This study proposes a method of processing cascade impactor data, called the “calibration matrix method,” and compares it with usual methods. This method takes into account the real collection efficiency curves obtained from cascade impactor calibration, in contrast to the usual methods which assume that cascade impactor collection efficiency is ideal. The comparison between the new and usual methods was carried out for sodium fluoride aerosols generated with five different nebulizers (Multisonic®, LS260 kit2601®, Sidestream®, PariLC+® and Aeroneb Go®) and one dry powder (terbutaline, Astra Zeneca, France), sized with the 8-stage Marple Model 298 Personal cascade impactor. Particle size distributions were represented as differential and cumulative distributions. Statistical differences were highlighted between the calibration matrix method and the usual methods in terms of MMAD and respirable fractions for all aerosols. The largest differences were 28% in terms of MMAD and 64% in terms of respirable fraction.
INTRODUCTION
The ability to characterize aerosol size distributions is of fundamental importance for aerosol-therapy, as the aerosol deposition site in the respiratory tract depends heavily on particle size distribution.
Cascade impactors are the most widely used means for determining in vitro the particle size distribution of aerosols generated from medical inhalers and nebulizers. They measure aerodynamic particle size directly, this being the most relevant parameter for predicting particle transport within the respiratory tract. At the same time, they provide the only way of quantifying the mass of active pharmaceutical agents as well as other non-physiologically active components of the formulation in different size ranges (CitationMitchell and Nagel 2003). Cascade impactors are potentially efficient size-separators in the range of size of aerosol droplets produced by nebulization and are therefore well suited for characterizing these aerosols.
For all these reasons, and even though it is generally agreed that the cascade impactor measurement process is complex and labor intensive, its use is recommended by the European Standard for nebulizers (EN 13544-1, Appendix CC.3, 2001) and by the United States Pharmacopoeia for MDIs and DPIs (CitationUSP 2005), as well as the European Pharmacopeia (European Pharmacopeia, chapter 2.9.18, 2002).
One key issue with cascade impactors is how to process the experimental data once measurements have been taken. Several ways have been described in the literature, resulting paradoxically in different particle size distribution for the same aerosol (CitationDunbar and Hickey 2000; CitationSmith and Jordan 1964) and therefore in different deposition site predictions.
This study describes a method inspired from the work of CitationPicknett (1972), hereafter called “calibration matrix method,” which has been compared with “usual methods.” In contrast to the usual methods, the calibration matrix method is not based on the hypothesis that cascade impactor collection efficiency is ideal, but takes into account the real collection efficiency curves obtained from cascade impactor calibration. The study compares the calibration matrix method and the usual methods applied to aerosols generated with five different nebulizers (Multisonic®, LS260 kit2601®, Sidestream®, PariLC+®, and Aeroneb Go® nebulizers) and one dry powder (terbutaline, Astra Zeneca, France), sized with the 8-stage Marple Model 298 Personal cascade impactor (Thermo Andersen Inc., Smyrna, Georgia, USA).
MATERIAL AND METHOD
Experimental Set-Up
Nebulizers
Two ultrasonic nebulizers (Multisonic® nebulizer (Schill GmbH & Co. KG, Germany) and LS260 kit2601® nebulizer (SYST'AM, France)), two jet nebulizers (Sidestream® nebulizer with Freeway® compressor (Medic-Aid, UK) and PariLC+® nebulizer with Turbo Boy N® compressor (Pari, Germany)) and one vibrating mesh nebulizer (Aeroneb Go® nebulizer (Aerogen, USA)) were used to nebulize 2.5%w/v sodium fluoride (NaF) in water. The resulting aerosol droplets were sized using the 8-stage Marple Model 298 Personal cascade impactor operating at 2 L/min. Absorbent glass fiber filter substrates (Binderless AE Fiber Glass 34 mm, Omega Specialty Instrument Co., USA) were deposited on each plate of the impactor. The Marple Model 298 Personal cascade impactor is proposed in the European Standard (EN 13544-1, Appendix CC.3, 2001) as the impactor of choice for the size characterization of aerosols. The use of absorbent glass fiber filter substrates increases its capacity to collect droplets without overloading (CitationMitchell and Nagel 2003).
