Abstract
As part of the California Regional PM 2.5 and PM 10 Air Quality Study (CRPAQS) particle size distributions were measured simultaneously at two sites; the city of Fresno and the agricultural site of Angiola. Reported here are data obtained by scanning mobility analysis over the size range from 10 nm to 400 nm for the intensive study period from December 1, 2000 through February 6, 2001. These high time resolution data show variability in the character of the distributions, as well as the in the total number concentrations. The most pronounced feature of the data set is a consistent, nighttime maxima in particle number concentrations with a modal diameter near 80 nm during the evening hours at the urban Fresno site. Although these maxima are correlated with CO, NO, and black carbon, the particle size is larger than the 30–40 nm modal diameters observed for traffic aerosols during the commute hours, and is attributed to a non-vehicle source. At the agricultural site, the morning maxima particle number concentration coincides with the maxima in NO concentration, but often precedes the morning maxima in black carbon. Values for the geometric mean particle diameter varied from day to day, but are correlated between the two sites, with somewhat larger particle sizes at Angiola during periods of stagnation.
INTRODUCTION
Recent laboratory and epidemiological studies have shown that “ultrafine” particles, loosely defined as those with diameters below 100 nm, are related to adverse health effects (CitationOberdorster et al. 1992, Citation1995; CitationDonaldson and MacNee 1998; CitationWichmann and Peters 2000). In the 1970s, Whitby showed that the concentrations of these particles, then referred to as “nuclei mode” are elevated along freeways, as a result of direct emission from combustion sources (CitationWhitby 1978; CitationWhitby and Sverdrop 1980). More recent studies have examined the dynamics of these size distributions near freeways, and have shown that the ultrafine particles persist downwind, with little change in size distribution (CitationZhang and Wexler 2004; CitationZhang et al. 2004), and can envelop nearby buildings close to a freeway (CitationMorawska et al. 1999).
Several recent air quality studies have included monitoring of particle size distributions over extended periods. Campaigns in the cities of Atlanta (CitationWoo et al. 2001), Pittsburgh (CitationStanier et al. 2004), Houston (CitationGasparini et al. 2004), Los Angeles (CitationKim et al. 2002), Fresno (CitationWatson et al. 2002, Citation2005), and several European cities including Erfurt and Leipzig, Germany (CitationTuch et al. 2003; CitationBirmili et al. 2001), Helsinki, Finland (CitationLaasko et al. 2003), and Zurich, Switzerland (CitationBukowiecki et al. 2003) provide data for periods of one year or more. The European studies include direct comparison among urban, near-city, rural, and background sites (Citationvan Dingenen et al. 2004), as well as curb-side or street canyon and urban locations (CitationWehner et al. 2002; CitationLongley et al. 2003). These studies have shown that not only are ultrafine particles associated with vehicular traffic, but even in urban areas may result from “nucleation events” with bursts of new particle formation in the 3 to 10 nm size range that may grow into larger sizes. CitationRuuskanen (2001) compares simultaneous measurements among three European cities during a three-month winter period, and found that while particle mass concentrations varied, the particle number concentrations among the cities were similar, and uncorrelated with PM2.5 mass.
In this paper we present simultaneous measurements of dry particle size distributions in an urban location and at an agricultural site in California's central valley region, with comparison to profiles for NO, O3, black carbon and nitrate. Measurements were made as part of the California Regional PM2.5 and PM10 Air Quality Study (CRPAQS) that was conducted to understand the character, sources, and regional nature of the particles associated with high, wintertime particulate concentrations found within California's San Joaquin Valley (CitationMagliano et al. 1999; CitationWatson and Chow 2002).
EXPERIMENTAL
The size distributions of atmospheric particles at ambient dew point were measured over the diameter size range from 10 nm to 400 nm at two sites in the San Joaquin Valley, as shown in . Urban measurements were made in the city of Fresno, at the Air Resources Board monitoring site located on First St, 4.6 km NE of the downtown area (N 36° 46′ 54″, W 119° 46′ 24″). The monitoring station was housed on the second floor of a two-story office building situated in a mixed use commercial and residential neighborhood, and is bordered by major thoroughfares 10 m to the east and 90 m to the south. Freeways lie 1 km to the west, 2 km to the east and 3 km to the south, with average daily traffic loads of 120000, 60000 and 80000 vehicles, respectively (CitationCa. Dept. Transportation 2001). In 2000 the city had a population of 430,000. Measurements indicative of an agricultural area were made at the CRAPQS Angiola site located in a farming area 98 km southwest of Fresno, and 87 km NW of the city of Bakersfield (N 35° 56′ 53″, W 119° 32′ 16″). The site was surrounded by open fields planted in cotton and wheat, with the exception of the area within 100 m which was not cultivated. The nearest traffic is from a one-lane, dirt-and gravel-surfaced farm road. The town of Corcoran, with 9500 inhabitants is located 15 km to the north. The Interstate freeway runs 30 km west of the site, and the state highway lies 20 km to the east, each carrying approximately 30000 vehicles per day. This site meets the criteria of “rural” proposed by the European Environmental Agency, as described by Larssen et al. (1999). Reported here are measurements conducted as part of the CRPAQS, during the period from December 1, 2000 to February 6, 2001.
