Abstract
This study demonstrates an approach for evaluating a molecular level sampling and analysis protocol for organic marker compounds at the high picogram m−3 (ppt) to low nanogram m−3 (ppb) mass concentrations in urban and background receptor sites. The Speciation of Organics for Apportionment of PM2.5 in the New York City Area (SOAP) project was conducted from May 2002 to May 2003 at four sites in New York City, New Jersey, and Connecticut. Its chief objectives were to expand the chemical characterization of organic compounds and to estimate the source contributions of carbonaceous fine particles at urban and background monitoring sites. Two major challenges were faced in order to successfully implement the SOAP sampling network. First, collection of adequate fine PM mass was necessary for successful quantitation of organic marker compounds. Second, sufficiently low blank levels were required for each marker compound for accurate identification and quantitation needed for source-receptor modeling. Initial field tests of representative samplers designed for sampling PM chemical species indicated insufficient sample mass collection, unless analytical sensitivity for organic markers could be greatly improved. Adequate PM mass was collected using a Tisch TE-1202 sampler that operated at a much higher flow rate (113 lpm). Preliminary field tests also revealed unacceptably high travel blank levels for n-alkanes and carboxylic acids. The mass of organic marker compounds observed on travel blank filters was reduced significantly by shipping filters in sealed filter holders. Further evaluation of the Tisch TE-1202 sampler also demonstrated the sampler was free of organic components and impactor grease upstream of the filter. These features also reduced the contribution of carbonaceous species to system blanks and therefore, to the total mass collected. As a result, blank levels for hopanes, PAHs, and dicarboxylic acids were below limits of detection (LOD), and n -alkanes (C25 to C32), n-alkanoic acids (C12, C14, C16, and C18), and phthalic acid exhibited acceptable low levels in all SOAP blanks ranging from 1 to 10 times the limit of detection for each compound class. Overall, adequate sample mass and sufficiently low blank levels were achieved successfully with the SOAP fine particle collection protocol.
INTRODUCTION
More than 32 million people in four major urban areas along the Northeast Corridor of the United States live in counties that are in nonattainment of the new National Ambient Air Quality Standards for fine particulate matter (PM2.5) (USEPA 2005). Altogether, the metropolitan areas of New York, Philadelphia, Baltimore, and Washington, D.C., account for more than one third of those living in PM2.5 nonattainment areas, with most in the New York City metropolitan area (USEPA 2005). Carbonaceous PM is a major if not the largest contributor to PM2.5 mass in many U.S. cities, including New York City (USEPA 2004). Since 1980, several field studies have measured and identified extractable organic matter associated with PM in the New York City metropolitan area as part of short-term intensive campaigns (CitationDaisey et al. 1980, Citation1982, Citation1984; CitationLioy et al. 1983; Harkov et al. 1984; CitationOffenberg et al. 2003; CitationCass, 1998; CitationFine et al. 2002). These studies focused on specific chemical groups such as PAH compounds and organic acids, or specific source components such as wood smoke markers. The results provided early insights on the composition and sources of organic particulate matter in New York City and the Northeastern United States based on these target groups. The Speciation of Organics for Apportionment of PM2.5 in the New York City Area (SOAP) project was initiated for the purposes of expanding the chemical characterization and estimating organic source contributions for urban and background PM2.5.
Molecular organic markers in particulate organic matter are useful indicators of PM2.5 sources that can be used for source apportionment to help develop control strategies (CitationSimoneit et al. 1980; Currie et al. 1982; CitationSimoneit and Mazurek, 1982; CitationMazurek, 2002; CitationMazurek and Simoneit, 1984; CitationSimoneit 1984, Citation1985, Citation1986, Citation1989; CitationMazurek et al. 1989, Citation1991, Citation1997; CitationHildemann et al. 1991; CitationRogge et al. 1993; CitationBrown et al. 2002; CitationCabada et al. 2002; CitationChen et al. 2002; CitationFraser et al. 2002, Citation2003; CitationChow et al. 2004, Citation2007; CitationLee et al. 2002, Citation2004; CitationZheng et al. 2002, Citation2006, Citation2007; CitationSchauer et al. 1996; CitationManchester-Neesvig et al. 2003; CitationSheesley et al. 2004; CitationYue and Fraser, 2004a,Citationb; Citationde Gouw et al. 2005; CitationGorin et al. 2006; CitationHerkes et al. 2006; CitationHerner et al. 2006; CitationOndov et al. 2006a,Citationb; CitationPekney et al. 2006; CitationRinehard et al. 2006; CitationRobinson et al. 2006a, Citationb, Citationc; CitationSubramanian et al. 2006a,Citationb; CitationWard et al. 2006; CitationBrook et al. 2007; CitationKleeman et al. 2007). We have refined a method for analysis of molecular markers in fine particulate matter (CitationLi et al. 2005, Citation2006), and reported earlier the detailed organic chemical composition of particulate matter in Philadelphia (CitationLi et al. 2006; CitationSihabut et al. 2005; CitationRay and McDow, 2005). Organic matter emitted from sources such as motor vehicle exhaust, biomass burning, and cooking typically accounts for a considerable fraction of urban fine particles. The organic fraction contains numerous compound classes and individual molecules that can be used as markers which link to sources and/or atmospheric transformation processes. Gas chromatography/mass spectrometry (GCMS) methods were developed by Mazurek et al. (Citation1987, Citation1989) for analysis of molecular markers associated with urban sources. This protocol has been applied successfully in several U.S. airsheds for source apportionment (e.g., CitationSchauer et al. 1996; CitationSchauer and Cass, 2000; CitationZheng et al. 2002, Citation2006, Citation2007; CitationFraser et al. 2002, Citation2003; CitationManchester-Neesvig et al. 2003; CitationYue and Fraser, 2004a,Citationb). In this article we describe research results centered on the evaluation and implementation of sampling and analysis procedures for a three state regional PM2.5 sampling network. The purpose of the SOAP experiment was to collect fine particulate organic matter to evaluate the molecular markers present and relate this information to emission sources. SOAP results will assist NY, NJ, and CT with the development of air quality management plans aimed at reducing emissions from sources of carbonaceous fine particles. The results we report in this paper focus on a two-year effort that evaluated existing standard operating protocols for Speciation Trends Network (STN) chemical species and modified these protocols for the molecular level sampling and analytical requirements of the SOAP network.
Although the primary purpose of the SOAP project was to support source apportionment specifically in the New York City metropolitan area, the approach and challenges described here can be more broadly applied to other PM sampling networks focusing on source apportionment, epidemiological, and atmospheric chemical studies requiring molecular level organic composition information. For example, existing sampling networks such as STN or the Interagency Montitoring of Protected Visual Environments (IMPROVE) routinely operate multiple sampling channels that can be modified for sampling organic components. Also, archived filters from these networks have great potential for use in understanding sources of exposure and health impacts of species or sources. In the SOAP study, samplers already in use by the STN network were considered carefully and the feasibility of using these samplers was evaluated. Key challenges included collection of sufficient mass reduction of blank levels for accurate quantitation of marker compounds and total organic carbon (OC) aerosol mass, and acceptable sample handling and storage procedures. The evaluation of these sampling challenges and approaches to addressing and validating acceptable alternative procedures are presented.