The methodology corresponded to that given in the European Standard (EN 13544-1, Appendix CC.3, 2001). The cascade impactor was connected to the nebulizer mouthpiece by a T-piece. Excess aerosol was filtered by an absolute filter (breathing filter, BB50TE, Pall, UK) connected to a 13 L/min vacuum pump (). The 2 L/min pump (cascade impactor pump) and 13 L/min pump (vacuum pump) were simultaneously turned on, then the compressor was immediately turned on to start nebulization. After 5 minutes of nebulization, the pumps and the compressor were stopped and the cascade impactor was dismantled. Each impactor stage filter was then placed in a tube (T420, Simport, Canada) containing 10 ml of a solution made up of 1L of 25% TISAB solution in water mixed with 1ml of 1%w/v sodium fluoride (NaF) in water. Time was allowed for the NaF solution deposited on the filter to migrate entirely into the TISAB solution. For each tube, the concentration of NaF was measured with a fluoride electrode. This experiment was carried out six times for each nebulizer (n = 6).
Dry Powder Inhaler (DPI)
Aerosol generated by a dry powder inhaler (Bricanyl® Turbuhaler®, AstraZeneca, France) was also sized with the 8-stage Marple Model 298 Personal cascade impactor operating at 2 L/min. The experimental set-up differed slightly from the one used for nebulizers. In particular, the glass fiber filters were replaced by substrates coated with silicone oil, which is better adapted for dry aerosol sizing as it avoids particle bounce. The vacuum pump was regulated at 60 L/min to generate the dry powder (). After the pumps were turned on, the inhaler was placed carefully so that it was air-tight with the T-piece, and 20 puffs were generated. At the end of the experiment, the substrates were placed in a tube (T420, Simport, Canada) and 4 ml of NaOH 0.1 M were added. The mass of active compound (terbutaline) was measured by UV Spectrophotometry at 243 nm, blank being made from a substrate coated with silicone oil + 4 ml of NaOH 0.1 M.
Cascade Impactor Collection Efficiency
The collection efficiency is defined as the fraction of particles of a specific size entering an impactor stage which are collected on the impaction plate. The collection efficiency (Ei,j) of each stage (j) for a specific particle size (in the study, monodisperse aerosol of diameter Dcali) is defined as (CitationMitchell et al. 1988):
Ideal Collection Efficiency
In the model of an ideal impactor, there would be complete separation into two classes of particles at each stage, and the particle collection efficiency (E), expressed as a percentage, would increase stepwise between limits of zero to 100% ().
The size at which separation takes place is the cut-off diameter of the corresponding stage Dcj. Particles with diameters higher or equal to Dcj are assumed to be fully collected, whereas all particles finer than Dcj are assumed to pass through the stage with the air stream (CitationMitchell and Nagel 2003).
Real Collection Efficiency
In practice, separation never appears that dramatically. In the case of a well-designed stage, E is a monotonic sigmoidal function of particle aerodynamic diameter that increases steeply from E of ≈ 0% to > 95%, reaching maximum steepness when E is about 50% (). This means that the stage corresponding to the cut-off diameter Dcj does not capture all particles with diameters greater or equal to Dcj but lets some pass to the following stage, and also collects some smaller ones.
In the case of solid particles, this imperfect collection is mainly due to the boundary layer effect on the air flow profile (CitationMarple 1970). CitationRader and Marple (1985) also investigated the importance of gravitational sedimentation, in particular ultra-Stokesian behavior in stage collection efficiency characteristics, and CitationRubow et al. (1987) showed that particle retention occurred on the walls and the stage metalwork of the impactor.
The calibration process involves determining the collection efficiency vs. particle size curve for each stage, in order to establish the cut-off diameter defined by the particle size collected with 50% efficiency (E = 50%) (). This process is accomplished using a large number of monodisperse particles of different sizes in the appropriate range (CitationMitchell and Nagel 2003). Thus the collection efficiency of every stage can be calculated for each monodisperse aerosol and plotted as a function of aerodynamic diameter.
Usual Methods for Analyzing Cascade Impactor Measurements
The mass fraction of NaF (Fj), defined as the mass of NaF collected on stage Dcj divided by the total deposited mass in the impactor, was calculated. The usual methods assume that impactor collection efficiency is ideal, so that particles deposited on this stage are considered to have an aerodynamic diameter (AED) between Dcj and Dcj− 1. The mass fraction of NaF deposited on each stage can be represented in the form of a column matrix (Matrix A):
The particle size distribution was then graphically represented (X-axis = aerodynamic diameter (AED) Dcj; Y-axis = mass fraction Fj).