FIG. 1 Location of the Fresno, California and Angiola monitoring sites (indicated by stars) with respect to the Interstate Freeway 5 and US Highway 99, and the neighboring cities.
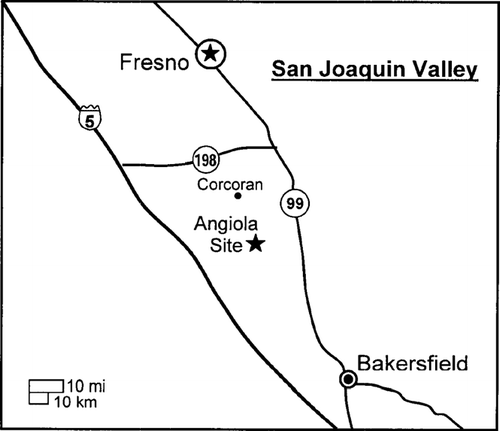
Particle size distributions were measured using Scanning Mobility Particle Sizer (SMPS, TSI Model 3936L10) equipped with the “long” mobility column (TSI Model 3081) and a TSI Model 3010 butanol-based condensation particle counter (CPC). This CPC operates at an aerosol flow rate of 1 L/min, and has a lower cutpoint of 10 nm. The SMPS was operated with a 120-s upscan, followed by a 30-s downscan. One size distribution is reported every five minutes, consisting of an average of data from the two upscans. The data are reduced at the time of collection, and reported with a size resolution of 32 channels per decade of particle diameter. The relative humidity at the point of measurement was evaluated for each hour of the study using measurements of the outdoor dew point and SMPS sheath flow temperature. At both sites aerosol was sampled through a 16.7 L/min PM10 inlet, mounted 2 m above rooftop, from which a 1 L/min isokinetic sample was extracted for the SMPS measurements. The point of extraction was inside the room, approximately 4 m below the PM10 inlet, and 2 m from the SMPS inlet. Transport lines were either metal or conductive flexible tubing. Calculated diffusional losses are 12% at 15 nm.
At the time of this study, the commercial software provided by TSI to control the SMPS did not have the capability for continuous monitoring. The number of consecutive samples was limited to 999 (or 3.5 days for 5-min sampling); system parameters such as flows, pressures and temperatures were not recorded; and there was no serial output for externally logging by the site data acquisition system. Thus, a QuickBasic program (SMPSDAT) was written that operated alongside the TSI software. Overall control of the SMPS system, including voltage scanning and logging of the raw particle counts, was handled by the TSI program. For each 5-minute size distribution the TSI software created a new data file containing the raw count data from the CPC. The companion SMPSDAT program looked for these new files. When found, SMPSDAT would read the system parameters (temperatures, pressures, flows, and flags), process the count data using the current operating conditions, and send reduced size distribution data and system parameters to the site data acquisition computer and to a locally stored file. Additionally, SMPSDAT would move, rename and compress the files of raw tenth-second count data from the TSI software into a monthly archived file.
SMPSDAT calculated size distributions using the theoretical relationships to convert from scan time to particle electric mobility to particle diameter, as described by CitationWang and Flagan (1990). Volumetric flow rates were corrected for the pressure drop across the precut impactor at the SMPS inlet. Particle mobility calculations were done at the measured sheath flow temperature and pressure. Size-dependent aerosol charge fractions and size-dependent CPC detection efficiencies were based on Wiedensohler et al. (Citation1988, Citation1997), as provided by TSI. Data were reduced using 64 channels per decade, and then regrouped into 32 channels per decade to provide a data set with same particle size boundaries for all distributions, regardless of shifts in sheath flow, pressure or temperature which affect the voltage–particle size relationship.
During the study the SMPS system was serviced every third day, including resetting of the TSI software, zeroing of the pressure drop transducers, and cleaning and greasing of the inlet impactor collection plate. At less frequent intervals the impactor orifice was also cleaned. On an approximate weekly basis or more frequently the butanol reservoir in the CPC was refilled. Monthly scheduled maintenance included flows checks of the DMA sheath and CPC sample flows using a bubble flow meter (Gilibrator) placed in-line. Dynamic zero concentration checks with an inlet filter and particle size calibration checks with monodisperse polystyrene latex (PSL) spheres were performed periodically (roughly once a month) on the SMPS.
All data were post-processed based on quality assurance review and data flags. The system was configured such that the sheath and bypass flows were operated in series, and both flows were recorded by SMPSDAT software. In the quality assurance review the flow readings for these two sensors were compared. At the Angiola site there were frequent discrepancies between these two readings which were traced to improper zeroing of the sheath flow transducer. These data were post-processed based on readings from the bypass flow sensor, with validation based on review of the sizing of the PSL standards. In the post-processing, all of the data were corrected for multiple-charging.
AVERAGE SIZE DISTRIBUTIONS
Mean particle size distributions from the Fresno and Angiola sites are presented in . Shown are averages over 30 “episode” days during the study period, taken to be those for which the 24-hr averaged PM2.5 mass at the Fresno site exceeded 40 μg/m3. These dates were December 22–January 7, January 15–22, January 31–February 6. Size distributions are shown for weekdays (27 days), and for weekends and holidays (9 days), at four different times of the day: 7:00–8:00, 12:00–13:00, 17:00–18:00, and 21:00–22:00 PST. The average over weekends and holidays include Saturdays and Sundays, as well as the holidays of December 25, January 1 and January 15, each of which fell on a Monday.
