Abstract
This study presents results from tail pipe emissions of particulate matter from a turbocharged common rail 2-stroke marine diesel engine (4RTX-3 from Wärtsilä) investigated at various operating conditions and using two different fuels. Size distributions were measured with a SMPS (Scanning Mobility Particle Sizer). A thermodesorber (TD) was applied to remove volatile material. In addition, filter samples were taken for gravimetric and chemical analysis. The mean diameters of the particles ranged between 20–40 nm, which is considerably smaller than the diameter of particles known from 4-stroke diesel engines as used in cars. A TD operated at 400°C evaporated the majority of the particles. The particle mass is dominated by volatile organic material, the fraction of which is significantly higher than for engines in cars. A high nucleation mode was found instead of a pronounced accumulation mode as known from 4-stroke diesel engines.
INTRODUCTION
While in the early days of diesel engines their smoke plumes caused poor visibility when driving behind a truck, with the now-clean diesel engines another issue arises. Although no visible emission is left, diesel engines were found to emit small particles, which according to studies done in the past years can cause significant health problems (e.g., CitationLighty et al. 2000). Many toxicological and epidemiological studies established adverse health effects by particulate matter (PM10, PM2.5). There is increasing evidence that several health effects are associated with the ultrafine particles with diameters below 100 nm (CitationBrown et al. 2001). Recent research shows that they can penetrate the cell membranes, enter into the blood and even reach the brain (CitationOberdörster et al. 2004).
Therefore, great efforts are taken to investigate and reduce particle emissions from diesel engines installed in on-road and off-road vehicles like heavy-duty trucks or cars. Within the last years a large number of studies on particle emissions were done, which focus on particle emissions from on-road and off-road vehicles; yet studies on particle emissions from ships are quite rare. CitationCorbett and Koehler (2003) published a comprehensive study on emissions from ocean shipping that focused on gaseous emissions. The presented emission factors for marine diesel engines are reportedly very uncertain because they are difficult to measure and also a reliable database is not available at the moment. The world fleet annual PM emission is estimated at 1.64 Tg PM10 (CitationCorbett and Koehler 2003).
Whereas for on-road vehicles only 4-stroke engines (gasoline and diesel) are applied, both 2-stroke and 4-stroke diesel-type engines are used for marine applications, although serving different purposes. In this context, engines with bores larger than 200 mm are referred to as marine diesel engines. Small 4-stroke marine diesel engines are used as auxiliary engines onboard ships, while large ones for passenger transportation (ferries, cruise ships). Two-stroke diesel engines generally power vessels intended for transportation of goods. This splitting is mainly due to 4-stroke engines running more smoothly than 2-stroke engines, which makes them more convenient for transportation of passengers. Also their piston displacement is smaller, which requires less space on the vessel and allows for smaller engine rooms. Two-stoke engines have larger displacements and are more powerful than 4-stroke engines with similar bores, making them the ideal choice for fast and reliable transportation of goods where delivery dates are crucial.
Two studies (CitationLyyränen et al. 1999; CitationLyyränen et al. 2002) deal with emissions from 4-stroke medium-speed engines operated with heavy fuel oil. The author observed two distinct particle modes, the transition being at about 400 nm. The small particles were found to have diameters smaller than 400 nm, with the particle mode being between 40–45 nm, independent on engine load and these particles were found make up for 60–70% of the total mass. Within the publication from 2002 particles larger than 400 nm are classified as “coarse mode” with an average aerodynamic diameter that reportedly ranges about 10 μm.
While the results from these two studies help to understand data obtained from our study, the presented work compares particle emissions from 2-stroke marine diesel engines and 4-stroke motor vehicle engines.
Differences between on-road and off-road and marine diesel engines are quite significant. First, they are operated with highly different fuels. Fuels used for engines operated on land are refined, high-quality products, while marine diesel engines are operated on bunker fuels. The latter are basically waste products from refinery processes, which implies that all ingredients one does not want to be burned (and its residuals being emitted on land) end up in being emitted on sea, where particulate emissions contribute to cloud formation and thus have an impact on global climate.
Geometric size is another main difference that has an imminent impact on thermodynamics. Large 2-stroke marine diesel engines, with bores up to 960 mm, run at very low speeds (up to 130 rpm) accounting for rather long combustion process. Moreover, as opposed to engines with small displacement, large marine diesel engines (with displacements up to 1809 l) with correspondingly larger combustion chambers offer possibilities to model and improve injection, because large combustion chambers help prevent the fuel from hitting the cylinder walls. As commonly known, due to diesel fuel sprayed onto combustion chamber walls, the lubricating oil film on its surface dissolves, usually resulting in increased particulate matter formation. Hence, combustion is likely to become cleaner due to improved design conditions.
