Abstract
A one-year (from June 2003 to May 2004) study of airborne microbial concentration and size distribution was conducted systematically at three selected sampling sites in Beijing. Microbial samples were collected in triplicate for 3 min, 3 times per day, for 3 consecutive days of each month using FA-I sampler (imitated Andersen sampler, made by Applied Technical Institute of Liaoyang, China). Results showed that the concentration of total culturable microorganisms ranged from 4.8 × 10 2 colony forming units (CFU)/m 3 to 2.4 × 10 4 CFU/m 3 , with an arithmetic mean of 3.7 × 10 3 ± 2.1 × 10 2 (standard deviation) CFU/m 3 . As a whole, the percentage of airborne bacteria, accounting for 59.0% of the total culturable microorganisms, was significantly higher than those of airborne fungi (35.2%) and actinomycetes (5.8%). At RCEES (Research Center for Eco-Environmental Sciences—a culture and education area) and XZM (Xizhimen—a main traffic line), significantly higher percentages of airborne bacteria were found as compared to airborne fungi. No significant difference was observed between airborne bacteria and fungi at BBG (Beijing Botanical Garden—a green garden area). The total microbial concentration was significantly higher at RCEES than at XZM and BBG (*P < 0.05), and no significant difference between XZM and BBG was observed (P > 0.05). Total microbial concentrations were higher in summer and autumn, and lower in spring and winter at RCEES and XZM (*P < 0.05). At BBG, higher concentration was observed in summer during the sampling periods (***P < 0.001). The size distribution of airborne bacteria showed a skewed distribution at three sampling sites. The proportion of bacterial particles decreased gradually from Stage 1 ( > 7.0 μm) to Stage 6 (0.65 ∼ 1.1 μm), and there was an obvious downtrend on Stage 2 (4.7 ∼ 7.0 μm). Bacterial particles were mainly distributed at the first four Stages ( > 2.1 μm), accounting for 78.6% at RCEES, 84.0% at XZM, and 81.5% at BBG. The size distribution pattern of airborne fungi was found with normal logarithmic distribution at three sampling sites. Fungal particles were mainly distributed at Stage 3 (3.0 ∼ 6.0 μ m), Stage 4 (2.0 ∼ 3.5 μ m) and Stage 5 (1.0 ∼ 2.0 μ m), composing 71.6% of the population at RCEES, 74.2% at XZM, and 68.3% at BBG. However, different distribution patterns were found in different dominant fungal genera. Cladosporium, Penicillium, and Aspergillus were present with normal logarithmic distribution, while Alternaria and sterile mycelia were found with skewed distribution. In opposition to the distribution pattern of fungal particles, the actinomycete particles at all sampling sites were primarily collected on Stage 1, Stage 5, and Stage 6, accounting for 61.1% at RCEES, 60.7% at XZM, and 64.8% at BBG.
INTRODUCTION
The presence of airborne microbes and their relationship to disease has become an increasingly important area of study as many bioaerosols in indoor and outdoor environments have been found to cause adverse health effects (CitationDen Boer et al. 2002; CitationDouwes et al. 2003; CitationDouwes et al. 2000). Bioaerosols defined as airborne particles consisting of or originating from microorganisms (bacteria, viruses, molds), for example metabolites, toxins, or fragments of micro-organisms are associated with respiratory diseases and increased mortality and morbidity (CitationLee et al. 2006a). Elevated microbial concentrations in the air have much possibility of resulting in infectious diseases (CitationDen Boer et al. 2002; CitationMahoney et al. 1992), respiratory diseases (CitationOstro et al. 2001; CitationVogelzang et al. 1998), and cancer (CitationKhuder et al. 1998). In the atmosphere, airborne fungi exist primarily in the form of spores. Bacteria are attached to the surface of dust particles (CitationChe, 2004). The particle size of anemophilous plant pollen is 17–58 μ m, 1–30 μ m for fungal spores, and 0.25 μ m for individual bacterial particles (CitationGregory 1973; CitationStanley et al. 1974). CitationLee et al. (2006a) reported that composition, concentration, and size distribution of airborne microorganisms affects respiratory deposition and ensuing health effects. Concerning fungi, larger spores (> 10 μ m) have been shown to deposit in the upper airway (e.g., nose and pharynx) while smaller spore particles (< 5 μ m) can penetrate the lower airways leading to hay fever symptoms and other allergic reactions/asthma, respectively. Fungal particles in the submicrometer range (< 1 μ m) have been shown to be able to penetrate into the deepest parts of the respiratory tract (CitationHorner et al. 1995). Airborne microorganisms have a specific aerodynamic size and respiratory diseases are significantly impacted by particle size and deposition. Therefore, in order to correlate human disease, it is critical to measure the size distribution of bioaerosols in different environments to which people are exposed.
Numerous studies have been conducted in order to determine the species composition and concentration distribution of airborne microorganisms in outdoor environments (CitationKlaric and Pepeljnjak 2006; CitationLee et al. 2006b; CitationLighthart and Shaffer 1995; CitationShaffer and Lighthart 1997; CitationTong and Lighthart 1999; CitationTong and Lighthart 2000; CitationZoppas et al. 2006). The most commonly reported airborne bacteria genera are Bacillus, Micrococcus, Staphylococcus, and Pseudomonas (CitationMancinelli and Shulls 1978; CitationShaffer and Lighthart 1997), whereas Cladosporium, Alternaria, Penicillium, Aspergillus species dominate the fungal composition of outdoor atmospheric environments (CitationEl-Morsy 2006; CitationFang et al. 2005). The concentration of microbes in the air is affected by several factors, including daily and seasonal meteorological variations, vegetation, air pollution, agricultural, industrial, and other human activity (CitationKlaric and Pepeljnjak 2006; CitationLevetin and Dorsey 2006; CitationLin and Li 2000; CitationPasanen 1992).