FIG. 2 Average at four times of day of the distribution of particle number concentration with respect to size at Fresno and Angiola for 17 weekdays (top), and 13 weekends and holidays (bottom) during periods of high particulate concentrations.
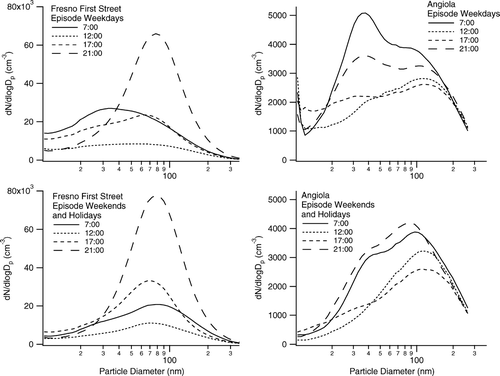
Notable is the contrast between the two sites. At Fresno the maximum particle number concentrations occurred in the evening hours, with a single mode peaking at 80 nm. This feature is present on both weekdays and weekends, as illustrated by the distribution from 21:00 to 22:00 P.M. The size distribution measured during the weekday morning rush hour, from 7:00–8:00 A.M., is characterized by smaller particle diameters and higher total number concentration than for the same period during the weekends and holidays. However, this difference is less pronounced than at the agricultural site of Angiola.
Important to the interpretation of the diurnal variation in the size distribution is that the relative humidity at the point of measurement was consistently low, varying from 15% to 40% throughout the entire study period, with a mean value of 27 ± 5%RH. In addition, the measurement relative humidity did not follow a consistent diurnal pattern. This is because the temperature of the SMPS sheath flow was nearly constant, and thus the relative humidity value dependended primarlily on dew point, which varies slowly. Specifically, the average of the SMPS relative humidity over the hours from 4 P.M.–8 P.M. was 26%, and the average over the hours from 8 P.M. until midnight was also 26%. Thus the larger particle size observed during the evening hours is not a direct result of relative humidity induced hygroscopic growth.
Angiola exhibits a much stronger difference between weekdays and weekends and holidays than does the Fresno site. The maximum particle number concentrations at Angiola are observed in the morning hours, rather than in the evening. The trace for 7:00–8:00 A.M. exhibits two modes, with modal maxima at 35 nm and 100 nm. The 35 nm mode is more pronounced on weekdays, while that at 100 nm, is more pronounced on weekends and holidays. Perhaps surprisingly, this result indicates a larger relative importance of weekday morning activities in the farming area of Angiola than in the city of Fresno. The overall lower traffic densities, and the absence of non-workday residential activity, contribute to the sharper weekday/weekend contrast observed here.
The mean value in particle number concentrations for the source-rich urban area of Fresno was 20,200 ± 14,700, as compared to 3,650 ± 1,840 at the agricultural Angiola site, for this two-month winter period. The value for Fresno is comparable for that reported for wintertime in the European cities of Alkamar, Erfurt, and Helsinki, with mean number concentrations over the particle diameter range between 10 nm and 100 nm of 16,200, 17,700, and 18,300 cm−3, respectively (CitationRuuskanen et al. 2001; CitationLaakso 2003), and to the winter averages of approximately 20,000 cm−3 for London, Leipzig and Milano (Citationvan Dingen et al. 2004). It is also similar to annual averaged values reported for the city of Atlanta, Georgia (21,400 ± 1200 cm− 3, CitationWoo et al. 2001) and the city of Pittsburgh, Pennsylvania (14,300 cm−3, CitationStanier et al. 2004). Those values at Angiola are somewhat lower than the value of 6,500 cm−3 reported by Stanier for a rural site 38 km downwind of Pittsburgh.
compares the 24-h running average of the number-weighted geometric mean diameter, D gN , obtained from the SMPS size distributions at Fresno and Angiola throughout the two-month study period (December 1, 2000 through February 6, 2001). Periods of elevated particulate levels are indicated through the plot of running, 24-h average nitrate concentration. During periods of elevated concentration, this graph clearly shows larger geometric mean diameter at Angiola than at Fresno. The relative humidity at the point of measurement was low, and approximately the same at both sites, as noted above, and thus this does not account for the observed difference in particle sizes.
FIG. 3 Comparison of the running, 24-h average of the number-weighted geometric mean diameter (D gN ) at Fresno (solid line) and Angiola (dotted line) during the study period. The lower portion of the graph shows concurrent, 24-h running average of the particulate nitrate concentrations for the two sites.
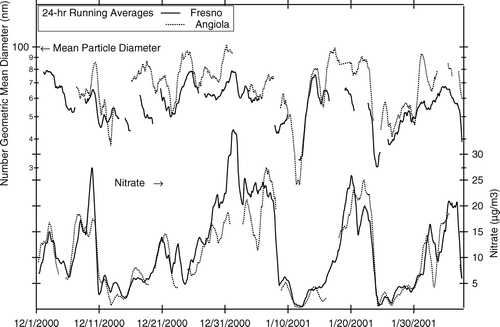
Statistics for the two sites are compared in . Median, quartile (75th percentile) and 90th percentile values are given for the number concentration from 10 to 400 nm, and the number-weighted geometric mean diameter, D gN . The median particle number concentrations are slightly lower than the mean values quoted above, due to the log-normal nature of the frequency distribution. The median particle size at Fresno is smaller than at Angiola, with a median value of D gN of 55 nm, and upper quartile value (25% of the values greater than) 69 nm. The corresponding median and upper quartile values of the D gN at Angiola are 68 nm and 84 nm, or consistently 25% greater than at Fresno. Hourly averaged values of D gN exceeded 100 nm—10% of the study period at Angiola—as compared to 0.1% at Fresno.