The third big difference is engine operation and engine operating conditions. Four-stroke engines are mainly operated in transient operating conditions, which is reflected in legislation by defining a particulate mass limit measured over a driving cycle including transient operation. Mass limits and driving cycles vary from country to country. For marine diesel engines only gaseous emissions are limited yet, but in view of legislation to come it is important to characterize particle emissions from those engines. The legislative body, the International Maritime Organization (IMO), limited NOX emissions for marine diesel engines running at speeds lower than 130 rpm to 17 g/kWh. This value is to be obtained according to the rules of IMO NOX Technical Code (Annex VI of the MARPOL 73/78 convention), which states that NOX emissions have to be recorded at four constant speed operation points: 100%, 75%, 50%, and 25% load. Each NOX value is weighted according to the IMO NOX Technical Code and their sum yields the current NOX emission value for one engine. This procedure reflects the mode in which the engines are meant to be operated; travelling long distances at constant speed and loads, which range between 75–90% load of CMCR (contracted maximum continuous rating). Low loads, smaller than 25% load of CMCR are mostly coupled to transient conditions basically required while maneuvering in harbors. Correspondingly, no transient test cycle but four distinct and typical load points were chosen by the IMO to determine an emission limit. Following this procedure these four load points—100%, 75%, 50%, and 25%—were chosen to evaluate particulate matter emission within this study.
In view of the discussed differences between marine diesel engines and their motor vehicle counterparts it has to be expected that their particulate matter emissions will be different.
tTo obtain comprehensive characterization of particulate emissions, exhaust gas from a 2-stroke marine diesel engine for tanker applications was investigated. Testing took place at the test bed engine of Wärtsilä Switzerland Ltd. Number size distributions were measured and chemical analyses of particle mass accumulated on filter samples were performed. The same instrumentation generally used for on-road vehicles was used to investigate particulate emissions to allow for comparison of the results.
EXPERIMENTAL SETUP
As already mentioned, a 2-stroke engine for tanker application was investigated here. shows a photograph of the engine. The person standing on the stairs (in between the white bars) visualizes the engine size.
Engine specifications are listed in . To provide comparison of scales the corresponding specifications for a 1.9 l passenger car diesel engine are shown in a second column. This table quantifies the above-discussed differences between a typical on-road diesel engine and the 2-stroke marine diesel engines.
TABLE 1 Engine specifications
The engine was operated at five different operation points, the relation between speed and torque being given by propeller characteristics. At start-up, the engine was conditioned for about 45 minutes to stabilize temperatures before the actual measurements were started. Following IMO requirements 100% to 25% load were the chosen loads. Additionally 1% load was considered as low load operation point representative for maneuvering. Load, speed, and corresponding exhaust gas temperatures as measured at the particle sampling port are listed in .
TABLE 2 Operation points and associated corresponding exhaust gas temperatures, which were recorded during measurement
Two different types of fuel were used in this study: Marine Diesel Oil (MDO) and Heavy Fuel Oil (HFO). While MDO is the standard reference fuel for marine diesel engines, HFO is the fuel actually used on board. Different qualities of HFO are available on the market with IMO limiting the sulfur content since 2005 generally to maximum 2.5% on open sea and 1.5% in coastal regions. HFO is not a distilled fuel, but basically the waste product from refinery processes and typically contains products like ash, asphaltenes, and other sediments. HFO has a thick, honey-like consistency and its color varies from shades of black and brown. It is necessary to filter and heat the fuel to 140–150°C prior to injection. MDO is a rather high-quality distilled fuel, more expensive than HFO, and free of asphaltenes and ash; moreover the sulfur content is limited to 2%. Nevertheless MDO is not suitable for use in on-road and off-road diesel engines.
shows the major fuel specifications of the two fuels used and for comparison specifications of typical diesel oil as available at the pump is listed in a third column. Both fuels used were of relatively good quality with low sulfur content, which still is very high compared to on-road diesel vehicle fuel sold in Europe where sulfur content is usually less than 0.005% (EN590). Ash and vanadium contents in HFO were low, given that upper limits of 0.15% and 300 mg/kg are allowed.