TABLE 1 Target measurements at SOAP 2002–2003 network sites
EXPERIMENTAL
Sampling Network
The SOAP sites were located at existing air quality monitoring sites operated by NY, NJ, and CT (). Sites were selected to correspond to existing speciation network sites of the Air Quality System (AQS) PM2.5 and the Speciation Trends Network so that simultaneous mass and total organic and elemental carbon concentrations were available. The four sites included the principal U.S. EPA supersite in New York City in Queens, NY; an urban site in Elizabeth, NJ that generally is upwind of the supersite; a suburban site in Westport, CT that usually is downwind of the supersite and is within 1 km of the Long Island Sound; and a regional background site in Chester, NJ. The Queens College site is in an urban area with typical urban traffic patterns. Mobile sources also are likely to contribute a large portion of the fine particulate matter at the Queens site because two busy interstate highways are within 1 km of the site: the Long Island Expressway (I-495) and the Van Wyck Expressway (I-678). Interstate 95 and La Guardia airport also are near the Queens site. Elizabeth, NJ is an urban industrial site adjacent to Toll Plaza 13 of the New Jersey Turnpike. Approximately 220,000 vehicles per day traverse the Turnpike section which is within 500 m of the Elizabeth site (CitationOzbay, 2006). Three major industrial facilities are located within 1.25 km of the site, and frequently there is heavy air traffic above the site with aircraft en route to Newark Liberty Airport approximately 0.8 km away. The Elizabeth, NJ site is within 0.5 km of Port Elizabeth which is part of the Port of NY/NJ. The port complex is the largest maritime transportation center in the Eastern U.S. Westport, CT is a coastal suburban site located in Sherwood Island State Park in Fairfield County, approximately 0.5 km south of I-95. Although the immediate vicinity of the site is sparsely populated, it is surrounded by residential suburban neighborhoods beginning within 1 km of the site. Chester, NJ is a rural, commercial site with moderate traffic from local New Jersey Routes 513 (372 meters north) and 24 (221 meters west). Other potential industrial PM2.5 sources are at least 5 km away.
FIG. 1 Map of the SOAP sampling network showing Queens College (Flushing, NY), Elizabeth, NJ, Westport, CT, and Chester, NJ sites.
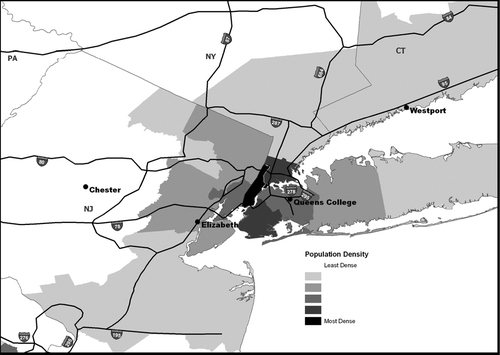
TABLE 2 Molecular level testing of common analytical and sampling background contaminants
Sampling began May 2, 2002 and continued through May of 2003. Samples were collected with a Tisch TE-1202 sampler for 24 hours, from midnight to midnight every third day, following a sampling schedule that was identical to that used by the Speciation Trends Network. Seasonal sample composites were obtained by combining five to ten 24-hour samples at least once per season at each site. Collection dates are listed for composite samples in Supplementary and for blanks in Supplementary . Generally, the number and dates of samples included in a composite were based on the number of samples collected successfully at all four sites in a given season. Westport was excluded from the first two composites because of early sampling problems at that site. Over 700 successful filters were obtained; however, selection of individual samples for composites was based mainly on completeness of the data. In several instances one or more of the samples either failed to sample for the entire period or were otherwise invalidated. Although completeness exceeded 80% at individual sites, successful collection at all four sites was required for including a sample in a composite. A travel blank and four field blanks were analyzed from each site. A field blank was collected during each season (spring, summer, fall, and winter) at each site, and one additional field blank was collected at Chester during the collocated sampler comparison for a total of four travel blanks and seventeen field blanks. In addition to collecting PM2.5 mass and speciation data, criteria pollutants, meteorological data and other pollutants such as volatile organic compounds (VOCs), carbonyls, and polycyclic aromatic hydrocarbons (PAHs) were collected at some of the network sitesk ().
Organic Compound Chemical Analysis
The full description of the molecular marker extraction and GCMS analysis procedures is reported by Li et al. (Citation2005, Citation2006). Perdeuterated n-tetracosane (nC24D50) was added as the internal standard to all blank filter composites prior to solvent extraction. A uniform mass (10 ng) was added to each filter blank composite. The same mass of nC24D50 was added to the entire set of ambient filter composites, thereby allowing direct quantitative comparison of the blank organic compositions with the SOAP ambient samples.
Travel, dynamic and field blank filter composites were extracted by soxhlet extraction in 250 ml mixture of 1:1 methylene chloride: acetone. The extracts were evaporated to 5 ml by using a Kuderna-Danish apparatus and then to 100 μ L by high-purity nitrogen gas. The extracts were divided into two portions for further analysis by a Varian Saturn 3800 GC Gas Chromatograph/Ion Trap Mass Spectrometer (GCMS). One aliquot was derivatized by adding freshly prepared diazomethane (CAS number 334-88-3) to convert organic acids and phenolic compounds to their methyl derivatives (Fatty Acid Methyl Ester “FAME” fraction) (CitationMazurek et al. 1987, Citation1989). Approximately 1 μ l of concentrated extract was injected in splitless mode onto a DB-1701 fused silica capillary column to separate organic mixture components (30 m length, 0.25 μ m coating thickness and 0.25 mm internal diameter, Agilent/J&W Scientific, Wilmington, Delaware). The GC temperature program consisted of the following steps: (1) initial isothermal hold for 3 minutes at 50°C; (2) a temperature ramp of 20°C /min up to 150°C; (3) isothermal hold for 3 minutes; (4) temperature ramp of 4°C/min to 280°C; and (5) a final isothermal hold of 17 minutes.
Selective ion monitoring (SIM) was used to test for background levels of common contaminants that have the potential to interfere with organic marker analysis. Common classes of contaminants tested are summarized in and are listed individually in Supplementary . Standard mixtures were prepared for the SOAP ambient molecular markers (), providing the highest level of compound authentication and quantitation in the organic extract mixtures. The internal standard (nC24D50) was added to the standard suites. A single quantitation ion (m/z) was used with two confirming ions (m/z) for the compound measurement. Quantitation was based on linear five-point calibration curves (R2 > 0.95) for each compound (CitationLi et al. 2005) determined before and after sample analysis.
TABLE 3 SOAP molecular markers
RESULTS AND DISCUSSION
Speciation Sampler Evaluation
Because STN samplers have been operating routinely throughout the United States for more than seven years, a convenient and efficient approach for collecting organic PM for understanding source contributions to PM ambient levels would first involve little to no modification of these existing collectors. The suitability of the STN speciation samplers for subsequent molecular level analysis of the PM filters was evaluated with a subset of molecular marker compounds in this study. The test markers included the n-alkanes, PAHs, hopanes, and n-alkanoic and dicarboxylic acids (as methyl esters). The two objectives of this evaluation were (1) to verify that sufficient fine PM2.5 mass was collected in a composite sample for each of the target compounds to be analyzed, and (2) to verify that blank levels were sufficiently low for each marker, and thereby avoid sampling bias that could cause interference with accurate identification and measurement.
