Exposure to airborne pollen from certain plants can cause allergic disease, leading to acute respiratory symptoms. Whole pollen grains, 15–90 μ m-sized particles, provoke the upper respiratory symptoms of rhinitis (hay fever), while smaller pollen fragments capable of depositing in the lower respiratory tract have been proposed as the trigger for asthma. In order to understand factors leading to pollen release and fragmentation we have examined the rupture of Chinese elm pollen under controlled laboratory conditions and in the outdoor atmosphere. Within 30 minutes after immersion in water, 70% of fresh Chinese pollen ruptures, rapidly expelling cytoplasm. Chinese elm flowers, placed in a controlled atmosphere chamber, emitted pollen and pollen debris after a sequential treatment of 98% relative humidity followed by drying and a gentle disturbance. Immunologic assays of antigenic proteins specific to elm pollens revealed that fine particulate material (D p < 2 μ m) collected from the chamber contained elm pollen antigens. In a temporal study of the outdoor urban atmosphere during the Chinese elm bloom season of 2004, peak concentrations of pollen and fine pollen fragments occurred at the beginning of the season when nocturnal relative humidity (RH) exceeded 90%. Following later periods of hot dry weather, pollen counts decreased to zero. The Chinese elm pollen fragments also decreased during the hot weather, but later displayed additional peaks following periods of more moderate RH and temperature, indicating that pollen counts underestimate total atmospheric pollen allergen concentrations. Pollen fragments thus increase the biogenic load in the atmosphere in a form that is no longer recognizable as pollen and, therefore, is not amenable to microscopic analysis. This raises the possibility of exposure of sensitive individuals to pollen allergens in the form of fine particles that can penetrate into the lower airways and pose potentially severe health risks.
INTRODUCTION
Airborne pollen is important from a health perspective because it can trigger acute respiratory symptoms. Pollen grains contain water-soluble antigens, non-native molecules that stimulate the production of antibodies in the human body. A subset of antigens are allergens that elicit an allergic response in atopic individuals, that is, people who are capable of forming immunoglobulin E (IgE) antibodies against foreign molecules. Allergic symptoms resulting from pollen exposure can range from the typical sneezing and watery eyes of hay fever sufferers to wheezing, inflamed constricted airways, and difficult breathing in asthmatics. Hay fever and asthma afflict about 20% and 10% of the U.S. population, respectively. Whole pollen grains, mostly 15–90 μm-sized particles, trigger hay fever by depositing in the eyes and nasal-pharyngeal region of the respiratory tract. They are, however, too large to enter the small airways in appreciable numbers to provoke asthma (CitationBusse et al. 1972). Particles smaller than pollen grains that contain pollen specific antigens have been found in the ambient atmosphere—first from ragweed (CitationBusse et al. 1972), then grass (CitationStewart and Holt 1985), and birch (CitationRantio-Lehtimäki et al. 1994). Concentrations of birch and grass pollen allergens detected in particles smaller than 7.2 μm increase after rain (CitationSchäppi et al. 1997, Citation1999). Intact grass pollen grains have been observed to rupture when placed in water, releasing small (< 3 μm) starch granules and other cytoplasmic debris that contain allergenic material (CitationSuphioglu et al. 1992). More recently, the release of allergen-laden cytoplasm from ruptured grass, birch, alder, and hazel pollens has been demonstrated (CitationTaylor et al. 2002; CitationGrote et al. 2003; CitationTaylor et al. 2004). (CitationTaylor and colleagues 2002, Citation2004) used a controlled emission chamber to show that grass and birch flowers readily released respirable-sized aerosols of pollen cytoplasmic debris in response to a cycle of wetting and drying that was followed by a wind disturbance.
The Chinese elm tree (Ulmus parvifolia, Jacq) is wind-pollinated and a prolific pollen producer. Furthermore, Chinese elm pollen is highly allergenic. In Pasadena, California, where our study was conducted, about 30% of atopic patients show positive skin tests and there is an increase in symptoms of rhinitis and airway hyper-reactivity in patients that correlates with the Chinese elm flowering season. Chinese elm was introduced into the Western United States; there are no native elms west of the Rocky Mountains (CitationSmith 1990). In contrast to other elm species that bloom in the spring in Pasadena, Chinese elm sheds large amounts of pollen in the fall months of August and September, and is the largest contributor to airborne pollen in Pasadena.