TABLE 3 Major fuel specifications
Particle emissions were measured at each load point with both fuels. Each load point was run once for approximately 45 min, except the 1% load point, where two runs were done to show repeatability. To learn more about repeatability measuring every operating point several times would have been desirable. However, for several reasons this was not possible.
As the test bed is located in the suburbs of Oberwinterthur there are environmental and health issues that restrict the operation of the engine, although it is equipped with a selective catalytic reduction device. Also for economic reasons the test bed cannot be operated like a motor vehicle test bed.
Nevertheless there are several reasons to be confident that the measurements are representative. First, long-term experience with gaseous emissions from marine diesel engines shows that once the engine is warmed up these emissions are constant. Furthermore the measurements presented here showed that during the 45 minutes time for each operating point the particle emissions were very stable. Also based upon this long-term experience the Technical Code requires a sampling time of only 3 min for representative NOX sampling once the engine is conditioned. All this justifies the assumption that the particulate emission measurement will also be repeatable.
shows the measurement set-up that contained basically two units. The gravimetric/coulometric unit (right side in ) was used to take filter samples in order to analyze them gravimetrically and chemically.
FIG. 2 Experimental setup: right unit for taking filter samples, left unit for measuring the particle size distribution. The left-most path is necessary to assure the correct flow rate for isokinetic sampling (MFC: mass flow controller).
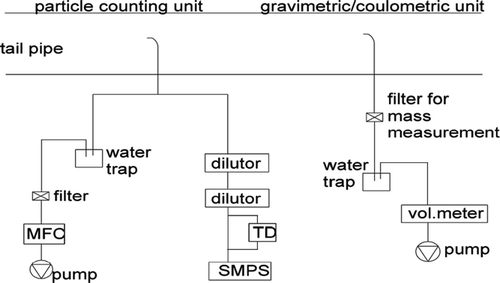
Two different types of filters were used for coulometric analysis and extraction analysis. Gravimetric analysis has been performed with both types. Quartz fiber filters were used to sample particles for coulometric analysis to determine the content of elemental carbon (EC) and organic carbon (OC). The blank filters were preconditioned by exposure to 550°C for six hours. In a first step the sample filter is heated in a nitrogen flow to volatilize organic carbon, which subsequently is oxidized with copper oxide. In a second step the elemental carbon fraction is burnt in an oxygen atmosphere. Carbon dioxide formed is analyzed in the test cell of the coulomat that is filled with highly alkaline barium perchlorate solution. The carbon dioxide falls out as barium carbonate, thereby decreasing the alkalinity, which is measured. The amount of electric charge needed to titrate the alkalinity back to its initial pH value is proportional to the absorbed amount of carbon dioxide and therefore proportional to the carbon content in the sample (VDI Guideline 2465).
Teflon-coated glass fiber filters were used to sample particles for Soxhlet extraction. According to ECE standards the filters were conditioned at 20°C and 50% relative humidity for a time period of six hours before weighting the blank samples. Filters were sampled at 120°C. After sampling the filters and before processing the sample analytically the probes were stored during one hour at 50% relative humidity and 20°C. From these filters the soluble organic fraction (SOF) and the soluble inorganic fraction (SINOF) of particle matter were determined. SOF was extracted with dichlormethane and the weight of the extracted material is determined by comparing the remaining mass of the sample after evaporation of the solvent to the total particle mass. A similar procedure with water/isopropanol-mixture as solvent yielded the SINOF mass fraction. The sulfate content was determined by means of ion chromatography from the solution. The insoluble mass resulting from these extractions contains the residue material, which is usually ashes, wear metals, insoluble minerals, and additive metal oxides.
Particle size distributions were determined with a particle-counting unit (left side of ). Both sampling lines were operated isokinetically to avoid size dependent sampling efficiency. The leftmost path in was used to maintain isokinetic conditions independent of the flow through the instruments. Size distributions of particles were measured with a Scanning Mobility Particle Sizer (SMPS, CitationWang and Flagan 1990). A two-step ejector dilution pump (CitationKoch 1988) was provided to avoid condensation and to obtain concentrations adequate for the instruments.
To prevent condensation dilution air, all tubes from the tail pipe to the two measuring units and the first of the two ejector dilutors were heated. Temperatures are listed in .