We first investigated the feasibility of molecular marker analysis generated from composite samples. The ambient samples were collected on 47 mm quartz fiber filters using an extra channel of an approved speciation sampler. Original plans called for the evaluation of four samplers available for speciation sampling: Anderson Reference Ambient Air Sampler (RAAS) Met One Speciation Air Sampling System (SASS), University Research Glassware (URG) Mass Aerosol Speciation Sampler (MASS), and Rupprecht & Patashnick (R&P) Partisol Model 2300 Speciation Sampler. Except for the R&P 2300 Partisol Sampler, each sampler was evaluated for total organic carbon mass in PM2.5 samples by CitationSolomon et al. (2000). At the time of implementation, all three states involved in the SOAP study had selected either the Met One SASS or the R&P Partisol 2300 Speciation Sampler for use in the Speciation Trends Network so initial sampler evaluation was limited to these two instruments.
A composite fine PM sample was generated by sampling all channels in parallel with the Met One SASS and the R&P Partisol 2300 instruments. The Met One SASS was operated at a flow rate of 6.7 liters per minute (9.6 m3 sampled air volume) and the R&P Partisol 2300 sampled at a flow rate of 10.0 liters per minute (14.4 m3 sampled air volume). The Chester, NJ, site upwind of the NYC metropolitan area was selected as the evaluation site since it consistently had the lowest PM2.5, EC and OC masses of all four sites based on evaluation of STN reported data. Consequently, the Chester, NJ, site would provide the greatest challenge for collection of sufficient PM2.5 mass and associated organic molecular marker quantitative measurement.
TABLE 4 Composition of trace level organic background chemicals contributed from extraction solvents in SOAP travel and field blanks
TABLE 5 Comparison of area ratios of molecular marker quantitation ions to m/z to n-C24D50 internal standard m/z 82 for tracers present above the instrument detection levels for the Varian Saturn 3800 GCMS
Neither the Met One SASS nor the R&P Partisol 2300 speciation sampler collected sufficient mass for quantitative analysis of all classes of compounds by GCMS. Ample amounts of n-alkanes and n-alkanoic acids were collected with both samplers. There was no difficulty quantifying alkanes or carboxylic acids from the composite ambient test samples. The n-alkanes contained 22 to 28 carbon atoms and each homolog ranged in concentration from about 10 to 50 ng/m3. However, other key species of high value for source apportionment such as hopanes and PAHs, were not detected because insufficient PM2.5 total mass was collected. In earlier source apportionment studies of particulate organic matter, hopanes were identified as a valuable marker for motor vehicle exhaust (CitationSchauer et al. 1996). Only two hopanes were detected of the eight hopanes reported in relevant motor vehicle source profiles. The two hopane homologs were barely above limits of detection (∼ 0.01 ng m− 3). Detection limits for the marker compounds as groups were determined by GCMS analysis of low concentration standards with concentrations prepared in the range of 0.001 to 0.1 μ g/mL. Mass-to-charge (m/z) ions were monitored corresponding to the quantitation ion of the molecular markers ( and ). If the area of the m/z ion was greater than 5 times the area of the baseline noise peak, the compound was judged “detected” by the Varian ion trap GCMS. M/z areas were converted to ambient mass concentrations using compound response factors and the air volume for a typical sample composite. For polycyclic aromatic hydrocarbons, concentrations of all target compounds were consistently below the detection limit (∼ 0.05 ng m–3 for the PAH compounds). Detection limits for n-alkanes were 0.01 ng m–3, and were 0.02 ng m–3 for diacids and n-alkanoic acids (CitationLi et al. 2005). Based on these results from the Met One SASS and R&P 2300 Partisol speciation samplers, we concluded that greater sensitivity was required for these samplers to be used routinely for molecular marker analysis. The Tisch TE-1202 sampler was selected as a candidate sampler for evaluation because its substantially higher flow rate allowed collection of greater PM2.5 mass.
The Tisch TE-1202 sampler had a considerably higher flow rate than the other speciation samplers tested. The sampler operated at 113 liters per minute (162.7 m3 sampled air volume) and was equipped with a 2.5 μ m cyclone inlet (Part No. TE-3-900-20). It was designed to sample with a filter and polyurethane foam sorbent in series. For sampling in the SOAP network, just the filter portion of the Tisch collector was used because only the particulate components were of interest. Particles were collected on quartz fiber filters which were processed at 550–600°C, to produce low carbon background levels needed for molecular marker chemical analysis (CitationMazurek et al. 1987, Citation1989).
Bulk Carbon Background Evaluation
Besides PM2.5 sample mass, a second major objective of this project was to obtain blank levels low enough for quantitative analysis of each marker compound. This goal required identification of all background organic matter and verification that contaminant mass levels were approximately a factor of 10 less than the 300 pg m–3 to 10 ng m–3 ambient concentrations typical of most molecular markers. Therefore, early in the SOAP network design an initial study was conducted on the existing STN samplers at the four SOAP sites as potential instruments for fine particle organic molecular marker analysis. Using an approved speciation sampler was a desired outcome for two reasons. First, the samplers were already at the sites, and second, state operators were experienced in the standard operating procedures involving the instruments. Inspections were performed of the candidate samplers and interviews were conducted with the site operators at the collocated SOAP and STN sites. During this preliminary step, potential sources were noted of organic contamination stemming from sample collection and handling procedures. Molecular-level GCMS analysis of a combination of travel, dynamic and field blanks were used to isolate the source(s) of carbon contamination to ambient samples. Field blanks were filters placed in the sampler for the same period as a real sample, but for which the sampler was not in operation (i.e., no air flow through the sampler). The dynamic blank test for potential contaminants from the sampler was carried out by placing the filter holder in the sampler, turning the sampler on for 10 minutes, and removing the blank filter. In all other aspects, field and dynamic blanks were treated as samples, and underwent the same shipping and storage procedures. The travel blank was prepared and shipped in the same manner as the samples, but was not removed from the polyethylene bags by the site operators. Only laboratory personnel opened the travel blanks after the blanks were returned from the field. Multiple blanks of each type were collected to correspond to the anticipated number of ambient filters that would eventually be grouped to form a composite.
The quartz microfiber sampling filters usually collect considerable organic carbon mass on passive exposure to ambient air (CitationMcDow and Huntzicker 1990). Also, impactor greases and plastic sampler parts can be sources of organic contaminants (CitationMazurek et al. 1991). Common organic contaminants that may be contributed from commercial sampler components include plastic, rubber, oils, and hydrocarbon and silahydrocarbon greases (). Artifacts can be single compounds or, more frequently, as homologous series and complex mixtures present in greases and oils. Molecular level analysis of several blanks was performed by GCMS analysis of the filter extracts. Selected ion monitoring (SIM) targeted homologous compound series and individual compounds in the blank samples ().