It can be represented as either a differential or a cumulative mass-weighted size distribution (CitationDunbar and Mitchell 2005) ().
TABLE 1 Calculation of differential and cumulative mass distributions from inertial impaction data for the usual methods
The differential distribution can be represented in three possible ways:
• | A normalized histogram of the differential distribution: levels are equal to p((Dcj−1+Dcj)/2) from Dcj− 1to Dcj. | ||||
• | A normalized differential distribution linking the points (Dcj−1+Dcj)/2 (Middle of cut-off diameters), p((Dcj−1+Dcj)/2) (CitationDunbar and Hickey 2000). | ||||
• | The size distribution presented as fitting with a log-normal function. The log-normal distribution model depends on mass median aerodynamic diameter (MMAD) and geometric standard deviation (σg) (CitationSmith and Jordan 1964; CitationDunbar and Hickey 2000; CitationThiel 2002; CitationO'Shaughnessy and Raabe 2003); the normalized log-normal distribution p(dp) is defined as: |
The cumulative distribution can be represented in one of the following ways:
• | The European Standard (EN 13544-1, Appendix CC.3, 2001) recommends plotting the cumulative distribution of aerosol whose particle mass is < Dcj (undersize cumulative mass). | ||||
• | The cumulative log-normal distribution model which depends on mass median aerodynamic diameter (MMAD) and geometric standard deviation values (CitationSmith and Jordan 1964; CitationDunbar and Hickey 2000; CitationThiel 2002; CitationO'Shaughnessy and Raabe 2003). |
The calculation of differential and cumulative mass distributions from inertial impaction data for the usual methods is summarized in .
Calibration Matrix Method for Analyzing Cascade Impactor Measurements
Collection efficiency data were taken from the study of CitationRubow et al. (1987), which used monodisperse aerosols ranging from 0.48 μ m to 23 μ m in diameter. From these collection efficiency values, it is possible to calculate corresponding mass fractions of monodisperse aerosols of diameter Dcali deposited on impactor stage Dcj, symbolized by fi,j, (detailed calculation in Appendix 1). Matrix B represents the mass fraction of monodisperse aerosols deposited on the stages.
Mass distribution of monodisperse aerosols for calibration (Matrix B)
The calibration matrix (Matrix B) calculated and used in this study, corresponding to the Marple Model 298 Personal cascade impactor, is given in Appendix 2.
To obtain the corrected distribution of aerosol, taking into account imperfect stage collection efficiency, the particle size distribution of each stage (Matrix B) is multiplied by the mass fraction deposited on each stage (Matrix A), to give Matrix C:
A best fit curve linking the points (X-axis = Monodisperse aerosol diameters Dcali; Y-axis = corrected distribution Mi = ∑limits j = 1 n f i,j*Fj) is then plotted:
As in the usual methods, it can be represented as a differential or a cumulative distribution. The calculation of differential and cumulative mass distributions from impactor calibration data for the calibration matrix method is summarized in and .
TABLE 2 Calculation of differential and cumulative mass distributions from impactor calibration data for the calibration matrix method
To compare the distribution curves of the different methods, the differential distribution obtained with the calibration matrix method must be normalized, which implies that the area under the curve equals 1. Thus, the corrected distribution (Matrix C) has to be corrected again so that the area under it equals 1. To do this, the area under the curve between Dcali and Dcali+ 1 is calculated as the area of a trapezoid (bases are Mi and Mi+ 1, height is (Dcali+ 1− Dcali), e.g., ). The total area under the curve is calculated by summing all the trapezoid areas for every Dcali. Each trapezoid area is then divided by the total area under the curve to be normalized, their sum equaling 1. Finally, corresponding values of new Mi (named Mi′) are calculated from expressions of normalized trapezoid areas. Mi′ is calculated from Mi− 1′, so the first M1′ value must be known in order to calculate all the following Mi′ values (). It was hypothesized that no particle would have a diameter equal to or less than 0.1 μ m (origin of the logarithmic scale used), so M1′, corresponding to Dcal1 = 0.1 μ m, was fixed at 0. This explains the first line of the calibration matrix corresponding to Dcal1 = 0.1 μ m (Appendix 2). The last stage of the impactor was made of an absolute filter efficient for diameters equal to or more than 0.1 μ m (CitationEN 1822-1, 1998), which justifies this assumption.
