Abstract
Generated aerosol particle deposition has been applied in laboratory scale to induce deactivation of commercial Selective Catalytic Reduction (SCR) catalysts of V2O5-WO3/TiO2 monolithic type. The monolithic catalyst has been exposed to the generated submicrometer particle of inorganic salts, KCl, K2SO4, and ZnCl2 at 200°C in a tubular reactor. The generated particles have been deposited on the catalytic surfaces by utilization of an electrostatic field. Physical characterization of the generated aerosol particles were conducted by Scanning Mobility Particle Sizer (SMPS) and Electric Low Pressure Impactor (ELPI) with and without catalyst in order to investigate the magnitude of the particle deposition. Particle charge distribution was also evaluated with a Tandem Differential Mobility Analyser (TDMA) set up.
SCR is the most common method to commercially reduce NOx emissions from combustion processes. Catalyst lifetime is important for process economics and extending catalyst life can allow future strengthened emission legislation and diminished NOx emissions.
Verification of particle deposition has been conducted through comparison with catalyst samples exposed to commercial biomass combustion condition.
The reactivity of both fresh and exposed catalyst samples as well as commercially used samples was examined in SCR reaction and the methods of deposition as well as the influence of the different salts on catalytic performance have been explored.
Catalyst samples have been evaluated with Scanning Electron Microscopy (SEM) with respect to surface morphology of the catalyst material. The laboratory deactivated catalyst samples showed resemblance with the commercially exposed catalyst sample with respect to salts concentration and deposition of the salts particles. The obtained influence on catalyst activity was comparable with commercially obtained catalyst activity reductions at comparable potassium concentration levels.
1. INTRODUCTION
Selective Catalytic Reduction (SCR) is commercially used for reduction of NOx emitted from combustion plants using coal, oil, or biomass as fuels. NOx is reacted with ammonia to generate nitrogen and water. A V2O5-WO3/TiO2 catalyst of monolithic type is used. During commercial operation, however, the catalyst is influenced by deposition or interaction of compounds in the flue gas resulting in a reduced chemical activity as well as decreased lifetime of the catalyst. This leads both to environmental and economical drawback.
Burning biomass has beneficial effects on the environment, such as decreasing CO2 emissions in the atmosphere and producing energy from renewable sources. Nevertheless, biomass combustion originates particulates, with a high concentration in submicrometer particle emissions. The fine mode of the flue gas particles is formed via condensation, nucleation, and chemical surface reactions of inorganic compound vapors when the gases are cooled downstream the boiler.
Concerns about submicron particles have been raised for their effect on human health. Furthermore, the presence of transition metals can lead to particle toxicity (CitationLighty et al. 2000).
Previous studies by CitationStrand et al. (2002), CitationPagels et al. (2003), and CitationWierzbicka et al. (2005) about particles emitted from small grate type boilers using woody-based biomass fuels showed a particle median diameter of 90–125 nm. The three most abundant elements in the fine mode were K, S, Cl, in agreement with the findings that the main components was K2SO4 and KCl. Moreover a high concentration of zinc has been found for some biomass installations. Therefore, in this work, these three salts are chosen as model compounds in the evaluation of impact on activity of SCR catalyst that are exposed to the flue gas from biomass burning.
The deactivation of SCR catalysts under biomass combustion conditions has been ascribed to the potassium salts (CitationKhodayari et al. 2001) and the submicrometer inorganic particles produced from biomass combustion can poison catalyst active sites and mask them through pore fouling. CitationMoradi et al. (2003) found that aerosol deposition on the catalyst surface can resist the reagent species diffusion, therefore slowing down the reaction activity. Moreover the authors reported that inorganic submicrometer particle penetration in the pore structure depends on the nature of the species.