The present study examines the contribution of Chinese elm to the allergen load of the atmosphere under controlled conditions both in the laboratory and in the outdoor urban environment. We first characterized pollen rupture, and then investigated the release of Chinese elm pollen and pollen fragments into a controlled atmosphere. Finally, we quantified the amount of Chinese elm pollen and associated pollen fragments released into the ambient atmosphere during the bloom period, and investigated the influence of meteorological factors on their release from the flower.
MATERIALS AND METHODS
Controlled Studies for Pollen Rupture and Emission
The methodology used for the study of pollen rupture and aerosolization was described in CitationTaylor et al. (2002). Briefly, branches containing flower buds were collected from local Chinese elm trees, and transported to the laboratory. Pollen grains that had been collected from open anthers by contact with a microscope slide were evaluated for rupture by the addition of water or by exposure to high relative humidity (RH). Pollen rupture was viewed with a Nikon 80i light microscope with bright-field optics. Images were recorded with a Nikon DXM1200 digital camera using the ACT-1 image capture program (Nikon Instruments Inc., Melville, NY) and Camtasia, version 2.1, image analysis software (TechSmith, Okemos, MI). To study the emission of pollen fragments into the air, flowering branches were placed in a Plexiglas chamber that was supplied air, at controlled relative humidity (RH) and temperature (T) through a HEPA filter to ensure a particle-free background (CitationTaylor et al. 2004). Prior to performing the emission experiments, flowering branches were kept in the chamber for at least 3 h at 95% RH. Dry air was then passed through the chamber to stimulate opening of the flowers. Upon flowering, the chamber was humidified to 98% RH for 5 h, followed by drying at 25% RH for 1 h. An electric fan was used to generate a wind disturbance. The air exhausted from the chamber was sampled for particle size distribution measurements using a TSI Model 3321 Aerodynamic Particle Sizer (TSI Inc., Shoreview, MN). Samples were also collected for immunoanalysis using an AGI-30 all-glass impinger (Ace Glass Co., Vineland, NJ). Two stages of a Marple Cascade Impactor (Sierra Instruments Inc., Monterey, CA) with greased impaction substrates were installed upstream of the impinger to remove particles larger than 2 μm diameter from the air stream. Microscopic examination indicated that the impinger liquid contained particles of a diameter less than 2 μm.
Ambient Pollen Counts
Throughout the 2004 Chinese elm bloom season pollen was sampled with a Burkard 7-day volumetric spore trap (Burkard Manufacturing, Rickmansworth, Hertsfordshire, UK) located on the roof of a three-story building on the campus of the California Institute of Technology, Pasadena, CA. The sampling location was chosen to measure pollen counts and allergen levels reflecting ambient atmospheric concentrations in broad regions, not affected by close proximity to a particular tree. There were no Chinese elm trees closer than 150 m. The sampler, operated at an air flow rate of 10 1/min, collected pollen in the air stream by impaction onto Mylar tape that was pre-coated with Sylgard 527 silicone dielectric gel (Dow Corning, Midland, MI) and mounted on a rotating drum. The tape was removed after 7 days and mounted on microscope slides with an adhesive mixture (35 g Mowiol grade 40–20, 100 ml H2O, 50 ml Glycerol, 2 g Phenol). Pollen was identified and counted by a pollen counter certified with the US National Allergy Bureau utilizing a Nikon light microscope (Nikon Instruments Inc., Melville, NY), and results were expressed as counts/m3 of air, 12 h averaged.
Ambient Air Samples
Fine particulate matter was initially collected in September of 2003, utilizing the same sampling configuration as described above for the collection of emissions from the Chinese elm flowers in the controlled chamber studies. During the Chinese elm season the following year, fine ambient particulate matter was sampled adjacent to the Burkard spore trap from August 26 to September 21, 2004, and subsequently immunoanalyzed for elm pollen antigens. These ambient aerosol samples were collected downstream of an AIHL-design cyclone separator (CitationJohn and Reischl 1980) that was machined at the California Institute of Technology (Pasadena, CA) and that has been utilized extensively by the Cass group (CitationSchauer et al. 2002 and references therein). The cyclone was operated at 25.7 1/min, to remove particles with aerodynamic diameters larger than 2 μm and samples collected down stream, by filtration or impingment, contained no pollen or large particles when examined by light microscopy. The exit of the cyclone was divided between two AGI-4 all glass impingers (Ace Glass Co., Vineland, NJ) with a 4 mm nozzle-to-plate distance. Twenty ml of sterile sampling liquid (MilliQ water (Millipore, Billerica, MA), containing 0.2% Na azide) were added to the impingers. Under normal operating conditions, about 1% of the impinger liquid is evaporated per min (CitationLin et al. 1997). To compensate for this loss, the impingers were modified to maintain sampling liquid volume by adding a constant stream of sterile water using a syringe pump throughout the 8 h collection period. Experiments using dye tracers indicate 25–30% sample loss during the sampling period.