Statistical Analysis of the Aerosol Particle Size Distributions
Aerosol particle size distributions were expressed in terms of two metrics: Mass Median Aerodynamic Diameter (MMAD) and respirable fraction, i.e., percentage of particles with diameters between 1 μ m and 5 μ m (CitationMorrow 1974). These two parameters were expressed for each aerosol generator and each processing method as the mean for the six experiments ± standard deviation.
“Permutation tests for two related samples” were applied to the results to highlight the potential significant differences between values of MMAD and respirable fraction obtained with the calibration matrix method and with the European Standard method, and between values of MMAD and respirable fraction obtained with the calibration matrix method and with the log-normal model. These statistical tests were performed using StatXact software (version 3.0.2, Cytel Software Corporation). A “p” value < 0.05 was considered as a significant difference.
RESULTS
(a to f) represents the differential mass distributions for Multisonic®, Sidestream®, Aeroneb Go®, PariLC+®, LS260 kit2601® nebulizers, and Turbuhaler® respectively, obtained by applying the different data processing methods: Histogram (straight continuous line), “Middle of cut-off diameters” method (open square), log-normal model (curved continuous line), and calibration matrix method (open circle).
FIG. 4 Differential mass distributions represented by histogram (straight line), “middle of cut-off diameters” method (−· ⊟– ·−), log-normal model (curved line) and calibration matrix method (
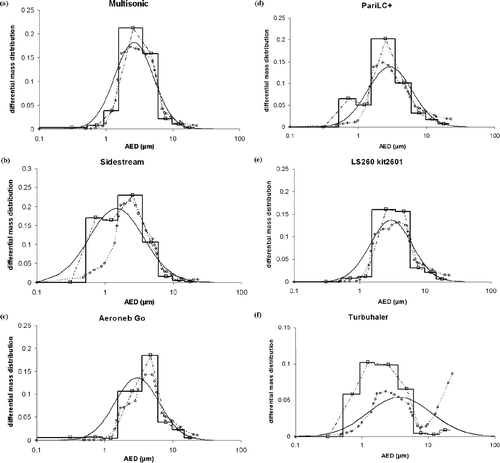
(a to b) represents the cumulative mass distributions for Sidestream® and Turbuhaler®, respectively, obtained by applying the different data processing methods: European Standard (open triangle), log-normal model (black continuous line), and calibration matrix method (open circle).
FIG. 5 Cumulative mass distributions represented by the European Standard method (−·Δ−·), log-normal model (—–), and calibration matrix method (
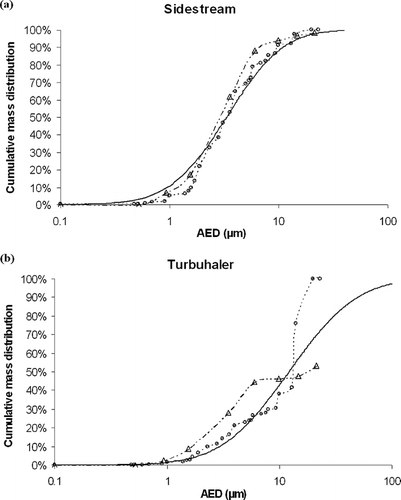
and summarize means ± standard deviations of MMAD and respirable fractions based on the six replicate experiments for the different methods (European Standard method, log-normal model and calibration matrix method) and the different nebulizers (Multisonic®, Sidestream®, Aeroneb Go®, PariLC+®, LS260 kit2601®), as well as Turbuhaler®.
TABLE 3 MMAD for the different methods (European Standard method, log-normal model and calibration matrix method) and the different aerosol generators (Multisonic®, Sidestream®, Aeroneb Go®, PariLC+®, LS260 kit2601®, Turbuhaler®), expressed as mean ± standard deviationFootnote 1 ,Footnote 2
TABLE 4 Respirable fraction (percentage of particles with diameters between 1 μ m and 5 μ m) for the different methods (European Standard method, log-normal model and calibration matrix method) and the different aerosol generators (Multisonic®, Sidestream®, Aeroneb Go®, PariLC+®, LS260 kit2601®, Turbuhaler®), expressed as mean ± standard deviationFootnote 1 ,Footnote 2
MMADs were significantly different between the calibration matrix method and the log-normal model for Multisonic® nebulizer and Turbuhaler®, and between the calibration matrix and European Standard methods for Aeroneb Go® nebulizer, PariLC+® nebulizer, and LS260 kit2601® nebulizer (p < 0.05). Regarding Sidestream® nebulizer, a p value of 0.06 was obtained when comparing the calibration matrix and European Standard methods, which indicates a strong tendency for these two methods to be different.