TABLE 1 Summary Statistics for Particle Size Distributions measured from December 1, 2000 through February 6, 2001
DIURNAL PROFILES
and show the diurnal patterns for the period from December 16 through February 7, for days when the 24-h average PM2.5 mass at the Fresno site exceeded 40 μg/m3. The daily pattern in the particle number concentration is displayed as an “hourly residual,” defined as the difference between the measured concentration and the 24-h average concentration centered on that hour. This approach allows us to more easily distinguish the diurnal pattern from the more slowly varying, multiday changes in concentration that result from the meteorologically driven periods of stagnation. The particle diameter, which is an intensive parameter, is plotted directly. All values are plotted as a function of the time of day, with separate traces for each day. The weekdays (Monday through Friday) are distinguished from the weekends and holidays as described above. For this study period both Christmas Eve and New Year's Eve fell on a Sunday, and thus were automatically grouped with the weekend-holiday set. Traces for Christmas and New Year's Day are shown by the solid symbols.
FIG. 4 Traces for 17 individual weekdays and 13 weekend/holidays of the residual in the particle number concentration and the geometric mean diameter at Fresno, where the residual is defined as the difference from the 24-h running average. Christmas Day is shown by the solid circle, and New Years Day is shown by the solid triangle.
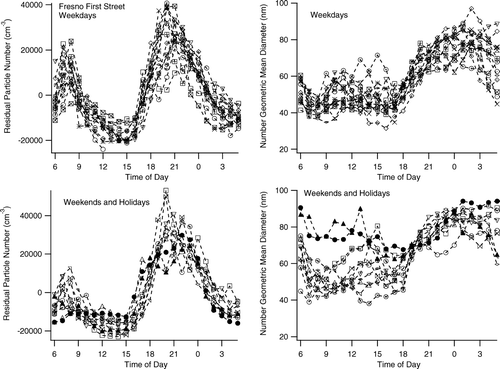
FIG. 5 Traces for 17 individual weekdays and 13 weekend/holidays of the residual in the particle number concentration and the geometric mean diameter at Angiola, where the residual is defined as the difference from the 24-h running average. Christmas day is shown by the solid circle, and New Years Day is shown by the solid triangle.
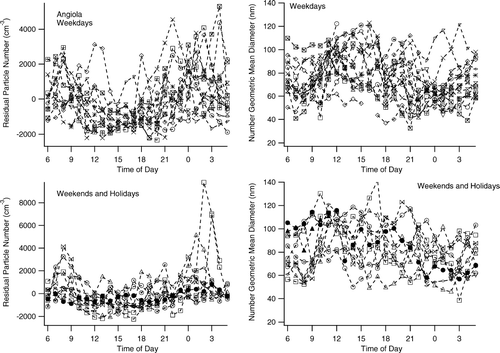
The data for Fresno show a very distinct pattern in the total number concentration and geometric mean diameter for both weekdays and weekends. Pronounced are the evening maxima in number concentration, which are observed between 20:00 and 22:00 on most days. These evening particle size distributions are characterized by an increase in the geometric mean diameter. Daytime size distributions were more variable than at night. A second, weaker maxima is seen at 7:00 to 8:00 in the mornings, coincident with a decrease in the geometric mean particle diameter. A secondary minimum is observed between 16:00 and 18:00 on some, but not all weekdays. This “rush hour” pattern is more prominent on weekdays than weekends, and is missing on Christmas Day (solid circle). The lowest particle number concentrations are observed midday, noon to 3 P.M., when the mixing height is higher, and the ventilation parameter, defined as the product of wind speed and mixing height, is greatest (CitationMacDonald et al. 2006).
The Angiola site does not exhibit the strong diurnal pattern observed at Fresno. The most consistent feature is a slight increase in the mean diameter between 9:00 and noon, coincident with the increase in the mixing heights. This feature is also seen in the Fresno data. Notable is the absence at Angiola of the consistent evening increase in particle concentrations and geometric mean diameter observed at Fresno.
The trends in particle number concentration observed for Fresno are in direct contrast to that reported for Pittsburgh, where the largest particle number concentrations are seen midday (CitationStanier et al. 2004). This was most pronounced for particles between 3 and 10 nm in diameter, but was also observed for particles in the size range from 10 to 50 nm. At Pittsburgh, Stanier et al. report that particle nucleation events were observed on 30–50% of the days, typically occurring in the morning hours. These particle nucleation events are characterized by a burst in small particle number concentration forming a mode that increases in diameter over a period of several hours, and contributed to the midday peak in particle number concentrations.