Immunoassays for Elm Pollen Antigens
Elm pollen antigens were detected and quantified using a modification of the immunoblot method of (CitationMiguel et al. 1996, Citation1999). Test samples and Chinese elm pollen reference standard (Hollister-Stier Labs, Spokane, WA) dilutions were applied to nitrocellulose membranes, using a dot micro-filtration apparatus (Bio-Rad Laboratories, Hercules, CA). The membranes were washed with TBS (0.05 M Tris-buffered saline), blocked with 2% polyvinyl alcohol for 1 h and washed with TBS containing 0.05% Tween 20. A rabbit anti-American elm (Ulmus americana) pollen IgG antibody (Greer Laboratories, Lenoir, NC) was added for the detection of elm antigens. The membranes were incubated overnight at 4°C. After washing, a conjugate antibody (goat, anti-rabbit IgG alkaline phosphatase) was added. The membranes were then incubated for 1 h, washed, and developed in Western Blue stabilized substrate (Promega, Madison, WI). The quantity of antibody bound was determined by measuring the relative color development using an Alpha Manager 2200 documentation and analysis system (Alpha Innotech Corporation, San Leandro, CA). The following controls were performed: omission of rabbit antibody, use of non-immune rabbit sera, and omission of the air sample extract. Tests using recombinant birch allergen, r Bet v 1, and rabbit anti-rBet v 1 antibody were performed to investigate antigen specificity.
Meteorological Data
Meteorological parameters were measured at 10 min intervals using a Vantage Pro Plus weather station (Davis Instruments, Hayward, CA) equipped with a data logger connected feed to a computer data storage. The station was located 3.8 m above (on a penthouse) and 41 m south of the Burkard pollen trap and fine particle impinger samplers.
RESULTS
Pollen Rupture
Freshly harvested Chinese elm pollen grains are 18–30 μm in diameter. When exposed to 98% RH for 10 min before immersion in water, about 70% of the freshly collected grains ruptured, rapidly expelling cytoplasm (, and Supplemental Material, Video S1). The period between immersion and rupture was approximately 30 min, but the interval lengthened for pollen that was collected during hot, dry conditions. Prior to pollen rupture, the pollen grain swells to about 26–36 μm, and the cell membrane distends out of the germinal pore as seen in . Upon exposure to high RH, either alone or with immersion in water, a pollen tube often emerged from the germinal pore, but rapid osmotic swelling causes the pollen grains to rupture and release the cytoplasmic contents into the water.
Chamber Release of Aerosol
To examine the emission of pollen debris under controlled atmospheric conditions, fresh Chinese elm branches were stimulated to flower within a chamber. Upon exposure to drying conditions, the anthers opened (dehisced). As previously found for grass pollen (CitationTaylor et al. 2002), the vast majority of pollen remained on the anthers. Flowers exposed to a cycle of wetting and drying, followed by a wind disturbance, released particles into the atmosphere. The number size-distribution measured after this cycle () shows the emission of whole Chinese elm pollen, peaking at 22 μm, and a large number of smaller particles with a peak at about 3 μm and ranging down to 1.6 μm.
FIG. 2 Emissions from Chinese elm flowers housed within a controlled emission chamber showing the number size distribution, of pollen grains, collapsed pollen, and pollen cytoplasmic fragments released into each cubic centimeter of sampled air.
