Abstract
A vacuum ultraviolet (VUV) photoionization aerosol time-of-flight mass spectrometer (VUV-ATOFMS) has been developed for real-time, quantitative chemical analysis of organic particles in laboratory environments. A nozzle of ∼ 0.12 mm orifice combined with an aerodynamic lens assembly and a three stage differential pumping system is used to sample particles at atmospheric pressure. The particles are vaporized on a thermal heater, and then the nascent vapor is photoionized by light generated with a RF-powered VUV lamp. A 0.41 V/cm electric field is used to drive the ions from the ionization region into the ion extraction region where a positive electric pulse repels the ions into a reflectron mass spectrometer. The mass resolution of the spectrometer is ∼ 350 and the detection limit is ∼ 400 μ m 3 . The signal intensities observed are linear with the mass concentration of aerosols. Oleic acid particles are well quantified with an uncertainty of 15% in mass concentrations ranging from 3.9 mg/m 3 to 392 mg/m 3 . The VUV-ATOFMS has substantial potential for the use in laboratory investigations on organic aerosol chemistry.
INTRODUCTION
Urban organic aerosols account for 20–50% of the total fine particle (PM2.5) mass (CitationJacobson et al. 2000; CitationRogge et al. 1993; CitationTurpin et al. 2000), exerting significant influence on the natural environment and human health. In addition to aerosols that form through primary sources, secondary organic aerosols can form by photochemical oxidation reactions of gas-phase trace species (CitationRudich 2003). Therefore, characterization in real time is important to understanding the formation and reaction mechanism of organic aerosols.
Recently, many efforts have been made to obtain the parent ion mass spectra of organic aerosols by coupling vacuum ultraviolet (VUV) photoionization sources with aerosol mass spectrometry (AMS) (CitationCanagaratna et al. 2007; CitationGloaguen et al. 2006; CitationMysak et al. 2005; CitationNorthway et al. 2007; CitationOktem et al. 2004; CitationStreibel et al. 2007; CitationSykes et al. 2002; CitationWoods et al. 2001; CitationWoods et al. 2002). With this technique, the chemical species within complex organic aerosols are easier to identify in real-time. A coherent 118.5 nm laser pulse generated by tripling 355 nm output from a Nd:YAG laser, a synchrotron VUV beam generated from a undulator inserted in the synchrotron electron ring, and radiation from a VUV lamp were reported as ionization sources coupled with AMS (CitationMysak et al. 2005; CitationNorthway et al. 2007; CitationWoods et al. 2001). Among these three VUV light sources, the VUV lamp is the cheapest and the most compact. VUV lamps have been used for analytical chemistry for a long time, although they have had the disadvantages of low photon fluxes and a wide solid angle of radiation. Wieser and coworkers reported a VUV light source which generates radiation by impacting noble gases with an electron beam passing through a 300 nm thick SiNx film (CitationWieser et al. 1997). With this method, a photon flux of 1.9 × 1019 photon/s was achieved. However, this product has not yet been commercialized. An RF-powered VUV lamp is another, relatively new, VUV light source. It can produce radiation with a photon flux of 1017 photon/s (http://resonance.on.ca/RF_Powered_Lamps.html), which is higher than that produced by the synchrotron VUV light source (CitationMysak et al. 2005). With these high photon fluxes, VUV lamps may have the potential to be widely used as VUV ionization sources for AMS.
In this article, we report the construction of a home-built VUV photoionization aerosol time-of-flight mass spectrometer (VUV-ATOFMS) with a RF-powered VUV lamp. Since Northway et al. have previously reported their success in coupling a VUV lamp with AMS (CitationNorthway et al. 2007), here we only present technical information and simple results from our VUV-ATOFMS which may be useful to AMS users. The main challenge with coupling a VUV lamp to an AMS is the numerous photoelectrons emitted from the metal ion optics and the heater tip, which generate huge noise. This problem is overcome by separating the ion extraction region from the ionization region. With this design, the heater tip can be put in any part of the ionization region without producing photoelectron noise and distorting the extraction electric field. Therefore, the vaporization and photoionization efficiencies of particles can be maximized. The details of this design are described in following sections.
