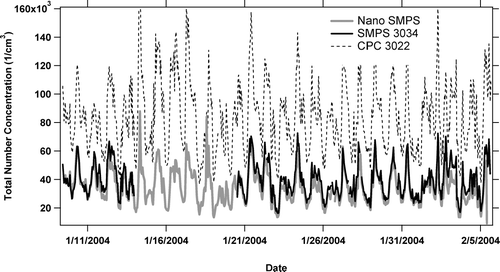
Agreement between the total number concentrations from the SMPS 3034 and Nano SMPS is very good throughout the whole campaign. Aerosol size distributions, as measured by the SMPS 3034 and the Nano SMPS over the week of 01/26/2005–02/01/2005 are shown in . Both instruments agree quite well over the entire overlap size range (10–104 nm). shows the results of the comparison of the 30-minute average particle number median diameter measured by the SMPS 3034 and the Nano SMPS throughout the entire campaign. The slope of the linear regression is 1.01, the intercept is approximately 3 nm and R2 is 0.93. The 30-minute total average number concentrations in the diameter range of 10–104 nm from both instruments were compared as well (). The data are very well correlated (R2 of 0.95). The slope of linear regression is 1.10, and 1.16 if the intercept is set to 0, indicating that the number concentrations agree within 16% in this overlap range. The fact that the SMPS 3034 measures more particles can be attributed to a higher sample flow and shorter particle path inside the SMPS 3034. The reasons for the relatively high intercept of approximately 2.0 × 103 particles/cm3 are not clear.
FIG. 7 Weekly average (01/26/04–02/01/04) number size distributions as measured by the SMPS 3034 and the SMPS with Nano DMA.
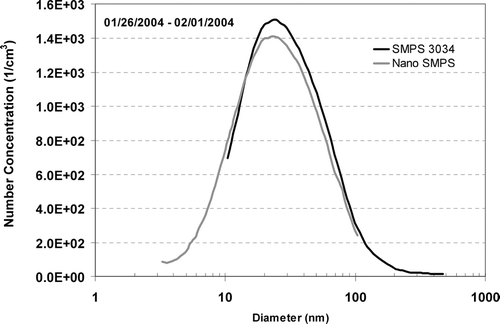
FIG. 8 SMPS 3034 number median diameter data (30-minute averages) plotted versus data from the SMPS 3936 with the Nano DMA.
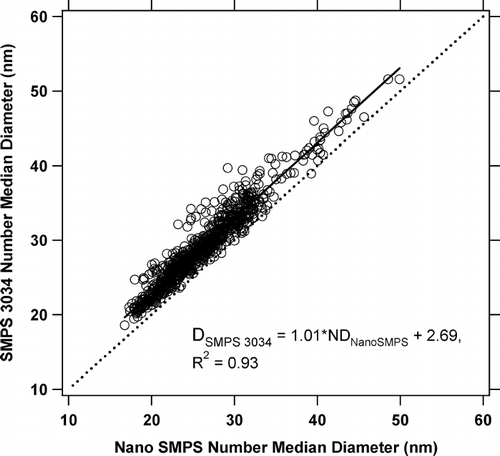
FIG. 9 SMPS 3034 number concentration data (30-minute averages) plotted versus data from the SMPS 3936 with the Nano DMA. Only number concentrations from common size bins (10–104 nm) are considered in this comparison.
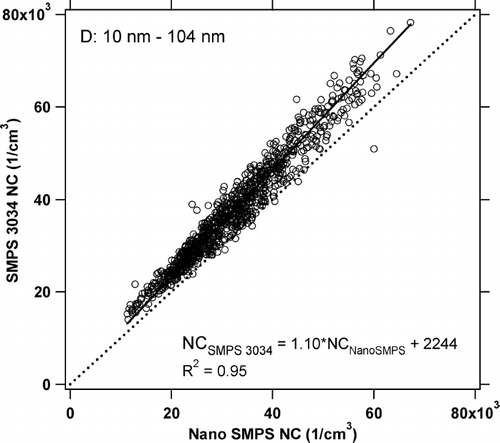
The time series of hourly total particle mass concentrations measured by the SMPS 3034, as well as those measured by an APS and an FDMS TEOM monitor are shown in . Since there are no available data on density of aerosol particles in the New York City area, we have used the value of 1.5 g/cm3 to convert SMPS 3034–measured number concentration into mass concentration. This number is consistent with the lower value of density of urban atmospheric aerosol particles measured in Atlanta, GA (CitationMcMurry 2002) and with the average density value inferred from measurements in Pittsburg, PA (CitationKhlystov et al. 2004). An FDMS TEOM monitor was chosen for comparison with the SMPS 3034 in order to evaluate the ability of the latter to efficiently detect changes in the aerosol mass concentration.