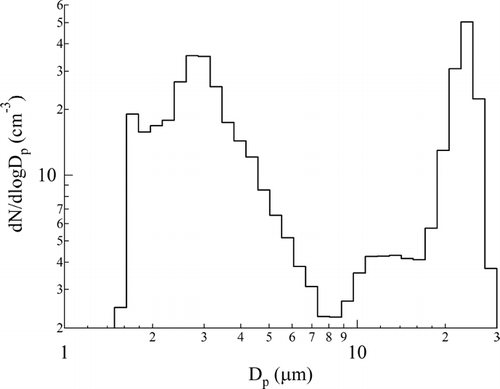
The fine particles collected from the chamber contained up to 2.9 μg of elm pollen antigens/m3 of air sampled (), indicating the presence of pollen rupture fragments. The spot density on the dot blots is proportional to the amount of antibody bound and consequently to the amount of pollen antigen in the spot. Cross-reactivity between Chinese elm and American elm antigens occurs because their proteins are closely related and have the same structure in the antibody binding regions. There was no color present on a blot of birch pollen allergen (rBet v 1) probed with rabbit anti-American elm pollen antibody (not shown), indicating no cross-reactivity. No color was present on the negative control blots probed with serum from rabbits that had not been immunized.
FIG. 3 Immuno-dot blot analysis of Chinese elm pollen antigens in fine particles (Dp < 2 μm) collected from the controlled emission chamber, and from outdoor air samples. Samples (and air volume sampled per spot): (A) indoor chamber (20 1); (B) Ambient (870 1); (C) Field—disturbed trees (50 1); (D) Ambient (190 1).
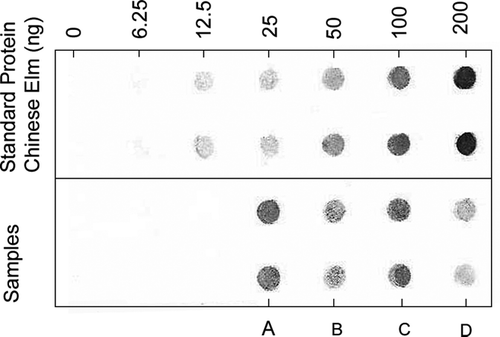
Pollen Counts and Pollen Fragment Concentrations in the Outdoor Aerosol and the Effects of Meteorological Parameters
In the outdoor urban environment, elm pollen antigens were also detected in atmospheric fine particulate matter. Initial sampling during September 2003 showed concentrations of elm pollen antigens that varied from 2 μg/m3 under a Chinese elm tree that was physically disturbed by shaking, to a range of 0.014–0.266 μg/m3 in ambient field samples collected at a site located at least 150 m from the nearest Chinese elm tree ().
shows the temporal distribution of ambient atmospheric pollen grains and fragments, and the concurrent meteorological parameters for the period August 25 to September 21, 2004. Pollen counts were consistently lower at night than during the day. At the beginning of the sampling period, Chinese elm pollen was about 15% of the total pollen count, increasing to 96–99% (up to 560 counts/m3 of air, ∼ 3 μg/m3 of whole pollen) during the 60 h peak in emissions, and then declining abruptly on September 1. The remainder of the pollen was identified as weed pollen from the Chenopodiaceae family. Pollen was not counted during 2.5 days due to sampler failure. The ambient fine particulate matter (Dp < 2 μm), collected each day for an 8 h period starting at approximately 9:00 AM was screened for the presence of elm pollen antigens to assess the level of airborne pollen fragments. The highest concentrations of pollen antigens (23.5 ng/m3) coincided with the peak period of high Chinese elm pollen counts. However, elm pollen antigens were still detected (8 ng/m3) after the pollen concentration had fallen below 20 m−3 of air, less than 5% of the peak Chinese elm pollen count.
FIG. 4 Total pollen count, Chinese elm pollen count, and concentration of fine-sized Chinese elm pollen antigens (Dp < 2 μm), in ambient air samples compared with meteorological parameters during the 2004 Chinese elm bloom that began in August 25. Pollen counts from the night (11 PM–11 AM) and day (11 AM–11 PM) are given per cubic meter of air, 12 h averaged. Concentrations of elm pollen antigen are expressed per cubic meter, and were sampled during the day (8 h). Comparisons are made with relative humidity (RH), temperature (T), and wind speed.