One important parameter affecting microbial exposures is the particle size (CitationMeklin et al. 2002). The size of microbial particles may also vary depending on the age of the spores and the nutrient in the growth medium (CitationEllis 1981), or due to physical conditions (CitationPasanen et al. 1991). However, most previous studies in aerobiology have focused primarily on microbial composition and concentration in the atmosphere in different environments, and few studies have evaluated particle size distribution of airborne microorganisms in outdoor environments. In this study, a year-round investigation of microbial concentration and size distribution in the air of three selected sampling sites was carried out in outdoor environments of Beijing. The aim of this study was to determine the airborne microbial concentration and particle size distribution in outdoor environments in Beijing, Data presented herein indicate the need to control environmental pollution and thereby prevent human respiratory disease as a result of outdoor atmospheric exposures.
MATERIALS AND METHODS
Sampling Sites
Research Center for Eco-Environmental Sciences (RCEES), Chinese Academy of Sciences, was selected to investigate bioaerosol of cultural and educational area. RCEES is a research institute of environment and ecology employing more than 300 employees. It is situated in the Haidian district approximately 17 km from the city center. Green vegetation, including poplar, birch and some virescence grass, contributes to about 50% of the total area. Laboratory buildings, office buildings, and several concrete roads are found in the courtyard where sampling was performed. Personnel pass through the area with relatively high frequency and there is little vehicle traffic in the courtyard. The sampling point was located above the grass at a point 20 m from the main research building.
Xizhimen (XZM), located in the Xicheng district near the city center, was selected to represent bioaerosol sampling in a heavy vehicle traffic area. XZM is a traffic junction with green area amounting to no more than 5% vegetation coverage. The sampling point, which was at the side of a two-lane, two-way road with a major route from south to the north, was located on an asphalt ground near an apartment building. There exists a overbridge near the sampling location. Traffic was fairly steady throughout the day except the rush hour. There was a high frequency of vehicles (total 150 vehicles per min flow of traffic) and considerable air pollution. Traffic consisted primarily of buses, cars and occasional pickup trucks.
Beijing Botanical Garden (BBG) was selected to represent bioaerosol sampling in a green garden area. It is located on the Haidian district over 30 km from the city center, with 95% total green area. There are several kinds of trees, shrubbery, and many herbaceous plants. The sampling point was on the grassy area, about 30 m away from several trees. Near the sampling point, there are almost no buildings and asphalt roads. Vehicles and personnel were rare near the sampling point.
Sampler Introduction
Culturable bioaerosols were collected at each location using a six-stage culturable FA-I sampler (imitation Andersen sampler) designed and constructed by Applied Technical Institute of Liaoyang, China. The FA-I was based on the Andersen impaction sampler commonly used in bioaerosol studies. The sampler consisted of air pump, flow meter, and glass plate. Each stage of the sampler includes a plate with 400 tiny holes of uniform diameter. Air was drawn at a rate of 28.3 liters per min and impacted onto Petri dishes containing agar media specific for the class of airborne microorganisms cultured. Airborne particles were separated into six size fractions: > 7.0 μ m (Stage 1), 4.7∼ 7.0 μ m (Stage 2), 3.3∼4.7 μm (Stage 3), 2.1∼3.3 μ m (Stage 4), 1.1∼2.1 μ m (Stage 5), and 0.65∼1.1 μ m (Stage 6) ().
TABLE 1 Characteristics of FA-I sampler
Sampling Methods and Culture Medium
Bioaerosol samples (airborne bacteria, fungi, and actinomycete) were collected from June 2003 to May 2004 at three typical sites in Beijing. At each sites, the sampler was set up with a platform at a height representative of the normal human breathing zone (1.5 m above floor level). Because of much effects of weather condition on airborne microbial type, number and size distribution, sunny days were selected for sampling throughout the year for consistency. Culturable bioaerosol samples were collected in triplicate for three minutes each at three times (9:00, 13:00, and 17:00) during the sampling day. This was performed three consecutive days of each month. For each sampling point, the FA-I sampler was loaded with 90-mm Petri dishes containing suitable culture medium as described below.
Nutrient agar (3 g beef extract, 10 g peptone, 5 g sodium chloride, 15 g agar, 1,000 ml distilled water, pH = 7.2) was used for the sampling and cultivation of airborne bacteria. Sabouraud agar (40 g glucose, 10 g peptone, 20 g agar, 1000 distilled water, pH = 6.2, 0.1 mg/ml chloramphenicol added to inhibit bacterial growth) was used for fungi. Gause's synthetic agar (20 g soluble starch, 1 g KNO3, 0.5 g NaCl, 0.5 g K2HPO4 3H2O, 0.5 g MgSO47H2O, 0.01 g FeSO47H2O, 20 g agar, 1000 ml distilled water, pH = 7.2, 0.01% potassium dichromate added to inhibit bacterial and fungal growth) was used for cultivation of actinomycetes. After sampling, bacterial samples were incubated at 37°C for 48 h, and fungal samples at 25°C for 72 h. Actinomycete samples were incubated at 30°C for 120 h.
Microbial Identification
After incubation, microbial colonies were counted. Fungi were identified microscopically according to the morphology of the observed hyphae, conidia, and sporangia. Fungi subcultured onto malt extract agar (MEA) that did not develop sporulating structures after 14 days were described as “mycelia isolates” (CitationWei 1979).
Sample Analysis
Colony forming units (CFU) on each plate were counted following incubation. Concentration was expressed as CFU per cubic meter of air (CFU/m3). However, because there was colony superposition when the microbial particles impact the same spot through the same sieve pore, the enumerated colony data were revised by Equation (Equation1) according to co-manufacturer recommendation (CitationHu et al. 1997). Total bacterial, fungal, and actinomycete concentration on different stage (Stage 1 to Stage 6) of the sampler were calculated using Equations (2), (3), and (4), respectively. Bacterial, fungal and actinomycete concentration was calculated using Equations (5), (6), and (7), respectively. The total microbial concentration was calculated using Equation (Equation8). Bacterial, fungal, and actinomycete concentration percentage was calculated using Equations (9), (10), and (11), respectively.