TABLE 4 Temperature settings of the experiment
To further investigate the volatile fraction of the particles the exhaust was alternatively led through a TD prior to entering the SMPS; the corresponding temperatures are also shown in . In the TD the exhaust gas is first heated to evaporate all volatile material and then guided through an activated charcoal adsorber, where the vapor is absorbed and thus removed from the exhaust gas. Characterization of this TD with special regard to the possible losses occurring in the TD was presented by CitationBurtscher et al. (2001). Because this device is used for the presented experiments the processes leading to losses are shortly summarized. Basically Brownian diffusion, thermophoresis, and sedimentation lead to particle loss in the TD. Sedimentatplion dominates at large particle sizes; approximately 2.2% of the 1 μm particles are lost. Diffusion losses increase with decreasing particle size; at 20 nm about 10% of the particles are lost, while only about 1% of the 100 nm particles do not exit the TD. Close to the TD walls thermophoresis is the dominant processes leading to particle losses. Within a 1.1 mm layer close to the wall the losses amount of less than 22%, when considering that the wall temperature is higher than room temperature. These theoretical losses were shown to agree with experimental data. In the presented work the TD removes up to 90% of the total particle number concentration, which does not make the losses insignificant, but it shows that more than 60% of the particles removed cannot be ascribed to losses in the TD. Data presented is corrected for estimated losses.
Different temperature settings were applied to investigate if they had an impact on the amount of volatile material. Experiments showed that particulate measurements were independent of temperature settings.
RESULTS AND DISCUSSION
Measurements Made with Marine Diesel Oil
Number size distributions were measured at each load point. While each measurement was performed once, the 1% load point was repeated (). Stable results were obtained during the measurement time (45′). In the following averaged results are presented: , , , and show the averaged number size distributions for 25, 50, 75, and 100% load measured with and without TD. Though there are some differences, the 4 size distributions are similar. The mean particle diameter (25–40 nm) is smaller compared to that found for road vehicle engines and the number concentration is higher throughout all operation points. The accumulation mode, well known from road vehicles, seems to be completely missing or is covered.
FIG. 3 Average SMPS size distributions as measured at 1% load with (thin line and thin line with cross) and without TD (thick line and dotted line). The measurement was performed twice.
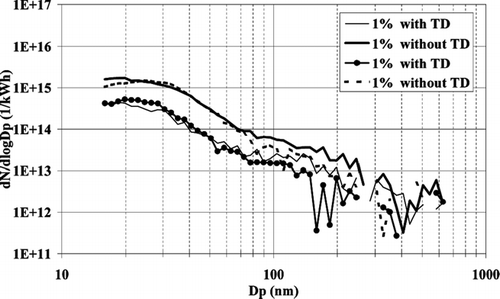
FIG. 4 Average SMPS size distributions as measured at 100% load with (thin line) and without TD (thick line).
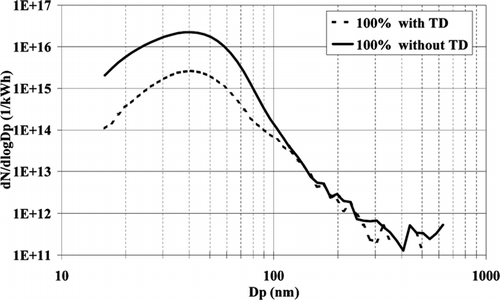
FIG. 5 Average SMPS size distributions as measured at 75% load with (thin line) and without TD (thick line).
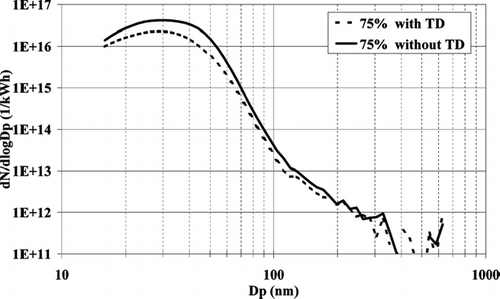
FIG. 6 Average SMPS size distributions as measured at 50% load with (thin line) and without TD (thick line).
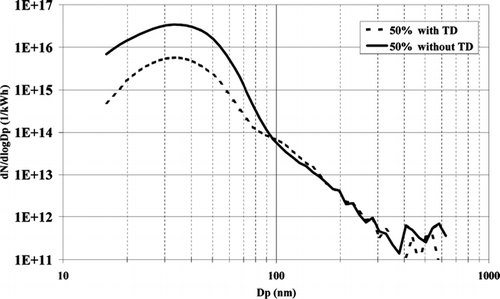
FIG. 7 Average SMPS size distributions as measured at 25% load with (thin line) and without TD (thick line).