Previous studies of the impact of potassium salts on SCR catalysts have been carried out utilizing wet impregnation method of the catalyst with diluted salt solutions (CitationChen et al. 1990; CitationChen and Yang 1990; CitationKamata et al. 1999; CitationKhodayari et al. 2000; CitationKhodayari 2001; CitationLisi et al. 2004; CitationZheng et al. 2004). It appears that the impregnating catalyst sample by a solution of poison is not applicable to study catalytic deactivation of SCR catalyst in a commercial plant. Since in the commercial plant the SCR catalyst will principally be deactivated by exposure to the inorganic aerosol particles. In view of the fact that the subject concerning emission of submicron particulate from combustion process and their impact on catalytic deactivation is not well explored, in this work a commercial SCR monolithic V2O5-WO3/TiO2 catalyst has been exposed to generated aerosol particles with a well-defined size distribution in laboratory scale. The major challenge of the work are technical issues to simulate in a short time a catalytic deactivation process in laboratory scale that resembles the decay process in a commercial plant. The catalytic deactivation under commercial conditions is a process of several years, while laboratory conditions must allow an accelerated deposition method. Deactivating the catalysts by one substance at a time also allows for a detailed understanding of the deactivation mechanisms, while in a real plant the catalyst will be exposed to all the emitted deactivation species at the same time.
In the present work, the SCR catalyst was placed in a tubular reactor. The catalyst was exposed to potassium salts and ZnCl2 in submicrometer size. Potassium salts were chosen based on their presence in biomass combustion and zinc chloride for its strong deactivation impact on SCR catalysts (CitationHerrlander 1990). For the purpose of verification a catalyst sample exposed to commercial biomass combustion conditions was also evaluated.
2. EXPERIMENTAL
2.1 Catalyst
A commercial catalyst from a V2O5-WO3/TiO2 type was used for the purpose of the deactivation experiments. The catalyst supplied by Cormetech Inc. was of a monolithic type. All samples used had a square cell opening of 6.4 mm, a wall thickness of 1 mm and a length of 160 mm.
2.2 Deactivation Species
The most abundant compounds found in the submicrometer particle range in biomass burning flue gases, KCl, K2SO4 as well as ZnCl2, known to have a strong impact on SCR catalyst activity, have been used as model compounds for the study of the deposition process.
2.3 Aerosol Particle Deposition Procedure
2.3.1 Aerosol Measurements
The generated aerosol particle flow was physically characterized using the set-up shown in .
The sampling was carried out isokinetically using a glass-sampling probe. To decrease the gas temperature, particle concentration, and relative humidity, the gas sample was diluted with particle-free, dry pressurized air (20°C) to give a ratio of 1/10. The relative humidity after dilution was measured with a TH-Calc 8720 (TSI Inc) to 12%.
The number size distribution for a size range of 20 to 284 nm of the aerosol particle flow was measured with a Scanning Mobility Particle Sizer SMPS (TSI Inc, Model 3936) that classifies aerosol particles according to their electrical mobility. The sample flow to the SMPS was further diluted to a ratio of 1/10, giving a total dilution of 1/100. A flow of 1 L/min was fed into the instrument.
The particle mass size distribution was measured with an ELPI (Electrical Low Pressure Impactor) from Dekati, Ltd., allowing classification of the aerodynamic diameter of the aerosol particles in the range 30–10,000 nm. According to CitationPagels et al. (2005) the ELPI measures the mass reliably for aerosol particles below an aerodynamic diameter of 3000 nm. At larger aerodynamic diameters the measured mass can be overestimated, which was neglected in further measurements. The sample flow into the ELPI was 10 L/min.
Particle charge distribution was evaluated using a Tandem Differential Mobility Analyser (TDMA) set up described by CitationKim et al. (2005). An initial aerosol classifier, DMA1 (TSI Inc, Model 3071) was used to separate the aerosol particles based on their electrical mobility before characterization by the SMPS (TSI Inc., Model 3936). Both negative and positive electrical potential scans were applied to the DMA1 for evaluation of negatively and positively charged aerosols. Uncharged aerosols were measured after removal of all charged aerosols in DMA1 by application of maximum electrical potential.
2.3.2 Electrostatic Deposition
The aerosol particles for deposition were generated by a pneumatic atomizer (Palas GmbH, AGK-2000). A liquid solution of deionized water and the respective salt with a concentration of 50 g/l was fed continuously into the atomizer reservoir. The gauge pressure was 1 bar. To decrease the relative humidity and avoid particle growth by condensation the flow from the atomizer (4 L/min) was mixed with particle free dried 20°C pressurized air (7 L/min). The gas mixture was then passed through an inertial impactor to separate large solution droplets before entering an electrically heated oven set at 300°C. Due to heat losses, the average temperature in the catalyst channels was 200°C (Microtherma 2 thermometer). The relative humidity was measured with a TH-Calc 8720 (TSI) to less than 1% at 200°C. According to CitationHinds (1999), the drying time of water droplets at these conditions are in the range of millisecond, with residence time of the gas in the oven being seconds. Drying of salt aerosols involves also the equilibrium between water content in the gas and the hygroscopic properties of the salts, which influences the nature of the generated aerosol particles also described by CitationHinds (1999). Deliquescence properties of KCl and K2SO4 have been reported by CitationPagels et al. (2003) to effloresce below relative humidities of 80 and 95%, respectively. To determine the aerosol properties in the experiment particles were collected on a filter and were examined. The salts were collected in particulate form.