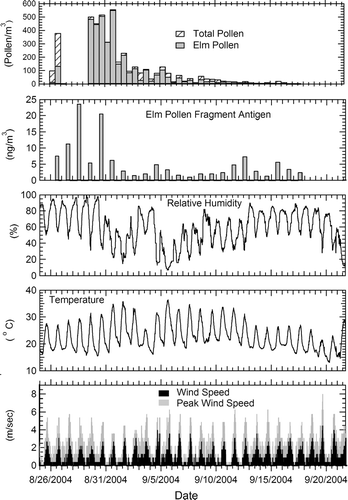
The meteorological conditions throughout the study showed normal diurnal patterns (). For the 10 days preceding the initiation of Chinese elm flowering, RH peaked at 86–98% during the night, dropping to a low of 45–50% in the day (data not shown). During the same period, the daily peak temperature was 25–20°C, while the nightly low was 16–17°C. Three episodes of unusually dry weather, occurring on 8/29–9/2, 9/4–9/8, and 9/19, broke the seasonal norm. RH as low as 15%, 7%, and 12% were reached during the day in episodes 1, 2, and 3, respectively, while peak night-time RH only reached 55%, 44%, and 31%, respectively. During the first two events, temperatures were unusually high, with maxima reaching 35.8°C and 36.4°C. Winds were consistently lower at night than during the day. Nightly wind speeds, averaged over 10 min intervals, were below 1.5 m/s with gusts occasionally reaching 2.5 m/s while average values during the day reached 2.5 m/s with gusts in the 5–6 m/s range.
DISCUSSION
The laboratory studies presented here reveal that Chinese elm pollen ruptures in moist weather and emits numerous fine particles into the air. The germinal pore of the pollen grain ruptures under osmotic pressure and releases its contents within seconds. This mode of release is similar to that found in an earlier study of highly allergenic rye-grass pollen (CitationTaylor et al. 2002). Rupture also occurred after growth of a short pollen tube, as previously reported for birch, alder and hazel pollen (CitationGrote et al. 2003; CitationTaylor et al. 2004). We have observed that the rupture process is not unique to allergenic wind-dispersed pollen, but also occurs with pollen types that are usually spread by contact with insects or birds. Our controlled chamber experiments showed that Chinese elm flowers, exposed to high RH followed by drying winds, release particles with a wide range of sizes into the atmosphere. Evident in the particle number size distribution are three size modes. The largest diameter peak (18–30 μm), near the upper limit of the particle size range detected by the Aerodynamic Particle Sizer Spectrometer, corresponds to intact pollen, while the intermediate mode comprises partially emptied and collapsed pollen grains. The third mode has large numbers of particles from 7 μm down to 1.6 μm. Even though no particles were apparent near the lower limit of the size distribution measurements at 0.5 μm, it is quite likely that smaller particles were shed. Results from an earlier study of grass, utilizing an optical particle counter, showed the release of particles in the 0.12–4.67 μm range (CitationTaylor et al. 2002). The size distribution of birch pollen fragments, measured using a differential mobility analyzer, revealed particle emissions in the 30–700 nm range (CitationTaylor et al. 2004). Our detection of elm pollen antigens in the respirable aerosol that was collected during the controlled chamber experiments confirmed that considerable amounts of highly allergenic pollen fragments are emitted from flowers, and these aerosols can be quantified using an appropriate air sampling and immunoanalysis technique.
Results from our outdoor time-course studies show that RH, T and wind all affect the production and dispersal of pollen grains and fragments. In southern California, Chinese elm is a prolific pollen producer from late August through September when diurnal variations in RH range from above 40% in the day to above 80% at night, and T varies from 16°C to 25°C. As in previous seasons, the Chinese elm pollen count rose abruptly at the start of the 2004 season. However, our results showing an abrupt drop in pollen counts after a three-day episode of Santa Ana winds, reveal the importance of RH and T on pollen production and release. During Santa Ana conditions, air sweeps over the local mountains and undergoes compression heating as it descends. During the first Santa Ana episode, the afternoon minimum RH ranged from 15% to 31%, and T exceeded 30°C, whereas peak nighttime RH ranged from 55% to 73% with T below 20°C. These hot, dry Santa Ana winds led to high vegetative transpiration and surface evaporation, which, in turn, desiccated the flowers, and, eventually, the flower buds. The stress produced by these severe meteorological conditions shortened the Chinese elm pollination period in the 2004 season. Previous seasons showed a second peak in flowering that correlated with the onset of cooler, moist weather. The severity of the Santa Anna in 2004 likely prevented a substantial second peak in flowering, even after subsequent intermittent periods of more moderate weather. We observed higher pollen counts during the day, when average wind speeds reached 3 m/sec in the afternoon, with gusts in the 5.5–6.5 m/sec range, as compared to the night, when average wind speeds were often zero with occasional gusts up to 2.5 m/sec. Thus, wind speeds above 2.5 m/sec appear to be needed to disperse pollen grains from the flowers. The higher wind speeds that are often found during the day result in considerable emission and distribution of pollen grains.