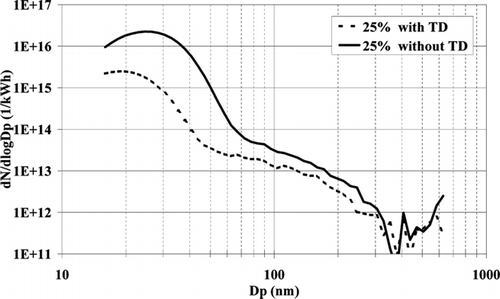
shows the total number concentrations (TNC), calculated from size distributions recorded with and without TD. For better comparison all results are displayed in the same diagram. TNC was found highest at 75% load and has a tendency to decrease with decreasing load. Despite differences in size distributions the TNC at 100% load and 25% load are quite similar. The two measurements performed at 1% load show that in terms of number concentration the measurement is reproducible. This statement is corroborated by the measurement performed with TD that does not show significant deviations between the two measurements either. Comparing results with and without TD shows that the number concentration with TD is up to an order of magnitude lower. The smallest decrease is found at 75% load, meaning that at this load point the least amount of semivolatile material is contained. Results at other load points indicate that the emitted particulate matter contains a high fraction of volatile material.
shows the mean particle diameter determined from number size distributions as measured at each operation point. Particle diameter increased over the whole load range, but is generally small and varies between 25 and 40 nm. Whereas the TD reduced TNC by up to 90% (at 25% and 100% load), it did not significantly influence the particle diameter. Nevertheless the small, but clearly observable, decrease in diameter shows that what is assumed to be the solid fraction is coated with volatile material.
FIG. 9 Particle diameter as function of engine load. Measurements were performed with and without TD.
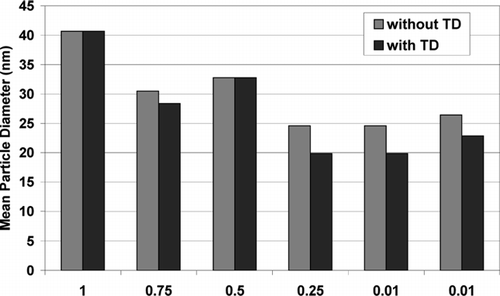
In , a size distribution of the marine diesel engine is compared to one from a passenger car. The two size distributions differ in two main characteristics:
• | The total number concentration emitted by the marine diesel engine was higher than that of the passenger car engine. | ||||
• | The typical particle size distribution emitted by this marine diesel engine shows a distinct mode with particle sizes smaller than 50 nm. The typical accumulation mode particles ranging between 50–100 nm, which are emitted from 4-stroke diesel engines are missing. |
FIG. 10 Typical number size distributions for this marine diesel engine (circles) at 100% load and a diesel passenger car (triangles) at 100% load.
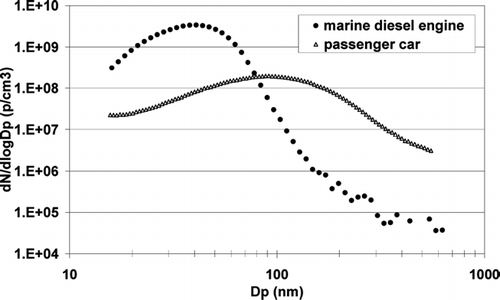
The above-mentioned features of the size distribution from the marine diesel engine were independent of the operation point. CitationLyyränen et al. (1999) Citationand (2002) reported similar results for the 4-stroke marine diesel engines. The mean diameter of the particles they found was small and increased with load (from 20–40 nm). Particles with size between 40–90 nm were attributed to nucleation of the gaseous fuel oil ash species. Also it was found that the fine mode included nearly 60% of the total particle mass concentration, a finding similar to the results presented here. According to Lyyränen this high particle mass at small diameters suggested high volatility of the diesel fuel components vanadium, sodium, calcium, nickel, aluminum, and silicon (referred to as diesel fuel ash in the paper) and moreover that the volatility might be even higher when taking into account that not all of these particles were primary particles, but some of the agglomerates consisted of primary particles formed by nucleation of gaseous ash species. In other words, these small particles result from ash components evaporating during combustion and subsequently form nucleation mode particles out of the gas phase.
displays total mass concentrations determined by gravimetric (Teflon and quartz filters) and coulometric analysis and also as calculated from measured size distributions with and without TD. Spherical particles of unit density (1 g/cm3) were assumed for calculation. In addition to results for total mass, residue mass after removing water-and organic-soluble fraction are shown.