CitationWoo et al. (2001) observed particle nucleation events in Atlanta, with maxima in the concentration of 3–10 nm particles occurring midday. Unlike the Pittsburgh measurements, the observed Atlanta events did not lead to an increase in the midday concentration of particles above 10 nm in diameter. In Atlanta the average of particle number concentrations over each hour for the one-year study period showed afternoon minima for particles between 10 to 200 nm, much as observed here.
COMPARISON WITH OTHER CONSTITUENTS
shows a contour plot of the size distributions at Fresno over the period from December 21 to January 6. The high particle concentration events, occur consistently in the evening hours, ending at midnight except on New Year's Eve, where the high concentrations extend into the early morning hours of January 1. The mean particle size during these events is between 80 to 90 nm. These events are coincident with increases in NO, CO, and black carbon, as shown by the overlay plot.
FIG. 6 Contour plot or particle number concentration (dN/dlogDp) at Fresno, overlayed with concentrations of black carbon (BC), NO, and CO.
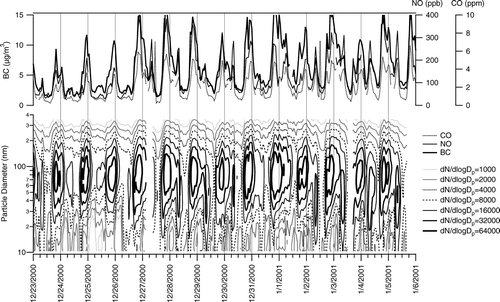
On December 28–30 (Thursday through Saturday) and again on January 2 through 5 (Tuesday through Friday) the Fresno data exhibit a secondary maxima in particle concentration, NO, CO, and black carbon appearing between 7:00 and 8:00 PST, associated with the morning traffic. The morning rush hour traffic occurs prior to the lifting of the nighttime inversion, under conditions of low wind speed (mean value of 0.8 m/s). The evening rush hour is not readily discernable, in part because it is more spread in time, and because the average wind speeds are higher (mean value of 1.3 m/s). The dominance of the morning rush hour traffic peak over that in the afternoon is noted by CitationWehner et al. (2002), who attributed this in part to the lower proportion of truck traffic during the afternoon rush hour.
The coincidence of high particle number concentrations and high CO, NO, and black carbon (BC) concentrations is seen in the average profile plot of , where data from each hour of the day for all episode periods have been averaged together. To more clearly show diurnal behavior, these patterns are calculated by averaging the difference between the measured value and the running 24-h average (i.e., average over residuals such as shown in and ). Black carbon and particle number concentrations correlate throughout the day. The NO and CO is higher with respect BC and particle number concentration in the morning than in the evening.
FIG. 7 Average profiles over 30 episode days during the winter study period of the particle number concentration, black carbon (BC), NO, CO, particle geometric mean diameter (D gN ), nitrate, and ozone at Fresno and Angiola.
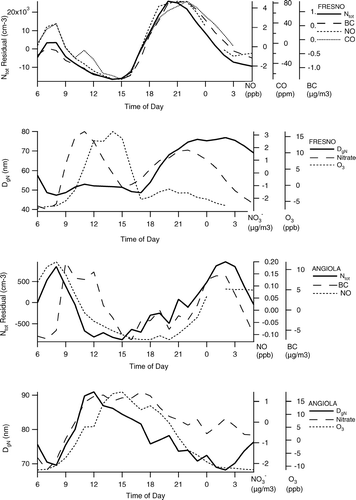
Unlike the evening maxima, the morning secondary maxima are characterized by smaller particle diameters. shows that the geometric mean particle diameter reaches a minimum of 40–50 nm in the morning, and again in the evening, between 16:00 and 18:00 PST. The observation of traffic-related aerosols has been reported by many investigators, and are characterized by the smaller sizes seen here for the smaller morning peak. Modal diameters for traffic-related particulate emissions above 10 nm are in the range of 20–60 nm (CitationKittelson et al. 2004; CitationZhang et al. 2004, 2005; CitationZhu et al. 2002; CitationMorawska et al. 1999; CitationWehner et al. 2002). These morning distributions at Fresno are typical of the size distributions observed in traffic-dominated urban areas, such as have been reported for Manchester, England (CitationLongley et al. 2003); Zurich, Switzerland (CitationBukowiecki et al. 2003), and Leipzig and Ehrfurt, Germany (CitationTuch et al. 2003). However, in contrast to these cities, the maximum particle number concentrations reach their maximum in the evening, and correspond to much larger geometric mean diameters, approaching 80 nm.
The larger evening particle sizes observed in Fresno cannot be explained by coagulation. CitationZhang and Wexler (2004) have shown that the particle size does not change by coagulation, and that the characteristic size of the vehicular emissions is stable within a few seconds after exiting the tail pipe. Modeling by CitationWehner (2003) shows that between a street canyon and the urban area, coagulation leads to an increase in modal diameters to only 30 nm. Similarly, diurnal patterns in relative humidity do not account for the size change observed. The mean value of the ambient relative humidity was higher in the evening than in the late afternoon (with mean values of 76% and 55% for the evening and afternoon respectively over the December 22–January 7 time period), but the relative humidity at the point of measurement was equally low for both periods (26%). Even allowing for hysteresis and efflorvescence in the loss of water associated with the aerosol, the factor of 1.6 change in particle diameter is too large to be attributed to ambient relative humidity changes. For these reasons we conclude that the 80-nm mode particle size distribution observed in the evening hours does not arise from vehicular emissions.