Data Analysis and Statistical Analysis
All the experiment data (3 locations × 3 days × 3 time course × 12 months = 324 samples), which were collected in triplication from June 2003 to May 2004, were analyzed using Microsoft Office Excel 2003 and SPSS Version 10.0 (SPSS. Inc., Standard Version). All the figures were made by Microsoft Office Excel 2003. One-way analysis of variance (ANOVA) was used to compare the difference between different sampling sites and times with SPSS Version 10.0.
RESULTS
Airborne Microbial Concentration Distribution
Background Concentration of Airborne Microorganisms
Variation in the concentration of airborne microbes was found in this study (). The concentration range of total culturable microorganisms ranged from 4.8 × 102 to 2.4× 104 CFU/m3 with an arithmetic mean concentration of 3.7× 10 3± 2.1 × 102 CFU/m3. Bacterial concentrations ranged from 7.1 × 10 CFU/m3 to 2.2 × 104 CFU/m3 with an arithmetic mean concentration of 2.2 × 103± 1.2 × 102 CFU/m3 (CitationFang et al. 2007). Fungal concentrations ranged from 2.4 × 10 CFU/m3 to 1.4 × 104 CFU/m3, and the arithmetic mean concentration was 1.2 × 103 ± 7.3 × 10 CFU/m3 (CitationFang et al. 2005). The actinomycete concentrations, attributing little to the total concentrations, ranged from 0 to 9.8 × 102 CFU/m3, with an arithmetic mean concentration of 1.4 × 102± 8.0 CFU/m3. Actinomycete were significantly lower than those of airborne bacteria and fungi (***P < 0.001).
TABLE 2 Microbial concentration background in the Beijing atmosphere (CFU/m3); containing three sampling sites; June 2003–May 2004
Concentration of Airborne Bacteria, Fungi, and Actinomycete
At RCEES and XZM, significantly higher percentages of airborne bacteria were found when compared to those of airborne fungi (***P < 0.01) (). No significant difference was observed between airborne bacterial and fungal concentration at BBG (P > 0.05). With regard to airborne actinomycetes, the percentage was no more than 6.5% for all sampling sites. Overall, the percentage of airborne bacteria, accounting for 59.0% of the total microorganisms, was significantly higher than those of airborne fungi (35.2%) and actinomycetes (5.8%) in the three tested atmospheres in Beijing. At different sampling sites, bacterial percentage was highest at XZM and lowest at BBG (*P < 0.05), while the fungal percentage was highest at BBG and lowest at XZM (*P < 0.05).
Spatial Variation Pattern of Total Microbial Concentration
Total microbial concentration varied greatly at different sampling sites. Significantly higher total concentration was observed at RCEES than at XZM and at BBG (*P < 0.05). No significant differences were found between XZM and BBG (P > 0.05).
Seasonal Variation Pattern of Total Microbial Concentration
Total microbial concentrations were higher in summer and autumn, and lower in spring and winter at RCEES and at XZM (*P < 0.05), with the highest concentration observed in autumn (). At BBG, higher microbial concentration was observed in summer during the sampling periods (*P < 0.05).
Temporal Variation Pattern of Total Microbial Concentration
Significantly higher total microbial concentrations were recorded at 09:00 and 17:00 than those at 13:00 for all three sampling time at different sampling sites () (*P < 0.05).
Concentration and Particle Size Distribution of Airborne Microorganisms
Concentration and Particle Size Distribution of Airborne Bacteria
The concentration and particle size distribution data of airborne bacteria at different sampling sites are shown in . The mean bacterial concentration was significantly lower at BBG than at RCEES and XZM at every stage of the sampler (**P < 0.01). However, there were no substantial differences in size distribution found at the three sampling sites. The particle size distribution data demonstrated a skewed distribution at the three sampling sites. The percentage of bacterial particles decreased gradually from Stage 1 to Stage 6 and there was an observed obvious downtrend on Stage 2. The bacterial particles were mainly distributed among the first four Stages (> 2.1μ m), accounting for 78.6% at RCEES, 84.0% at XZM, and 81.5% at BBG. The highest percentage of culturable bacteria was recorded on Stage 1, where 23.5% (RCEES), 27.0% (XZM), and 25.3% (BBG) of the total concentration of culturable bacteria were collected. The lowest percentage was found on stage 6 (**P < 0.01) with 6.1% (RCEES), 6.9% (XZM), and 7.2% (BBG), respectively.
Concentration and Particle Distribution of Airborne Fungi
Significantly higher fungal concentrations were observed at RCEES and BBG on every stage of the sampler as compared to XZM () (**P < 0.01) With respect to the particle size distribution of airborne fungi, the same pattern was found with normal logarithmic distribution at all three sampling sites. Fungal particles were collected mainly on Stage 3 (3.0 ∼ 6.0 μ m), Stage 4 (2.0 ∼ 3.5μ m) and Stage 5 (1.0 ∼ 2.0μ m), composing 71.6% of the total airborne fungi at RCEES, 74.2% at XZM, and 68.3% at BBG. The highest percentage of culturable fungi was detected on stage 4 (2.0–3.5 μ m), where it was 28.0% (RCEES), 36.2% (XZM), and 29.5% (BBG). The lowest values were associated with Stage 6 (< 1.0 μ m; **P < 0.01) for which the percentage were 5.0%, 4.5%, and 5.6% at RCEES, XZM, and BBG, respectively. For the remaining stages, the percentages varied from 10.0% to 27.6% at RCEES, 9.9% to 20.7% at XZM, and 12.6% to 20.6% at BBG.