Travel blanks from the initial sampler tests with the Met One SASS and R&P Partisol 2300 speciation samplers indicated unacceptably high n-alkane levels. The presence of the n-alkanes most likely was due to grease or oils contributed by sample storage and handling operations, and unrelated to sampler materials or characteristics. However, because of the small sampling flow rates, a relatively small mass of n-alkanes in the travel blanks translated into substantial contributions to apparent ambient concentrations, in excess of 1 ng m–3. Therefore, before proceeding with the Tisch TE-1202 sampler evaluation, an effort was devoted to improving the design of the filter housing cassettes and shipment packaging materials. The filter cassettes would be used to store the unsampled blanks and sampled filters during shipping and storage at the sites. The goal of the design effort was to produce a shipping module that would substantially reduce or eliminate the unacceptable n-alkane levels in the travel blanks. The sample filters used for the study were baked at 600°C in a muffle furnace for 4 hours to remove organic contaminants. Immediately after baking out, filters were placed in shipping modules specially designed for this project. A shipping module is shown in . It consisted of three parts: (1) the filter holder to be used in the sampler; (2) an aluminum shield that seals off the upstream portion of the filter holder from exposure to airborne contaminants; and (3) a plug that seals off the downstream portion. The shipping module was placed in a resealable polyethylene bag and placed in a commercial, well-insulated cooler (36 quart volume) and chilled to ≤ 4°C using 6 to 8 freeze-packs (Little Shivers, Lifoam Corp.). The coolers were shipped to the state agencies responsible for sampling. Once collected, the site operators were instructed to store the filter holders in onsite freezers until shipping by overnight express delivery to the laboratory. Upon return to the PI's analytical laboratory, sampled filters were removed from the filter holders and stored frozen at –10 C in prefired glass jars to prevent alteration of the organic compounds prior to the molecular marker analysis process.
The Tisch TE-1202 sampler is well suited for the collection of organic PM. As previously indicated in samplers not specifically designed for this purpose, the impactor greases and plastic and rubber sampler parts can be sources of organic contaminants collected along with the ambient PM (CitationMazurek et al. 1991). In the Tisch sampler the PM2.5 cutpoint is achieved with a cyclone inlet and inlet tubes and filter holders are constructed from anodized aluminum. Therefore, impactor greases and organic polymeric materials such as plastic, rubber or other carbon-containing components are not present upstream of the sample filter.
After developing and incorporating the filter shipping modules, the carbon blank levels associated with the Tisch sampler were evaluated using field, dynamic and travel blanks. The purpose of this study was to investigate the importance of deposition and contamination of the prefired microquartz filters. In an initial field test of the Tisch sampler, the n-alkanes were the only compounds detected in any of the blanks, and in all cases, average blank levels were present at concentrations under 300 pg m− 3. These blank levels were generally less than about 10% of ambient sample concentrations of 2 to 8 ng/m3 recently observed in Philadelphia ambient PM10 samples (CitationLi et al. 2006). Field blanks appeared to be greater than the travel blanks, and dynamic blanks indicated no significant contribution from the sampler. Overall, the Tisch TE-1202 sampler was judged to be the only sampler option for the SOAP network because of two factors: 1) larger ambient sample size, and 2) significantly lower background organic carbon levels. New Tisch TE-1202 (2-channel) or TE-1204 (4-channel) samplers were purchased by the states and installed and tested at the 4 sites before beginning operation of the SOAP network for ambient samples.
SOAP Network 2002–2003 Blanks
Blanks consisted of 1 to 4 filters that were scheduled during the SOAP network operation (13 months). Every sixth sampling day corresponding to the STN PM sampling schedule (i.e., every calendar eighteenth day) was designated for field blank collection (Supplementary ). Travel blanks were never opened except by laboratory personnel while in the analytical lab. During operation of the SOAP network, field blanks were collected on non-sampling days designated by the STN annual schedule. Field blanks were exposed from 1–2 days within the Tisch collector to monitor the exposure of ambient filters while the sampler was off (no air flow through sample filters). Since no significant contribution from the Tisch sampler was indicated by the initial field test, dynamic blanks were not collected during the SOAP 2002–2003 field experiment due to scheduling and logistical constraints at the 3 state-operated STN/SOAP sites.
All blanks were treated identically to the ambient filters, including molecular level analysis by GCMS. Composites were generated seasonally to coincide with the seasonal composites of the SOAP ambient samples. shows the GCMS plots of the Reconstructed Ion Current (RIC) for the Elizabeth, NJ, travel blank, summer 2002, fall 2002, winter 2002/03, and spring 2003 field blank, and the summer 2002 ambient PM sample composite (July 25, 28, August 12, 15, 18, 21, 24) for comparison. The SOAP travel and field blanks from all 4 sites were virtually the same in composition and abundance to those blank composites shown for the Elizabeth, NJ site.
FIG. 3 Comparison of the organic complex mixture (as methyl derivatized fraction, Fatty Acid Methy Esters, FAME) extracted from Elizabeth, NJ, travel and seasonal composite field blanks with a summer ambient sample composite. The reconstructed ion current (RIC) intensity is plotted against elution time.
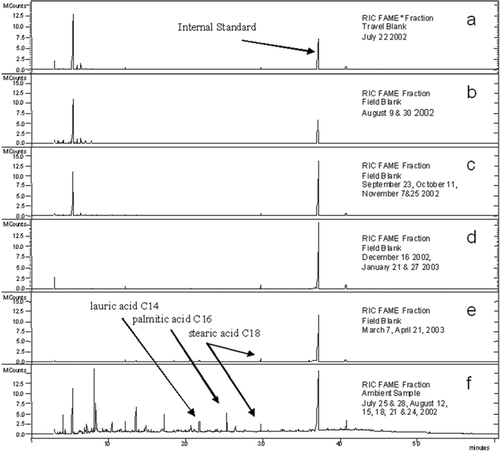
lists the retention time, compound identity, molecular weight (MW), Chemical Abstracts Service (CAS) number, and quantitation ions for peaks present in the Elizabeth, NJ blanks. Contaminant compounds were identified by comparing the peak mass spectral fragmentation pattern with the NIST/EPA/NIH Mass Spectral Database (release 2, 2002). The early peaks < 10 min are low MW polar compounds that are trace impurities in the high-purity extraction solvents and reagents. Siloxanes are another subgroup of contaminants eluting before 10 min and likely are from distillation apparatus used in the manufacture of distilled-in-glass grade high-purity solvents. The siloxanes demonstrated m/z 73 ions in the mass spectra and the spectra matched well with the NIST database. The single large peak at 37.39 minutes is 1,2-benzenedicarboxylic acid, mono-2-ethylhexyl ester (“diethylhexyl phthalate,” DEHP). DEHP has a base peak of m/z 149 and was present in all SOAP blanks. DEHP is a common plasticizer found in vinyl and plastic products, including chemical packaging materials, plastic tubing and laboratory vinyl gloves. The impurities were present at roughly the same level in all blanks and ambient samples and did not interfere with the identification and measurement of the molecular markers because Selected Ion Monitoring detection (SIM) was used for quantitation. Peaks eluting before 10 min were not generally of interest for the SOAP study. The absence of many unresolved peaks in the blank RIC plots from 30 to 50 minutes demonstrates hydrocarbon lubricating greases and oils were not present. Because most compounds present in the travel and field blanks were semivolatile compounds eluting before 10 min, we believe the organic contaminants were contributed from extraction solvents and chemical reagents, most likely during the solvent extraction and diazomethane addition steps. Very little organic background was contributed to the organic mass during the sample collection, handling and storage stages based on the comparison of the SOAP travel and field blanks (21 total blanks). The absence of higher molecular weight compounds, specifically n-alkanes and the polysiloxanes as monitored by GCMS SIM, verify low and acceptable organic carbon background levels during sample collection, transport, and storage.