The deposition of aerosol particles was carried out in the set up shown in . The catalyst samples consisted of a monolithic channel with a length of 160 mm with the flow passing axially though the catalyst channel.
To enhance the particle deposition rate, an electrostatic field was applied to the catalyst channel. An electrical field was obtained by placing a steel wire inside the catalyst channel and surrounding the catalyst channel walls with aluminum foil, as shown in . The wire was centered in the square channel of the monolithic catalyst. A differential potential generator was connected to the steel wire and the aluminum foil, grounding the aluminum foil; connecting the steel wire to a positive electrical potential. To obtain the optimal electrical field strength the voltage was screened and a corresponding value of 2.5 kV was chosen. Each catalyst channel was exposed to a gas flow of 1 L/min for a period of 10 h.
2.4 Commercial Biomass Combustion Exposure
A catalyst sample used in a commercial biomass combustion plant for a period of 6500 h was examined for verification. The fuel comprised 90% wood chips and 10% peat. The catalyst reactor was placed in the economizer section at a temperature of 350°C. The operating conditions for the catalyst are shown in . According to CitationStrand et al. (2004), the particle characteristics of the boiler flue gas are 1.2 × 107 particles/cm3 n with a geometric mean diameter of 68 nm, consisting of K, S, and Cl.
TABLE 1 Operating conditions of the commercial biomass combustion plant
2.5 Catalyst Activity Measurement
The catalyst samples reactivity was tested in the SCR reaction in order to reduce nitrogen oxides by reaction with ammonia. The reaction conditions are presented in , and the experimental set-up is shown in .
The percent total removal of NOx (X) has been calculated as:
TABLE 2 The reference conditions used for the activity measurements
2.6 Catalyst Characterization
Scanning electron microscopy (SEM), LEO, Gemini 1550 SEM was used for the surface morphology evaluation of the exposed samples. The catalyst surface was examined for particle deposition using SEM equipped with EDS (Energy Dispersive Spectroscopy).
Chemical analysis of the catalysts with respect to bulk concentrations of potassium and zinc was conducted with ICP-AES (Inductively Coupled Plasma—Atomic Emission Spectroscopy).
3. RESULTS
3.1 Physical Characterization of Generated Aerosol Particle
The physical properties of KCl, K2SO4, and ZnCl2 were measured using SMPS and ELPI before and after the flow was passed through the catalyst channel. The number size distribution was measured using SMPS and the mass concentration calculated from the ELPI measurements assuming effective densities presented in . The concentrations and mean diameters were compared. The results are presented at normal temperature and pressure conditions: 0°C, 1.01 × 105 Pa taking into account dilution factor.
TABLE 3 The characteristics of generated aerosol particle
Since the generated aerosol salts differ in density a mass difference was detected. The particle characteristics are presented in , the number size distributions in and the mass size distributions in .
FIG. 4 Number size distribution of the generated aerosol particles from different salts measured by SMPS.
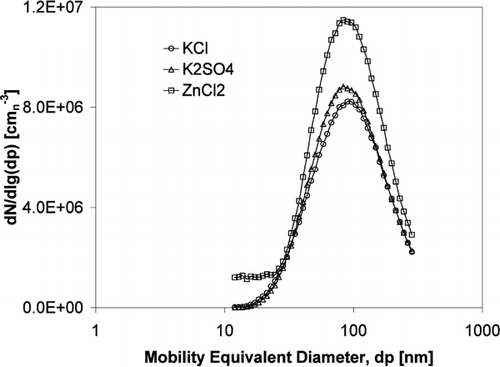
FIG. 5 Mass size distribution of the generated aerosol particles from different salts calculated from ELPI measurements using the salt bulk density.