FIG. 5 Concentration and size distribution of airborne fungi at three sampling sites in Beijing, China; June 2003–May 2004.
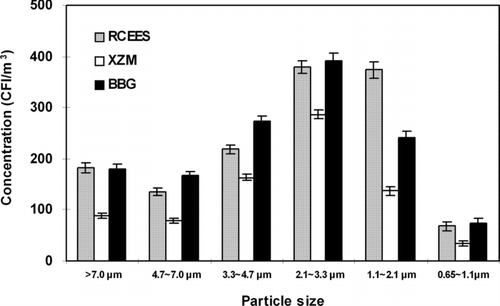
The concentration and size distribution patterns of dominant airborne fungi at different sampling sites were showed in . Concentrations of Cladosporium and Alternaria were significantly lower at XZM than at RCEES and BBG at every stage of the sampler (**P < 0.01), while no significant differences in airborne Penicillium and Aspergillus species concentration were observed among the three sites (P > 0.05). Sterile mycelia had higher concentrations at BBG than at RCEES and XZM at every stage of the sampler.
FIG. 6 Concentration and size distribution of dominant fungi at three sampling sites in Beijing, China; June 2003–May 2004.
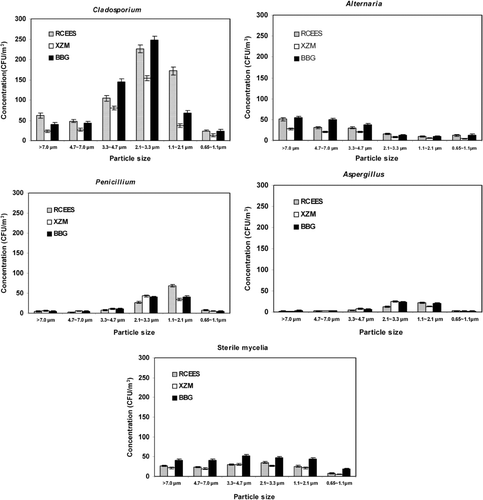
The size distribution patterns showed high consistency at the three sites. However, different distribution patterns were observed among different fungal genera (). Cladosporium, Penicillium, and Aspergillus were presented with normal logarithmic distribution, while Alternaria and sterile mycelia exhibited a skewed distribution. Cladosporium species were detected most often on Stages 3–5, accounting for more than 80% of total Cladosporium on six stages. The highest percentage was recorded on Stage 4, contributing to 36.1% at RCEES, 46.2% at XZM, and 43.6% at BBG, while the lowest percentage was found on Stage 6, accounting for 3.6% (RCEES), 3.9% (XZM), and 4.2% (BBG). Penicillium and Aspergillus species were collected primarily on Stage 4 and 5, accounting for approximately 75% of the total Penicillium and Aspergillus on six stages. Alternaria species and sterile mycelia were observed mainly on the first three (> 3.0 μ m), and five stages (> 1.0 μ m) of the sampler, respectively, and contributed to approximately 80 and 90% of the total collected fungi.
Particle Size Distribution of Airborne Actinomycetes
No significant differences were found in actinomycete concentration among the three sampling sites () (P > 0.05). Unlike the fungal distribution, most of the actinomycete particles at all sampling sites were collected on Stage 1, 5, and 6, accounting for 61.1% at RCEES, 60.7% at XZM, and 64.8% at BBG. Relatively uniform percentages were detected on Stages 2–4 at the three sampling sites, ranging from 10% to 14%.
DISCUSSION
To further understand the relationship between airborne microorganisms and human disease, we must first identify the microbial background, including the type, concentration, and size distribution of microorganisms in the atmosphere. To meet this end, many surveys of airborne microorganisms in various environments have been carried out over the past several decades (CitationEl-Morsy 2006; CitationHerrero et al. 2006; CitationKarwowska 2005; CitationKlaric and Pepeljnjak 2006; CitationLee et al. 2006b; CitationMancinelli and Shulls 1978; CitationMedrela-Kuder 2003; CitationPady and Kelly 1953; CitationShaffer and Lighthart 1997; CitationShelton et al. 2002). In this study, airborne microbial concentration and size distribution analysis were conducted over the course of one year at three sites in Beijing, China. Results showed that the mean concentration of total microorganisms was 3.7 × 103, which was less than the reported values in other cities in China (Xie et al. 2004). This may be attributed to dissimilar meteorological and environmental conditions in different cities, and varying sampling methods used by the researchers. Compared to other cities in the world however, the microbial concentration in the atmosphere was higher in Beijing (CitationDiGiorgio et al. 1996). The high microbial concentrations may be a reflection of China's recent economic growth in the past several decades.
Microbial concentration in the air depends on both internal biological factors and on external environmental factors such as temperature, relative humidity, and rainfall (CitationLarsen 1981). In Beijing, higher microbial concentrations in the atmosphere were detected at RCEES and XZM in summer and autumn, when the temperature and humidity provided the optimum conditions for the exacerbation of airborne microorganisms. Low temperatures in winter and spring, and the absence of rainfall in winter in Beijing, which is located in the northern part of China, may have contributed to the low observed microbial concentrations in the atmosphere during these seasons (CitationJones and Harrison 2004; CitationLi and Kendrick 1995).