The present study demonstrates that meteorological conditions also impact the formation and distribution of allergen-bearing pollen fragments. Immunological analysis of outdoor air samples confirmed the presence of Chinese elm pollen fragments in particles smaller than 2 μm. This observation is consistent with previous published observations of grass and birch pollen fragments in the air, especially after rain or a thunderstorm (CitationStewart and Holt 1985; CitationRantio-Lehtimäki et al. 1994; CitationSchäppi et al. 1997, Citation1999). In Southern California, Chinese elm typically blooms at a time of the year when there is no precipitation. This suggests another trigger for the observed levels of pollen fragments. Our laboratory studies and ambient data suggest that RH is that trigger. At the beginning of the season, when the nocturnal RH consistently reached or exceeded 90%, the atmospheric fine pollen fragment concentrations increased as the pollen counts increased, and then declined as the pollen count dropped. Even though there was no second peak in the pollen count during the 2004 Chinese elm season, the airborne pollen fragment concentration peaked three additional times during the flowering season when the ambient nocturnal RH surpassed 80%. The pollen fragment release occurred two days after the return of high nocturnal RH. The RH levels on the surface of the flower may exceed that of the ambient air due to the boundary layer, plant transpiration, and dew formation.
The exposure to pollen allergens based on pollen counts clearly underestimates exposure since allergen-bearing pollen debris is also released into the air. Pollen fragments are found in the air even after pollen counts drop to zero. Thus, people are exposed to pollen allergens for longer times than would be predicted from pollen counts alone. Exposure to pollen fragments that are dispersed in the outdoor atmosphere presents a different health risk that posed by whole pollen. Pollen fragments may penetrate the lower airways where they may trigger a hyper-reactive response. The quantities of pollen and fragments that we measured in the atmosphere suggest that Chinese elm may contribute considerably to the hay fever and asthma risk not only in Pasadena, but across the urban environment of Southern California. It is worth noting that Chinese elm is recommended by the Pasadena City Council for use as a street tree.
While the initial discovery and study of pollen fragmentation has been driven by health concerns, it is important to note that pollen fragments may contribute to the atmospheric organic carbon content of particles in both the coarse and fine size ranges. About 90% of the organic mass of airborne fine particulate matter has yet to be identified (CitationRogge et al. 1993). Pollen fragments increase the biogenic content of atmospheric aerosols in a form that is no longer recognizable as pollen and is, therefore, not amenable to microscopic analysis. Attempts to characterize this biogenic fraction have been limited by the challenges of analyzing this heterogeneous mix of complex bio-compounds, especially when combined with the presence of anthropogenic air pollutants. Although this study only reports the quantification of one pollen type, many plants contribute to the biogenic aerosol burden. Potential environmental and climatological impacts of pollen fragments, including their possible role as cloud condensation nuclei, remain unknown at this time.
SUPPLEMENTAL MATERIAL AVAILABLE
Video SI. Video light microscopy of Chinese elm pollen after it was immersed in water and showing the release of fragments of cytoplasm as well as the two sperm cells. This video in real time, covers 20.1 sec, and is played at 12 fps. It is available free of change on the journal's online website in both AVI (Video Clip, 18.6 MB) and MOV (QuickTime, 2.68 MB) formats.
Supplementary_Video_2
Download Microsoft Video (AVI) (18.7 MB)Supplementary video (mov)
Download QuickTime Video (2.7 MB)Acknowledgments
We would like to thank Robert Esch of Greer Laboratories, Lenoir, NC, for his kind gift of rabbit anti-American elm pollen antibody used for the detection of elm antigens. This work was supported by Philip Morris USA, Inc., Philip Morris International, and the Southern California Environmental Health Sciences Center (NIEHS grant number 5P30 ES07048). P.T. was supported by a Boswell Fellowship from HMRI and Caltech.
REFERENCES
- Busse , W. W. , Charles , E. R. and Hoehne , J. H. 1972 . Where is the Allergic Reaction in Ragweed Asthma? II Demonstration of Ragweed Antigen in Airborne Particles Smaller than Pollen . Allergy Clin. Immunol. , 50 : 289 – 293 .