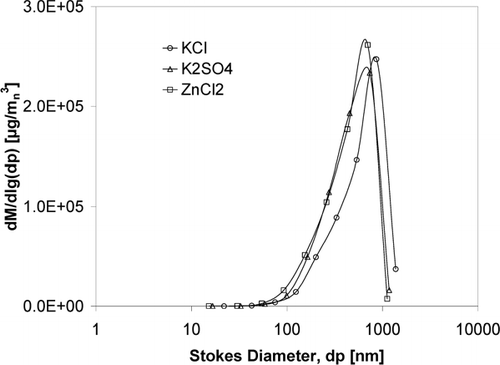
Aerosol charge distribution was assessed by measurement of charge fractions of generated particles. describes the fractions of positively or negatively charged particles depending on particle diameters for each of the three salts. For comparison, the Boltzmann charge distribution of positively or negatively charged particles is also displayed. The generated salt particles show few charged particles for diameters below 100 nm as compared to a Boltzmann distribution, while the charged particle fraction approaches a Boltzmann distribution at larger diameters. Charged particles are evenly distributed between positive and negative charges.
3.2. Particle Deposition by Electrostatic Field
In order to evaluate the optimal strength of the electric voltage applied to the catalyst channels, the field was varied from 0 to 3.5 kV. The number concentration of the KCl salt particle versus the electrical voltage was measured; results obtained are shown in . The deposition of particles increased with rising of the electrical voltage as shown in . For further deposition experiment the applied voltage was chosen as 2.5 kV. A further increase of voltage would not significantly enhance the particle deposition, but create instability of the electric field and increase spark-over between the electrode and the catalyst wall.
FIG. 7 Influence of different electrical voltage on deposition of the generated KCl aerosol particles on the catalyst: number concentration of particles exiting the catalyst channel using SMPS.
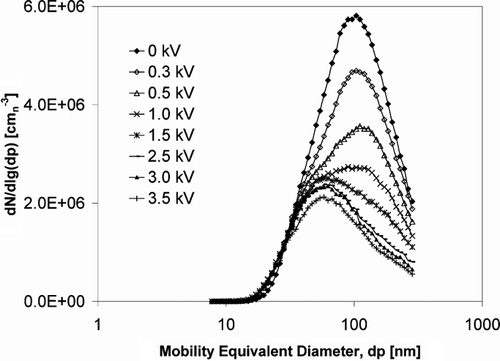
The influence of the electrical voltage was also investigated for K2SO4 and ZnCl2 salts. No significant differences were observed, the similar results were obtained for the K2SO4 and ZnCl2 salts.
The evaluation of the deposited particle in the catalyst channel was based on the measurement of the particle concentration at the reactor exit with and without applying electric field. The comparisons for the three salts are presented in and . The electrostatic force is predominantly acting on particles with a diameter larger than 90 nm and is showing the strongest deposition for particles above 100 nm. As displayed in , the particles smaller than 90 nm are mainly uncharged while the fraction of charged particles above 100 nm approaches a Boltzmann charge distribution.
FIG. 8 Relative difference of number size distribution of aerosol particles (with electric field/without electric field) using SMPS: (a) KCl with/KCl without, (b) K2 SO4 with/K2 SO4 without, (c) ZnCl2 with/ZnCl2 without.
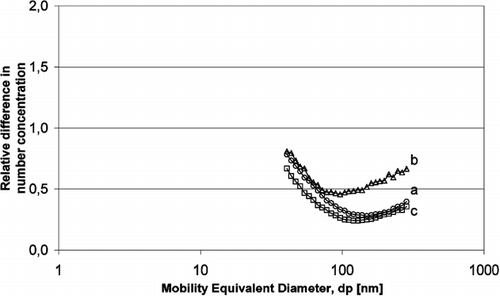
FIG. 9 Relative difference of mass concentration of aerosol particles (with electric field/without electric field) using ELPI: (a) KCl with/KCl without, (b) K2 SO4 with/K2 SO4 without, (c) ZnCl2 with/ZnCl2 without.
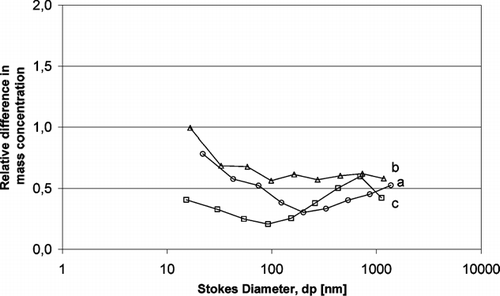
The experimental data in and , indicate a relative difference below 1 that confirm aerosol particle have been deposited on the catalyst surface.