The concentration of airborne bacteria was significantly higher than airborne fungi and actinomycetes at the tested sites in Beijing. Bacterial concentrations were highest at XZM and lowest at BBG (*P < 0.05), comprising about 60%, 69%, and 49% of the detected microorganisms at RCEES, XZM, and BBG, respectively. Significantly lower bacterial counts were found on every stage of the sampler at BBG as compared to the other sampling sites. These differences may have been caused by environmental conditions such as vegetation coverage and difference in personnel and vehicle traffic at the three sampling sites. A positive correlation between bacterial concentration in the atmosphere and ground disturbance has been shown; conversely, a negative correlation has been shown with vegetation coverage (CitationJu and Suo et al. 2003; CitationXie et al. 1999). At BBG, personnel and vehicle flow and human activities are infrequent while the vegetation coverage is more than 95%. Plants can leach bacteria from the atmosphere (CitationJu and Suo et al. 2003), and they can also kill bacteria by secreting volatile bactericidal products (CitationXie et al. 1999). This may have been the reason for the higher observed airborne bacterial counts were observed at RCEES and XZM versus at BBG. However, the major causative factors differed between the two sampling sites. At RCEES, human activities and personnel traffic were likely the leading factors whereas vehicle traffic may account for high bacterial concentrations at XZM.
In this study, significantly lower fungal concentrations were detected on every stage of the sampler at XZM versus the other two sampling sites. The same result was found by CitationJu et al. (2003) who studied the airborne microbes at different functional areas with natural precipitation method in Lanzhou, China. The results showed that the fungal concentrations in the greenbelt were 12 folds higher than in the traffic line. It was reported that the variation of airborne fungal concentration and type in outdoor environments may be attributed to human activities and type of vegetation (CitationAwad 2005; CitationPasanen 1992), and that the majority of airborne fungal spores come from vegetation rather than from soil. According to Awad's results, vegetation is one of the most important sources of fungal air contamination (CitationAwad 2005). During vigorous plant growth the leaf can provide a surface for fungal proliferation there by contributing greatly to the concentration of air fungal spores (CitationLevetin and Dorsey 2006). The plant phylloplane or leaf surface, which is a habitat characterized by complex interactions between the host plant and phyllosphere microorganisms, can provide nutrients exuded from the leaf (CitationInacio et al. 2002), and allow for the growth of saprophytic and parasitic fungi (CitationPicco and Rodolfi 2000). Various plants can be infected with fungal plant pathogens Fusarium and Alternaria, which can be dispersed into the air. Vegetation was considered the main source of Alternaria by CitationAwad (2005), which is consistent with our results that significantly higher concentration of Alternaria was observed at the sampling sites with more vegetation coverage compared to the sampling site with less vegetation coverage at every stage of the sampler. In addition to nutrient levels, plant growth, and the abundance of phylloplanes, fungi are also influenced by environmental factors such as water availability, UV radiation, and temperature (CitationNewsham et al. 1997) (CitationSundin 2002). Aspergillus, Penicillium, and Cladosporium were considered to be closely related to local microenvironments and urbanization (CitationAwad 2005). Many studies on airborne fungi showed that an increase in fungal spore concentration was seen at air temperatures between 15 and 25°C and relative humidity at 60–70% (CitationPepeljnjak et al. 2003). Temperature and humidity extremities may result in decreased airborne fungal spore concentration (CitationLevetin and Horowitz 1978).
Natural and anthropogenic sources, such as plants and vegetables (CitationLindemann et al. 1982; CitationLindemann and Upper 1985), soil (CitationLighthart and Shaffer 1994), water bodies (CitationTeltsch and Katzenelson 1978), and human activities (CitationLighthart 1984; CitationWilson et al. 2002; CitationZucker et al. 2000; CitationMartinez et al. 1988) are regarded as the predominant sources of airborne microorganisms in outdoor environments. Traffic flow (both human and vehicle) and vegetation coverage are usually considered to be the main contributing factors in concentration and particle size distribution of airborne microorganisms in outdoor environments (CitationSeino et al. 2005; CitationJu et al. 2003). We hypothesized that there were significant differences in concentration and size distribution of airborne microorganisms at three representative sampling sites (a culture and education area, a main traffic junction, and a lush, green garden area). However, our results demonstrated that the size distribution pattern at the three sites was similar. Airborne bacteria exhibited a skewed distribution, with the highest percentage of total culturable bacteria on stage 1 (> 7.0 μ m). This result was consistent to the size distribution pattern of airborne bacteria in Beijing and other cities. CitationHu et al. (1990) showed that the percentage of bacterial particles decreased gradually from Stage 1 to Stage 6, but without the obvious downtrend on Stage 2 that we observed. In the present study, the total percentage of bacteria detected on the first four stages (> 2.1 μ m) at XZM was significantly higher than at RCEES and BBG. This was most likely due to the increased presence of vehicle and personnel flow at XZM, which may have resulted in the disturbance of small soil particles and dust near the sampling atmosphere. It is known that airborne bacteria attach to the surface of such particles. This may have lead to the increase of large size bacterial particles at XZM (CitationChe 2004). This information provides us an idea that highly trafficked areas are more detrimental to human respiratory health close to large bacterial particles (> 2.1 μ m), and effective actions need to be taken to minimize the risk at the highly trafficked area in the urban.
This study on size distribution of airborne fungi at three sampling sites showed that the distribution patterns were similar for RCEES, XZM, and BBG. It presented a log-normal distribution, which was in accordance with the previous studies of CitationHu et al. (1990) in Beijing. The only difference was that the highest percentage of airborne fungi was in the 2.0 ∼ 3.5 μ m range in our study versus the 3.0 ∼ 6.0 μ m range reported by Hu et al. The results indicate that fungal size distribution patterns in Beijing did not change considerably with the urban development of the past of 15 years, except that the particle size of the largest group of detected organisms decreased.
Although no significant difference in size distribution patterns of total airborne fungi was found in this study, different fungal genera had different size distribution patterns. Cladosporium, Penicillium, and Aspergillus showed normal logarithmic distribution, while Alternaria and sterile mycelia were present with a skewed distribution. This distribution may have been affected by the different sources of various fungi (CitationReponen et al. 1992), and by meteorological and environmental factors such as air temperature and moisture at different sampling sites. Interestingly, a similar distribution pattern was observed between total airborne fungi and Cladosporium. The distribution pattern of airborne actinomycete was totally opposite to that of airborne fungi; the reason for this is unknown and warrants further research.