Additional verification was needed to confirm the suitability of the chemical analysis procedures for molecular marker quantitation using SIM and key m/z ions. All SOAP travel and field blanks were monitored for the complete suite of markers using the characteristic m/z ions (). An equivalent mass of the internal standard was added to each blank composite and ambient composite (n-C24D50 total added mass = 10 ng), which allowed a mechanism for quantitative comparison of the blank levels to the ambient samples. Area ratios of the marker compound m/z quantitation ion to m/z 82 of n-C24D50 were calculated for each ambient sample and blank (). The average area ratio, standard deviation (STD) and relative standard deviation (RSD) were calculated for each marker compound in all SOAP blanks (n = 20). The average blank area ratio for the marker compound was compared to the ambient sample average area ratio (n = 40) to verify whether the blank concentrations were low enough for accurate quantitation of that compound. Most marker compounds in the SOAP 2002–2003 blanks were below limits of detection. The limit of detection area ratios to n-C24D50 were calculated as: hopanes = 0.00073; n-alkanes = 0.00025; PAH = 0.00210; diacids and n-alkanoic acids = 0.00720.
Thirteen out of 39 molecular markers were detected in some blanks when monitored by SIM (). PAHs and hopanes were not detected in any blank. Low levels of C25 to C32 n-alkanes were detected by GCMS SIM analysis. These alkanes were detected in the Queens and Westport travel blanks and only 1, 2, or 3 homologs were detected in five out of 12 total field blanks. The average areas of the m/z quantitation ion for the marker compounds shown in were compared to the average areas of the same ion in the blanks. The average ambient levels for the individual n-alkanes were from 12 to 50 times higher than the average area ratio for that homolog in the blanks. The lowest ambient to blank comparisons were for the n-C30 (19 times) and n-C32 (12 times) alkanes and were approximately 4 to 6 times higher than the average blank levels. The highest ambient to blank comparisons were 45 (n-C25) to 50 (n-C29) times the blank levels.
Blank levels were generally lower than limits of quantitation. Guidelines provided by the ACS Committee on Environmental Measurements establish the limit of quantitation as 10 times the standard deviation just above the limit of detection (LOD) (CitationACS 1980). These guidelines have been updated by CitationKeith (1991) with the same recommended factor and have also been recognized by the U.S. EPA (CitationUSEPA 2003). With the exception of the n-C30 and n-C32 alkanes, the blanks were comfortably below the analytical method limits of quantitation for the n-alkane molecular markers. However, in the worst case blank levels of the n-C30 and n-C32 alkanes were still only about 5–10% of ambient levels for these compounds, and blank subtraction of these compounds is likely to have little effect on ambient concentration.
Mono- and dicarboxylic acids also were screened in the SOAP blanks. The average area ratios of target compounds in all blanks were calculated for each compound using the quantitation ion and m/z 82 of the internal standard (n-C24D50) (). Most compounds were below the detection limits (BDL, ratio of quantitation ion to m/z 82 for n-C24D50 = 0.0072 area ratio). Only the C12, C14, C16, C18, monocarboxylic acids and phthalic acid were present in the SOAP blanks above this threshold. The relative standard deviations of the field blanks for these compounds ranged from 46% (C16 acid) to 157% (phthalic acid). The high variability of the acids present in the SOAP blanks can be explained by the fact the measurements were conducted with marker concentrations ranging from 1–10 times the LOD level (0.00720 area ratio). Because the presence of the C10, C12, C14, C16, C18, and phthalic acids in the blanks were between only 1 to 10 times limits of detection, blank subtraction is likely to have little impact on ambient sample concentration. Overall, the SOAP 2002–2003 blanks were consistently below or near limits of detection for all target molecular markers.
CONCLUSIONS
Measurement of organic molecular markers in fine PM requires detailed chemical analysis at the bulk and molecular levels. Test ambient filters collected with candidate samplers must be screened for the presence of marker compounds and for adequate mass of each marker compounds for good quantitation. The SOAP 2002–2003 fine PM sampling network demonstrated high quality control levels with the 80% success rate of ambient filters collected and with the organic marker background levels in the field and travel blanks below limits of detection for 26 of 39 target compounds. The markers screened in the SOAP blanks using new Tisch speciation samplers were at very low levels. Over 67% of all molecular markers in the SOAP 2002–2003 blanks, including the compound classes of hopanes, PAHs, dicarboxylic acids, and about half of the monocarboxylic acid markers, were below limits of detection. The other 33% of the markers (C25–C32) were present in mass levels from 1 to 10 times the limit of detection. N-alkanes from 25 to 32 carbons are contained in petroleum based lubricating oils and greases. These compounds were seen initially at unacceptably high levels in test ambient filters collected with STN samplers available at the SOAP monitoring sites. Evaluation and slight modification of the Tisch speciation samplers eliminated the contribution of high background levels of the petroleum-derived n-alkanes. The Tisch samplers also provided PM2.5 samples with sufficient mass for good quantitation of the target organic markers given the higher operating flow rates with this sampler.
Limits of detection (LOD) and limits of quantitation (LOQ) were determined for all organic markers using the current U.S. EPA guidelines. Because the background levels of marker compounds were near or below detection limits (BLD), we developed an alternative method of comparing SOAP blank mass levels with the ambient mass levels for each marker. The target n-alkanes were 12 to 50 times greater in mass compared to the average levels determined for 20 SOAP field and travel blanks. N-alkanoic acids and phthalic acid had lower mass ratios of the average ambient samples to average blanks that ranged from 3 to 7.
Incorporating routine evaluation of field and travel blanks provided good knowledge of the background levels of organic markers. This practice is essential for confirming molecular marker ambient concentrations as well as for bulk carbonaceous fine PM fractions such as elemental (EC) and organic carbon (OC).
Three major outcomes of this effort to establish and validate the SOAP network sample collection procedures are relevant to adapting current monitoring networks and to designing new field studies requiring collection of organic molecular markers. First, greater sensitivity is required for STN samplers to be useful for quantitative marker analysis. Second, shipping filters in sealed containers significantly reduces semivolatile organic contaminants. Third, sampling in the absence of impactor grease or plastic, rubber or polymeric organic carbon sampler components provided sufficiently low travel blanks. This step avoided organic species blank problems that otherwise would have introduced interferences, preventing accurate detection and quantitation of molecular markers. The focus on the molecular level organic constituents benefited OC mass levels in the SOAP field and trip blanks. These results are useful guidelines for designing new sampling or updating current protocols for field measurements of organic marker compounds.
Acknowledgments
The authors thank the New York State Department of Environmental Conservation, the New Jersey State of Environmental Protection, and the Connecticut State Department of Environmental Protection for in-kind support for ambient fine particle collection.
This research was supported by the Speciation of Organics for Apportionment of PM-2.5 (SOAP) through Northeast States for Coordinated Air Use Management (NESCAUM). Additional support from the National Science Foundation Atmospheric Chemistry Grant ATM-0120906 and from New York State Energy and Research Development Authority (NYSERDA) Agreement 7616 is gratefully acknowledged.
The United States Environmental Protection Agency through its Office of Research and Development partially funded the research described here under contract number 4D-5840-NAEX to Rutgers University. It has been subjected to Agency review and approved for publication.
Notes
a Queens is an EPA Supersite.
*Used as SOAP molecular markers.
a GC/MS retention times based on the following program: Total run time of 60.5 minutes with the following steps: 1) initial isothermal hold for 3 minutes at 50°C; 2) a temperature ramp of 20°C /min up to 150°C; 3) isothermal hold for 3 minutes; 4) temperature ramp of 4°C/min to 280°C; and 5) a final isothermal hold of 17 minutes.
a Standard Deviation (STD) calculated as the square root of the mean of the squared deviation values.
b Relative Standard Deviation (RSD) calculated as coefficient of variation = (STD/Mean)*100
c LOD expressed as area ratios to the internal standard n-C24D50 were: hopanes, 0.00073; n-alkanes.