The estimated mass fraction deposition based on the experimental data for KCl, K2SO4, and ZnCl2, respectively, is represented in .
TABLE 4 Estimated depositions of different salts on catalyst sample based on mass concentration measurements with ELPI
It is expected that the negative fraction of the generated particles deposit on the catalyst channel and the positive on the electrode wire and the sampling system. Nevertheless the forced deposition of particles by electric field is pronounced. The salts deposited on the catalyst sample were also visible when applying electrical field.
3.3 Catalyst Chemical Composition
Concentrations of potassium and zinc in the catalyst bulk material obtained by ICP-AES analysis are shown in . Increased potassium levels obtained through electrostatic deposition of particles for 10 h are comparable to potassium increases obtained through commercial exposure for 6500 h. Accumulation of zinc are not possible to compare as no considerable accumulation was found in the commercially exposed catalyst sample.
TABLE 5 Potassium and zinc concentration in the fresh and exposed catalyst samples
3.4 Catalyst Surface Morphology
The exposed catalyst surfaces have been examined by SEM. The deposition of the salts on the surface of the catalyst surfaces perpendicular to the flow of generated particles have been evaluated. The comparison for samples exposed to the salts using electrostatic deposition as well as commercially exposed catalyst sample is presented in a–d. The results indicate that the deposition of KCl by electrostatic forces produces cavity deposition of the KCl salt particles (). Samples exposed to K2SO4 and ZnCl2 salts also showed similar deposition pattern, and c. Salts depositions show agglomerates of particles with particle diameters in the 100 nm ranges. Larger agglomerates are also visible which may be a result of sintered particles.
FIG. 10 SEM-image of catalyst surface exposed to laboratory generated particles of (a) KCl, (b) K2SO4, (c) ZnCl2, and (d) commercial biomass combustion, SEM/EDS analysis is marked with +.
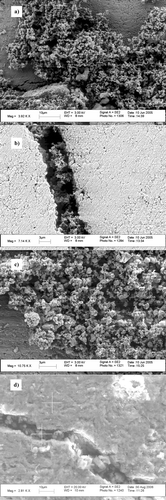
For verification of the particle deposition, the commercial catalyst sample was analyzed, (). Particle deposition in cavities is visible, although present at greater catalyst depths. The cavity deposition as well as catalyst surface (marked with + in ) was analyzed with EDS. Cavity deposition showed to consist of mainly Ca, K, S, Si, and O while the catalyst surface contains the catalyst components Ti, W, V, and O.
3.5 Catalyst Activity
The performance of fresh and exposed catalyst samples has been examined in a model reaction using selective catalytic reduction of NO with NH3 (SCR reaction).
The activities of the catalyst samples in the SCR reaction was compared at a reference temperature of 310°C with the results shown in . The measured activity reduction of KCl exposed sample was 11%, while a severe deactivation of 41% was observed for the sample exposed to ZnCl2. The reactivity of exposed K2SO4 samples remains nearly unchanged, only a 4% reduction was observed. Improvement of catalytic activity has been observed when other catalyst samples were exposed to K2SO4 in earlier work by CitationOlofsson (2004). An activity reduction of 15% was obtained during commercial exposure to biomass combustion conditions. It was observed that the catalyst samples exposed to generated particles for 10 h show activity reductions comparable to commercial catalyst samples exposed for 6500 h.
4. DISCUSSION
4.1 Salt Deposition Phenomena
Monolithic catalyst activity is limited by diffusion of species in the bulk phase under laminar flow conditions. Therefore, a suitable device to produce a deactivated catalyst sample in a short time through aerosol particle deposition process in laboratory scale experiment is fairly problematic. The catalytic deactivation that arises from particles deposition in a commercial biomass boiler is a prolonged process. This long-term deactivation process takes 3–4 years in full-scale experiment. The opportunity to evaluate the impact of the aerosol particles on the catalyst activity at laboratory scale is dependent on a reliable method that accelerates the exposure and deposition procedure.
Applying an electric field to the generated charged particles enhances the deposition by influencing the migration towards the catalyst channel walls. The electrostatic velocity (V) can be described as the particle electrical mobility (Z) times the electrical field strength (E) and is influenced by particle diameter (d) and charge (e) with Cc as Cunningham slip factor and η the viscosity according to equation Equation2 (CitationHinds 1999).