Respirable fractions were significantly different between the calibration matrix method and both the European Standard method and the log-normal model for Multisonic® nebulizer (p < 0.05). They were significantly different between the calibration matrix and European Standard methods for Sidestream® nebulizer, Aeroneb Go® nebulizer, PariLC+® nebulizer, LS260 kit2601® nebulizer, and Turbuhaler® (p < 0.05).
DISCUSSION
This work focused on how cascade impactor data processing can influence aerosol particle size distribution obtained from nebulizers and DPIs, and therefore predictions of aerosol deposition in patients' airways. Commonly used methods of processing cascade impactor data were compared with each other and with a proposed calibration matrix method specifically developed in this study.
Among the usual methods, the log-normal model is often used to approximate aerosol particle size distribution as it is based on determining only two parameters (MMAD and geometric standard deviation) and is therefore rapid to use (CitationSmith and Jordan 1964; CitationDunbar and Hickey 2000; CitationThiel 2002; CitationO'Shaughnessy and Raabe 2003). However, it is based on the assumption that the particles are log-normally distributed and uni-modal, which is not always true. Moreover, in certain circumstances it may be necessary to use software designed to fit the distribution on a log-normal scale by linear or non-linear regression (e.g., SigmaPlot for Windows software). Regarding the differential distributions, the log-normal model gives a biased view of the scale and can mislead the interpretation. In particular there is a gap for low diameters between the calibration matrix method and the log-normal model curves on , especially for Sidestream® and Aeroneb Go® nebulizers. This gap appears wider than in reality for particles less than 1.0 μ m due to the log-normal scale, and would not be so clearly visible with a normal scale.
The method recommended by the European Standard (EN 13544-1, Appendix CC.3, 2001) is based on the assumption that cascade impactor collection efficiency is ideal, which is not the case, but it has the advantage of being a simple and rapid method of plotting aerosol particle size distribution.
The “middle of cut-off diameters” method developed by Dunbar (CitationDunbar and Hickey, 2000), which consists in dividing the mass fractions by the Dcj ranges to normalize the distribution (), can result in a representation of the distribution which varies widely from other methods, as Dcj ranges decrease sharply from the first to the last impactor stage.
The calibration matrix method which has been developed in this study represents a more precise way to process cascade impactor data, as it takes into account imperfect collection efficiency of the impactor and is based on many individual calibration attempts using different sizes of monodisperse particles. In contrast to the study of CitationPicknett (1972) which considered only cumulative distributions, our study focuses not only on cumulative distribution but also on differential distribution. Moreover, our study develops the matrix concept as an easier way to calculate distributions by simple multiplication on a spreadsheet (Excel file). The assumption used for the calibration matrix method, that no particles less than 0.1 μ m would be measured, is also justifiable by the fact that for all the nebulizers and the DPI tested, deposition on the final filter (0.1–0.52 μ m) was less than 0.3% of the total deposited mass, which is negligible. The impactor considered in the study of Picknett (May's cascade impactor) consisted of 4 stages only and was characterized by collection efficiency curves which were far from sharp, especially for stage 1. Thus the method developed by Picknett may lead to larger differences when compared to other methods, in comparison with our study which considers an 8-stage impactor with sharp collection efficiency curves. As the calibration matrix method is more precise than usual methods, it achieves more accurate results and its use in our study is therefore of great interest to highlight the differences with usual methods. The authors would be pleased to make this ready-prepared spreadsheet (Excel file) available to anyone who would be interested in using the calibration matrix method.
Differences between the methods appear larger when looking at the differential distributions than at the cumulative distributions because the cumulative distribution, which represents the sum of drug deposited from the first to the last impactor stage, lessens the differences between the distribution curves due to the summation.