REFERENCES
- American Chemical Society and Committee on Environmental Improvement . 1980 . Guidelines for Data Acquisition and Data Quality Evaluation in Environmental Chemistry . Analyt. Chem. , 52 : 2242 – 2249 .
- Brook , J. R. , Graham , L. , Charland , J. P. , Cheng , Y. , Fan , X. , Lu , G. , Li , S. M. , Lillyman , C. , MacDonald , P. , Caravaggio , G. and MacPhee , J. A. 2007 . Investigation of the Motor Vehicle Exhaust Contribution to Primary Fine Particle Organic Carbon in Urban Air . Atmos. Environ. , 41 ( 1 ) : 119 – 135 .
- Brown , S. G. , Herckes , P. , Ashbaugh , L. , Hannigan , M. P. , Kreidenweis , S. M. and Collett , J. L. 2002 . Characterization of Organic Aerosol in Big Bend National Park, Texas . Atmos. Environ. , 36 ( 38 ) : 5807 – 5818 .
- Cabada , J. C. , Pandis , S. N. and Robinson , A. L. 2002 . Sources of Atmospheric Carbonaceous Particulate Matter in Pittsburgh, Pennsylvania . J. Air Waste Manage. Assoc. , 52 ( 6 ) : 732 – 741 .
- Cass , G. R. 1998 . Organic Molecular Tracers for Particulate Air Pollution Sources . Trac–Trends in Analytical Chemistry , 17 ( 6 ) : 356 – 366 .
- Chen , L. W. A. , Doddridge , B. G. , Dickerson , R. R. , Chow , J. C. and Henry , R. C. 2002 . Origins of Fine Aerosol Mass in the Baltimore–Washington Corridor: Implications from Observation, Factor Analysis, and Ensemble Air Parcel Back Trajectories . Atmos. Environ. , 36 ( 28 ) : 4541 – 4554 .
- Chow , J. C. , Watson , J. G. , Kuhns , H. , Etyemezian , V. , Lowenthal , D. H. , Crow , D. , Kohl , S. D. , Engelbrecht , J. P. and Green , M. C. 2004 . Source Profiles for Industrial, Mobile, and Area Sources in the Big Bend Regional Aerosol Visibility and Observational study . Chemosphere , 54 ( 2 ) : 185 – 208 .
- Chow , J. C. , Watson , J. G. , Lowenthal , D. H. , Chen , L. W. A. , Zielinska , B. , Mazzoleni , L. R. and Magliano , K. L. 2007 . Evaluation of Organic Markers for Chemical Mass Balance Source Apportionment at the Fresno Supersite . Atmos. Chem. Phys. , 7 ( 7 ) : 1741 – 1754 .
- Currie , L. A. 1992 . “ Source apportionment of atmospheric particles ” . In Environmental Analytical and Physical Chemistry Series, Environmental Particles , Edited by: Buffle , J. 3 74 Boca Raton : Lewis Publishers .
- Daisey , J. M. , Hershman , R. J. and Kneip , T. J. 1982 . Ambient Levels of Particulate Organic Matter in New York City in Winter and Summer . Atmos. Environ. , 16 ( 9 ) : 2161 – 2168 .
- Daisey , J. M. , Morandi , M. , Lioy , P. J. and Wolff , G. T. 1984 . Regional and Local Influences on the Nature of Airborne Particulate Organic Matter at Four Sites in New Jersey during summer 1981 . Atmos. Environ. , 18 ( 7 ) : 1411 – 1419 .
- Daisey , J. M. 1980 . Organic Compounds in Urban Aerosols . Ann. NY Acad. Sci. , : 50 – 69 .
- de Gouw , J. A. , Middlebrook , A. M. , Warneke , C. , Goldan , P. D. , Kuster , W. C. , Roberts , J. M. , Fehsenfeld , F. C. , Worsnop , D. R. , Canagaratna , M. R. , Pszenny , A. A. P. , Keene , W. C. , Marchewka , M. , Bertman , S. B. and Bates , T. S. 2005 . Budget of Organic Carbon in a Polluted Atmosphere: Results from the New England Air Quality Study in 2002 . J. Geophys. Res.—Atmos. , 110 ( D16 )
- Fine , P. M. , Cass , G. R. and Simoneit , B. R. T. 2002 . Organic Compounds in Biomass Smoke from Residential Wood Combustion: Emissions Characterization at a Continental Scale . J. Geophys. Res.—Atmos. , 107 ( D21 )
- Fraser , M. P. , Yue , Z. W. and Buzcu , B. 2003 . Source Apportionment of Fine Particulate Matter in Houston, TX, Using Organic Molecular Markers . Atmos. Environ. , 37 ( 15 ) : 2117 – 2123 .
- Fraser , M. P. , Yue , Z. W. , Tropp , R. J. , Kohl , S. D. and Chow , J. C. 2002 . Molecular Composition of Organic Fine Particulate Matter in Houston, TX . Atmos. Environ. , 36 ( 38 ) : 5751 – 5758 .
- Gorin , C. A. , Collett , J. L. and Herckes , P. 2006 . Wood Smoke Contribution to Winter Aerosol in Fresno, CA . J. Air Waste Manage. Assoc. , 56 ( 11 ) : 1584 – 1590 .
- Harkov , R. , Kebbekus , B. , Bozzelli , J. W. and Lioy , P. J. 1983 . Measurement of Selected Volatile Organic Compounds at Three Locations in New Jersey During the Summer Season . J. Air Pollut. Control Assn. , 33 ( 12 ) : 1177 – 1183 .
- Herckes , P. , Engling , G. , Kreidenweis , S. M. and Collett , J. L. 2006 . Particle Size Distributions of Organic Aerosol Constituents During the 2002 Yosemite Aerosol Characterization Study . Environ. Sci. Technol. , 40 ( 15 ) : 4554 – 4562 .
- Herner , J. D. , Ying , Q. , Aw , J. , Gao , O. , Chang , D. P. Y. and Kleeman , M. J. 2006 . Dominant Mechanisms that Shape the Airborne Particle Size and Composition Distribution in Central California . Aerosol Sci. Technol. , 40 ( 10 ) : 827 – 844 .
- Hildemann , L. M. , Mazurek , M. A. , Cass , G. R. and Simoneit , B. R. T. 1991 . Quantitative Characterization of Urban Sources of Organic Aerosol by High–Resolution Gas Chromatography . Environ. Sci. Technol. , 25 ( 7 ) : 1311 – 1325 .
- Keith , L. H. 1991 . Environmental Sampling and Analysis: A Practical Guide , Boca Raton, FL : Lewis Publishers .
- Kleeman , M. J. , Ying , Q. , Lu , J. , Mysliwiec , M. J. , Griffin , R. J. , Chen , J. J. and Clegg , S. 2007 . Source Apportionment of Secondary Organic Aerosol During a Severe Photochemical Smog Episode . Atmos. Environ. , 41 ( 3 ) : 576 – 591 .
- Lee , J. H. , Yoshida , Y. , Turpin , B. J. , Hopke , P. K. , Poirot , R. L. , Lioy , P. J. and Oxley , J. C. 2002 . Identification of Sources Contributing to Mid-Atlantic Regional Aerosol . J. Air Waste Manage. Assoc. , 52 ( 10 ) : 1186 – 1205 .