Applying Equations (Equation2) and (Equation3) to the charged salt particles evaluated by the TDMA with respect to their electrical mobility allows for evaluation of the particle collection efficiencies possible in the experimental set up. Acknowledging that the particles have multiple charges an average particle charge for the salts of 5 was calculated based on the TDMA measurements. Average particle charges have been reported by CitationTsai et al. (2005) to be in the order of 2 for atomizing of salt liquids, but charges of 8 was also reported depending on particle diameter and concentration of the salt solution. Theoretically possible collection efficiencies can thus be estimated and are presented in . Collection efficiencies for the three salts have been evaluated based on the results presented in and are compared with the theoretical levels in . Both KCl and ZnCl2 show evaluated collection efficiencies approaching the theoretical estimate for particle diameters of 100 nm while deviating at larger diameters and for KCl also for smaller particle diameters. K2SO4 show significantly lower collection efficiency. K2SO4 is seen both in the evaluation of catalyst composition () as well as in the estimated mass deposition () to indicate lower concentration and deposition. The electrical field was not uniform at the same distance from the wire inside the catalyst channel due to the square shape of the monolithic catalyst openings and to the heterogeneity present in the catalytic material. Furthermore the electric field may interfere with the catalyst material that result in the distortion of the electric field inside the channel. Thus the deposition will differ on each catalyst wall in this work. The morphological investigation of the deposition also reveals some non-uniform layers of salt particles on the catalyst surface, which confirms the above statement.
FIG. 12 Comparison of collection efficiencies for the three generated salt particles with theoretically estimated levels.
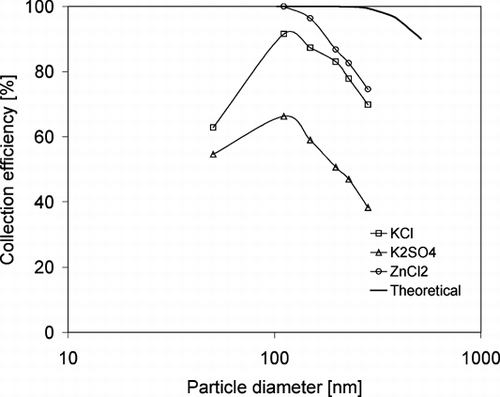
Resemblance between the deposition of the generated particles and the commercially exposed catalysts is also found in the morphological analysis. Particle agglomerates deposited in the commercial catalyst structure indicate similarities in the deposition processes although the electrostatic deposition could be foreseen to more strongly force particles towards the catalyst surface compared to deposition by particle diffusion.
Further improvements of the electrostatic deposition method are possible. A predetermined charging of the particles prior to entering the electrical field allows for more predictable particle deposition. Increasing the fraction of charged particles for diameter smaller than 100 nm would also improve the collection of the small particles extending further the simulation of process conditions available in biomass combustion.
4.2 Catalyst Deactivation Toward Exposure to Potassium Salts and Zinc Salt by Electrostatic Deposition
Previous investigations on V2O5-WO3/TiO2, SCR catalyst deactivations at laboratory scale have mostly focused on wet impregnation method with salt solutions.
The aerosol particle deposition methods of the present investigation show a pronounced catalytic deactivation by applying electric forced deposition. The poisoning effect is stronger for deposited KCl salt, while K2SO4 tends to promote the catalyst activity in correlation with previous work reported.
The results of the present deposition deactivation experiments also show a strong pronounced impact of ZnCl2 salt on the catalyst sample activity.
Verification of induced catalyst decay by comparisons with commercially exposed catalyst sample showed correlation between accumulation of potassium and decrease of catalyst activity. Laboratory exposed and commercial samples are comparable with respect to potassium concentration and activity decrease. A similar comparison for zinc was not possible due to lack of zinc accumulation during commercial exposure at the specific plant investigated.
The present work aimed to improve the aerosol mass deposition device in order to study the chemical and physical impact of the deposited aerosol particle from different salts and a better understanding of the catalytic decay of the SCR catalyst used in biomass combustion process. In the short-term deactivation process, the electrostatic deposition method was shown to give samples with quantities of deposited salts as well as activity reductions comparable with commercially exposed catalyst samples. Therefore, the electrostatic deposition method allows for evaluation of the catalytic deactivation mechanism by exposure to the aerosol salts in laboratory scale. In future work, it would be possible to investigate deactivation mechanisms of SCR catalyst in combustion process by exposing the catalyst to mixtures of different salts in the presence of an electrostatic field.