FIG. 10 The time series of hourly aerosol mass concentrations from the SMPS 3034, the APS and the FDMS TEOM. FDMS TEOM size cut-point is 2.5 μ m. While the total mass concentration from the SMPS 3034 is shown, the APS data include only mass concentrations corresponding to the particle diameters of 480 nm to 2.0 μ m (corresponds to aerodynamic diameter of 2.5 μ m). Particle density (SMPS3034 and APS) was assumed to be 1.5 g/cm3.
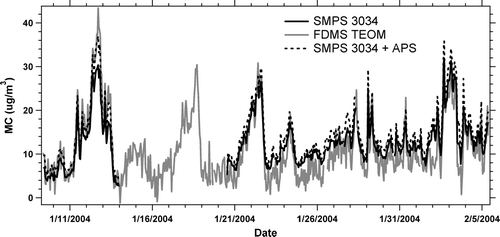
The FDMS TEOM monitor is a self-referencing continuous mass-measuring instrument, accounting for both non-volatile and semi-volatile components of PM mass (more detailed description of the instrument can be found in CitationSchwab et al. (2006)). During the winter field campaign a 2.5 μ m cyclone was used at the FDMS TEOM monitor inlet and the TEOM filter temperature was set to 30°C. The time series of hourly averaged mass concentrations as measured by the FDMS TEOM monitor is also shown in . While the SMPS 3034 (black line) and the TEOM (gray line) data are well correlated (R2 of 0.80), the SMPS 3034 under-estimates the aerosol mass concentration especially during the first part of the campaign. This is not surprising considering that the largest particles the SMPS 3034 is capable of measuring are those with a diameter of 487 nm. In an attempt to close the mass balance, we have used APS data. The APS is a time-of-flight spectrometer that measures the velocity of particles in an accelerating airflow, and thus their aerodynamic diameters. The physical diameter of a spherical particle can be calculated by dividing its aerodynamic diameter by the square root of the particle density (CitationSeinfeld and Pandis 1998). Thus, the mass concentration of particles with aerodynamic diameters of 580 nm to 2.5 μ m (which correspond to physical diameters of 480 nm to 2.0 μ m, when using the assumed particle density of 1.5 g/cm3) was calculated and added to the total SMPS 3034-measured mass concentration (, dashed line). Overall, this reconstructed “SMPS+APS” mass concentration stays well correlated with the FDMS TEOM data (R2 = 0.82) throughout the campaign. Inspection of shows that the agreement between the FDMS TEOM monitor and the “SMPS+APS” is much better for the first part of the campaign. During much of the last part of the campaign there is a roughly 50% discrepancy between the two, with the “SMPS 3034 + APS” mass concentrations being higher. Throughout the campaign there is better agreement at higher mass concentrations (15 μ g/m3 and higher, as measured by the FDMS TEOM monitor). We have assumed a single value for particle density throughout the study, which may explain part of the discrepancy. Examination of the chemical composition and comparison of size distributions of different chemical species as measured by an Aerosol Mass Spectrometer (Aerodyne Research, Inc.) may help to resolve this problem (CitationWeimer et al. 2006).
SUMMARY
A new “single-box” SMPS (TSI Model 3034) was successfully deployed and operated during the PMTACS-NY Winter 2004 intensive campaign in Queens. The SMPS 3034 operated continuously from 01/09 to 02/05. Approximately 25% of the data had to be discarded due to a malfunction of the instrument (possibly a cyclone-induced leak) at the beginning of the campaign. Since this malfunction can be identified by high background number concentrations readings, it should be noted that it is essential to perform a dynamic filter blank test after each time a mini-cyclone was removed for cleaning. No other problems in instrument operation or maintenance were encountered during the study.