TABLE 5 Comparison of gravimetric particle mass from filter samples and masses as calculated from number size distributions measured with and without TD. Mass unit is corrected for ambient conditions and given in mg/Nm3 (milligram/norm cubic centimeter) unless otherwise indicated
The correspondence between results from quartz and Teflon filters is good and shows a maximum deviation of 18.5%. Masses from gravimetric and coulometric measurement do not agree very well. Generally these results must be looked at carefully because the sampling is different and it has an impact on the fraction of volatile material captured on the filter sample.
Summarizing the result shows that the mass as calculated from SMPS number size distributions measured without TD yielded higher values than the mass determined from filter samples although to the SMPS only a restricted size range is available, which is not the case for filters. One explanation might be the volatilization of material off of the filter during PM collection. Also the sampling differed for every applied method and influenced the outcome. A high uncertainty lies within the calculation that assumes unit density for the particles, which is justified for droplet-like structures, but not for soot particles, which are known to have fractal densities that decrease with increasing particle size (CitationBurtscher 2005).
shows results from Soxhlet extraction (SOF—soluble organic fraction, WSF—water soluble fraction, sulfates, and the residue) and coulometry (OC—organic carbon and EC—elemental carbon) from the filter samples, which were normalized to engine power. Dominant fractions were the organic carbons. The OC stems from sampling Quartz filters and the SOF from Teflon filter samples. WSF had no major contribution to the total mass.
FIG. 11 Summary of results from coulometric analysis and extraction of organic material (OC, organic fraction; EC, elemental carbon; SOF, soluble organic fraction; WSF, water soluble fraction).
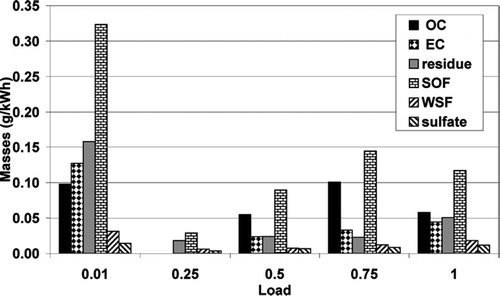
Although the SOF was highest at 1% load and 75% load, the TD removed only 77% of particle number concentration at 1% load and even less, 47% at 75% load, which means that the SOF contains material that does not evaporate at temperatures up to 350°C. This may also be the reason for the large difference between SOF and OC, which can only be explained by material, which is extracted by Soxhlet procedure, but not evaporated during coulometry. Volume-based concentrations are lowest at 1% load. The high values result from the normalization to engine power.
From the measured sulfate concentrations the sulfate/SO2 ratio was determined. Results are calculated at 100% load. Engine specific fuel consumption was approximately 180 g/kWh and the sulfur content for MDO used was 0.155% of mass, resulting in 279 mg/kWh of emitted sulfur. The estimated lube oil consumption was typically 1 g/kWh with a sulfur content of 0.5%, which yields additional 5 mg/kWh. Total sulfur emissions sum up to 284 mg/kWh.
The measured sulfate concentration of 12 mg/kWh corresponds to a sulfur concentration of 4 mg/kWh indicating that 1.4% of the fuel sulfur is converted to sulfate; the rest is probably emitted as SO2.
Comparison of PM Emission When Running the Engine with Marine Diesel Oil and Heavy Fuel Oil
The influence of the two fuels used (MDO and HFO) has been investigated at: 1% load and full load.
displays the TNC as calculated from size distributions and the corresponding particle diameter at 1% load and at both fuel qualities. As it had to be expected, the emissions are higher for the low-quality fuel HFO. The number concentration and the mean diameter are higher. This is a consequence of the significantely higher contents of asphaltenes, Nickel, Vanadium, and Iron in HFO when compared to MDO (see also ). CitationLyyränen et al. (1999) suggest that particles formed in the exhaust gas contain a lot of the impurities from the fuel oil.
FIG. 12 (a) Total number concentrations as calculated from number size distributions and (b) mean particle diameter as measured at 1% load with both fuels.