- Lee , J. H. , Gigliotti , C. L. , Offenberg , J. H. , Eisenreich , S. J. and Turpin , B. J. 2004 . Sources of Polycyclic Aromatic Hydrocarbons to the Hudson River Airshed . Atmos. Environ. , 38 ( 35 ) : 5971 – 5981 .
- Li , M. , McDow , S. R. , Tollerud , D. and Mazurek , M. A. Quantitation, Detection and Measurement Precision of Organic Molecular Markers in Urban Particulate Matter from Philadelphia . PA. Conference Proceedings, AWMA 98th Annual Meeting . June 21–24 , Minneapolis, MN.
- Li , M. , McDow , S. R. , Tollerud , D. and Mazurek , M. A. 2006 . Seasonal Abundance of Organic Molecular Markers in Urban Particulate Matter from Philadelphia, PA . Atmos. Environ. , 40 : 2260 – 2273 .
- Lioy , P. J. and Daisey , J. M. 1983 . The New Jersey Project on Airborne Toxic Elements and Organic Substances (ATEOS): A Summary of the 1981 Summer and 1982 Winter Studies . Journal of the Air Pollution Control Association , 33 ( 7 ) : 649 – 657 .
- Manchester-Neesvig , J. B. , Schauer , J. J. and Cass , G. R. 2003 . The Distribution of Particle-Phase Organic Compounds in the Atmosphere and Their Use for Source Apportionment During the Southern California Children's Health Study . J. Air Waste Manage. Assoc. , 53 ( 9 ) : 1065 – 1079 .
- Mazurek , M. A. 2002 . Molecular Identification of Organic Compounds in Atmospheric Complex Mixtures and Relationship to Atmospheric Chemistry and Sources . Environ. Health Perspect. , 110 : 995 – 1003 .
- Mazurek , M. A. , Cass , G. R. and Simoneit , B. R. T. 1989 . Interpretation of High-Resolution Gas Chromatography and High-Resolution Gas Chromatography/Mass Spectrometry Data Acquired From Atmospheric Organic Aerosol Samples . Aerosol Science Technology , 10 : 408 – 420 .
- Mazurek , M. A. , Cass , G. R. and Simoneit , B. R. T. 1991 . Biological Input to Visibility-Reducing Aerosol Particles in the Remote Arid Southwestern United States . Environ. Sci. Technol. , 25 : 684 – 694 .
- Mazurek , M. A. , Hildemann , L. M. , Cass , G. R. , Simoneit , B. R. T. and Rogge , W. F. 1993 . “ Methods of analysis for Complex Organic Aerosol Mixtures from Urban Emission Sources of Particulate Carbon ” . In Measurement of Airborne Compounds: Sampling, Analysis, and Data Interpretation , American Chemical Society Symposium Series Edited by: Winegar , E. D. 177 – 190 . Boca Raton, FL : CRC Press, Inc. .
- Mazurek , M. , Masonjones , M. C. , Masonjones , H. D. , Salmon , L. G. , Cass , G. R. , Hallock , K. A. and Leach , M. 1997 . Visibility-Reducing Organic Aerosols in the Vicinity of Grand Canyon National Park: Properties Observed by High Resolution Gas Chromatography . J. Geophys. Res.—Atmos. , 102 ( D3 ) : 3779 – 3793 .
- Mazurek , M. A. and Simoneit , B. R. T. 1984 . “ Characterization of Biogenic and Petroleum-Derived Organic Matter in Aerosols Over Remote, Rural and Urban Areas ” . In Identification and Analysis of Organic Pollutants in Air , Edited by: Keith , L. H. 353 – 370 . Boston, MA : Ann Arbor Science/Butterworth .
- Mazurek , M. A. , Simoneit , B. R. T. , Cass , G. R. and Gray , H. A. 1987 . Quantitative High-Resolution Gas Chromatography and High–Resolution Gas Chromatography/Mass Spectrometry Analyses of Carbonaceous Fine Aerosol Particles . Int. J. Environ. Anal. Chem. , 29 : 119 – 139 .
- McDow , S. R. and Huntzicker , J. J. 1990 . Vapor Adsorption Artifact in the Sampling of Organic Aerosol: Face Velocity Effects . Atmos. Environ. , 24A : 2563 – 2571 .
- McMurray , P. H. , Shepherd , M. F. , Vickery , J. S. and NARSTO . 2004 . Particulate Matter Science for Policy Makers: A NARSTO Assessment , New York : Cambridge University Press .
- Offenberg , J. H. , Eisenreich , S. J. , Chen , L. C. , Cohen , M. D. , Chee , G. , Prophete , C. , Weisel , C. and Lioy , P. J. 2003 . Persistent Organic Pollutants in the Dusts that Settled Across Lower Manhattan after September 11, 2001 . Environ. Sci. Technol. , 37 ( 3 ) : 502 – 508 .
- Ondov , J. M. , Buckley , T. J. , Hopke , P. K. , Ogulei , D. , Parlange , M. B. , Rogge , W. F. , Squibb , K. S. , Johnston , M. V. and Wexler , A. S. 2006a . Baltimore Supersite: Highly Time- and Size-Resolved Concentrations of Urban PM2.5 and its Constituents for Resolution of Sources and Immune Responses . Atmos. Environ. , 40 : S224 – S237 .
- Ondov , J. M. , Buckley , T. J. , Hopke , P. K. , Ogulei , D. , Parlange , M. B. , Rogge , W. F. , Squibb , K. S. , Johnston , M. V. and Wexler , A. S. 2006b . Baltimore Supersite: Highly Time– and Size–Resolved Concentrations of Urban PM2.5 and its Constituents for Resolution of Sources and Immune Responses . Atmos. Environ. , 40 : S224 – S237 .
- Ozbay , K. 2006 . Average Daily Number of Motor Vehicles Passing NJ Turnpike Vicinity of Exit 13 (private Communication, Center for Advanced Infrastructure and Transportation, Rutgers, University)
- Pekney , N. J. , Davidson , C. I. , Robinson , A. , Zhou , L. M. , Hopke , P. , Eatough , D. and Rogge , W. F. 2006 . Major Source Categories for PM2.5 in Pittsburgh Using PMF and UNMIX . Aerosol Science and Technology , 40 ( 10 ) : 910 – 924 .
- Ray , J. and McDow , S. R. 2005 . Dicarboxylic Acid Concentration Trends and Sampling Artifacts . Atmos. Environ. , 39 : 7906 – 7919 .
- Rinehart , L. R. , Fujita , E. M. , Chow , J. C. , Magliano , K. and Zielinska , B. 2006 . Spatial Distribution of PM2.5 Associated Organic Compounds in Central California . Atmos. Environ. , 40 ( 2 ) : 290 – 303 .
- Robinson , A. L. , Subramanian , R. , Donahue , N. M. , Bernardo-Bricker , A. and Rogge , W. F. 2006a . Source Apportionment of Molecular Markers and Organic Aerosol. 3. Food cooking emissions . Environ. Sci. Technol. , 40 ( 24 ) : 7820 – 7827 .
- Robinson , A. L. , Subramanian , R. , Donahue , N. M. , Bernardo-Bricker , A. and Rogge , W. F. 2006b . Source Apportionment of Molecular Markers and Organic Aerosols-1. Polycyclic Aromatic Hydrocarbons and Methodology for Data Visualization . Environ. Sci. Technol. , 40 ( 24 ) : 7803 – 7810 .