DESIGN AND EXPERIMENTAL
The design of the home-built VUV-ATOFMS is similar to the one reported by Shu (CitationShu et al. 2006). A nozzle of ∼ 0.12 mm orifice combined with an aerodynamic lens assembly and a three stage differential pumping system is used to sample particles directly at atmospheric pressure. The aerodynamic lens assembly is machined using the design considerations reported by Zhang (CitationZhang et al. 2002). The flow of sample is ∼ 1.3 cm3 atm s−1, which is calculated taking into account the pumping speed of the turbo pump and the pressure in the chamber. The three stage differential pumping system is composed of three collimated chambers: a source chamber, a differential chamber, and a detection chamber. An aperture with a 3 mm diameter is used to connect the source chamber and the differential chamber, while an aperture with a 4 mm diameter is used to connect the differential chamber and the detection chamber. The distance between the exit of aerodynamic lens assembly and the center of the photoionization region is 178 mm. The source and detection chambers are each pumped by a 320 L/s turbo pump. The differential chamber and the reflectron mass spectrometer are each pumped by a 190 L/s turbo pump. The turbo pumps pumping the source chamber and the differential chamber are backed by an 8.3 L/s rotary pump. The turbo pumps pumping the detection chamber and the reflectron mass spectrometer are backed by a 4 L/s rotary pump. The pressures in all chambers are below 5 × 10−5 Pa without sampling. The pressures in the source chamber and the detection chamber are ∼ 0.4 Pa and ∼ 5 × 10−4 Pa, respectively, during aerosol sampling.
A heater tip used to vaporize organic particles is placed in the detection chamber. The heater tip (capable of temperatures up to 600 K) is an 8 mm diameter copper rod coupled to a cartridge heater driven by a DC power supply. The heater is mounted on a linear translator, which can change the distance between the hot surface and the VUV light. The center of the particle spot deposited on the heater tip is about 1 mm off the center of the copper rod. No adjustment is made to correct for this deviation of the particle beam. The vapor generated from particles is photoionized with light radiated from a RF-powered VUV lamp. The VUV lamp (model KrCM-L) was purchased from Resonance Ltd., Canada in 2005. The photon energy of the main output is 10 eV (123.6 nm, Kr atom resonance line). The total photon current of the VUV radiation measured with a copper panel is about 300 nA, which can roughly be converted to a photon flux of 2 × 1012 photon/s without considering the photoemission efficiency of the copper panel. This value is much lower than the photon flux of 1017 photon/s specified for the latest model of the company. The VUV lamp is coupled to the detection chamber with a conflat flange in a perpendicular direction to the plane defined by the particle beam and the reflectron mass spectrometer. The exit of the VUV lamp is ∼ 55 mm from the particle beam. An f = 50 mm MgF2 lens is located at the exit of the VUV lamp. The VUV radiation profile is measured by detecting the photocurrent emitted from a 4.5 mm (horizontal) × 44 mm (vertical) copper piece which is translated through the photoionization region in 1 mm steps. The solid angle of the VUV radiation output from the VUV lamp is ∼ 0.15 steradians. After addition of the lens and the aperture, the VUV radiation is contracted into a solid angle of ∼ 0.005 steradians, which is equal to a spot with a diameter of ∼ 4 mm in front of the heater tip (55 mm away from the exit of the VUV lamp). The focused and apertured VUV light excites ∼ 44 nA photocurrent, which can be roughly converted into a photon flux of 2.8 × 1011 photon/s.