Further investigation on the comparison of deactivation of monolithic SCR catalysts by particle deposition as compared to wet impregnation with the evaluated salts have been carried out by the authors (CitationLarsson et al. 2006) indicating a strong resemblance between commercially exposed catalyst samples and samples deactivated by deposition of particles as compared to wet impregnated samples. Comparisons were carried out with respect to physical as well as chemical parameters and indicate the necessity to recreate the deactivation phenomena of the industrial process under laboratory conditions.
5. CONCLUSION
Concerning the decay effect of generated aerosol salts from potassium and zinc on monolithic SCR catalyst most of the previous investigations have primarily focused on wet impregnation methods. It is believed that the most of inorganic salts are present in submicrometer size when interacting with the monolithic catalyst under commercial combustion conditions. This investigation focused on the exposure of fresh catalyst to a defined particle size distributions of potassium and zinc salts.
Preparing the deactivated catalyst samples by applying an electric field to the catalyst give rise to enhanced mass deposition of the respective generated submicrometer salts resulting in increases in particle deposition comparable to what has been obtained through commercial operation of catalyst samples for 6500 h.
The deposited particle morphology has been assessed by SEM. The electrostatic deposition samples contribute to the irregular deposition of material on the external catalyst surface and pronounced deposition in catalyst surface cavities. Evaluation of commercial catalyst samples shows comparable deposition of particles in catalyst cavities.
The catalyst activity was reduced for the electrostatic deposition samples by 11% for KCl, nearly unchanged for K2SO4 and decreased to 41% for ZnCl2 compared to a 15% catalyst activity decrease obtained during commercial operation for 6500 h.
The deposition by electrostatic method of the submicrometer particles on monolithic SCR catalyst in laboratory scale can be further used to evaluate the impact of other inorganic salts present in biomass combustion as well as for evaluation of influences of commercial operating conditions on catalyst deactivation by deposition of submicrometer particles.
Acknowledgments
Financial support from the Swedish Research Council is gratefully acknowledged. The European Commission is also acknowledged for co-financing the Framework 6 project related to this research: Integrated Project “CHRISGAS” (contract SES6-CT-2004-502587).
Notes
* Normal temperature and pressure conditions: 0°C, 1.01 × 105 Pa.
a SMPS measurement
b ELPI measurement.
REFERENCES
- Chen , J. P. , Buzanowski , M. A. , Yang , R. T. and Cichanowicz , J. E. 1990 . Deactivation of the Vanadia Catalyst in the Selective Catalytic Reduction Process . J. Air Waste Manage. Assoc. , 40 : 1403 – 1409 .
- Chen , J. P. and Yang , R. T. 1990 . Mechanism of Poisoning of the V2O5/TiO2 catalyst for the reduction of NO by NH3, . J. Catal. , 125 : 411 – 420 .
- Hinds , W. C. 1999 . Aerosol Technology: Properties, Behavior, and Measurements of Airborne Particles , New York : John Wiley & Sons, Inc. .
- Herrlander , B. SCR DeNox at the Munich South Waste Incinerator . Air Waste Manage. Assoc., 83 rd Annual Meeting & Exhibition Pittsburg . June 24–29 , Pennsylvania , USA.
- Kamata , H. , Takahashi , K. and Odenbrand , C. U. I. 1999 . The Role of K2O in the Selective Reduction of NO with NH3 over a V2O5 (WO3)/TiO2 Commercial Selective Catalytic Reduction Catalyst . J. Mol. Catal. , 139 : 189 – 198 .
- Khodayari , R. 2001 . Selective Catalytic Reduction of NOx: Deactivation and Regeneration Studies and Kinetic Modelling of Deactivation , Lund , Sweden : Lund Institute of Technology . Doctorial Dissertation ISBN 91-7874-122-X
- Khodayari , R. , Andersson , C. , Odenbrand , C. U. I. and Andersson , L. H. Deactivation and regeneration of SCR catalysts used in bio fuel power plants . Proceedings of the 5th European Conference on Industrial Furnaces and Boilers, vol II . April 11–14 2000 , Espinho-Porto-Portugal . Edited by: Reis , A. , Ward , J. , Leuckel , W. and Collin , R.