Statistical comparisons of the calibration matrix method with the European Standard method and the log-normal model highlighted differences (p < 0.05)—or a strong tendency to be different (p < 0.10)—in terms of MMAD and respirable fractions for all aerosol generators. Differences observed between the different methods were fairly large for Turbuhaler®, and slightly lower for nebulizers. The largest differences were obtained for the PariLC+® nebulizer in terms of MMAD (28%), and for Turbuhaler® in terms of respirable fraction (64%).
However, the usual methods would result in acceptable predictions for nebulized aqueous solutions such as those considered in this study, as for a single nebulizer, the prediction of deposition sites in the respiratory tract would be similar whatever the method used to calculate the MMAD value. According to the European Standard (EN 13544-1, Appendix BB, 2001), most particles with a diameter larger than 5 μ m deposit in the oropharyngeal region, particles with a diameter between 2 μ m and 6 μ m mainly deposit in the trachea and the bronchi, and particles with a diameter between 0.5 μ m and 3 μ m deposit in the bronchiolar and alveolar region. For all the nebulizers, each method predicts a major deposition in the pulmonary region, and for the DPI they all predict a major deposition in the oropharyngeal region. Considering only aerosols generated by nebulizers, the log-normal model fitted the distributions reasonably correctly, which is probably mainly explained by the fact that aqueous aerosols produced by nebulizers are usually considered as distributing log-normally. This model is widely used to fit aerosol distributions (e.g., CitationLeung et al. 2005; CitationKwong et al. 2000; CitationAug et al. 1991). With regard to the calibration matrix method, no fundamental changes were observed in terms of either MMAD or respirable fraction compared to usual methods as they all predicted similar deposition sites. This could be explained by the fact that if the mass of particles larger than Dcj that penetrate the stage is compensated exactly by the mass associated with particles finer than this size collected (i.e., if the real collection efficiency curve for every stage is symmetrical compared to the point (Dcj, E = 50%), the particle size distributions plotted following the usual methods and the calibration matrix method may be very similar.
Although this work focused mainly on aqueous aerosols generated by nebulizers, a DPI-produced aerosol was tested using an experimental set-up similar to the one used in the study of CitationRubow et al. (1987) from which calibration data were taken. As expected, the DPI was characterized by a considerable deposition of large particles, resulting in a bi-modal particle size distribution. DPIs are usually sized with impactors including an induction port (throat), and deposition of large particles on this induction port is not taken into account when calculating MMAD and respirable fraction values. In this study, the impactor used did not include any induction port, so that all the large particles, usually deposited in the throat, were deposited on the first stage. This explains the high value of MMAD (around 13 μ m), and also the shape of the curves. Indeed, the cumulative mass distribution obtained with the European Standard method stops at 55% because deposition on the first stage is not taken into account as it is the undersize cumulative mass, i.e., distribution “smaller than the cut-off diameter” (). The differential mass distribution shows that the calibration matrix method is the only one to highlight the bi-modal distribution, which can be explained by the fact that the Histogram and Middle of cut-off methods do not take into account the deposition on the first stage either (). Indeed, the middle of the cut-off diameters for the first stage is undefined as there is no previous stage.
The same kind of result may be obtained for Metered Dose Inhalers (MDIs) (CitationIula et al. 1997; CitationLeach et al. 2005) and aerosols from ambient atmosphere which contain both small and large particles, and may also be represented by a bi-modal distribution or a dissymmetrical distribution on a log-normal scale. Like the DPI Turbuhaler®, the distribution could not be approximated by a unimodal log-normal model as it may give disparate values of MMAD and respirable fraction compared to the other methods.
MMAD and respirable fraction are the parameters most frequently taken into account to estimate aerosol particle deposition in patients' airways. However, MMAD may not be sufficient to describe distribution, especially bi-modal distribution. Thus, it can be imagined that more precise methods of deposition site prediction, breaking down the distribution into smaller diameter ranges to estimate precisely the fraction of aerosol deposited in all the bifurcations of the airways, would produce greater divergence between the methods.