In summary, distribution patterns observed for airborne bacteria, fungi, and actinomycetes in Beijing were unique and require further research. It has been reported that the size and shape of fungal particles may be very important in determining their specific biological responses (CitationOberdorster et al. 1992). Therefore, for future investigations, it will be important to find the potential relationships between size distribution patterns of bioaerosols and associated human respiratory diseases. The distribution patterns of differing types of microbes might have a close relationship to the microbial source in nature. Therefore, microbial source characteristics at different sampling sites should be investigated in detail. Similarly, future bioaerosol sampling studies need to be performed under a variety of environmental conditions in order to assess the impact of the environment on microbial bioaerosol patterns and associated human health risks.
CONCLUSION
In this study, the concentration and size distribution patterns of airborne microorganisms were studied in three representative Beijing areas. From the results, we can conclude that: (1) As a whole, the concentration percentage of airborne bacteria (59.0%) was significantly higher than those of airborne fungi (35.2%) and actinomycetes (5.8%); (2) Significantly lower concentration of total airborne microorganisms were detected at sampling sites consisting of high traffic or increased vegetations while higher concentrations were observed at the sites consisting of both high frequency human activities and lush vegetation; (3) In a year, total microbial concentration in the air was higher in summer and autumn, but lower in spring and winter. In a single day, higher concentration was found at 09:00 and 17:00 than 13:00; (4) Different type of airborne microbes has different size distribution patterns; and (5) Different distribution patterns were found in different dominant fungal genera. Cladosporium, Penicillium, and Aspergillus were present with normal logarithmic distribution, while Alternaria and sterile mycelia were found with skewed distribution.
Acknowledgments
This study was financially supported by the Project of Knowledge Innovation of the Chinese Academy of Sciences (KZCX2-YW-422, KZCX3-SW-424). The authors would like to gratefully acknowledge PatriciaWaldron from the Institute for Environmental Genomics (IEG), Department of Botany and Microbiology, University of Oklahoma in the United States of America who made valuable technical and grammatical revisions for this article. The authors also wish to thank the other graduate students and teachers who provided their valuable suggestions in our research group and in the State Key Laboratory of Urban and Regional Ecology, Research Center for Eco-Environmental Sciences, Chinese Academy of Sciences, Beijing, 100085, China.
REFERENCES
- Awad , A. H. A. 2005 . Vegetation: A Source of Air Fungal Bio-Contaminant . Aerobiologia , 21 : 53 – 61 .
- Che , F. 2004 . The Principle and Application of Airborne Microbiology , 1 – 41 . Beijing : Science Press . (in Chinese)
- Den Boer , J. W. , Yzerman , E. P. F. , Schellekens , J. , Lettinga , K. D. , Boshuizen , H. C. , Van Steenbergen , J. E. , Bosman , A. , Van den Hof , S. , Van Vliet , H. A. Peeters , M. F. 2002 . A Large Outbreak of Legionnaires' Disease at a Flower Show, the Netherlands, 1999 . Emerging Infectious Diseases , 8 : 37 – 43 .
- DiGiorgio , C. , Krempff , A. , Guiraud , H. , Binder , P. , Tiret , C. and Dumenil , G. 1996 . Atmospheric Pollution by Airborne Microorganisms in the City of Marseilles . Atmos. Environ. , 30 : 155 – 160 .
- Douwes , J. , Thorne , P. , Pearce , N. and Heederik , D. 2003 . Bioaerosol Health Effects and Exposure Assessment: Progress and Prospects . Annals of Occupational Hygiene , 47 : 187 – 200 .
- Douwes , J. , Wouters , I. , Dubbeld , H. , van Zwieten , L. , Steerenberg , P. , Doekes , G. and Heederik , D. 2000 . Upper Airway Inflammation Assessed by Nasal Lavage in Compost Workers: A Relation with Bio-Aerosol Exposure . Amer. J. Indust. Med. , 37 : 459 – 468 .
- El-Morsy , E. S. M. 2006 . Preliminary Survey of Indoor and Outdoor Airborne Microfungi at Coastal Buildings in Egypt . Aerobiologia , 22 : 197 – 210 .
- Ellis , J. J. 1981 . The Effect of Medium, Temperature, and Age on Rhizopus Delemar Sporangiospore Size . Mycologia , 73 : 362 – 368 .
- Fang , Z. , Ouyang , Z. , Hu , L. , Wang , X. , Zheng , H. and Lin , X. 2005 . Culturable Airborne Fungi in Outdoor Environments in Beijing, China . Sci. Total Environ. , 350 : 47 – 58 .
- Fang , Z. G. , Ouyang , Z. Y. , Zheng , H. , Wang , X. K. and Hu , L. F. 2007 . Culturable Airborne Bacteria in Outdoor Environments in Beijing, China . Microb. Ecol. , 54 : 487 – 496 .
- Gregory , P . 1973 . Microbiology of the Atmosphere . J. Aerosol Sci. , 4 ( 4 ) : 339
- Herrero , A. D. , Ruiz , S. S. , Bustillo , M. G. and Morales , P. C. 2006 . Study of Airborne Fungal Spores in Madrid, Spain . Aerobiologia , 22 : 135 – 142 .
- Hu , Q. X. , Xu , G. Q. Xu , X. Z. 1990 . Size Distribution of Airborne Microbial Particle . Environmental Science in China , 10 ( 2 ) : 99 – 102 . (in Chinese)
- Hu , Q. X. , Xu , X. Z. and Jiang , L. 1997 . Effect of Sampling Time on Sampling Efficiency of Airborne Microbes . Shanghai Environmental Sciences , 16 ( 5 ) : 17 – 20 .