- Khodayari , R. and Odenbrand , C. U. I. 2001 . Regeneration of Commercial Catalysts by Washing and Sulphation: Effect of Sulfate Groups on the Activity . J. Appl. Cat. B , 33 : 277 – 291 .
- Kim , S. H. , Woo , K. S. , Liu , B. Y. H. and Zachariah , M. R. 2005 . Method of Measuring Charge Distribution of Nanosized Aerosols . J. Colloid Interface Sci. , 282 : 46 – 57 .
- Larsson , A. C. , Einvall , J. , Andersson , A. and Sanati , M. 2006 . Targeting by Comparison with Laboratory Experiments the SCR Catalyst Deactivation Process by Potassium and Zinc Salts in a Large Scale Biomass Combustion Boiler . Energy & Fuels , 20 : 1398 – 1405 .
- Lighty , J. S. , Veranth , J. M. and Sarofim , A. F. 2000 . Combustion Aerosols: Factors Governing Their Size and Composition and Implications to Human Health . J. Air Waste Manage. Assoc. , 50 : 1565 – 1618 .
- Lisi , L. , Lasorella , G. , Malloggi , S. and Russo , G. 2004 . Single and Combined Deactivation Effect of Alkali Metals and HCl on Commercial SCR Catalysts . Appl. Catal. , 50 : 251 – 258 .
- Moradi , F. , Brandin , J. , Sohrabi , M. , Faghihi , M. and Sanati , M. 2003 . Deactivation of Oxidation and SCR Catalysts Used in Flue Gas Cleaning by Exposure to Aerosols of High-and Low Melting Point Salts, Potassium Salts and Zinc Chloride . Appl. Catal. , 46 : 65 – 76 .
- Olofsson , G. 2004 . A Study of Biomass Combustion Problems and the Selective Catalytic Oxidation of Ammonia , Lund , Sweden : Lund Institute of Technology . Doctorial Dissertation ISBN 91-7422-067-5
- Pagels , J. , Strand , M. , Rissler , J. , Szpila , A. , Gudmundsson , A. , Bohgard , M. , Lillieblad , L. , Sanati , M. and Swietlicki , E. 2003 . Characterisation of Aerosol Particles Formed During Grate Combustion of Moist Forest Residue . Aerosol Sci. , 34 : 1043 – 1059 .
- Pagels , J. , Gudmundsson , A. , Gustavsson , E. , Asking , L. and Bohgard , M. 2005 . Evaluation of Aerodynamic Particle Sizer and Electrical Low-Pressure Impactor for Unimodal and Bimodal Mass-Weighted Size Distributions . Aerosol Sci. Technol. , 39 : 871 – 887 .
- Strand , M. , Pagels , J. , Szpila , A. , Gudmundsson , A. , Swietlicki , E. , Bohgard , M. and Sanati , M. 2002 . Fly Ash Penetration through Electrostatic Precipitator and Flue Gas Condensor in a 6 MW Biomass Fired Boiler . Energy Fuels, , 16 : 1499 – 1506 .
- Strand , M. , Bohgard , M. , Swietlicki , E. , Gharibi , A. and Sanati , M. 2004 . Laboratory and Field Test of a Sampling Method for Characterization of Combustion Aerosols at High Temperatures . Aerosol Sci. Technol. , 38 : 757 – 765 .
- Tsai , C. J. , Lin , J. S. , Deshpande , C. G. and Liu , L. C. 2005 . Electrostatic Charge Measurement and Charge Neutralization of Fine Aerosol Particles during the Generation Process . Part. Part. Syst. Charact. , 22 : 293 – 298 .
- Zheng , Y. , Jensen , A. D. and Johnsson , J. E. 2004 . Laboratory Investigation of Selective Catalytic Reduction Catalysts: Deactivation by Potassium Compounds and Catalyst Regeneration . Ind. Eng. Chem. Res. , 43 : 941 – 947 .
- Wierzbicka , A. , Lillieblad , L. , Pagels , J. , Strand , M. , Gudmundsson , A. , Gharibi , A. , Swietlicki , E. , Sanati , M. and Bohgard , M. 2005 . Particle Emissions from District Heating Units Operating on Three Commonly Used Biofuels . Atmospheric Environment , 39 : 139 – 150 .