- Robinson , A. L. , Subramanian , R. , Donahue , N. M. , Bernardo-Bricker , A. and Rogge , W. F. 2006c . Source Apportionment of Molecular Markers and Organic Aerosol. 2. Biomass Smoke . Environ. Sci. Technol. , 40 ( 24 ) : 7811 – 7819 .
- Rogge , W. F. , Hildemann , L. M. , Mazurek , M. A. , Cass , G. R. and Simoneit , B. R. T. 1991 . Sources of Fine Organic Aerosol: 1. Charbroilers and Meat Cooking Operations . Environ. Sci. Technol. , 25 : 1112 – 1125 .
- Rogge , W. F. , Mazurek , M. A. , Hildemann , L. M. , Cass , G. R. and Simoneit , B. R. T. 1993 . Quantification of Urban Organic Aerosols at a Molecular Level: Identification, Abundance and Seasonal Variation . Atmos. Environ. , 27A ( 8 ) : 1309 – 1330 .
- Schauer , J. J. , Rogge , W. F. , Hildemann , L. M. , Mazurek , M. A. and Cass , G. R. 1996 . Source Apportionment of Airborne Particulate Matter Using Organic Compounds as Tracers . Atmos. Environ. , 30 ( 22 ) : 3837 – 3855 .
- Schauer , J. J. and Cass , G. R. 2000 . Source Apportionment of Wintertime Gas-Phase and Particle-Phase Air Pollutants Using Organic Compounds as Tracers . Environ. Sci. Technol. , 34 ( 9 ) : 1821 – 1832 .
- Sheesley , R. J. , Schauer , J. J. , Bean , E. and Kenski , D. 2004 . Trends in Secondary Organic Aerosol at a Remote Site in Michigan's Upper Peninsula . Environ. Sci. Technol. , 38 ( 24 ) : 6491 – 6500 .
- Sihabut , T. , Ray , J. , Northcross , A. and McDow , S. R. 2005 . Sampling Artifact Estimates for Alkanes, Hopanes, and Aliphatic Carboxylic Acids . Atmos. Environ. , 39 : 6945 – 6956 .
- Simoneit , B. R. T. 1984 . Organic Matter of the Troposphere—-III. Characterization and Sources of Petroleum Derived and Pyrogenic Residues in Aerosols Over the Western United States . Atmos. Environ. , 18 ( 1 ) : 51 – 67 .
- Simoneit , B. R. T. 1985 . Application of Molecular Marker Analysis to Vehicular Exhaust for Source Reconciliations . Int. J. Environ. Anal. Chem. , 22 : 203 – 233 .
- Simoneit , B. R. T. 1986 . Characterization of Organic Constituents in Aerosols in Relation to Their Origin and Transport: A Review . Int. J. Environ. Anal. Chem. , 23 : 207 – 237 .
- Simoneit , B. R. T. 1989 . Organic Matter of the Troposphere—-V: Application of Molecular Marker Analysis to Biogenic Emissions into the Troposphere for Source Reconciliations . J. Atmos. Chem. , 8 : 251 – 275 .
- Simoneit , B. R. T. , Mazurek , M. A. and Cahill , T. A. 1980 . Contamination of the Lake Tahoe air Basin by High Molecular Weight Petroleum Residues . Journal Air Pollution Control Association , 30 ( 4 ) : 387 – 390 .
- Simoneit , B. R. T. and Mazurek , M. A. 1982 . Organic Matter of the Troposphere—II. Natural Background of Biogenic Lipid matter in Aerosols Over the Rural Western United States . Atmos. Environ. , 16 ( 9 ) : 2139 – 2159 .
- Solomon , P. S. , Mitchell , W. , Michael , Tolocka , Norris , G. , Gemmill , D. and Wiener , R. 2000 . 1 – 400 . Research Triangle Park, NC : U.S. EPA . Evaluation of PM2.5 Chemical Speciation Samplers for Use in the EPA National PM2.5 Chemical Speciation Network—-Volume I. Introduction, Results, and Conclusions, Edited by Office of Air Quality Planning and Standards
- Subramanian , R. , Donahue , N. M. , Bernardo-Bricker , A. , Rogge , W. F. and Robinson , A. L. 2006a . Contribution of Motor Vehicle Emissions to Organic Carbon and Fine Particle Mass in Pittsburgh, Pennsylvania: Effects of Varying Source Profiles and Seasonal Trends in Ambient Marker Concentrations . Atmos. Environ. , 40 ( 40 ) : 8002 – 8019 .
- Subramanian , R. , Donahue , N. M. , Bernardo-Bricker , A. , Rogge , W. F. and Robinson , A. L. 2006b . Contribution of Motor Vehicle Emissions to Organic Carbon and Fine Particle Mass in Pittsburgh, Pennsylvania: Effects of Varying Source Profiles and Seasonal Trends in Ambient Marker Concentrations . Atmos. Environ. , 40 ( 40 ) : 8002 – 8019 .
- U.S. Environmental Protection Agency . 2004 . 1 – 27 . Research Triangle, NC The Particle Pollution Report, EPA 454–R–04–002, EPA Office of Air Quality Planning and Standards
- U.S. Environmental Protection Agency . 2005 . Air Quality Designations and Classifications for the Fine Particles (PM2.5) National Ambient Air Quality Standards . Federal Register , 70 : 943 – 1019 . Washington, DC, Government Printing Office
- U.S. Environmental Protection Agency . 2003 . Technical Support Document for the Assessment of Detection and Quantitation Approaches: EPA–821–R–03–005 , Washington, DC : U. S. Environmental Protection Agency .
- Ward , T. J. , Rinehart , L. R. and Lange , T. 2006 . The 2003/2004 Libby, Montana PM2.5 Source Apportionment Research Study . Aerosol Sci. Technol. , 40 ( 3 ) : 166 – 177 .
- Yue , Z. W. and Fraser , M. P. 2004a . Characterization of Nonpolar Organic Fine Particulate Matter in Houston, Texas . Aerosol Sci. Technol. , 38 : 60 – 67 .
- Yue , Z. W. and Fraser , M. P. 2004b . Polar Organic Compounds Measured in Fine Particulate Matter During TexAQS 2000 . Atmos. Environ. , 38 ( 20 ) : 3253 – 3261 .
- Zheng , M. , Cass , G. R. , Schauer , J. J. and Edgerton , E. S. 2002 . Source Apportionment of PM2.5 in the Southeastern United States Using Solvent–Extractable Organic Compounds as Tracers . Environ. Sci. Technol. , 36 ( 11 ) : 2361 – 2371 .
- Zheng , M. , Ke , L. , Edgerton , E. S. , Schauer , J. J. , Dong , M. Y. and Russell , A. G. 2006 . Spatial Distribution of Carbonaceous Aerosol in the Southeastern United States using Molecular Markers and Carbon Isotope Data . J. Geophys. Res.—Atmos. , 111 ( D10 ) : 2006
- Zheng , M. , Cass , G. R. , Ke , L. , Wang , F. , Schauer , J. J. , Edgerton , E. S. and Russell , A. G. 2007 . Source Apportionment of Daily Fine Particulate Matter at Jefferson Street, Atlanta, GA, During Summer and Winter . J. Air Waste Manage. Assoc. , 57 ( 2 ) : 228 – 242 .