Total particle number concentrations measured by the SMPS 3034 are highly correlated with those from the Nano SMPS and the CPC 3022. Inferred mass concentrations from the SMPS 3034 are well correlated with mass concentrations from the FDMS TEOM mass monitor. The SMPS 3034 and Nano SMPS number concentrations agree within 16% in the overlap diameter range (10–104 nm) and the number median diameters agree within 3 nm. It appears that the SMPS 3034 captures the main features of a size distribution correctly and is also as sensitive to small particles (diameters 10–104 nm) as the Nano SMPS. It was also shown that the SMPS 3034 mass concentration measurements track those of the FDMS TEOM reasonably well (R2 = 0.82), albeit using an assumed particle density.
The SMPS 3034 can be used as an easy-to-operate and easy-to-transport alternative to the SMPS 3936, especially for long-term field measurements. In certain cases of ultrafine particle measurements, the SMPS 3034 can serve as a replacement for the combination of the Nano SMPS and the SMPS with the Long DMA, since it can measure particles in the diameter range of 10–487 nm. Although a compact design of the instrument makes moving and set up relatively easy, it can also make it harder to perform repairs in field conditions. Since operation parameters are fixed, it is much easier to start and run the instrument, as well as compare the data from several SMPS 3034 instruments. On the other hand, fixed flows (and consequently the diameter range over which the number concentrations are measured) and scan time may limit the use of the SMPS 3034 for research purposes. Perhaps adding an option to vary the flow, as well as the scan time, may broaden the area of this instrument's use.
This work was supported in part by the New York State Energy Research and Development Authority (NYSERDA), contract # 4918ERTERES99, the U.S. Environmental Protection Agency (EPA) cooperative agreement # R828060010 and New York State Department of Environmental Conservation (NYS DEC), contract # C004210. Although the research described in this article has been funded in part by the U.S. Environmental Protection Agency, it has not been subjected to the Agency's required peer and policy review and therefore does not necessary reflect the views of the Agency and no official endorsement should be inferred.
The authors would like to thank TSI Incorporated for lending us an SMPS 3034 unit for the campaign, Wei Liu, Maynard Havlicek and Steven W. Kerrigan (TSI Inc.) for their advice and helpful discussions, as well as Dirk Felton and Aaron Pulaski (NYS DEC). The authors would also like to acknowledge Queens College for cooperation and logistical support during the campaign.
Notes
* A dryer was installed in the sheath flow.
**Electrical mobility diameter, corresponds to physical diameter for spherical shape particles.
*** Aerodynamic diameter, corresponds to physical diameters of approximately 443–16,167 nm, assuming density of 1.5 g/cm3.
**** 25% of data had to be discarded due to a possible cyclone-induced leak.
REFERENCES
- Charlson , R. J. , Schwartz , S. E. , Hales , J. M. , Cess , R. D. , Coakley , J. A. Jr. , Hansen , J. E. and Hofmann , D. J. 1992 . Climate Forcing by Antropogenic Aerosols . Science , 255 : 423 – 430 . [CSA]
- Collins , D. R. , Cocker , D. R. , Flagan , R. C. and Seinfeld , J. H. 2004 . The Scanning DMA Transfer Function . Aerosol Sci. Technol. , 38 : 833 – 850 . [CROSSREF] [CSA]
- Finlayson-Pitts , B. J. and Pitts , J. N. 1986 . Atmospheric Chemistry: Fundamentals and Experimental Techniques , New York : John Wiley and Sons, Inc. .