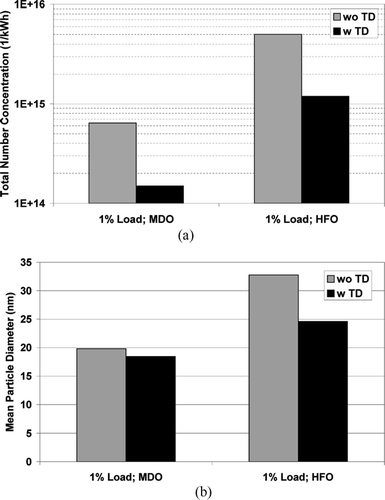
The graphs in display absolute masses and cumulative mass fractions resulting from chemical analyses of the sampled filters. As expected the mass emitted was higher when operating the engine on HFO. All mass fractions increased, but especially the one attributed to the organic soluble fraction (76%). The residue fraction increased about 51%. This result reflected the strong increase in particle number concentration.
At 100% load (), the trend is just opposite; number concentration and mean diameter become considerably smaller when running the engine on HFO. While sampling the exhaust gas through the TD, the TNC decreases about 86%, but the effect of the TD on the mean particle diameter lies within the range of error (2 nm). The smaller amount of particles might be influenced by the high content of Iron in the HFO, which acts as an additive. Matter and Siegmann (CitationMatter et al. 1997) demonstrated that seeding a commercially available diesel fuel with ferrocene (Fe(C5H5)2, 120 mg per kg fuel) reduced the number of carbonaceous particles emitted by heavy-duty construction engines about 50%. In a laboratory experiment, CitationKasper et al. (1999) showed that when seeding an acetylene/argon flame with ferrocene vapor, the carbonaceous particles are almost completely removed above the seeded flame due to the catalyzing character of the ferrocene particles.
FIG. 14 (a) Total number concentrations as calculated from number size distributions and (b) mean particle diameter as measured at 100% load with both fuels.
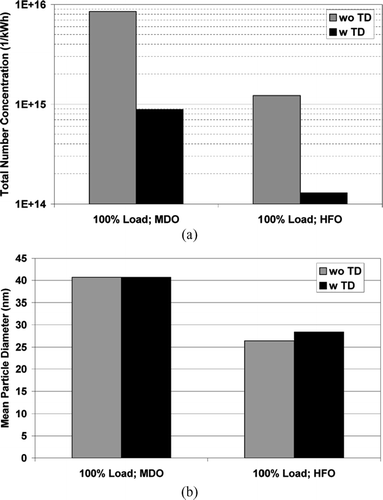
displays the absolute masses as extracted from filters sampled at 100% load with both fuels. These measurements were in line with the expectations, namely fuelling the engine with HFO yielded higher emitted masses. Here too, all mass fractions increased, but especially the one attributed to the organic soluble fraction (71%).
Comparing the emissions at 100% and 1% load one observes the generally smaller particle mass emitted at full load. While particles emitted at 100% (with MDO) are larger than their counterparts measured at 1% load, it is just the opposite when fuelling the engine with HFO. This also mirrors in the total number concentrations of emitted particles. What seems like a contradiction when comparing masses suggests that enough large particles were emitted that were out of range for the SMPS to measure, and as such contribute significantly to the mass but not to total number concentration.
CONCLUSIONS
Independent of load a high amount of small particles was observed with diameters ranging between 20–40 nm. Size distributions did not exhibit a pronounced accumulation mode like that known from diesel passenger cars. Most of the particles consisted of volatile material. The TD removed up to 90% of the particle number concentration and 80% of the particle mass concentration. Mean particle size increased with load, but it did not change when applying the TD. The formation of nuclei particles was enhanced by the sulfur content of the fuel. Chemical analyses yielded a high amount of organic soluble material, whereas the water-soluble fraction was much lower. Only 1.4% of fuel sulfur was converted into sulfates.
The emission of generally smaller solid particles than the ones known from the fast running diesel passenger cars was likely to be a consequence of slow engine speed of maximum 105 rpm and the large piston displacement (2.4 m). Though the two fuels differed significantly in quality the chemical analyses the filter samples showed no very significant differences in mass fractions. The soluble fractions were subject to the largest variations and dominated in both cases the mass.
This study on particle emissions from a 2-stroke low-speed marine diesel engine shows significant differences to the results known from particle emission studies on 4-stroke on-road diesel engines but also some common features with particulate emissions from 4-stroke medium-speed marine diesel engines. The presented experiments yielded a lot of information about particle measurement and issues one might run into when dealing with large 2-stroke engines. In future more detailed investigations are necessary to not just being able to characterize the emissions of this kind of engine but also come to a better understanding of the results of this study.