CitationWatson and Chow (2002) report elevated concentrations of black carbon during winter evening hours at Fresno, and suggest that this may arise from residential heating and wood combustion. Recent organic speciation of fine particles in Fresno show high values of levoglucosan, a marker for wood combustion (CitationRinehart et al. 2006), similar to that reported earlier for the city of Bakersfield, located to the south of Fresno in the San Joaquin Valley (CitationSchauer and Cass 2000). Further, the evening size distribution data are consistent with the hypothesis of wood burning. CitationHildemann et al. (1991) report that residential fireplace wood combustion and industrial boiler fuel oil combustion produce relatively fewer particles in the 20 nm to 40 nm size range than do catalyst-equipped vehicles. Most other studies in urban areas have found the largest particle number concentrations are associated with traffic sources, or occasional daytime particle nucleation events (CitationRuuskanen et al. 2001; CitationStanier et al. 2004; CitationWoo et al. 2001; CitationGasparini, 2004). Only CitationHarrison et al. (1999), who conducted measurements in Birmingham, England during November “bonfire” celebrations, report higher particle number concentrations in the evening, and this is associated with the holiday fires and fireworks.
Like Harrison's “bonfire” events, the Fresno data show that the largest particle number concentrations during the evening. Further, the size distribution of these particles differs from that of the morning traffic emissions, indicating that these high particle numbers are not associated with vehicular traffic. Several epidemiological and toxicological studies have linked health effects to particles below 100 nm in diameter, and have associated these ultrafine particles with vehicular traffic. It is significant that during the winter months presented here the dominant contribution to ultrafine particles is not vehicular traffic.
The average patterns for Angiola differ from those at Fresno. The evening, 80 nm modal diameter particle number concentration maxima is not consistently present. While there is a morning increase in particle number concentration correlated with NO concentrations, it is not, on average, correlated with the black carbon concentrations (). Instead, the daily increase in the black carbon concentration is coincident with the increase in nitrate, and with an increase in the geometric mean particle diameter ().
Several investigators have suggested that the morning increase in nitrate occurs when the inversion heights lift and nitrate formed aloft via nighttime mechanisms is mixed to the ground (CitationPun and Seigneur 2001; CitationWatson et al. 2002; and Lurmann et al. 2005). The midmorning increase in nitrate is seen at both sites, and slightly precedes the increase in ozone concentration. The offset between black carbon and particle number concentration, and the coincidence of the black carbon with nitrate shown in suggest that much of the midday black carbon at Angiola is not from fresh local emissions, but rather is transported within the upper mixed layer during the night and mixed to ground level along with the nitrate in the late morning.
SUMMARY
At Fresno, the particle number concentrations in the 10 to 100 nm size range are comparable to that reported for other urban areas, such as Atlanta, Pittsburgh, and the European cities of Erfurt, Leipzig, Alkmaar, and Helsinki. However, in contrast with those cities, the maximum concentrations are found during the evening hours, several hours after the evening traffic commute. Although these maxima were correlated with CO, NO, and black carbon, the evening-time geometric mean particle diameter is larger than observed for traffic aerosols during the commute hours, indicative of a non-traffic, combustion source.
At Angiola, particle concentrations were lower than at Fresno, and the morning particle size distributions show a more distinct mode at 30 nm than those at Fresno. The total particle number follows the NO concentrations, but often precedes the morning maxima in black carbon suggesting that a significant portion of the midday loadings of black carbon do not arise from local traffic at this site, and instead are consistent with nighttime transport within the upper air mass which mixes to the ground in the morning when the inversion lifts. At both sites the geometric mean particle diameter values vary from day to day, but are correlated between the two sites, with somewhat larger particle sizes at the Angiola during periods of stagnation.
Acknowledgments
This study was supported by San Joaquin Valley Air Pollution Study Agency, under the management of the California Air Resources Board, as part of the CRPAQS. The authors gratefully acknowledge the support of K. Magliano, and the input from other investigators in the study, especially D. Blumenthal, F. Lurmann, C. MacDondald, J. Watson, J. Chow, and D. Lowenthal.
REFERENCES
- Birmili , W. , Wiedensolher , A. , Heintzenber , J. and Lehmann , K. 2001 . Atmospheric Particle Number Size Distribution in Central Europe: Statistical Relations to Air Masses and Meteorology . J. Geophys. Res. , 106 ( D23 ) : 32, 005 – 32,018 .
- Bukowiecki , N. , Dommen , J. , Prevot , A. S. H. , Weingartner , E. and Baltensperger , U. 2003 . Fine and Ultrafine Particles in the Zurich (Switzerland) Area Measured with a Mobile Laboratory: An Assessment of the Seasonal and Regional Variation throughout a Year . Atmos. Chem. Phys. , 3 : 1477 – 1494 .