The schematic diagram of the ion optics is shown in . A 0.41 V/cm electric field is used to drive the ions produced by VUV photoionization into the second stage of ion optics where a positive electric pulse repels the ions into the reflectron mass spectrometer. The setting of the ion optics is critical for design of VUV-TOFMS with a VUV lamp as an ionization source. The divergence of the radiation from a VUV lamp is broad and irregular. It cannot be focused into as small a region as a laser or synchrotron radiation beam. The stray VUV light continuously generates numerous photoelectrons from ion optics plates and the heater tip. These photoelectrons can be accelerated to kinetic energies of hundreds of eV if a pulsed field used to extract ions is applied in the ionization region. The vapor or gas in the photoionization region would be ionized when impacted with these high energy electrons. Consequently, the ions generated by the electron impact ionization would disturb the VUV mass spectra. With the setting of the ion optics shown in , the photoelectrons are quenched on the bottom plate. Another benefit of this design is that the heater tip can be moved into the photoionization region without distorting the electric field for ion extraction. Thus, the vaporization and photoionization efficiencies of particles can be maximized. A positive pulse is provided with a HTS 31 fast high voltage transistor switch (Behlke Electronic GMBH, Germany). The rise time of the positive pulse is ∼ 100 ns and the width is 3 μ s. The repetition rate of the pulse is 12 kHz for this experiment. The reflectron mass spectrometer is characterized by a field free flight distance of 1.4 m, an ion mirror, and a chevron multichannel plate detector. The ion signal is amplified by a 20× amplifier (Ortec VT120C) and recorded with a time-of-flight multiscaler (FAST Comtec, P7888).
The aerosol particles are generated by atomizing a small amount of chemicals in solution. The gas flow for the atomization is controlled by a ball-float flowmeter, such that a ∼ 1.5 l/min flow is maintained in all the experiments. Liquid consumption rates of isopropyl alcohol and ethanol in the experiment are ∼ 0.33 ml/min. Therefore the mass concentration of the aerosols produced can be calculated with the formula c× r/f (c: concentration of the solution; r: liquid consumption rate; f: flow rate of gas).
RESULTS
shows mass spectra of oleic acid and cholesterol particles. The vaporization temperature is 402 K. Each spectrum was collected in 30 s. The particles are produced by atomizing 0.1% (V/V) oleic acid in isopropyl alcohol and the solution of 0.1 g cholesterol in 100 ml ethanol. The average diameter of particles is estimated to be about 100–200 nm. The density of oleic acid is 0.89 g/ml. The mass concentrations of the oleic acid and cholesterol particles produced are 196 mg/m3 and 220 mg/m3, respectively. The formula of oleic acid is C18H34O2 with a molecular weight of 282. The formula of cholesterol is C27H46O with a molecular weight of 386. The peaks at m/z = 282, 280, and 264 in are assigned to C18H34O2 +, C18H32O2 + (H2 loss), and C18H32O+ (H2O loss). Measured with the peak of mass 280, the mass resolution of the mass spectrum shown in is about 350.
FIG. 2 Mass spectra of oleic acid and cholesterol particles. The particles were vaporized at 402 K. and show the mass spectrum of oleic acid and cholesterol particles, respectively. The zoomed plot in presents a detail of the mass spectrum of oleic acid around m/z = 282. Each spectrum was collected in 30 s. The mass concentrations of the oleic acid and cholesterol particles produced are 196 mg/m3 and 220 mg/m3, respectively.