- Grote , M. , Valenta , R. and Reichelt , R. 2003 . Abortive Pollen Germination: A Mechanism of Allergen Release in Birch, Alder, and Hazel Revealed by Immunogold Electron Microscopy . Allergy Clin. Immunol. , 111 : 1017 – 1023 . [CROSSREF]
- John , W. and Reischl , G. 1980 . A Cyclone of Size-Selective Sampling of Ambient Air . JAPCA , 30 : 872 – 876 . [CSA]
- Lin , X. , Willeke , K. , Ulevivius , V. and Grinshpun , S. A. 1997 . Effect of Sampling Time on the Collection Efficiency of All-Glass Impingers . Am. Ind. Hyg. Assoc. J. , 58 : 480 – 488 . [CSA]
- Miguel , A. G. , Cass , G. R. , Weiss , J. and Glovsky , M. M. 1996 . Latex Allergens in Tire Dust and Airborne Particles . Environ. Health Persp. , 104 : 1180 – 1186 . [CSA]
- Miguel , A. G. , Cass , G. R. , Glovsky , M. M. and Weiss , J. 1999 . Allergens in Paved Road Dust and Airborne Particles . Environ. Sci. Technol. , 33 : 4150 – 4168 . [CSA] [CROSSREF]
- Rantio-Lehtimäki , A. , Viander , M. and Koivikko , A. 1994 . Airborne Birch Pollen Antigens in Different Particle Sizes . Clin. Exp. Allergy , 24 : 23 – 28 . [CSA] [CROSSREF]
- Rogge , W. F. , Mazurek , M. A. , Hildemann , L. M. , Cass , G. R. and Simoneit , B. R. T. 1993 . Quantification of Organic Aerosols at a Molecular Level: Identification, Abundance and Seasonal Variation . Atmos. Environ. , 27 : 1309 – 1330 . [CSA]
- Schäppi , G. F. , Suphioglu , C. , Taylor , P. E. and Knox , R. B. 1997 . Concentrations of the Major Birch Tree Allergen Bet v 1 in Pollen and Respirable Fine Particles in the Atmosphere . Allergy Clin. Immunol. , 100 : 656 – 661 . [CSA] [CROSSREF]
- Schäppi , G. F. , Taylor , P. E. , Pain , P. A. , Cameron , A. W. , Dent , A. W. , Staff , I. A. and Suphioglu , C. 1999 . Concentrations of Major Grass Group 5 Allergens in Pollen Grains and Atmospheric Particles: Implications for Hay Fever and Allergenic Asthma Sufferers Sensitized to Grass Pollen Allergens . Clin Exp. Allergy , 1999 : 633 – 641 . [CSA]
- Schauer , J. J. , Fraser , M. P. , Cass , G. R. and Simoneit , B. R. T. 2002 . Source Reconciliation of Atmospheric Gas-Phase and Particle-Phase Pollutants during a Severe Photochemical Smog Episode . Env. Sci. Technol. , 36 : 3806 – 3814 . [CSA] [CROSSREF]
- Smith , E. G. 1990 . Sampling and Identifying Allergenic Pollens and Molds , San Antonio, TX : Blewstone Press .
- Stewart , G. A. and Holt , P. G. 1985 . Submicronic Airborne Allergens . Med. J. Aust. , 143 : 426 – 427 . [INFOTRIEVE] [CSA]
- Suphioglu , C. , Singh , M. B. , Taylor , P. , Bellomo , R. , Holmes , P. , Puy , R. and Knox , R. B. 1992 . Mechanism of Grass-Pollen-Induced Asthma . Lancet , 339 : 569 – 572 . [INFOTRIEVE] [CSA] [CROSSREF]
- Taylor , P. E. , Flagan , R. C. , Valenta , R. and Glovsky , M. M. 2002 . Release of Allergens as Respirable Aerosols: A Link Between Grass Pollen and Asthma . Allergy Clin. Immunol. , 109 : 51 – 58 . [CSA] [CROSSREF]
- Taylor , P. E. , Flagan , R. C. , Miguel , A. G. , Valenta , R. and Glovsky , M. M. 2004 . Birch Pollen Rupture and the Release of Aerosols of Respirable Allergens . Clin. Exp. Allergy , 34 : 1591 – 1596 . [INFOTRIEVE] [CSA] [CROSSREF]