- Horner , W. E. , Helbling , A. , Salvaggio , J. E. and Lehrer , S. B. 1995 . Fungal Allergens . Clin. Microbiol. Rev. , 8 : 161 – 179 .
- Inacio , J. , Pereira , P. , de Carvalho , M. , Fonseca , A. , Amaral-Collaco , M. T. and Spencer-Martins , I. 2002 . Estimation and Diversity of Phylloplane Mycobiota on Selected Plants in a Mediterranean-Type Ecosystem in Portugal . Microbial. Ecol. , 44 : 344 – 353 .
- Jones , A. M. and Harrison , R. M. 2004 . The Effects of Meteorological Factors on Atmospheric Bioaerosol Concentrations—A Review . Sci. Total Environ. , 326 : 151 – 180 .
- Ju , T. Z. , Suo , A. N. Tian , Y. J. 2003 . Analysis on Aerobiologia in Lanzhou . Ind. Saf. Environ. Pot. , 29 : 17 – 19 . (in Chinese)
- Karwowska , E. 2005 . Microbiological Air Contamination in Farming Environment . Polish J. Environ. Studies , 14 : 445 – 449 .
- Khuder , S. A. , Mutgi , A. B. and Schaub , E. A. 1998 . Meta-Analyses of Brain Cancer and Farming . Amer. J. Indust. Med. , 34 : 252 – 260 .
- Klaric , M. S. and Pepeljnjak , S. 2006 . A Year-Round Aeromycological Study in Zagreb Area, Croatia . Annals of Agricult. & Environ. Med. , 13 : 55 – 64 .
- Larsen , L. S. 1981 . A 3-Year Survey of Micro-Fungi in the Air of Copenhagen 1977–79 . Allergy , 36 : 15 – 22 .
- Lee , S. A. , Adhikari , A. , Grinshpun , S. A. , McKay , R. , Shukla , R. and Reponen , T. 2006a . Personal Exposure to Airborne Dust and Microorganisms in Agricultural Environments . J. Occupat. & Environ. Hyg. , 3 : 118 – 130 .
- Lee , T. , Grinshpun , S. A. , Martuzevicius , D. , Adhikari , A. , Crawford , C. M. and Reponen , T. 2006b . Culturability and Concentration of Indoor and Outdoor Airborne Fungi in Six Single-Family Homes . Atmos. Environ. , 40 : 2902 – 2910 .
- Levetin , E. and Dorsey , K. 2006 . Contribution of Leaf Surface Fungi to the Air Spora . Aerobiologia , 22 : 3 – 12 .
- Levetin , E. and Horowitz , L. 1978 . One-Year Survey of Airborne Molds of Tulsa, Oklahoma. 1. Outdoor Survey . Annals of Allergy , 41 : 21 – 24 .
- Li , D. W. and Kendrick , B. 1995 . A Year-Round Study on Functional-Relationships of Airborne Fungi with Meteorological Factors . Intl. J. Biometeorol. , 39 : 74 – 80 .
- Lighthart , B. 1984 . Microbial Aerosols—Estimated Contribution of Combine Harvesting to an Airshed . Appl. & Environ. Microbiol. , 47 : 430 – 432 .
- Lighthart , B. and Shaffer , B. T. 1994 . Bacterial Flux from Chaparral into the Atmosphere in Midsummer at a High Desert Location . Atmos. Environ. , 28 : 1267 – 1274 .
- Lighthart , B. and Shaffer , B. T. 1995 . Airborne Bacteria in the Atmospheric Surface-Layer—Temporal Distribution above a Grass Seed Field . Appl. & Environ. Microbiol. , 61 : 1492 – 1496 .
- Lin , W. H. and Li , C. S. 2000 . Associations of Fungal Aerosols, Air Pollutants, and Meteorological Factors . Aerosol Sci. Technol. , 32 : 359 – 368 .
- Lindemann , J. , Constantinidou , H. A. , Barchet , W. R. and Upper , C. D. 1982 . Plants as Sources of Airborne Bacteria, Including Ice Nucleation-Active Bacteria . Applied and Environmental Microbiology , 44 : 1059 – 1063 .
- Lindemann , J. and Upper , C. D. 1985 . Aerial Dispersal of Epiphytic Bacteria over Bean-Plants . Appl. & Environ. Microbiol. , 50 : 1229 – 1232 .
- Mahoney , F. J. , Hoge , C. W. , Farley , T. A. , Barbaree , J. M. , Breiman , R. F. , Benson , R. F. and Mcfarland , L. M. 1992 . Community-Wide Outbreak of Legionnaires-Disease Associated with a Grocery Store Mist Machine . Journal of Infectious Diseases , 165 : 736 – 739 .
- Mancinelli , R. L. and Shulls , W. A. 1978 . Airborne Bacteria in an Urban-Environment . Applied and Environmental Microbiology , 35 : 1095 – 1101 .
- Martinez , K. , Sheehy , J. Jones , J. 1988 . Microbial Containment Conventional Fermentation Processes . Appl. Ind. Hyg. , 3 : 177 – 181 .
- Medrela-Kuder , E. 2003 . Seasonal Variations in the Occurrence of Culturable Airborne Fungi in Outdoor and Indoor Air in Cracow . International Biodeterioration & Biodegradation , 52 : 203 – 205 .
- Meklin , T. , Reponen , T. , Toivola , M. , Koponen , V. , Husman , T. , Hyvarinen , A. and Nevalainen , A. 2002 . Size Distributions of Airborne Microbes in Moisture-Damaged and Reference School Buildings of Two Construction Types . Atmospheric Environment , 36 : 6031 – 6039 .