- California Department of Transportation . 2001 . Traffic and Vehicle Data Systems Unit http://traffic-counts.dot.ca.gov/2001all.htmAll Traffic Volumes on CSHS
- Donaldson , K. and MacNee , W. 1998 . “ The Mechanism of Lung Injury Caused by PM10 ” . In Issues in Environmental Science and Technology , Edited by: Hester , R. E. and Harrison , R. M. London : The Royal Society of Chemistry . 1998
- Gasparini , R. , Li , R. and Collins , D. R. 2004 . Integration of Size Distributions and Size-Resolved Hygroscopicity Measured during the Houston Supersite for Compositional Categorization of the Aerosol . Atmos. Environ. , 38 : 3285 – 3303 .
- Harrison , R. M. , Shi , J. P. and Jones , M. R. 1999 . Continuous Measurements of Aerosol Physical Properties in the Urban Atmosphere . Atmos. Environ. , 33 : 1037 – 1047 .
- Hildemann , L. M. , Markowski , G. R. , Jones , M. C. and Cass , G. R. 1991 . Submicrometer Aerosol Mass Distributions of Emissions from Boilers, Fireplaces, Automobiles Diesel Trucks and Meat-Cooking Operations . Aerosol Sci. Technol. , 14 : 138 – 152 .
- Kim , S. , Shen , S. , Sioutas , C. , Zhu , Y. and Hinds , W. 2002 . Size Distributions and Diurnal and Seasonal Trends of Ultrafine Particles in Source and Receptor Sites of the Los Angeles Basin . J. Air & Waste Manage. Assoc. , 52 : 297 – 307 .
- Kittleson , D. B. , Watts , W. F. and Johnson , J. P. 2004 . Nanoparticle Emissions on Minnesota Highways . Atmos. Environ. , 38 : 9 – 19 .
- Laakso , L. , Hussein , T. , Aarnio , P. , Komppula , M. , Hiltunen , V. , Viisanen , Y. and Kulmala , M. 2003 . Diurnal and Annual Characteristics of Particle Mass and Number Concentrations in Urban, Rural and Arctic Environments in Finland . Atmos. Environ. , 37 ( 19 ) : 2629 – 2641 .
- Longley , I. D. , Gallagher , M. W. , Dorsey , J. R. , Flynn , M. , Allan , J. D. , Alfarra , M. R. and Inglis , D. 2003 . A Case-Study of Aerosol (4.6 nm < Dp < 10 μ m) Number and Mass Size Distribution Measurements in a Busy Street Canyon in Manchester, UK . Atmos. Environ. , 37 : 1563 – 1571 .
- Lurmann , F. W. , Brown , S. G. , McCarthy , M. C. and Roberts , P. T. 2006 . Processes Influencing Secondary Aerosol Formation in the San Joaquin Valley during Winter . J. Air Waste Management Association. , in press
- MacDonald , C. P. , McCarthy , M. C. , Dye , T. S. , Wheeler , N. J. M. , Hafner , H. R. and Roberts , P. T. 2005 . Transport and Dispersion During Wintertime Particulate Matter Episodes in the San Joaquin Valley, California . J. Air Waste Management Association. , 56 : 961 – 976 .
- Magliano , K. L. , Hughes , V. M. , Chinkin , L. R. , Coe , D. L. , Haste , T. L. , Kumar , N. and Lurmann , F. W. 1999 . Spatial and Temporal Variations in PM10 and PM2.5 Source Contributions and Comparison to Emissions During the 1995 Integrated Monitoring Study . Atmos. Environ. , 33 : 4757 – 4773 .
- Morawska , L. , Thomas , S. , Gilbert , D. , Greenaway , C. and Rijnders , E. 1999 . A Study of the Horizontal and Vertical Profile of Submicrometer Particles in Relation to a Busy Road . Atmos. Environ. , 33 : 1261 – 1274 .
- Oberdorster , G. , Ferin , J. , Gelein , R. , Soderholdm , S. C. and Finkelstein , J. 1992 . Role of Alveolar Macrophage in Lung Injury; Studies with Ultrafine Particles . Environ. Health Perspect. , 102 : 173 – 179 .
- Oberdorster , G. , Gelein , R. M. , Ferin , J. and Weiss , B. 1995 . Association of Particulate Air Pollution and Acute Mortality: Involvement of Ultrafine Particles . Inhal. Toxicol. , 7 : 111 – 124 .
- Pun , B. K. and Seigneur , C. 2001 . Sensitivity of Particulate Matter Nitrate Formation to Precursor Emissions in the California San Joaquin Valley . Environ. Sci. Technol. , 35 : 2979 – 2987 .
- Rinehart , L. R. , Fujita , E. M. , Chow , J. C. , Magliano , K. and Zielinska , B. 2006 . Spatial Distribution of PM2.5 Associated Organic Compounds in Central California . Atmos. Environ. , 40 : 290 – 303 .
- Ruuskanen , J. , Tuch , Th. , Ten Brink , H. , Peters , A. , Khystov , A. , Mirme , A. , Kos , G. P. A. , Brunekreef , B. , Wichmann , H. E. , Buzorius , G. , Vallius , M. , Kreyling , W. G. and Pekkanen , J. 2001 . Concentrations of Ultrafine, Fine and PM2.5 Particles in Three European Cities . Atmos. Environ. , 35 : 3729 – 3738 .
- Schauer , J. and Cass , G. R. 2000 . Source Apportionment of Wintertime Gas-Phase and Particle-Phase Air Pollutants using Organic Compounds as Tracers . Environ. Sci. Technol. , 34 : 1821 – 1832 .