As the ultimate purpose of cascade impactor measurement is to predict aerosol particle deposition in patients' airways, differences in particle size distributions between the different methods used would lead to differences in determining the suitability of an aerosol for pulmonary inhalation. However, it must be emphasized that other parameters can have greater influence on aerosol particle size and prediction of particle deposition in the respiratory tract than the way cascade impactor data is processed. Humidity is well known to have a strong effect on particle size, as hygroscopic particles may grow when entering the respiratory tract which is saturated in humidity (CitationFerron 1977). Patients' breathing (CitationHeyder et al. 1980) and respiratory tract morphology (CitationHeyder et al. 1988) can also have a strong influence on particle deposition in the airways. Consequently, all these parameters should also be taken into account when predicting the aerosol deposition site. Future models of deposition prediction which include these parameters, and requiring precise aerosol particle size distribution such as that obtained with the calibration matrix method, are worth developing.
APPENDIX 1
Calculation of Mass Fractions from Collection Efficiency Data
The collection efficiency (Ej) of stage (j) for a specific particle size is defined as (CitationMitchell et al. 1988):
For the first stage of the impactor (j = 1): E
1 =
This expression corresponds to the definition of the mass fraction collected on stage 1, so:
Equation [Equation1] is equivalent to f j = E j∑k = j n f k, which is equivalent to f j = E j(∑k = 1 n f k− ∑k = 1 j− 1 f k)
The definition of the mass fraction implies ∑k = 1 n f k = 1, thus Equation [Equation1] is equivalent to:
It is then possible to calculate the mass fractions of monodisperse aerosols deposited on each stage (Matrix B in the study) as:
• | Ej is known from study of CitationRubow et al. (1987), | ||||
• | f1 = E1, | ||||
• | Expression [Equation4] allows calculation of fj from Ej and fj− 1, …, f1. |
APPENDIX 2 Calibration matrix corresponding to the Marple Model 298 Personal cascade impactor
Notes
1Significant statistical differences between the calibration matrix method and the two other methods are indicated with ‘*’.
2(1) ultrasonic nebulizers, (2) jet nebulizer, (4) vibrating mesh nebulizer, (4) dry powder inhaler (DPI).
1Significant statistical differences between the calibration matrix method and the two other methods are indicated with ‘*’.
2(1) ultrasonic nebulizers, (2) jet nebulizer, (4) vibrating mesh nebulizer, (4) dry powder inhaler (DPI).
REFERENCES
- Aug , C. , Perry , R. J. and Smaldone , G. C. 1991 . Technetium 99m Radiolabeling of Aerosolized Drug Particles from Metered Dose Inhalers . J. Aerosol Med. , 4 : 127 – 138 . [INFOTRIEVE] [CSA]
- Dunbar , C. A. and Hickey , A. J. 2000 . Evaluation of Probability Density Functions to Approximate Particle Size Distributions of Representative Pharmaceutical Aerosols . J. Aerosol Sci. , 31 : 813 – 831 . [CROSSREF] [CSA]
- Dunbar , C. and Mitchell , J. 2005 . Analysis of Cascade Impactor Mass Distributions . J. Aerosol Med. , 18 : 439 – 451 . [INFOTRIEVE] [CROSSREF] [CSA]
- EN 13544-1 . 2001 . Respiratory therapy equipment—Part 1: Nebulising systems and their components , Official Journal of the European Union .
- EN 1822-1 . 1998 . High efficiency air filters (HEPA and ULPA). Classification, performance testing, marking , British-Adopted European Standard .