- Newsham , K. K. , Low , M. N. R. , McLeod , A. R. , Greenslade , P. D. and Emmett , B. A. 1997 . Ultraviolet-B Radiation Influences the Abundance and Distribution of Phylloplane Fungi on Pedunculate Oak (Quercus robur) . New Phytologist , 136 : 287 – 297 .
- Oberdorster , G. , Ferin , J. , Gelein , R. , Soderholm , S. C. and Finkelstein , J. 1992 . Role of the Alveolar Macrophage in Lung Injury—Studies with Ultrafine Particles . Environmental Health Perspectives , 97 : 193 – 199 .
- Ostro , B. , Lipsett , M. , Mann , J. , Braxton-Owens , H. and White , M. 2001 . Air Pollution and Exacerbation of Asthma in African-American Children in Los Angeles . Epidemiology , 12 : 200 – 208 .
- Pady , S. M. and Kelly , C. D. 1953 . Numbers of Fungi and Bacteria in Transatlantic Air . Science , 117 : 607 – 609 .
- Pasanen , A. L. 1992 . Airborne Mesophilic Fungal Spores in Various Residential Environments . Atmospheric Environment Part A—General Topics , 26 : 2861 – 2868 .
- Pasanen , A. L. , Pasanen , P. , Jantunen , M. J. and Kalliokoski , P. 1991 . Significance of Air Humidity and Air Velocity for Fungal Spore Release into the Air . Atmospheric Environment Part A—General Topics , 25 : 459 – 462 .
- Pepeljnjak , S. and Šegvic , M. 2003 . Occurrence of Fungi in Air and on Plants in Vegetation of Different Climatic Regions in Croatia . Aerobiologia , 19 : 11 – 19 .
- Picco , A. M. and Rodolfi , M. 2000 . Airborne Fungi as Biocontaminants at Two Milan Underground Stations . International Biodeterioration & Biodegradation , 45 : 43 – 47 .
- Reponen , T. , Lehtonen , M. , Raunemaa , T. and Nevalainen , A. 1992 . Effect of Indoor Sources on Fungal Spore Concentrations and Size Distributions . J. Aerosol Sci. , 23 : 663 – 666 .
- Seino , K. , Takano , T. , Nakamura , K. and Watanabe , M. 2005 . An Evidential Example of Airborne Bacteria in a Crowded, Underground Public Concourse in Tokyo . Atmospheric Environment , 39 : 337 – 341 .
- Stanley , R. and Linskins , H. 1974 . Pollen: Biology, Chemistry and Management , Berlin : Springer-Verlag .
- Sundin , G. W. 2002 . “ Ultraviolet Radiation on Leaves: Its Influence on Microbial Communities and Their Adaptations ” . In Phyllosphere Microbiology , Edited by: Lindow , S. E. , Hecht-Poinar , E. I. and Elliott , V. J. 27 – 42 . St. Paul : APS Press .
- Shaffer , B. T. and Lighthart , B. 1997 . Survey of Culturable Airborne Bacteria at Four Diverse Locations in Oregon: Urban, Rural, Forest, and Coastal . Microbial Ecology , 34 : 167 – 177 .
- Shelton , B. G. , Kirkland , K. H. , Flanders , W. D. and Morris , G. K. 2002 . Profiles of Airborne Fungi in Buildings and Outdoor Environments in the United States . Appl. & Environ. Microbiol. , 68 : 1743 – 1753 .
- Teltsch , B. and Katzenelson , E. 1978 . Airborne Enteric Bacteria and Viruses from Spray Irrigation with Wastewater . Appl. & Environ. Microbiol. , 35 : 290 – 296 .
- Tong , Y. Y. and Lighthart , B. 1999 . Diurnal Distribution of Total and Culturable Atmospheric Bacteria at a Rural Site . Aerosol Sci. Technol. , 30 : 246 – 254 .
- Tong , Y. Y. and Lighthart , B. 2000 . The Annual Bacterial Particle Concentration and Size Distribution in the Ambient Atmosphere in a Rural Area of the Willamette Valley, Oregon . Aerosol Sci. Technol. , 32 : 393 – 403 .
- Vogelzang , P. F. J. , van der Gulden , J. W. J. , Folgering , H. , Kolk , J. J. , Heederik , D. , Preller , L. , Tielen , M. J. M. and van Schayck , C. P. 1998 . Endotoxin Exposure as a Major Determinant of Lung Function Decline in Pig Farmers . Amer. J. Respir. & Critical Care Med. , 157 : 15 – 18 .
- Wilson , S. C. , Morrow-Tesch , J. , Straus , D. C. , Cooley , J. D. , Wong , W. C. , Mitlohner , F. M. and McGlone , J. J. 2002 . Airborne Microbial Flora in a Cattle Feedlot . Appl. & Environ. Microbiol. , 68 : 3238 – 3242 .
- Wei , J. C. 1979 . Fungal Identification Handbook , Shanghai : Shanghai Science and Technology Press .
- Xie , H. L. , Li , S. R. Yuan , X. Y. 1999 . Study on the Disinfection of Plant Volatile Secretion to the Microorganism Content in the Air . Acta Agriculturae Universitatis Henanensia , 33 ( 2 ) : 127 – 133 . (in Chinese)
- Zoppas , B. C. D. , Valencia-Barrera , R. M. , Duso , S. M. V. and Fernandez-Gonzalez , D. 2006 . Fungal Spores Prevalent in the Aerosol of the City of Caxias Do Sul, Rio Grande Do Sul, Brazil, over a 2-year Period (2001–2002) . Aerobiologia , 22 : 119 – 126 .
- Zucker , B. A. , Trojan , S. and Muller , W. 2000 . Airborne Gram-Negative Bacterial Flora in Animal Houses . Journal of Veterinary Medicine Series B—Infectious Diseases and Veterinary Public Health , 47 : 37 – 46 .