- Stanier , C. , Khlystov , A. and Pandis , S. N. 2004 . Ambient Aerosol Size Distributions and Number Concentrations Measured during the Pittsburgh Air Quality Study (PAQS) . Atmos. Environ. , 38 : 3275 – 3284 .
- Tuch , T. , Wehner , B. , Pitz , M. , Cyrys , J. , Heinrich , J. , Kreyling , W. G. , Wichmann , H. E. and Wiedensohler , A. 2003 . Long-Term Measurements of Size-Segregated Ambient Aerosol in Two German Cities Located 100 km Apart . Atmos. Environ. , 37 ( 33 ) : 4687 – 4700 .
- Van Dingenan , R. , Raes , F. , Putaud , J.-P. , Baltensperger , U. , Charron , A. , Facchini , M.-C. , Decesari , S. , Fuzzi , S. , Gehrig , R. , Hansson , H.-C. , Harrison , R. M. , Cristoph , H. , Jones , A. M. , Laj , P. , Lorbeer , G. , Maenhaut , W. , Palmgren , F. , Querol , X. , Rodriguez , S. , Schneider , J. , Ten Brink , H. , Tunved , P. , Torseth , K. , Wehnen , B. , Weingartner , E. , Wiedensohler , A. and Wahlin , P. 2004 . “ A European Aerosol Phenomenology—1: Physical Characteristics of Particulate Matter at Kerbside, Urban, Rural and Background Sites in Europe ” . In Atmospheric Environment 2561 – 2577 .
- Wang , S. C. and Flagan , R. C. 1990 . Scanning Electrical Mobility Spectrometer . Aerosol Sci. Technol. , 13 : 230 – 240 .
- Watson , J. G. L. and Chow , J. C. 2002 . A Wintertime PM2.5 Episode at the Fresno, CA, Supersite . Atmos. Environ. , 36 : 465 – 475 .
- Watson , J. G. , Chow , J. C. , Lowenthal , D. L. , Kreisberg , N. M. , Hering , S. V. and Stolzenburg , M. R. 2005 . Variations of Nanoparticle Concentrations at the Fresno Supersite . Science of the Total Environment , 358 : 178 – 187 .
- Watson , J. G. , Chow , J. C. , Lowenthal , D. H. , Stolzenburg , M. R. , Kreisberg , N. M. and Hering , S. V. 2002 . Particle Size Relationships at the Fresno Supersite . J. Air & Waste Manage. Assoc. , 52 : 822 – 827 .
- Wehner , B. , Birmili , W. , Gnauk , T. and Wiedensohler , A. 2002 . Particle Number Size Distributions in a Street Canyon and their Transformation into the Urban-Air Background: Measurements and a Simple Model Study . Atmos. Environ. , 36 ( 13 ) : 2215 – 2223 .
- Whitby , K. T. 1978 . The Physical Characteristics of Sulfate Aerosols . Atmos. Environ. , 12 : 135 – 159 .
- Whitby , K. T. and Sverdrup , B. M. 1980 . “ California Aerosols: Their Physical and Chemical Characteristics ” . In The Character and Origins of Smog Aerosols , Edited by: Hidy , G. M. , Mueller , P. K. , Grosjean , D. , Appel , B. and Wesolowski , J. J. 477 – 517 . New York : J. Wiley Interscience .
- Wiedensohler , A. 1988 . An Approximation of the Bipolar Charge Distribution for Particles in the Submicron Size Range . J. Aerosol Sci. , 19 : 387 – 389 .
- Wiedensohler , A. , Orsini , D. , Covert , D. S. , Coffmann , D. , Cantrell , W. , Havlicek , M. , Brechtel , F. J. , Russell , L. M. , Weber , R. J. , Gras , J. , Hudson , J. G. and Litchy , M. 1997 . Intercomparison Study of the Size-Dependent Counting Efficiency of 26 Condensation Particle Counters . Aerosol Sci. Technol. , 27 : 224 – 242 .
- Wichmann , H.-E. and Peters , A. 2000 . Epidemiological Evidence of the Effects of Ultrafine Particle Exposure . Philosophical Transactions of the Royal Society A , 358 : 2751 – 2769 .
- Woo , K. S. , Chen , D. R. , Pui , D. Y. H. and McMurry , P. H. 2001 . Measurement of Atlanta Aerosol Size Distributions: Observations of Ultrafine Particle Events . Aerosol Sci. Technol. , 34 : 75 – 87 .
- Zhang , K. M. and Wexler , A. S. 2004 . Evolution of Particle Number Distribution Near Roadways. Part I: Analysis of Aerosol Dynamics and its Implications for Engine Emission Measurement . Atmos. Environ. , 38 : 6643 – 6653 .
- Zhang , K. M. , Wexler , A. S. , Zhu , Y. F. , Hinds , W. C. and Sioutas , C. 2004 . Evolution of Particle Number Distributions Near Roadways, Part II the Road-to-Ambient Process . Atmos. Environ. , 38 : 6655 – 6665 .
- Zhu , Y. , Hinds , W. C. , Kim , S. , Shen , S. and Sioutas , C. 2002 . Study of Ultrafine Particles Near a Major Highway with Heavy Duty Diesel Traffic . Atmos. Environ. , 36 : 4342 – 4335 .