- European Pharmacopeia . 2002 . “ Chapter 2.9.18—Preparations for inhalation: Aerodynamic assessment of fine particles ” . In European Pharmacopeia , 3rd ed. , Strasbourg, , France : Council of Europe . [Suppl 2001]
- Ferron , G. A. 1977 . The Size of Soluble Aerosol Particles as a Function of the Humidity of the Air. Application to the Human Respiratory Tract . J. Aerosol Sci. , 8 : 251 – 267 . [CROSSREF] [CSA]
- Heyder , J. , Gebhart , J. , Rudolf , G. and Stahlhofen , W. 1980 . Physical Factors Determining Particle Deposition in the Human Respiratory Tract . J. Aerosol Sci. , 11 : 505 – 515 . [CROSSREF] [CSA]
- Heyder , J. , Gebhart , J. and Scheuch , G. 1988 . Influence of Human Lung Morphology on Particle Deposition . J. Aerosol Med. , 1 : 81 – 88 . [CSA]
- Iula , A. K. , Flynn , C. L. and Deluccia , F. 1997 . Comparative Study of the In Vitro Dose Delivery and Particle Size Distribution Characteristics of An Azmacort Metered-dose Inhaler in Combination with Four Different Spacer Devices . Curr. Ther. Res. , 58 : 544 – 554 . [CROSSREF] [CSA]
- Kwong , W. T. J. , Ho , S. L. and Coates , A. L. 2000 . Comparison of Nebulized Particle Size Distribution with Malvern Laser Diffraction Analyser versus Andersen Cascade Impactor and Low-Flow Marple Personal Cascade Impactor . J. Aerosol Med. , 13 : 303 – 314 . [INFOTRIEVE] [CSA]
- Leach , C. L. , Davidson , P. J. , Hasselquist , B. E. and Boudreau , R. J. 2005 . Influence of Particle Size and Patient Dosing Technique on Lung Deposition of HFA-Beclomethasone from a Metered Dose Inhaler . J. Aerosol Med. , 18 : 379 – 385 . [INFOTRIEVE] [CROSSREF] [CSA]
- Leung , K. , Louca , E. , Gray , M. , Tipples , G. and Coates , A. L. 2005 . Use of the Next Generation Pharmaceutical Impactor for Particle Size Distribution Measurements of Live Viral Aerosol Vaccines . J. Aerosol Med. , 18 : 414 – 426 . [INFOTRIEVE] [CROSSREF] [CSA]
- Marple , V. A. 1970 . A Fundamental Study of Inertial Impactors , Ph.D. thesis Minneapolis : University of Minnesota .
- Marple , V. A. , Olson , B. A. , Santhanakrishnan , K. , Mitchell , J. P. , Murray , S. C. and Hudson-Curtis , B. L. 2003 . Next Generation Pharmaceutical Impactor (A New Impactor for Pharmaceutical Inhaler Testing). Part II: Archival Calibration . J. Aerosol Med. , 16 : 301 – 324 . [INFOTRIEVE] [CROSSREF] [CSA]
- Mitchell , J. P. , Costa , P. A. and Waters , S. 1988 . An Assessment of An Andersen Mark-II Cascade Impactor . J. Aerosol Sci. , 19 : 213 – 221 . [CROSSREF] [CSA]
- Mitchell , J. P. and Nagel , M. W. 2003 . Cascade Impactors for the Size Characterization of Aerosols from Medical Inhalers: Their Uses and Limitations . J. Aerosol Med. , 16 : 341 – 377 . [PUBMED] [INFOTRIEVE] [CROSSREF] [CSA]
- Morrow , P. E. 1974 . Conference on the Scientific Basis of Respiratory Therapy. Aerosol Therapy. Aerosol Characterization and Deposition . Am Rev Respir Dis. , 110 : 88 – 99 . [INFOTRIEVE] [CSA]
- O'Shaughnessy , P. T. and Raabe , O. G. 2003 . A Comparison of Cascade Impactor Data Reduction Methods . Aerosol Sci. Technol. , 37 : 187 – 200 . [CROSSREF] [CSA]
- Picknett , R. G. 1972 . A New Method of Determining Aerosol Size Distributions from Multistage Sampler Data . J. Aerosol Sci. , 3 : 185 – 198 . [CROSSREF] [CSA]
- Rader , D. J. and Marple , V. A. 1985 . Effect of Ultra-Stokesian Drag and Particle Interception on Impactor Characteristics . Aerosol Sci. Technol. , 4 : 141 – 156 . [CSA]
- Rubow , K. L. , Marple , V. A. , Olin , J. and McCawley , M. A. 1987 . A Personal Cascade Impactor: Design, Evaluation and Calibration . Am. Ind. Hyg. Assoc. J. , 48 : 532 – 538 . [INFOTRIEVE] [CSA]
- Smith , J. E. and Jordan , M. L. 1964 . Mathematical and Graphical Interpretation of the Log-Normal Law for Particle Size Distribution Analysis . J. Colloid Sci. , 19 : 549 – 559 . [CROSSREF] [CSA]
- Thiel , C. G. 2002 . Cascade Impactor Data and the Lognormal Distribution: Nonlinear Regression for a Better Fit . J. Aerosol Med. , 15 : 369 – 378 . [INFOTRIEVE] [CROSSREF] [CSA]
- USP 28-NF 23 . 2005 . Chapter 601—Physical Tests and Determinations: Aerosols , 2359 – 2377 . Rockville, MD : United States Pharmacopeia .