Acknowledgments
The Swiss Commission for Technology and Innovation (KTI) supported this program.
REFERENCES
- 1998 . Regulations for the Prevention of Air Pollution from Ships and NOx Technical Code Annex VI of MARPOL 73/78, Latest Revision 2004. IMO ISBN 92-801-6089-3. http://www.imo.org/home.asporhttp://www.dieselnet.com/standards/inter/imo.php
- Brown , D. M. , Wilson , M. R. , MacNee , W. , Stone , V. and Donaldson , K. 2001 . Size-Dependent Proinflammatory Effects of Ultrafine Polystyrene Particles: A Role for Surface Area and Oxidative Stress in the Enhanced Activity of Ultrafines . Toxicol. Appl. Pharmacol. , 175 : 191 – 199 .
- Burtscher , H. , Baltensperger , U. , Bukowiecki , N. , Cohn , P. , Hüglin , C. , Mohr , M. , Matter , M. , Nyeki , S. , Schmatloch , V. , Streit , N. and Weingartner , E. 2001 . Separation of volatile and non-volatile aerosol fractions by thermodesorption: instrumental development and applications . J. Aerosol Sci. , 32 : 427 – 442 .
- Burtscher , H. 2005 . Physical characterization of particulate emissions from diesel engines: a review . J. Aerosol Sci. , 36 : 896 – 932 .
- Corbett , J. J. and Koehler , H. W. 2003 . Updated emissions from ocean shipping . J. Geophys. Res. , 108 ( D20 ) : 4650 doi:10.1029/2003JD003751
- Kasper , M. , Sattler , K. , Siegmann , K. , Matter , U. and Siegmann , H. C. 1999 . The Influence of Fuel Additives on the Formation of Carbon during Combustion . J. Aerosol Sci. , 30 : 217 – 225 .
- Koch , W. , Lödding , H. , Mölter , W. and Munzinger , F. 1988 . Verdünnungssystem für die Messung hochkonzentrierter Aerosole mit optischen Partikelzählern . Staub-Reinhaltung der Luft , 48 : 341 – 344 . (Dilution Systems for Measurement of Highly Concentrated Aerosols with Optical Particle Counters. Keeping the Air Particle Free)
- Lighty , J. S. , Veranth , J. M. and Sarofim , A. F. 2000 . Combustion Aerosols, Factors Governing Their Size and Composition and Implications to Human Health . J. Air & Waste Management Assoc. , 50 ( 9 ) : 1565 – 618 .
- Lyyränen , J. , Jokiniemi , J. , Kauppinen , E. I. and Joutsensaari , J. 1999 . Aerosol Characterisation in Medium-Speed Diesel Engines Operating with Heavy Fuel Oils . J. Aerosol Sci. , 30 : 771 – 784 .
- Lyyränen , J. , Jokiniemi , J. and Kauppinen , E. I. 2002 . The Effect of Mg-based Additive on Aerosol Characteristics in Medium-Speed Diesel Engines Operating with Residual Fuel Oils . J. Aerosol Sci. , 33 : 967 – 981 .
- Matter , U. , Siegmann , H. C. and Burtscher , H. 1999 . Dynamic Field Measurement of Submicron Particles from Diesel Engines . Environ. Sci. Technol. , 33 ( 11 ) : 1946 – 1952 .
- Matter , U. and Siegmann , K. 1997 . The Influence of Particle Filter and Fuel Additives on Turbo Diesel Engine Exhaust . J. Aerosol Sci. , 28 ( 1 ) : 51 – 52 .
- Oberdörster , G. , Sharp , Z. , Atudorei , V. , Elder , A. , Gelein , R. , Dreyling , W. and Cox , C. 2004 . Translocation of Inhaled Ultrafine Particles to the Brain . Inhalation Toxicology , 16 : 437 – 445 .
- 1999–2005 . Measurement of soot (Ambient Air)—Thermographical determination of elemental carbon after thermal desorption of organic carbon, VDI/DIN-Reinhaltung der Luft , Berlin : Beuth Verlag . VDI Guideline 2465-2, http://www.vdi.de/vdi/english/vrp/richtlinien/search/10683/index.php
- Wang , S. C. and Flagan , R. C. 1990 . Scanning Electrical Mobility Spectrometer . Aerosol Sci. Technol. , 13 : 230 – 240 .