- Gupta , T. , Demokritou , P. and Koutrakis , P. 2004 . Effects of Physicochemical Properties of Ultrafine Particles on the Performance of an Ultrafine Particle Concentrator . Aerosol Sci. Technol. , 38 ( S2 ) : 37 – 45 . [CROSSREF] [CSA]
- Kaiser , J. 2005 . Mounting Evidence Indicts Fine-Particle Pollution . Science , 307 : 1858 – 1861 . [CSA]
- Khlystov , A. , Stanier , C. and Pandis , S. 2004 . An Algorithm for Combining Electrical Mobility and Aerodynamic Size Distributions Data When Measuring Ambient Aerosol . Aerosol Sci. Technol. , 38 ( S1 ) : 229 – 238 . [CROSSREF] [CSA]
- McMurry , P. H. , Wang , X. , Park , K. and Ehara , K. 2002 . The Relationship between Mass and Mobility for Atmospheric Particles: A New Technique for Measuring Particle Density . Aerosol Sci. Technol. , 36 : 227 – 238 . [CROSSREF] [CSA]
- Park , S. , Sakurai , H. , Vollmers , K. and McMurry . 2004 . Aersol Size Distributions Measured at the South Pole during ISCAT . Atmos. Environ , 38 : 5493 – 5500 . [CROSSREF] [CSA]
- Pope , C. A. , Burnett , R. T. , Thun , M. J. , Calle , E. E. , Krewski , D. , Ito , K. and Thurston , G. D. 2002 . Lung Cancer, Cardiopulmonary Mortality and Long-Term Exposure to Fine Particulate Air Pollution . JAMA , 287 : 1132 – 1141 . [INFOTRIEVE] [CROSSREF] [CSA]
- Samet , J. M. , Dominici , F. , Curriero , F. C. , Coursac , I. and Zeger , S. L. 2000 . Fine Particulate Air Pollution and Mortality in 20 U.S. Cities . N. Engl. J. Med. , 343 : 1742 – 1749 . [INFOTRIEVE] [CROSSREF] [CSA]
- Schwab , J. J. , Felton , H. D. , Rattigan , O. V. and Demerjian , K. L. 2006 . New York State Urban and Rural Measurements of Continuous PM2.5 Mass by FDMS TEOM and BAM: Evaluations and Comparisons with the FRM . JAWMA , 56 : 372 – 383 . [CSA]
- Seinfeld , J. H. and Pandis , S. N. 1998 . Atmospheric Chemistry and Physics , New York : John Wiley and Sons, Inc. .
- Shen , S. , Jaques , P. A. , Zhu , Y. , Geller , M. D. and Sioutas , C. 2002 . Evaluation of the SMPS—APS System as a Continuous Monitor for Measuring PM2.5, PM10 and Coarse (PM2.5 − 10) Concentrations . Atmos. Environ. , 36 : 3939 – 3950 . [CROSSREF] [CSA]
- Stanier , C. , Khlystov , A. Y. , Chan , W. R. , Mandiro , M. and Pandis , S. 2004 . A Method for the In Situ Measurement of Fine Aerosol Water Content of Ambient Aerosols: The Dry-Ambient Aerosol Size Spectrometer (DAASS) . Aerosol Sci. Technol. , 38 ( S1 ) : 215 – 228 . [CROSSREF] [CSA]
- Wang , S. C. and Flagan , R. C. 1989 . Scanning Electrical Mobility Spectrometer . J. Aerosol. Sci. , 20 : 1485 – 1489 . [CROSSREF] [CSA]
- Weimer , S. , Drewnick , F. , Hogrefe , O. , Schwab , J. J. , Rhoads , Orsini , Canagaratna , M. , Worsnop , D. R. and Demerjian , K. L. 2006 . Size-Selective Non-Refractory Ambient Aerosol Measurements During the PMTACS-NY 2004 Winter Intensive in New York City . J. Geophys. Res. [Atmospheres] , in press[CSA]
- Zhu , Y. , Hinds , W. C. , Kim , S. and Sioutas , C. 2002 . Concentration and Size Distribution of Ultrafine particles Near a Major Highway . JAMA , 52 : 1032 – 1042 . [CSA]
- Zhu , Y. , Hinds , W. C. , Shen , S. and Sioutas , C. 2004 . Seasonal trends of Concentration and Size Distribution of Ultrafine Particles Near Major Highways in Los Angeles . Aerosol Sci. Technol. , 38 ( S1 ) : 5 – 13 . [CROSSREF] [CSA]