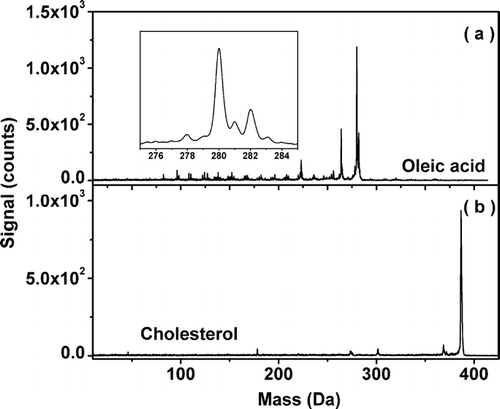
Quantification of aerosol content is a very important requirement for any ATOFMS. We tested this VUV-ATOFMS with oleic acid particles generated from 0.2% to 0.002% oleic acid in isopropyl alcohol solution with the atomizer. shows the experimental data from the measurements. The signal intensities and background are calculated by accumulating the total counts in the range of mass 280 ± 0.5 (see ). The signal intensities and the standard deviation are obtained from 5 measurements at each concentration. The background was measured prior to each signal measurement. The backgrounds listed in increase with particle concentration, which results from the oleic acid film deposited on ion optics by particle vaporization. The cholesterol particles show less residual background in the experiment. shows the dependence of the signal intensity on the mass concentration of oleic acid particles. The linearity of fit is 1.06 ± 0.04, suggesting adequate quantification. Since the maximum standard deviation of the signal data is 8.3% (217 ± 18), the 15% error bars are given to cover the fit line. The larger deviation from the fit line at the higher concentrations may result from sample preparation. The detection limit of the ATOFMS can be estimated with the data shown in and the average random noise measured with the clean chamber (after two days pumping). The average random noise in the range of one atomic mass unit is less than 3 counts measured with the clean chamber, sampling only air. Therefore, with the standard of 3 S/N, the detection limit of this VUV-ATOFMS is estimated to be ∼ 400 μ g/m3. Since the current detection limit is much higher than the level of the organic contents in the ambient aerosols (1–12 μ g/m3 for the urban particulate matter (CitationOktem et al. 2004)), it is impossible to measure ambient organic aerosols directly with the VUV-ATOFMS under the current condition. Nevertheless, the VUV-ATOFMS can be used as a laboratory tool for organic aerosol research, especially after upgrading to a more powerful VUV lamp with better focusing properties.
FIG. 3 Dependence of the signal intensity on the mass concentration of oleic acid particles. The signal intensities are calculated by accumulating the total counts in the range of mass 280 ± 0.5 (see ). The black squares are data points and the line is the result of a linear fit.
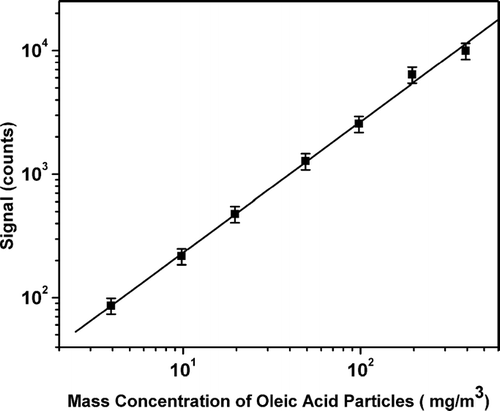
TABLE 1 Experimental data examining the dependence of the signal intensity on the mass concentration of oleic acid particles
CONCLUSIONS
The initial experimental results show that the AMS with a VUV lamp as an ionization source can be used to measure organic aerosols. The VUV-ATOFMS has the capability to quantify the contents of organic aerosols well. Currently, we are investigating soot formed in coal combustion with this VUV-ATOFMS. The mass spectra of soot obtained are quite satisfactory. Although the current detection limit is not sensitive enough to measure ambient organic aerosols, the VUV-ATOFMS has the potential to be used in laboratories for investigations into aerosol chemistry.
Acknowledgments
This research was supported by funding from the National Natural Science Foundation of China (Grant No. 20673138), funding for Creative Research Groups of China (Grant No. 50621804), and funding for scientific instrumentation development from the Chinese Academy of Science.
Notes
*C, S, SD, and B in stand for concentration, signal intensity, standard deviation, and background.
REFERENCES
- Canagaratna , M. R. , Jayne , J. T. , Jimenez , J. L. , Allan , J. D. , Alfarra , M. R. , Zhang , Q. , Onasch , T. B. , Drewnick , F. , Coe , H. , Middlebrook , A. , Delia , A. , Williams , L. R. , Trimborn , A. M. , Northway , M. J. , DeCarlo , P. F. , Kolb , C. E. , Davidovits , P. and Worsnop , D. R. 2007 . Chemical and microphysical characterization of ambient aerosols with the aerodyne aerosol mass spectrometer . Mass Spectrom. Rev. , 26 : 185 – 222 .
- Gloaguen , E. , Mysak , E. R. , Leone , S. R. , Ahmed , M. and Wilson , K. R. 2006 . Investigating the chemical composition of mixed organic-inorganic particles by “soft” vacuum ultraviolet photoionization: The reaction of ozone with anthracene on sodium chloride particles . Int. J. Mass Spectrom. , 258 : 74 – 85 .
- Jacobson , M. C. , Hansson , H. C. , Noone , K. J. and Charlson , R. J. 2000 . Organic atmospheric aerosols: Review and state of the science . Rev. , 38 : 267 – 294 .
- Mysak , E. R. , Wilson , K. R. , Jimenez-Cruz , M. , Ahmed , M. and Baer , T. 2005 . Synchrotron radiation based aerosol time-of-flight mass spectrometry for organic constituents . Anal. Chem. , 77 : 5953 – 5960 .
- Northway , M. J. , Jayne , J. T. , Toohey , D. W. , Canagaratna , M. R. , Trimborn , A. M. , Akiyama , K.-I. , Shimono , A. , Jimenez , J. L. , DeCarlo , P. F. , Wilson , K. R. and Worsnop , D. R. 2007 . Demonstration of a VUV lamp photoionization source for improved organic speciation in an aerosol spectrometer . Aerosol Sci. Technol. , 41 : 828 – 839 .
- Oktem , B. , Tolocka , M. P. and Johnston , M. V. 2004 . On-line analysis of organic components in fine and ultrafine particles by photoionization aerosol mass spectrometry . Anal. Chem. , 76 : 253 – 261 .
- Resonance Ltd. http://resonance.on.ca/RF_Powered_Lamps.html
- Rogge , W. F. , Mazurek , M. A. , Hildemann , L. M. , Cass , G. R. and Simoneit , B. R. T. 1993 . Quantification of Urban Organic Aerosols at a Molecular-Level—Identification, Abundance and Seasonal-Variation . Atmos. Environ. a-Gen , 27 : 1309 – 1330 .
- Rudich , Y. 2003 . Laboratory perspectives on the chemical transformations of organic matter in atmospheric particles . Chem. Rev. , 103 : 5097 – 5124 .
- Shu , J. N. , Wilson , K. R. , Ahmed , M. and Leone , S. R. 2006 . Coupling a versatile aerosol apparatus to a synchrotron: Vacuum ultraviolet light scattering, photoelectron imaging, and fragment free mass spectrometry . Rev. Sci. Instrum. , 77 043106
- Streibel , T. , Mitschke , S. , Adam , T. , Weh , J. and Zimmermann , R. 2007 . Thermal desorption/pyrolysis coupled with photo ionisation time-of-flight mass spectrometry for the analysis and discrimination of pure tobacco samples . J. Anal. Appl. Pyrol. , 79 : 24 – 32 .
- Sykes , D. C. , Woods , E. , Smith , G. D. , Baer , T. and Miller , R. E. 2002 . Thermal vaporization-vacuum ultraviolet laser ionization time-of-flight mass spectrometry of single aerosol particles . Anal. Chem. , 74 : 2048 – 2052 .
- Turpin , B. J. , Saxena , P. and Andrews , E. 2000 . Measuring and simulating particulate organics in the atmosphere: problems and prospects . Atmos. Environ. , 34 : 2983 – 3013 .
- Wieser , J. , Murnick , D. E. , Ulrich , A. , Huggins , H. A. , Liddle , A. and Brown , W. L. 1997 . Vacuum ultraviolet rare gas excimer light source . Rev. Sci. Instrum. , 68 : 1360 – 1364 .
- Woods , E. , Smith , G. D. , Dessiaterik , Y. , Baer , T. and Miller , R. E. 2001 . Quantitative detection of aromatic compounds in single aerosol particle mass spectrometry . Anal. Chem. , 73 : 2317 – 2322 .
- Woods , E. , Smith , G. D. , Miller , R. E. and Baer , T. 2002 . Depth profiling of heterogeneously mixed aerosol particles using single-particle mass spectrometry . Anal. Chem. , 74 : 1642 – 1649 .
- Zhang , X. F. , Smith , K. A. , Worsnop , D. R. , Jimenez , J. , Jayne , J. T. and Kolb , C. E. 2002 . A numerical characterization of particle beam collimation by an aerodynamic lens-nozzle system: Part I. An individual lens or nozzle . Aerosol Sci. Technol. , 36 : 617 – 631 .